- 1Department of Computer Science, DHA Suffa University, Karachi, Pakistan
- 2University of the West of Scotland, Paisley, United Kingdom
- 3Department of Computer Science, Institute of Business Administration, Karachi, Pakistan
- 4Trinity College Dublin and National University of Ireland, Maynooth, Ireland
Social distancing has been regarded as a key method by the authorities worldwide to manage the pandemic of COVID-19. Digital technologies play a crucial role to support the social, professional and economic activities when people are forced to stay locked-down in their homes. Internet of things (IoT) technologies have a track of providing high quality remote health care and automation services which could guarantee social distancing while maintaining health and well-being of populations. In this paper, we propose an end-to-end IoT architecture to support the social distancing in the event of pandemic. The architecture comprises of the major use cases of IoT in relevance with the COVID-19. Furthermore, we also present a short-term and long-term strategy to mange the social distancing methodology using the proposed IoT architecture. The challenges associated with each layer of architecture have been highlighted and design guidelines have been presented to deal with them.
Introduction
The global pandemic of Coronavirus Disease 2019 (COVID-19) outbreak emerged from the city of Wuhan, China in December 2019. Since then, the virus has spread to 213 countries and territories around the world and two international conveyances with confirmed 11,869,734 cases and death toll reaching 544,147 at the time of writing this article (Worldometer, 2020). Previous instances of emergence of other forms of coronavirus includes the 2002–2004 severe acute respiratory syndrome coronavirus (SARS-CoV) outbreak (Chan-Yeung and Xu, 2003) and the middle east respiratory syndrome coronavirus (MERS-CoV) infection of 2012 (Abeler et al., 2020). Coronaviruses are spherical RNA viruses with protein spikes protruding from the surface giving it a crown like look. It is extremely contagious with 1–14 days incubation period (a time during which the patients exhibit no symptoms); technically termed as SARS-CoV-2 or β-coronavirus.
Background
COVID-19 patients develop mild to moderate (fever, cough, fatigue, sputum production, sore throat, headache, chills) or severe (myalgia, shortness of breath, nausea, nasal congestion, diarrhea, haemoptysis, conjunctival congestion) or critical (bluish lip/face, acute respiratory distress syndrome (ARDS), acute heart injury, secondary infection, multiple organ dysfunction syndrome (MODS)) form of the disease (WHO, 2020f). However, older adults (people above 60 years) and people with existing medical conditions like diabetes, hypertension, asthma etc. are at higher risk of getting infected and developing critical conditions due to COVID-19 (CDC, 2020d).
COVID-19 being highly contagious, it is absolutely important to prevent and restrict the transmission mechanism. Though the actual propagation mechanism remain elusive, analysis has revealed the spread of respiratory droplets to be the primary reason behind transmission of infection from symptomatic people to asymptomatic ones. Infected surfaces (especially steel and plastic where the virus survive up to 3 days, or cupboard, where the lifespan is up to 24 h, or even copper, where it can live up to 4 h) are another reason for transmission of infection if a person comes in direct or indirect contact with them. Once inside the human body, the virus travels through the nasal passage, mucus membranes, cell membranes, up to the bronchial tubes, lungs and the host’s air-sacs (Belluck, 2020).
The COVID-19 pandemic is not the only global outbreak experienced by the human world till date. There have been past insurgencies of the global outbreaks of flu, caused by different types of influenza viruses; specifically 1) Spanish Flu (1918–1919) caused by the avian origin H1N1 virus resulting in a death-toll in excess of 50 millions (CDC, 2020a), 2) Asian Flu (1957–1958) caused by the avian-origin H2N2 virus yielding a global death toll of up to 1.1 million, 3) Hong Kong Flu (1968–1969) caused by H3N2 virus giving rise to a sporadic death toll of one million worldwide with more fatalities among people above the age of 65 years and 4) Swine Flu (2009–2010) caused by pork-origin H1N1 Type A virus ensuing into a death toll of 151,700 globally (CDC, 2020b). While the previous pandemics are caused by influenza viruses, COVID-19 is caused by coronavirus, previous versions of which have only spawned into major outbreaks like SARS (number of deaths is 774) and MERS (number of deaths is 871 as of April 2020) (CDC, 2020c).
The outbreak of COVID-19 in a particular country can be mapped into four incremental stages according to World Health Organization (WHO) (WHO, 2020b). This mapping has been formulated in order to facilitate a common code of understanding among all countries of the world. The four stages include; Stage I - Imported Cases: When the infection is usually limited to people who has recently travelled to or from an already infected zone, Stage II - Local Transmission: When people who are already infected transmit to people coming in close contact with them, Stage III - Cluster of Cases: the infection starts propagating within a geographical location infecting people without any travel history or close encounter with another infected individual, Stage IV - Community Transmission: Larger outbreaks of local transmission in a country leading to an excruciatingly high number of infected cases and deaths.
In order to limit transmission of infection, the outbreak of COVID-19 has given birth to a new concept of “social distancing” or physical distancing, which is defined as maintaining a distance of at least six feet (2 m) between people. The requirement of social distancing has resulted in of all non-essential services at a large scale world-wide. Hence, the financial growth and survival of several economic sectors has been severely affected due to the sudden drop of sales in automotive industry, high cancellation rate with the aviation and tourism industry, leading to collapse in prices with the oil industry, decline in real-estate demand and supply chain disruption in the food industry. While the above-mentioned arenas suffered from inactivity, the healthcare and medical sector took a heavy toll due to excessive activity and unprecedented pressure. Furthermore, the telecommunication industry also experienced a massive increase in traffic and bandwidth consumption owing to and everybody working from home (Goodman, 2020).
Motivation and Objectives
The main step towards handling COVID-19 include testing of symptomatic individuals, quarantine and treatment measures for infected individuals and adopting preventive measures to inhibit further spread of infection. The two principal ways of testing COVID-19 infection, as recommended by WHO, include, contact tracing (identifying people with a history of exposure to infected individuals) and clinical tests. Clinical tests can be conducted in two different but reliable ways; molecular tests based on WHO recommended Nucleic Acid Amplification Test (NAAT) (WHO, 2020e) and serological tests that detect the presence of antibodies in the bloodstream of the individual under clinical test (Hahn, 2020). However, serological test fails to detect the disease during its early days of the body building antibodies. Regarding treatment, there exists no definitive treatment or preventive vaccine to combat coronavirus at the time of writing this article. Till date, around 82 candidate vaccines have been tested in pre-clinical stage (WHO, 2020d), seven of which are under clinical evaluation, a few worth-mentioning are Adenovirus Type five Vector, ChAdOx1, mRNA, DNA plasmid vaccine with electroporation and LNP encapsulated mRNA (Park, 2020). Several potential drugs are also under trial for treating coronavirus at different stages; some worth-noting are Arbidol, Favipiravir, Remdesivir, Lapinavir/Ritonavir, Tocilizumale and RhACE2 APN01 (WHO, 2020g).
In absence of a reliable treatment or vaccine available, WHO has outlined some effective preventive measures that can minimize the likelihood of getting infected or becoming a casualty, if infected. The most important ones, worth-mentioning, include, cleaning hands frequently with water, soap or sanitizer, practicing social distancing, staying indoors in accustomed environment, avoiding touching eyes, nose and mouth without cleaning hands, disinfecting frequently touched surfaces, covering faces while coughing/sneezing and wearing face masks (WHO, 2020c).
It has always been very hard to contain pandemics such as COVID-19 because of their human-to-human transmissions. Another reason is that a person who is carrier of the virus may not show any symptom for 14 days (which is the incubation period for the virus), during which he may transmit the virus to a large number of people. According to Centers for Disease Control and Prevention (CDC), the best way to contain the disease is to maintain a safe social distance (Armitage and Nellums, 2020). Isolation of the identified cases and their contact has been identified as another major strategy to control the spread. Therefore, full or partial lock-down has been implemented by nearly all countries to slow-down and prevent the spread of COVID-19. The digital technologies have been helping the governments and individuals to maintain the social distancing through offering the tools such as Zoom/Microsoft Teams for online work and education, Facetime/Facebook for online connectivity with friends and family (Armitage and Nellums, 2020), and video streaming services such as Netflix for entertainment.
Simultaneously, a plethora of technologies have emerged for mitigating the impact of COVID-19, the major ones worth-mentioning include Internet-of-Things (IoT), Artificial Intelligence (AI), blockchain and next generation communication networks like 5G, beyond 5G (Ting et al., 2020). For example, IoT can provide efficient services of remote health monitoring and automation of facilities which could support the requirements of social distancing. AI can aid in disease surveillance, risk prediction, medical diagnosis and screening, virus modelling and analysis, host identification, enforcing measures and curative research for effectively controlling the COVID-19 pandemic related crisis (Garza, 2020). On the other hand, blockchain technology can contribute significantly towards facilitating increased testing and reporting, recording details of patients, managing implementation, enabling incentive-based volunteer participation and secure donation platforms and limiting supply chain disruptions during the pandemic (Alladi et al., 2019). Besides, together with other concomitant technologies like IoT and AI, 5G networks can empower the healthcare sector with technologies like, Picture Archiving and Communication Systems (PACS), thermal imaging for remote real-time diagnosis of COVID-19 patients as well as common control and monitoring platform for integrating IoT based telemedicine, robots, drones and medical sensing applications (Huawei, 2020).
IoT is going to transform our lives over the next few years and can be the principal ammunition for fighting the pandemic situation while sustaining norms of social distancing and self-quarantine. It is to be noted that it may take years to eliminate the pandemic of COVID-19 fully. In this context, it may be noted that the 10th wave of Ebola took nearly 6 years (2014–2020) to be completely vanish from West Africa (WHO, 2020a). Therefore, it seems to be the right time to capitalize in the IoT solutions which could offer a better management opportunity to deal with COVID-19. IoT solutions can be applied to monitor and transmit health information to the concerned healthcare professionals by using wearables or through ambient monitoring of the environments, track medication orders and monitor patients from a remote location. It can facilitate clinicians to evaluate, diagnose and treat patients without any physical interaction through application of IoT-based telemedicine platforms. Activities like crowd surveillance, public announcements, screening masses, spraying disinfectants, delivery of medical supplies and other essentials can be executed with minimum human interaction through the use of drones, where the drones are controlled through an IoT platform (Marr, 2020). Robots can be deployed to assist in treatment of patients and alleviate stress levels of healthcare workers, while autonomous vehicles (AV) can perform delivery while mitigating the risk of virus transmission where robots and AV’s are controlled and monitored through an IoT platform (Spice, 2020).
Critical IoT or healthcare-related IoT is referred to as the Internet of Medical Things (IoMT). With IoMT, an amalgamation of all kinds of medical devices like smart wearables, smart thermometers, etc. and software applications for controlling them, it is possible to create personalized health plans, telemonitor vital signs in a patient’s body and trigger an alarm with aberration in the vital measurements, remote visit and consultation between patients and clinicians or two clinicians, as well as remote surgery in emergency conditions, all executed while maintaining social distancing. Other supporting technologies also contribute towards different aspects of handling the COVID-19 situation. For example, Geographic Information System (GIS) can help in comprehending the origins of the outbreak, identifying high-risk areas. Bluetooth can be used in contact tracing and Global Positioning System (GPS) can be used in monitoring the real-time as well as historical locations of COVID-19 patients (TechRadar, 2020).
Motivated by the stringent requirements of social distancing imposed worldwide as a management strategy for COVID-19 pandemic and the recent success of IoT based technologies in providing high-quality remote health-care services, we concentrate our efforts towards,
• Preparing a detailed literature review focused on the specific use cases resulted due to the lockdown and social distancing measures of COVID-19.
• Developing an end-to-end IoT architecture for supporting the day to day activities of people including early diagnosis, remote patient monitoring, quarantine monitoring, food and medicine delivery etc.
• Proposing a combination of long term and short strategy for managing the impacts of COVID-19 through using innovative IoT solutions
• Suggesting the protocols pertaining to each layer of IoT architecture to support the COVID-19 use cases and articulating the challenges associated with implementation of IoT solutions in the serge of pandemic and to suggest way to deal with these challenges
Research Contribution and Methods
The primary contribution of this paper is three fold. Firstly, we provide an extensive yet collective review of the present and proposed use of IoT technologies for different applications like early diagnosis, remote patient monitoring, quarantine monitoring, food and medicine delivery etc., in support of preventive measures like social distancing and strict lock-down, commensurate with COVID-19 pandemic situation. Towards this end, we have conducted a detailed literature review. We conducted detailed web search to identify the innovative applications for which IoT solutions have been used during COVID-19; we came across various interesting applications such as using connected thermometers for early diagnosis and remote monitoring and using wrist bands for quarantine monitoring and sending preventive alerts.
Secondly, we propose an end-to-end IoT architecture, for facilitating the day-to-day social and professional activities during the pandemic crisis, with a combination of 1) short-term response of activating preventive alerts within seconds of recorded body parameters falling out-of-range and 2) long-term strategy encompassing collection of vital parameters over time, remote consultation, contact tracing, quarantine monitoring, food and medicine delivery, remote behavioral monitoring and emergency response, if needed.
Thirdly, we propose specific protocols for each layer in the IoT architecture for facilitating the pandemic related applications. In order to achieve that, we have conducted a review of existing protocols functioning at various IoT layered architecture; in the light of findings, we proposed the protocols to be used at each layer (including MQTT, AMQP or COAP for applicaiton layer; TCP or UDP for transport layer, IPv4 or IPv6 for network and WiFi, 5G (URLLC), LTE-M, Bluetooth, LTE-M, NB-IoT, Sigfox, LoRa, Zigbee, 802.15.4 or NB-IoT for MAC layer. The protocol suggestions have been made in line with the requirements of environment and application. We conclude the paper by identifying the challenges associated with the use of IoT technologies for managing pandemic and recommending methods to deal with them.
Rest of this paper has been organized as follows: IoT So-Far in Managing Social-Distancing presents a comprehensive review of use cases of different IoT applications during pandemic; Proposed End-To-End IOT Architecture for Managing Social-Distancing describes the proposed IoT architecture for supporting social-distancing along with the design guidelines for developing and implementing protocols at each layer of IoT architecture; Expected Challenges and Future Research Directions sheds light on the expected challenges for the deployment of proposed architecture and also suggest future research directions; Limitations details the limitations of present study and finally, Conclusion concludes the article.
IoT So-Far in Managing Social-Distancing
Here, we provide a summary on the use of IoT networks so-far for major applications that are existing as well as emerging owing to the pandemic situation (pictorially depicted in Figure 1). Moreover, the most common applications already emerged or expected to emerge due to COVID-19 have also been summarized in Table 1. This table details the environments, applications in each environment and the IoT sensors being used to collect data about different physical parameters which could help to monitor and manage the pandemic both at personal and organizational levels.
Early Diagnosis
The use of IoT based smart helmet for detecting the presence of fever in people arriving at the public spaces has been proposed by (Doffman, 2020) to replace time consuming infrared thermometers since the outbreak of COVID-19. The officer in charge wears the helmet, equipped with thermal camera, optical camera, GPS and LWPAN modules to identify the COVID-19 suspects. The thermal camera identifies the temperatures of surrounding objects. As soon as someone is detected with a high fever, the GPS tracker locates the person, optical camera captures the picture and an alert is sent to the mobile app of the officer through appropriate wireless link such as LTE-M. The image processing technologies are subsequently used to reveal the identification of person by connecting to centralized databases. Similarly, smart glasses equipped with thermal and optical cameras have also been proposed to diagnose the cases of COVID-19 among the crowds (Estimote, 2020). Hence, using IoT, it would become possible to identify the people suspected for COVID-19 without needing to come in close contact with them.
Remote Patient Monitoring
The conventional role of Body Area Networks (BAN) (a use case of IoT) has been to provide the remote health monitoring facility to the patients. The use of implanted/wearable or ambient sensors for monitoring and reporting the body parameters to the remote stations has been in use globally (Gupta et al., 2020). Often IoT solutions are coupled with other cutting-edge technologies such as smart homes, virtual assistants and specific medical sensors to ensure early diagnosis and timely delivery of the required healthcare service (Australian-Government, 2020). In addition to the wearable sensors, the IoT devices deployed at the bedside or wheelchairs of patients are also being used to monitor and transmit valuable information about the patient health state which could reduce the risk of diseases transmission to the visitors and staff at hospitals. Similarly, Ambient Assisted Living is an emerging concept where an ecosystem of different platforms including sensors, computers, mobile devices and software applications has been developed for telehealth facilities (Marques, 2019). One of the major focuses on AAL solutions is on providing safe and healthy life to independently living elderly. WiFi based sensors have been proposed not only for precisely locating the people in their indoor environments, but also for detecting relative positions of the part of their bodies to track their activities (Cai et al., 2019).
The remote monitoring use case of IoMT (Hellewell et al., 2020). became a reality for healthcare facility management during the global pandemic COVID-19. Moreover, the remote care using IoMT could significantly reduce the risk of exposure for the most vulnerable populations such as elderly with pre-conditions (Ienca and Vayena, 2020). Thus, it is essential to use remote monitors and diagnostic tools to enhance the care delivery while restricting the physical interaction to minimum. In addition to monitoring the body parameters, the IoMT can offer assistance in various other aspects such as maintaining the medication schedules by sensing the use of medicine bottles (Immutouch, 2020) and promoting physical activity by monitoring the physical movement and heart rates (Jovanov, 2019).
At China, Shanghai Public Health Clinical Center (SPHCC) and seven other hospitals used connected thermometers to monitor the diagnosed patients continuously (Kerlink, 2020). The focus of this monitoring was to improve the protection for hospital staff by reducing their physical contact with the infected patients. VivaLNK’s body temperature sensors coupled with Cassia IoT Access controller were used and the information about any changes in the body temperature of patients was wirelessly communicated to the nurse station. A single gateway had the capability to pair and connect up to 40 Bluetooth Low Energy (BLE) devices. Thus, the connected thermometers at China provided protection to the hospital staff while managing the patients from a safe distance.
Furthermore, the data generated by nearly one million connected thermometers was also used for producing daily data about the trends of Influenza-like Illness (ILI) at United States by Kinsa Health (Lin and Wu, 2020). The temperature data was collected from geo-spatial network of thermometers connecting the United States counties. It was found that the anomalies identified for ILI were strongly correlated with case counts for COVID-19 and hence, the connected thermometers cannot only be used for remote monitoring of the diagnosed cases but also for the prediction of pandemic outbreak. Specific to the respiratory diseases associated with the COVID-19, the wireless sensors connected to the chest of patients and communicating with the nursing station can significantly reduce the exposure of hospital staff to the virus. Using the chest motion sensors, it is possible for the nurses to identify the emergency situations. Also, the physiological sensors can be used to detect the presence of pain in the patient by monitoring blood pressure, heart rate and respiratory rate (Mahajan, 2020). Thus, using IOT, the nurses can attend patient, when needed, instead of physically visiting him for monitoring purposes.
Quarantine Monitoring
Mobile applications as well as IoT can facilitate the authorities to maintain the quarantine of people, particularly those with a travel history. Most particularly, the GPS trackers embedded with smart phones or IoT devices can provide the location data to the authorities who can take appropriate actions to control the movements of patients as well as suspected people. Smart devices have been in use at various parts of the world already to ensure that people maintain social distance.
Electronic tracker wristbands have been proposed in Hong Kong to send alerts about the body temperature variations to the authorities to detect the COVID-19 cases among the recent travelers during their quarantine period (McArthur, 2020). Smart wearable tracking devices have been used by South Korea, Singapore, Middle-Eastern and European countries to detect if recent travelers have broken the quarantine (Mohammed et al., 2020a). Different variants of smart wristbands comprise of GPS chips and/or couple with smart phones of the users to track their locations and movements.
Generating Preventive Alerts
Since the emergence of IoT devices in the healthcare sector, it was thought that the wearable sensors provide an opportunity of reducing the cost of treatment due to early detection and prevention (Mohammed et al., 2020b). In the recent times, the wearable sensors have also been found promising to maintain the social distance and hence reducing the rate of pandemic spread. In this context, various researchers from around the world have developed solutions based on wearable sensors. IIT-Istituto Italiano di Tecnologia has designed a smartband iFeel-You to measure the body temperature and send alerts (Mohammed et al., 2020c). If a user has temperature higher than 37.5°C, an alert can be generated. Furthermore, the smartband detects the human body movement and identifies the presence of another band in a certain radio range; as soon as it is found that another smartband is in proximity, both vibrate to intimate the users about social distancing. The frequency of smartband is 2.45 GHz like Bluetooth, however, IIT has developed a proprietary protocol for faster and easier proximity detection. The wristbands and other wearable devices have also been used by citizens in the other countries. Immunotouch (Muzny et al., 2020) is another version of smart wristband which vibrates as the user brings his hand near to his mouth, nose or eye. The wristband is integrated with accelerometer and tracks the movement of hand 10 times in each second. Immunotouch is supported by a mobile app which provides the users with history of how their progress has been to control their touching behaviors. The vibration in the wristbands can prevent the users from touching their face, which could in turn reduce the risk of COVID-19 spread.
Facility Cleaning
Cleaning of the facilities is one of the essential processes which needs to be automated to avoid the spread of viruses. The risk of cross-contamination and spread of pandemics can largely be controlled through cleaning and disinfecting the facilities, particularly those housing the diagnosed patients. In Vancouver, IoT buttons have been used to send alerts to the authorities for advising about cleaning and maintenance of the public facilities. These buttons have been designed by the Visionstate and known as Wanda QuickTouch (Kerlink, 2020). The major feature of these buttons is that they do not require any infrastructure and are battery operated. The alerts can be sent by public or staff of the facilities such as nursing stations, restrooms, patients’ rooms to immediately notify the management or cleaning staff. Furthermore, the buttons can be coupled with the mobile applications to help the management to track the cleaning activities, response time of staff, etc.
Contact Tracing for Diagnosed Patients
Contact tracing is crucial to suppress the spread of COVID-19 as a person can be carrier of the virus even before he develops the common symptoms of fever or dry cough. The risk of catching the infection is highest for the people who had been in contact with a patient within the proximity of 1.5–2 m for 10–15 min (Peak et al., 2020). Thus, it is required to identify the contacts of people who have been diagnosed positive. The proposed architecture can facilitate the contact tracing process for advising the contacts of diagnosed patients to get tested or to remain in quarantine for 2 weeks. The sensors which could mostly be used for the purpose include temperature, proximity, GPS and GIS. Several IoT solutions have already been proposed for contact tracing which could in turn escalate the process of quarantining the people at risk.
An innovative set of wearables have been developed by Estimote (Estimote, 2020) which do not only keep track of health of the user but can also play a crucial role for contact tracing. The wearable devices known as “proof of health” include GPS location sensing, proximity sensors based on Bluetooth, battery and LTE interface. Also, manual controls are provided to facilitate manual change of health status of the user, if required. The device uses proximity sensors and maintain a log of people with whom the user comes into contact. In addition to the list being stored on the wearable itself, the log is also maintained at a centralized dashboard to further facilitate the management. In case a person is detected positive for the COVID-19 and as he sets the health state to diagnosed, the device sends an alert to all the people who had been in contact earlier. These devices can also prioritize the list of people by providing details about the time for which each contact was active. Hence, the wearable could provide cost-effective and immediate assistance during the process of contact-tracing, at least at the level of workplace.
In addition to relying on the sensors data to trace contacts of the diagnosed patients, the reports of social media and records of calls have also been used Buchanan et al. (2020). In Australia, a smart phone app, “COVIDSafe” has been launched by the government which helps the health officials to identify the people who may be at risk of COVID-19 due to being in contact with a diagnosed patient (CDC and Prevention, 2020). In order to abide by the privacy laws, the health officials can only access the information provided by app when someone is diagnosed positive and also allows his data to be used.
Similarly, in China, it was advised to the patients that they fill in the necessary information online before making a visit to the outpatient departments (Ramson et al., 2020). This information included history of residence of visit in the areas already affected with COVID-19, history of contact with people located at such areas, history of contact with people diagnosed with COVID-19 and history of contacting someone with cough over last 3 weeks. All this information shall assist the authorities to trace the contacts in case an outpatient is diagnosed positive for the COVID-19.
The Quick Response (QR) codes have also been heavily used by the restaurants and retail sector for contact tracing in addition to facilitating the order placement and payment collection. In China, the QR codes were placed at the public places and customers were required to scan them using their smart phone camera’s. Using this information from the customer’s device, it became possible to trace contact based on the time and location information (Suryaatmadja and Maulani, 2020). Also, the red-yellow-green system of QR health codes has been an innovation to manage COVID-19 at China. The color of these codes changes according to the COVID-19 risk of each user, which is computed based on frequency and status of diagnostic test and interactions with people having different codes. At the points of entry of public places, such as Subways, supermarkets and communities, people have been required to scan their QR health codes in order to get permission to enter (Ye et al., 2020). Also, Bluetooth beacons have been installed at the locations such as subways, taxis, restaurants, retail stores (Liu et al., 2020). These beacons transmit random identifiers which are stored in the smart devices of customers and can later be used for contact tracing and alert transmissions to those who might have been infected.
Contact Tracing has also been a challenge for the people using specific devices by Apple and Google at various countries. For example, at United Kingdom, a contact tracing app Beta was developed which was incompatible with the API developed by Google and Apple, due to the requirement of transmitting Bluetooth signals continuously (Guinchard, 2020). This app used Bluetooth to create a log of the nearby devices and people who may have come into the contact of users. However, both Apple and Google restrict how the Bluetooth of devices can be used to access the location information due to privacy advocacy. As a result, there has been a need for the developers to design sensing solutions without relying on proprietary technology and devices. Also, specific data protection guidelines of National Health Services (NHS) have to be taken care of while developing such contact tracing solutions.
Food and Medicine Delivery to Patients at Hospitals or Homes
The proposed IoT architecture connects the end-users with the suppliers for ordering the necessary supplies or medicines while avoiding the need of physical contact. Firstly, the internet technologies integrated with web pages and mobile apps allow the end users to place their orders and secondly, various proposals have been made to use robots or drones to deliver the supplies to patients or quarantined people to ensure social distancing. The home automation technologies such as smart door locks, surveillance cameras, motion detectors and video phones allow the safe and contactless ordering and of supplies. Therefore, it has been estimated that the home automation industry would grow followed by COVID-19 (Rincon et al., 2019).
Workplace Safety
Workplace safety can also be ensured using simple IoT devices. For example, the mobile applications can be provided to the employees which remind them to wash their hands, the wearable devices could be provided to prevent them from touching the face, and range sensors could be used to maintain the safe distance at the public spaces of the workplace such as restrooms or canteens. As previously mentioned, Estimote has developed the proof of health sensors for ensuring safety of employees through rapid contact tracing.
Similarly, another version of contact tracing wearable devices or keychain has been developed by Microshare and Kerlink which are asset tracking companies (Song et al., 2020). The product designed has been specific for the companies which either do not allow their employees to carry smartphones or employees do not have them. In such situations, the wearable wristbands, keychains or badges equipped with Bluetooth can be issued to the employees. As the employees come near to each other, the employees’ unique ID’s along with the encrypted codes can be stored. The contact data is also uploaded on the secured and centralized database through Low Power Wide Area Network (LoRaWAN), which helps the authorities to trace the contact of the employees who are tested positive or develop symptoms.
In addition to contact tracing, the IoT devices at the workplace can also ensure that people do not come into physical contact with facilities, hence reducing the risk of contaminating them. For example, the workplace environment can be made safer by deploying IoT solutions such as smart water dispenser, smart check-in systems, smart thermostat controls, smart access controls and smart management of the facilities. Most of these solutions could rely on voice, gesture or mobile app control.
IoT systems in conjunction with workplace management software have also been used to create digital floor plans. The workstations are marked unavailable based on the social distancing requirement using location information (Nasajpour et al., 2020). The updated floor plans are conveyed to the employees using touch points as well as mobile applications. Employees are monitored for breaking social distancing rules using the image and motion data anonymously. Furthermore, the data generated by IoT sensors is transmitted to user-friendly dashboards for perusal of managers about the occupancy and usage of facilities.
Smart Metering
Smart meters offer the utility providers with an excellent opportunity of charging the consumers and providing the usual supply while maintaining safe distance between the employees and consumers (IIT, 2020). The smart meters also have the capability to notify the authorities in case any tampering is made, which could facilitate the decision making regarding disconnection of service. From the consumer perspective, smart meters provide real-time data about the consumption patterns which could help the consumers to control their bills during the pandemic when financial constraints have become severe. Therefore, by using smart metering, the authorities would not need to physically read the meters, send the monthly bills to the consumers or to visit the locations to check tampering. Despite the positive attribute associated with smart meters, these have not yet been implemented at most locations in the developing countries such as Pakistan, India (Chawla et al., 2020), Bangladesh etc. COVID-19 situation can be regarded as an opportunity for deploying the smart meters in such countries.
Fitness and Lifestyle
COVID-19 has severely affected the lifestyle of people all around the world and has raised negative implications for individual and family health. In this section, we cover the three areas of physical health and fitness, mental health and domestic stress and violence. Gyms, fitness and physical activity centers have been closed all over the world in order to reduce the spread of COVID-19. The IoT devices can also be used for facilitating the users in their fitness journeys during the period. Similarly, IoT devices can be used to detect the anxiety in individuals and can connect to the friends and family using digital networking tools which could reduce the risk of depression and other serious health risks.
Several proposals and product prototypes have been developed for promoting the physical activity of users while at homes. For example, the home care for elderly using low-cost sensors have been proposed by (Jovanov, 2019). The system EMERALD uses sensors Electrocardiogram (ECG), Electrodermal Activity (EDA), Inertial Measurement Unit (IMU); these sensors have been integrated with chest band and wristband) to identify the emotional and physical state of the elderly and suggests the exercises based on personalized profile assessment. Although social distancing promotes the risk reduction for the spread of pandemics, it also has several negative effects. A focus group study conducted at the United Kingdom revealed that the COVID-19 policy of social distancing and isolation has had substantial negative impacts over the mental health and well being of people (Thienemann et al., 2020). Furthermore, social exclusion has been associated with the chances of cognitive impairment, particularly for elderly who may suffer from loss of motivation, loss of life-meaning and loss of routine and structure (?). Even the risk of premature mortality for the elderly could increase due to the loneliness and high prevalence of neurological and vascular diseases (Plagg et al., 2020). Thus, the IoT use case of deploying sensors for monitoring anxiety can reduce the risks of cognitive impairment and other mental stress.
Proposed End-To-End IOT Architecture for Managing Social-Distancing
In this paper, we propose new ways of providing individuals for health and behavioral monitoring as well as fighting the pandemic, while sustaining social distancing and self-quarantine. The entire process is incorporated within an end-to-end critical IoT-based infrastructure operating mainly on a combination of short-term action and long-term strategy. Short-term strategy involves automated activation of preventive alerts, playing music or religious verses and directions for specific activities like taking pills, doing exercise, or taking the right food and long-term strategy encompasses collection of patients’ vital parameters over time in a database, remote consultancy, if needed, with relevant physician, nurse or psychologist and drawing out a defined care and cure plan for the patient along with remote regular monitoring of the patient’s conditions. An overview of such proposed long and short term measures is depicted in Figure 2.
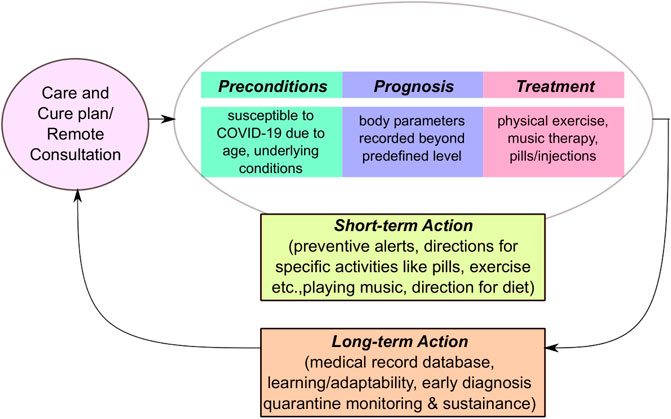
FIGURE 2. Methodology for sustaining social-distancing during COVID-19 pandemic encompassing short-term response and long-term management plans.
Proposed Methodology
The core of the infrastructure consists of a group of physiological body sensors and environmental sensors deployed on specific regions of the body as wearables and on specific regions of the house/apartment/room of an individual respectively for vital parameters and behavioral monitoring of that individual through ambient environmental monitoring.
The short-term response refer to the preventive measures applied during seconds and minutes after the deviations from the predefined threshold in recorded body parameters are observed in the monitored individuals. Based on the body and environmental parameters recorded by smart wearables and environmental sensing modules, relevant actions will be taken automatically using handheld mobile devices easily accessible to the individual without requiring any intervention from the monitored individual or any other human being. Actions that can be taken include automated activation of preventive alerts, playing of music or religious verses (for bringing down anxiety levels), specific direction for activities to be carried out like taking a pill, performing an exercise, calling an emergency help, or taking a certain kind of food such as drinking water, eating sugar if glucose level falls etc. depending on the range of the body or environmental parameters recorded. In case the monitored individual is diagnosed with COVID-19, the patient’s handheld device can leverage its Bluetooth connective history to trace all the people who had exposure to the infected individual. The traced people in turn are located and contacted to impose self-quarantine as immediately as possible.
The long-term management plan involve long-term adaptability planning for combating crisis situation due to pandemic while maintaining social distancing as well as minimizing the risk of the spread of the infection and future events of the pandemic surge. These measures include, but are no limited to the followings:
• Remote assessment and management for evaluating and preparing for the risks, like high blood pressure, diabetes, hyperthyroidism, COVID infection etc., introduced by deviations in body and environmental parameters and coming in close contact with already infected patients before quarantine measures were imposed on them.
• Accurate estimation of environmental parameters and body parameters for maintaining patient databases, infection preventive measures, preventive alerts and plan of activities for patients already suffering from chronic diseases like hypertension, diabetes, etc.
• Remote consultation with physician/nurse/psychologist in timely manner, remote patient and behavioral monitoring, proper guidance, upgrading care and cure plans with regular feedback from accumulated individual databases, increased preparedness and situation awareness through advanced monitoring and prediction tools.
• Early diagnosis through obtaining of nasal samples for testing with the use of robots, if found susceptible through contact tracing and aberration in vital or environmental parameters, thereby reducing chance of infection for healthcare workers.
• Smart metering and generating alerts on tampering through recordings from the environmental sensors deployed in the homes of the monitored individuals.
• Quarantine monitoring of individuals through the use of drones and smart phones, as soon as they are found positive through early diagnosis or are found susceptible due to underlying health conditions or close proximity with already infected patients. The location shall only be monitored once the person has been identified as a patient/carrier for the virus.
• Localization of the patients or those at risk of COVID-19 due to recently coming in contact with the positive cases using GPS in outdoor location and Angle-of-Arrival (AoA) technology over the indoor WiFi network. The AoA technique optimizes the arrival angle of the signals broadcasted by an electronic anklet to the antenna on the WiFi modem and/or WiFi access point within a larger premise, thereby enabling real-time localization even in an indoor environment. In this way, present limitations with GPS and Bluetooth technologies in indoor spaces, like high susceptibility to absorption, line-of-sight blockage and multipath reflections, can be easily overcome.
• Deployment of robots to assist individuals in quarantine through bringing them food and medical supply, sanitizing their environment, serving them with essentials as well as closely monitoring them in emergency situation, thereby alleviating stress levels of healthcare workers.
Proposed Architecture
• A group of non-invasive wearable physiological sensing modules capable of measuring vital body parameters like blood pressure, body temperature, blood oxygen level, pulse rate, respiratory rate, EEG, etc.
• A group of environmental sensors placed in the home of monitored individual for behavioral analysis without disturbing or disrupting their normal life.
• Dual GPS-WiFi enabled electronic anklet for patients tested positive for COVID and primary/secondary contacts of the patients, for localization, quarantine monitoring and isolation reinforcement. When outdoor, GPS links will be used for localization. In indoor scenario, in-house WiFi network will be used for localization.
• A communication module (also sometimes referred to as local processing unit (LPU)) consisting of the WiFi module to collect data from the sensor and connect micro-controller unit with the handheld smart phone or similar device belonging to the patient. The micro-controller unit together with the hand-held device are programmed to take automated decision of activating “Preventive Alerts”, if the parameters are outside the predefined threshold level as well as to transmit the data for storage at a network server.
• The hand-held device (LPU) also can consist of a Bluetooth module for “Contact Tracing”, alert creation module for generating an alarm when a measured vital sign falls outside a threshold predefined by a physician, audio/video module between users (patient-professional or professional-professional) with the possibility of sharing documents and vital signs measurements, notification system (e.g., SMS, email) for alerts when a specific activity has to be carried out (e.g., pills to be taken, measure to be taken, exercise to be performed), WiFi module for transmitting to the IoT gateway for further processing and global packet radio service (GPRS) module for sending the recorded data to a network server and database over a satellite communication network.
• IoT Gateway for forwarding the recorded patient/individual data to the cloud computing platform and to the utility companies over the internet for smart metering.
• Cloud computing platform capable of activating the proper service as per requirements depending on the situation and condition of an individual including early diagnosis, remote consultation or emergency services.
• A group of robots, drones and automated vehicles (AVs) that will deliver different services like sample collection, food and medical supply delivery, support for isolated people, crowd surveillance, screening, quarantine monitoring etc. and will be activated depending on the data collected and analyzed by the network server over the GPRS network.
Design Guidelines
Next, we outline the key design guidelines for the four-layer protocol stack, namely, for the physical (PHY), medium access control (MAC), network (NET) transport (TAP) and application (APP) layers for the proposed architecture, as shown in Figure 4.
1. Channel and PHY Layer - The propagation channel for the entire communication set-up in Figure 3 is intricately linked to a variety of environments (indoor/outdoor) depending on the mobility patterns of the monitored individuals, robots, AVs, drones, as well as the location of the infrastructural elements such as IoT gateway, base-stations (BS), network server, data center etc. Since field measurements would be extremely expensive and time consuming in different environments, network scenarios, stochastic and/or semi-stochastic mobility modeling may be more realistic. However, random mobility modelling may fail to accurately characterize the environment, since the robot/drone routes are typically pre-planned. Therefore pre-planned semi-stochastic mobility models (similar to Xu et al. (2013)) need to be developed for this kind of architectural set-up.
2. MAC Layer - The MAC layer takes care of the channel access control that make it possible for several nodes to communicate using a shared medium, without suffering from packet collisions transmitted by different nodes. In the proposed network scenario, the combination of having limited wireless spectrum, low latency requirements and large number of nodes with varying levels of mobility (high in case of drones) pose significant challenge on the MAC layer. The MAC protocol is expected to support diverse network topologies that vary dynamically owing to the large varieties of communication scenarios, end-nodes and applications. The MAC layer also has to avoid relying on an excessive number of radio frequency (RF) chains operating on different frequencies in an frequency division duplex (FDD) manner, which will not be suitable for small-size low-power IoT nodes. Alternatively, time division duplexing (TDD) may facilitate multiple nodes to access a shared medium, like Thiagarajan and Murthy (2015); Ding et al. (2018). Furthermore, token-based system and modern tools of edge-computing are needed to be explored for collision avoidance, reliability, efficiency, flexibility and robustness in the medium access control and topology management.
3. TAP and NET Layers - In the TAP and NET layers, scheduling and routing determine the multi-hop paths to be followed by packets between the source and destination nodes. More specifically, each hop to be taken by the data packets is decided dynamically and opportunistically at each stage of the multi-hop path rather than being decided by the source node (similar to Ali et al. (2020)). In particular, each packet may be received at more than one node and then forwarded by whichever has the first opportunity to transmit. This dynamic, opportunistic and redundant approach to the routing improves the network’s robustness to rapidly changing topologies, which is one of the main challenges for routing in the proposed network.
4. APP Layer - The design of the APP layer faces substantial challenges in terms of meeting diverse objectives like sending alerts to the user for regular activities like exercise, diet plan, medicine etc. as well as sending urgent alerts when an aberration is detected, and specific direction for coping with the situation. A major design guideline for handling such diverse objectives is to ensure minimum delay in generating the alerts due to latency in transferring information or requesting network services as per requirement. Another problem that needs to be avoided is the “disconnected” APP layer, where all the lower layers are functional while the application interface loaded on the mobile device of the user is not functioning correctly and is unable to provide essential patient data to the network for processing. Maintenance of patient confidentiality and anonymization of patient data is also an important factor that needs to be accounted for in the APP layer to avoid any privacy violation and security threats to individuals. In this regard, the proposals have already been made, such as using blockchain-enabled privacy preserving contact-tracing scheme Xu et al. (2020) or software defined security based data communication protocols Siriwardhana et al. (2020) which offers customized methods of data transmission based on patient’s health status.
Prospective Solutions
In order to address the major above-mentioned design challenges, we investigate some prospective solutions in terms of specific protocols and design technologies. It is worth-mentioning that the design challenges are associated not only with the proposed network architecture but also with the multiple network layers consisting of satellite, BSs, aerial drones, IoT gateways and ground robots while handling information dissemination across the multiple layers in heterogeneous environments, with the objective of meeting the stringent requirements of the proposed set-up in time-sensitive as well as life-critical applications. The stringent requirements for such a set-up include, information processing and taking preventive actions (refer to short-term actions from Figure 2) with minimal delay (typically within few milliseconds), communication of information with minimum probability of error (i.e., any error in transmitted information can result in the loss of vital patient data and unnecessary delay in taking proper action). Similarly, it is not possible for IoT to rely on a single protocol for various applications due to the diverse nature and hence, it is required to conduct an in-depth analysis of strengths and limitations of each protocol before selection.
Specific Protocols for COVID-19 Related Applications
The standard protocols which have been proposed for facilitating each application in the scenario of COVID-19, have been mentioned in Table 2. Here, the protocols at each layer (Application, Transport, Network and MAC) have been proposed considering the differentiated service requirements for each application. The emerging messaging protocols for application layer have been proposed based on the pros and cons, the interoperability requirements for each protocol and the message/data sharing required for each target application. The criteria such as message overhead, resource requirement power consumption, latency, bandwidth, reliability, security, provisioning and standardization have been considered. Similarly, for the Transport layer, the requirements of reliability or urgent delivery have been considered; for the MAC layer, the choice of protocols has again been made based on coverage and cost. Finally, the protocols for physical layer have been proposed considering communication environment and infrastructure, networking scenarios and expected mobility patterns of users in each application.
1. APP Layer - At application layer, the protocols have been selected from Message Queuing Telemetry Transport Protocol (MQTT), Advanced Message Queuing Protocol (AMQP) and Constrained Application Protocol (CoAP). MQTT has been designed for lightweight machine to machine (M2M) communication and follows the publish/transcribe method. MQTT uses TCP as a transport protocol and hence it facilitates connection-oriented reliable communication (Naik, 2017). Furthermore, MQTT offers three-level Quality of Service (QoS) which adds to the reliability. This protocol has been regarded as most suitable for the IoT applications where large network of small devices has to be managed. It is one of the simplest protocols which offers very few control options. CoAP is another lightweight M2M protocol and supports both architectures of resource/observe and request/response.
2. TAP Layer - CoAP uses User Datagram Protocol (UDP) at the transport layer and as a result, there is lesser reliability between the communication nodes due to use of connectionless datagrams. Two different level of QoS are used with “confirmable” or “non-confirmable” messages. As compared to MQTT, CoAP offers better control and functionality because of supporting content negotiation (Naik, 2017). Finally, AMQP is also designed for lightweight M2M protocol. It supports publish/subscribe and request/response architectures. AMQP also uses Transmission Control Protocol (TCP) at the transport layer and offers two levels of QoS, Settle format and Unsettle format. At transport layer, we have proposed either TCP or UDP. TCP is connection-oriented and reliable as it establishes a connection before transmitting data. On the other hand, UDP is less reliable but simple, and also faster than TCP.
3. NET Layer - At Network layer, standard protocol IPv4/IPv6 or IPv6 over Low -Power Wireless Personal Area Networks (6LoWPAN) should be used. 6LoWPAN is the compressed version for IoT applications where header bytes are significantly reduced as compared to IPv6, while providing full functionality.
4. PHY and MAC Layers - Finally, WiFi, Bluetooth, Zigbee, Long Term Evolution for Machines (LTE-M), Narrow Band-IoT (NB-IoT), Long Range (LoRa), 802.15.4 or 5G Ultra-Reliable Low-Latency Communication (URLLC) have been proposed to be used at the link layer. LTE-M is an IP based connection protocol and facilitates the connectivity of IoT devices to 4G (cellular networks) without the need of gateway. This technology has been optimized for mobile connections and high bandwidth supporting voice applications. NB-IoT was developed to utilize the established mobile networks to connect mass-distributed IoT devices and offers lower cost as compared to 4G, LTE-M and other General Packet Radio Service (GPRS) technologies but cannot connect to IP networks. NB-IoT is best suited for the IoT applications where small amount of data is frequently generated by devices. LoRa is a low power standard for wireless M2M communications. The standard enables devices to operate on extreme low signal levels which makes communication of distant nodes possible. Protocol 802.15.4 provides the link layer solution for low cost communication of nearby devices. URLLC is the specification provided by 5G for supporting IoT and Machine to Machine (M2M) communication at a massive scale. The major focus of URLLC is on the IoT applications that require ultra-low latency such as remote factory automation or surgeries.
Based on the above characteristics of different protocols, we have proposed protocols for each IoT application described in the IoT architecture Figure 3. As seen in Table 2, at application and transport layers, MQTT and TCP respectively, have been proposed where reliability is of crucial importance such as for early diagnosis, remote health monitoring, quarantine monitoring, contact tracing, workplace safety and smart metering. On the other hand, COAP and UDP have been proposed to be used where packet delivery and reliability can be compromised such as for the applications of generating preventive alerts, facility management, fitness and lifestyle management and delivering food and medicine. For network layer, any protocols from IPv6/IPv4 or 6LoWPAN could be used. On the link layer, Bluetooth, Zigbee and 802.15.4 have been proposed mostly for the applications where proximity is monitored and devices within close range need to communicate. NB-IoT has been proposed for the applications where frequent data will be generated in small packets. WiFi and LoRa has been regarded suitable for the indoor applications and LTE-M and 5G have been proposed for supporting applications with the requirements of long range and mobility.
Specific Design Technologies for COVID-19 Related Applications
1. Large-Scsale Antenna Arrays - Large scale Multiple-input-multiple-output (MIMO) employ hundreds of antennas for serving typically a few dozen terminals while sharing the same time-frequency resources. Therefore, it is possible to employ multiple antennas at the IoT gateway, BS and network server to enable energy-efficient transmission over the wireless environment suffering from deep fading and shadowing. In our proposed architecture (refer to Figure 3) if multiple users (hand-held devices equipped with single antenna) can communicate their body parameters simultaneously to an IoT gateway equipped with multiple antennas; a scenario referred to as virtual “MIMO” and virtual massive “MIMO” system Ciuonzo et al. (2012). Such a configuration will allow utilization of array processing gain providing spectral efficiency, fading mitigation and low-energy sensor adoption Dey et al. (2019).
2. Space-Time Spreading (STS) - A unique way of reducing energy consumption in an IoT network is to enable energy efficient transmission of sensor observations. This can be done by reducing the transmission energy required for fighting impairments like fading, shadowing, scattering inherent to the wireless propagation environment, as well as intrinsic interference resulting from superposition of multitude of sensor signals transmitted at the same time and over the same frequency band or closely-spaced frequency sub-bands. Therefore STS Dey et al. (2020b) of local sensor observations can be implemented before reporting them in order to reduce the chance of interference, thereby decreasing the requirement of transmission energy necessary to overcome interference due to superposition of transmitted sensor observations. In our envisioned architecture, if multiple users or hand-held devices are transmitting their information simultaneously over a fixed symbol duration, the information vector is first multiplied by a dispersion vector before transmission. In that case only one user is activated on a particular space-time (ST) block, when all the other users are kept silent. Such link set-up will offer significant improvement in performance and throughput and eliminate possibility of any interference between patient data streams, as is shown in our seminal work Siddiqui et al. (2021).
3. Selection Relaying - In our proposed architecture in Figure 4, any hand-held device can support diverse services in a location that has normal coverage. However, if the patient (hand-held device) is within a car, or travelling via high-speed train or through a tunnel, subway, or located in the basement, i.e., locations that exhibit challenging radio conditions and beyond the gateway coverage, the network will not be able to support different services like emergency calls, remote consultations. In such a scenario, the radio coverage can be extended by deploying a candidate set of low-complexity relays between the users and the gateway. From this set of relays, the single best relay having the highest estimated instantaneous signal-to-interference-plus-noise ratio can be selected; a technique referred to as selection relaying Khan et al. (2020). Such a system configuration will exploit diversity and cooperative transmission opportunistically thereby offering transfer of patient data with minimum latency and maximum energy efficiency Zou et al. (2019).
4. Cognitive Radio Communications - Existence of multiple users/hand-held devices as in case of our envisioned scenario (Figure 3) will lead to increased utilization of scarce spectrum. Therefore, dynamic spectrum management as well as energy efficient and environment-aware design should be jointly considered Salvo Rossi et al., 2013. A framework combining distributed decision fusion at the IoT gateway and orthogonal frequency division multiplexing (OFDM) based collaborative wideband spectrum sensing, similar to the one proposed in Dey et al. (2020a), can be used to address the above-mentioned challenges. In our proposed architecture, orthogonality of the multiple users/handheld devices transmitting simultaneously can be exploited by employing a massive antenna array at the gateway. The users scan the available bandwidth at regular intervals to search for unused bandwidth portions (commonly referred to as “spectrum holes” or “white spaces”) and transmit their information as soon as a “spectrum hole” is detected.
5. Resource Sharing and Allocation - Just like spectrum, existence of multiple users/hand-held devices will lead to increased usage of other resources like energy, computing memory, data processing tools etc. In order to handle and integrate computing resources in networks like the one proposed in Figure 3, distributed artificial intelligent (AI)-based methods can be investigated and implemented, similar to the ones proposed in Filippou et al. (2013), Butt et al. (2020). Such techniques will combine distribution of intelligence and computational resources among the network nodes (users, sensors, gateways, servers, etc.) by exploiting low performance learning methods, thereby reducing both latency and energy requirements.
6. Privacy Preserved Contact Tracing - Since the proposed architecture is based on collecting data from the wearable sensors, the users are expected to show reluctance towards the adoption. In order to overcome this challenge, the proposed architecture will make use of the contact-tracing scheme which have been developed with a focus on user’s privacy. The schemes which use advanced security algorithms and deploy efficient encryption schemes will be used for all the data collection activities. For example, the Apple/Google API does not collect location information and can still manage the contact tracing process by measuring the difference between the devices. Also, the Apple/Google API does not collect any data from non-COVID patients. Moreover, the contact tracing system powered by Blockchain, BeepTrace (Xu et al., 2020) has also recently been proposed where the privacy is even more preserved because of the blockchains bridging the user/patient. Only authorized solvers are allowed to desensitize the user information such as location and ID.
Expected Challenges and Future Research Directions
Challenges
Although IoT in tandem with other emerging technologies like 5G networks, AI and cloud-computing can revolutionize the healthcare sector, high cost associated with its mass-scale deployment has made it difficult for many telecommunication operators and governments to implement it over a large area with wide coverage. The lack of proper measures and guidelines in handling patient’s confidential data, algorithms for ensuring security and privacy increases vulnerabilities associated with the use of many technologies like drones, robots, autonomous vehicles etc. which are crucial supplements to the IoT platform. There is a considerable probability that the IoT devices can be tampered which may result in exploitation of data as well as possible miscommunication of information. Therefore, ensuring security of the devices used in the architecture makes an important challenge.
A common problem with most IoT devices is their limitation due to restricted battery life which inhibits their capability to operate for long duration and long distances in case of drones, robots etc. A robot can be sent to collect nasal samples for testing or rendering support to isolated patients, but will need a long battery life and extended capability to execute the entire task properly. In this regard, there has been numerous proposals for wireless charging of drones through laser (Rohan et al., 2019) or ambient radio frequencies (Mirzaeinia et al., 2019). Nevertheless, efficient energy harvesting and optimization techniques need to be deployed in order to charge the drones as they engage in the data collection activities.
Another plaguing issue is the adoption and acceptance of the developed technology and tools by the common population. The mere availability of IoT and telemedicine platforms, smart wearables, application platforms, drones and other technologies is futile until and unless they are trusted and used by patients, general pubic and health professionals. However, it is imperative that until a full-proof care and cure system is in place for handling COVID-19, the bulk of the responsibility lies with IoT and other emerging technologies in controlling the impact of this one or any other pandemic. Furthermore, the challenges specific to each layer of the IoT-based network architecture that are still need to solved moving beyond existing solutions for managing COVID-19 situations have been articulated below:
1. Channel and PHY Layer- For the PHY layer, the challenges associated with characterizing a diverse range of propagation environments and random mobility of the communicating entities can be catered to by ensuring inter-operability of various protocols/devices and wired/wireless links. Moreover, specific PHY layer algorithms have to be designed for avoiding interference between closely-spaced channels used by independent IoT devices for data transmission, ensuring high fault tolerance for all the equipment used, guaranteeing delivery of security and QoS requirements and dealing with varying data rates associated with majority of COVID-19 IoT applications. For example, the physical layer design WirelessHP proposed by Luvisotto et al. (2017) for critical control applications can be modified for the healthcare critical data transmission as well. Moroever, the 5G New Radio (NR) that has introduced massive Machine-Type Communications (mMTC) and Ultra-Reliable Low Latency Communications (URLLC) are expected to deal with the existing physical layer challenges for IoT, such as latency, reliability and security Ji et al. (2018).
2. MAC Layer- In order to address the challenge of medium and resource sharing over diverse network topologies and communication scenarios inherent to MAC, dynamic resource allocation algorithms have to be designed for timely transmission of data. Dynamic channels should be assigned based on traffic and user priority and control packets overhead should be efficiently managed in order to timely convey the urgent data about the applications such as remote healthcare, social distancing and quarantine management. Towards this end, prioritized data flow control can be applied to guarantee delay control and to ensure security against possible intrusion. In this context, various MAC layer schemes have been developed to ensure the timely delivery of data (Mwakwata et al. (2019); Misra et al. (2020); Olatinwo et al. (2020)); however, these solutions need to be selected specifically based on requirements for each application.
The synchronization between all the communicating entities is to be maintained without causing excessive synchronization packet overhead or high energy consumption due to idle listening and overhearing. The protocols must be selected such that highest possible throughput could be achieved, guaranteeing consistency for the format of frames and other information being generated by different IoT devices. The congestion at all network levels needs to be minimized by avoiding over-emitting. The packets must be scheduled effectively to facilitate thousands of independent nodes. Furthermore, designing multi-radio and multi-channel schemes is also crucial due to different requirements of IoT devices operating for COVID-19 application. Most of these latency and QoS issues are expected to be resolved by the designs of uRLLC and mMTC with the help of specific features such as transmission of short packets Bana et al. (2018) and prioritized allocation of bandwidth Beshley et al. (2020).
3. NET and TAP Layers- The problem of routing data packets through diverse platforms inherent to NET layer of the proposed architecture can be solved by applying optimum routing schemes, monitoring and managing network condition through load balancing, traffic control, traffic policing, and traffic shaping Malathy et al. (2020). Opportunistic routing can also be implemented for prioritizing data to ensure timely and reliable service for non-delay tolerant applications, ensuring localization and customization for each service, managing mobility developing and implementing security protocols, managing QoS for different applications and developing efficient fault tolerance mechanisms Zhong et al. (2020).
At the TAP layer, the challenge of scheduling transmission from multiple communication nodes firing simultaneously with urgent requirements can be circumvented by managing congestion throughout the network, providing means for self-configuration of mobile nodes, ensuring energy awareness of the nodes so they may take crucial routing and configuration decisions timely and refraining from the issues of constrained addressing and biased implementation. These routing issues are expected to be resolved by the novel routing protocols designed for the IoT such as backpressure routing Amiri et al. (2020) and uRLLC standard. To ensure timely transmission from each source, the uRLLC guarantees optimal resource allocation to each node Chang et al., (2019); the transmission power and bandwidth, both are allocated considering the constraints of network such that all the urgent data could be reliably transmitted.
4. APP Layer - The major design challenges with respect to application layer remains in developing an efficient interface to support users with varying levels of digital literacy Zallio et al. (2020). Some of the major challenges include ensuring data security to prevent hacking and intrusion, providing mechanisms for congestion control to timely report data to the required destination, managing flow control to avoid bottlenecks, and using appropriate methods to recover the packet loss wherever required. Moreover, the novel issues related to privacy have also been raised in the era of COVID-19 due to transmitting large amount of personal information over wireless networks Fang and Qian (2020). Various proposals and frameworks have recently been proposed to ensure reliable delivery of medical grade data using the uRLLC and mMTC scenarios, such as the use of security capacity formula Ren et al. (2020) and deep learning models Thantharate et al. (2020).
Future Directions
Most of the use cases included in the architecture proposed in this paper would impose restrictions to the freedom, privacy and even to the human rights of citizens. However, it is important to note that the level of intrusion into the private life expected to be made by the proposed architecture is meant to deal with the emergency situations only. The intrusions are temporary and could have exemption by the data protection laws. For example, the article nine of the European Data Protection Board states that “for reasons of public interest in the area of public health, such as protecting against serious cross-border threats to health” the personal data may be processed (Vaishya et al., 2020). Yet, wherever possible, the confidentiality and privacy of the citizens shall be ensured through responsible use of the devices, protocols and data. In this regard, there have been proposals where image sensors are used to detect the mood (Mohr et al., 2017) or heart rate (Malasinghe et al., 2018) in the real-time; this information is then communicated anonymously (without sending the images) to protect confidentiality of the users. Hence, the proposed architecture would ensure data in anonymous form to preserve the confidentiality of users.
Extending the lifetime of IoT devices without compromising its capabilities is also possible through several different ways; 1) by creating more energy efficient devices (wearables, sensors, actuators, drones, robots etc.), 2) by designing specific radio protocols to enhance energy conservation, 3) by activating the device only when and where necessary and 4) by enabling energy efficient transmission of required data which shall reduce energy consumption for combating wireless environmental impairments like fading, shadowing, interference etc. Though bringing reliable, precise and robust behavior to low-power IoT networks is a non-trivial task complicated by extremely low-energy, computational and overhead budgets; it is possible to implement an energy efficient IoT network using one of the or a combination of the above-mentioned methods. Similarly, it is also a crucial requirement to analyse the pros and cons of widely accepted and emerging messaging and communication protocols and developing more interoperable solutions to meet the needs of IoT applications. Finally, it is impending that the government, ministry, responsible authority, health professionals and the general public all should work together to accept, trust and adopt the available technology to reduce the impact of the extent of the havoc wreaked by the COVID-19 pandemic.
Limitations
The architecture proposed in this paper is based on the assumption of the presence of ubiquitous wireless communication networks. The presented architecture involves a combination of wireless sensor networks (WSNs), IoT networks and aerially deployed networks. Such networks or combination of them do not impose a high requirement in terms of bandwidth, data rate, resolution and high-end computational space, device or complexity. However, the proposed architecture do require network connectivity to be available between the communicating devices. Regions of the world that are not that well-covered for network services, like rural areas, sparsely populated regions, isolated islands etc., cannot be catered to by this architecture alone. Spotty network connections can also hinder the operation of the architecture; thereby adding latency in communication of vital data and loss in reliability of data transfer. Owing to latency in communication of vital parameters in patients can result in delay in deploying emergency response if required. A prospective solution for such a scenario will be to involve high altitude platform (HAP)-based networks like Google’s Project Loon or SpaceX’s Starlink, if available, and route all data through the HAP-based network rather than terrestrial IoT networks. However, HAP-based networks are not that common and widely deployed at present but can be widely available in future; an interesting addition that we would be considering in our future contributions.
The proposed architecture has been developed based on the assumption that the populations will be provide access to their data considering the fact that the data will be anonymized before they are shared over the network and patient-physician confidentiality will be maintained at all times. The actual protocol that will be followed to maintain anonymization, privacy and confidentiality is beyond the scope of this paper; an important generalization we plan to include in our future design.
The short-term and long-term actions devised in this paper do not require the patients to be highly Information Technology (IT)-literate, as most of the alerts, preventive measures will be activated automatically depending on the present condition of the patients. However, the actions do require the medical personnel’s involved in executing the actions to be IT-literate and skilled in handling online platforms and interpreting data received over such online platforms.
The proposed architecture being a combination of WSN and IoT infrastructure is highly energy efficient. The energy requirement for the processes of collecting data from the patient’s body, analyzing it, communicating it over the network to the intended destination and activating relevant response or emergency actions is quite low, even when seen from a cumulative perspective. However, the architecture will fail completely to respond if the handheld devices belonging to the individuals (patients, responders or consultants) are out of charge or battery back-up. The wearable and environmental sensors responsible for collecting patient data will also need continuous maintenance and regular battery replacement as and when required. Any of the initial devices being out of power can result in failure in launching of further response and relevant actions.
Conclusion
An end-to-end IoT enabled architecture has been proposed to manage social distancing during COVID-19 like pandemics. A detailed review of sensing and communication technologies which can be used for the purpose has been presented. It has been found that IoT technologies promised to support the requirement of social distancing at various indoors and outdoors environments due to the recent advancements in the design of sensing and communications infrastructure. Moreover, the challenges associated with each layer of IoT structure have been described and design guidelines to combat these challenges have also been presented. It is expected that the proposed architecture for ensuring end-to-end social distancing while providing the basic supplies shall help the individual citizens, governments and medical staff to deal with pandemics more efficiently.
Data Availability Statement
The original contributions presented in the study are included in the article/Supplementary Material, further inquiries can be directed to the corresponding author.
Author Contributions
SS conceived the idea, conducted the literature survey and participated in the write-up. MS suggested the protocols for each layer and participated in developing the framework. Being the senior most, MS also reviewed the paper and suggested improvements. AK worked on the proposed architecture, survey table and also developed the template. ID developed the methodology and framework diagrams and also wrote these sections.
Conflict of Interest
The authors declare that the research was conducted in the absence of any commercial or financial relationships that could be construed as a potential conflict of interest.
References
Abdel-Basset, M., Manogaran, G., Mohamed, M., and Rushdy, E. (2019). Internet of things in smart education environment: supportive framework in the decision-making process. Concurrency Comput. Pract. Experience. 31, e4515. doi:10.1002/cpe.4515
Abeler, J., Bäcker, M., Buermeyer, U., and Zillessen, H. (2020). Covid-19 contact tracing and data protection can go together. JMIR Mhealth and Uhealth. 8, e19359. doi:10.2196/19359
Akkaş, M. A., Sokullu, R., and Çetin, H. E. (2020). Healthcare and patient monitoring using iot. IoT. Elsevier B.V. located in Amsterdam, 11, 100173. doi:10.1016/j.iot.2020.100173
Alghadeir, A., and Al-Sakran, H. (2016). Smart airport architecture using internet of things. Int. J. Innovative Res. Comput. Sci. Tech. 4, 148–155.
Ali, A., Tariq, S., Iqbal, M., Feng, L., Raza, I., Siddiqi, M. H., et al. (2020). Adaptive bitrate video transmission over cognitive radio networks using cross layer routing approach. IEEE Trans. Cogn. Commun. Netw. 6, 935–945. doi:10.1109/tccn.2020.2990673
Alladi, T., Chamola, V., Parizi, R. M., and Choo, K-K. R. (2019). Blockchain applications for industry 4.0 and industrial IoT: a review. IEEE Access 7, 176935–176951. doi:10.1109/access.2019.2956748
Alvarez, J., Acero, A., Gutierrez, S., Rodrigo, P. M., and Lay-Ekuakille, A. (2018). “A low cost presence detection system for smart homes,” in 2018International Conference on Research in Intelligent and Computing in engineering (RICE), San Salvador, ELSL, August 22–24, 2018 (New York, NY: IEEE), 1–6.
Amiri, I. S., Prakash, J., Balasaraswathi, M., Sivasankaran, V., Sundararajan, T. V. P., Hindia, M. H. D. N., et al. (2020). Dabpr: a large-scale internet of things-based data aggregation back pressure routing for disaster management. Wireless Netw. 26, 2353–2374. doi:10.1007/s11276-019-02122-3
Armitage, R., and Nellums, L. B. (2020). Covid-19 and the consequences of isolating the elderly. Lancet Public Health 5, e256. doi:10.1016/s2468-2667(20)30061-x
Australian-Government (2020). Covidsafe app. Available at: https://www.health.gov.au/resources/apps-and-tools/covidsafe-app (Accessed May 13, 2020).
Baashirah, R., and Elleithy, K. (2019). “Automation of the baggage check-in process using rfid system in airports,” in IEEE long island systems, applications and technology conference(LISAT), Farmingdale, NY, May 3, 2019 (New York, NY: IEEE), 1–4.
Bai, L., Yang, D., Wang, X., Tong, L., Zhu, X., Bai, C., et al. (2020). Chinese experts’ consensus on the internet of things-aided diagnosis and treatment of coronavirus disease 2019. Clinical eHealth 3, 7–15. doi:10.1016/j.ceh.2020.03.001
Bana, A-S., Trillingsgaard, K. F., Popovski, P., and De Carvalho, E. (2018). “Short packet structure for ultra-reliable machine-type communication: tradeoff between detection and decoding,” in IEEE international conference on acoustics, speech and signal processing (ICASSP), Calgary, CA, April 15–20, 2018 (New York, NY: IEEE), 6608–6612.
Behar, J. A., Liu, C., Tsutsui, K., Corino, V. D. A., Singh, J., Pimentel, M. A. F, et al. (2020). Remote health monitoring in the time of covid-19. [preprint] (Accessed May 18, 2020).
Beshley, M., Kryvinska, N., Seliuchenko, M., Beshley, H., Shakshuki, E. M., Yasar, A-U-H., et al. (2020). End-to-End QoS "smart queue" management algorithms and traffic prioritization mechanisms for narrow-band internet of things services in 4G/5G networks. Sensors 20, 2324. doi:10.3390/s20082324
Buchanan, W. J., Imran, M. A., Ur-Rehman, M., Zhang, L., Abbasi, Q. H., Chrysoulas, C., et al. (2020). Review and critical analysis of privacy-preserving infection tracking and contact tracing. Front. Comms. Net. 2, 1–28. doi:10.3389/frcmn.2020.583376
Butt, M. M., Dey, I., Dzaferagic, M., Murphy, M., Kaminski, N., and Marchetti, N. (2020). Agent-based modeling for distributed decision support in an IoT network. IEEE Internet Things J. 7, 6919–6931. doi:10.1109/jiot.2020.2976802
Cai, Y., Genovese, A., Piuri, V., Scotti, F., and Siegel, M. (2019). “Iot-based architectures for sensing and local data processing in ambient intelligence: research and industrial trends,” in 2019 IEEE international Instrumentation and measurement technology conference (I2MTC), Auckland, NZ, May 20–23, 2019 (New York, NY: IEEE), 1–6.
CDC and Prevention (2020). Social distancing. Available at: https://www.cdc.gov/coronavirus/2019-ncov/prevent-getting-sick/social-distancing.html (Accessed May 17, 2020).
CDC (2020a). 1918 pandemic (H1N1 virus). Available at: https://www.cdc.gov/flu/pandemic-resources/1918-pandemic-h1n1.html (Accessed March 20, 2019).
CDC (2020b). 1957–1958 pandemic (H2N2 virus). Available at: https://www.cdc.gov/flu/pandemic-resources/1957-1958-pandemic.html (Accessed January 2, 2019).
CDC (2020c). 2009 H1N1 pandemic (H1N1pdm09 virus). Available at: https://www.cdc.gov/flu/pandemic-resources/2009-h1n1-pandemic.html (Accessed June 11, 2019).
CDC (2020d). People who are at higher risk for severe illness. Available at: https://www.cdc.gov/coronavirus/2019-ncov/need-extra-precautions/people-with-medical-conditions.html (Accessed March 15, 2021).
Çeken, C., and Abdurahman, D. (2019). Simulation modeling of an iot based cold chain logistics management system. Sakarya Univ. J. Comput. Inf. Sci. 2, 89–100. doi:10.35377/saucis.02.02.598963
Chai, P. R., Zhang, H., Jambaulikar, G. D., Boyer, E. W., Shrestha, L., Kitmitto, L., et al. (2019). An internet of things buttons to measure and respond to restroom cleanliness in a hospital setting: descriptive study. J. Med. Internet Res. 21, e13588. doi:10.2196/13588
Chang, B., Zhang, L., Li, L., Zhao, G., and Chen, Z. (2019). Optimizing resource allocation in urllc for real-time wireless control systems. IEEE Trans. Veh. Technol. 68, 8916–8927. doi:10.1109/tvt.2019.2930153
Chan-Yeung, M., and Xu, R-H. (2003). Sars: epidemiology. Respirology 8, S9–S14. doi:10.1046/j.1440-1843.2003.00518.x
Chatterjee, J. M., Kumar, R., Khari, M., Hung, D. T., and Le, D-N. (2018). Internet of things based system for smart kitchen. Int. J. Eng. Manufacturing 8, 29–39. doi:10.5815/ijem.2018.04.04
Chawla, Y., Kowalska-Pyzalska, A., and Skowrońska-Szmer, A. (2020). Perspectives of smart meters' roll-out in India: an empirical analysis of consumers' awareness and preferences. Energy Policy 146, 111798. doi:10.1016/j.enpol.2020.111798
Chehri, A., and Jeon, G. (2019). “The industrial internet of things: examining how the iiot will improve the predictive maintenance,” Innovation in medicine and healthcare systems, and multimedia (New York, NY: Springer), 517–527.
Choudhary, M. (2020). How iot can help fight covid-19 battle. Available at: https://www.geospatialworld.net/blogs/how-iot-can-help-fight-covid-19-battle/ (Accessed May 10, 2020).
Chowdhury, M. Z., Hossan, M. T., Shahjalal, M., Hasan, M. K., and Jang, Y. M. (2020). A new 5g ehealth architecture based on optical camera communication: an overview, prospects, and applications. IEEE Consum. Electron. Mag. 9, 23–33. doi:10.1109/MCE.2020.2990383
Ciuonzo, D., Romano, G., and Salvo Rossi, P. (2012). Channel-aware decision fusion in distributed MIMO wireless sensor networks: decode-and-fuse vs. decode-then-fuse. IEEE Trans. Wireless Commun. 11, 2976–2985. doi:10.1109/TWC.2012.061912.112049
Coelho, S., Rozario, R., Sharma, R., and Mehra, M. (2018). “An iot based smart cubicle system for effective power usage and employee monitoring in offices,” in 2018 International Conference on smart City and emerging technology (ICSCET), Mumbai, India, January 5, 2018 (New York, NY: IEEE), 1–6.
da Silva Medeiros, G. V., dos Santos, M. R., Lopes, A. S. B., and Neto, E. C. B. (2017). “Smartgas: a smart platform for cooking gas monitoring,” in 2017 IEEE First Summer School on Smart Cities (S3C), Natal, BR, August 7–11, 2017 (New York, NY: IEEE), 97–102.
Das, J., Pramanik, A., and Parui, S. K. (2020). “Smart garbage monitoring and alert system using iot,” in Proceedings of the 2nd international conference on communication, devices and computing, 14th–15th, March, Haldia, India, (New York, NY: Springer), 641–654.
Dey, I., Majid Butt, M., and Marchetti, N. (2019). Throughput analysis for virtual MIMO WSNs over measured MIMO channels. IEEE Trans. Instrum. Meas. 68, 297–299. doi:10.1109/tim.2018.2874370
Dey, I., Ciuonzo, D., and Salvo Rossi, P. (2020a). Wideband collaborative spectrum sensing using massive MIMO decision fusion. IEEE Trans. Wireless Commun. 19, 5246–5260. doi:10.1109/twc.2020.2991113
Dey, I., Joshi, H., and Marchetti, N. (2020b). Space-time spreading aided distributed MIMO-WSNs. IEEE Commun. Lett. [Early Access]. doi:10.1109/LCOMM.2020.3046231
Ding, T., Ding, M., Mao, G., Lin, Z., Zomaya, A. Y., and López-Pérez, D. (2018). Performance analysis of dense small cell networks with dynamic TDD. IEEE Trans. Veh. Technol. 67, 9816–9830. doi:10.1109/tvt.2018.2854287
Doffman, Z. (2020). Coronavirus police surveillance tags are now here: Hong Kong first to deploy. Available at: https://www.forbes.com/sites/zakdoffman/2020/03/17/alarming-coronavirus-surveillance-bracelets-now-in-peoples-homes-heres-what-they-do (Accessed May 09, 2020).
Doshi, A., Platt, Y., Dressen, J. R., Mathews, B. K., and Siy, J. C. (2020). Keep calm and log on: telemedicine for covid-19 pandemic response. J. Hosp. Med. 15, 302–304. doi:10.12788/jhm.3419
El-Din, D. M., Hassanein, A. E., Hassanien, E. E., and Hussein, W. M. (2020). E-quarantine: a smart health system for monitoring coronavirus patients for remotely quarantine. [preprint].(Accessed May 5, 2020).
Eriksson, J., Russo, N. L., and Marin, J. (2018). Using the internet of things to support emotional health. EAI Endorsed Trans. Ambient Syst. 18, e1. doi:10.4108/eai.23-3-2018.154372
Estimote (2020). Workplace safety with wearables. (estimote). Available at: https://estimote.com/wearable/ (Accessed May 14, 2020).
Fang, D., and Qian, Y. (2020). 5g wireless security and privacy: architecture and flexible mechanisms. IEEE Veh. Technol. Mag. 15, 58–64. doi:10.1109/mvt.2020.2979261
Filippou, M. C., Ropokis, G. A., and Gesbert, D. (2013). “A team decisional beamforming approach for underlay cognitive radio networks,” in IEEE PIMRC conference, London, United Kingdom, September 8–11, 2013 (New York, NY: IEEE), 5.
Garza, A. D. L. (2020). Coronavirus researchers using AI to predict virus spread. Available at: https://time.com/5780683/coronavirus-ai/ (Accessed June 22, 2020).
Ghazali, T-K., and Zakaria, N. H. (2018). Security, comfort, healthcare, and energy saving: a review on biometric factors for smart home environment. J. Comput. 29, 189–208. doi:10.3966/199115992018012901017
Goodman, D. (2020). World economy faces $5 trillion hit that’s like losing Japan, New York City: Bloomberg.
Guinchard, A. (2020). Our digital footprint under covid-19: should we fear the UK digital contact tracing app? International Review of Law. Comput. Tech. 35, 84–97. doi:10.1080/13600869.2020.1794569
Gupta, M., Abdelsalam, M., and Mittal, S. (2020). Enabling and enforcing social distancing measures using smart city and its infrastructures: a covid-19 use case. arXiv preprint arXiv:2004.09246 (Accessed April 13, 2020).
Hahn, S. M. (2020). Coronavirus (COVID-19) update: serological tests. Maryland, United States: U.S. food and drug administration.
Hellewell, J., Abbott, S., Gimma, A., Bosse, N. I., Jarvis, C. I., Russell, T. W., et al. (2020). Feasibility of controlling covid-19 outbreaks by isolation of cases and contacts. Lancet Glob. Health 8, E488–E496. doi:10.1016/S2214-109X(20)30074-7
Hondrou, C., and Caridakis, G. (2012). “Affective, natural interaction using eeg: sensors, application and future directions,” in Hellenic conference on artificial intelligence, Lamia, GR, May 28-31, 2012 (New York, NY: Springer), 331–338.
Hsu, W-L., Jhuang, J-Y., Huang, C-S., Liang, C-K., and Shiau, Y-C. (2019). Application of internet of things in a kitchen fire prevention system. Appl. Sci. 9, 3520. doi:10.3390/app9173520
Huawei (2020). Combating COVID-19 with 5G: opportunities to improve public health systems. Available at: https://www.thedatadriver.com/2020/03/combating-covid-19-with-5g.html (Accessed May 14, 2020).
Ienca, M., and Vayena, E. (2020). On the responsible use of digital data to tackle the covid-19 pandemic. Nat. Med. 26, 463–464. doi:10.1038/s41591-020-0832-5
IIT (2020). ifeel-you bracelet. Available at: https://www.iit.it/iit-vs-covid-19/ifeel-you-bracelet (Accessed May 10, 2020).
Immutouch (2020). Keep germs on your hands out of your mouth, nose, and eyes. Available at: https://immutouch.com/ (Accessed May 13, 2020).
iot. do (2020). Pantelligent smart frying pan. Available at: https://iot.do/devices/pantelligent-smart-frying-pan (Accessed May 10, 2020).
Jain, S., and Chandrasekaran, K. (2020). “Industrial automation using internet of things,” Security and privacy issues in sensor networks and IoT. (Hershey, US: IGI Global), 28–64.
Ji, H., Park, S., Yeo, J., Kim, Y., Lee, J., and Shim, B. (2018). Ultra-reliable and low-latency communications in 5g downlink: physical layer aspects. IEEE Wireless Commun. 25, 124–130. doi:10.1109/mwc.2018.1700294
Joo, I-Y., and Choi, D-H. (2017). Optimal household appliance scheduling considering consumer's electricity bill target. IEEE Trans. Consumer Electron. 63, 19–27. doi:10.1109/tce.2017.014666
Jovanov, E. (2019). Wearables meet iot: synergistic personal area networks (spans). Sensors 19, 4295. doi:10.3390/s19194295
Kerlink (2020). Kerlink and microshare design a contact-tracing solution to help suppress spread of the covid-19 pandemic. Available at: https://www.kerlink.com/blog/2020/05/07/kerlink-and-microshare-design-a-contact-tracing-solution-to-help-suppress-spread-of-the-covid-19-pandemic/ (Accessed May 14, 2020).
Khan, M. A., Shahid, M. H. B., Mansoor, H., Shafique, U., Khan, M. B., et al. (2019). “Iot based grocery management system: smart refrigerator and smart cabinet,” in 2019 International conference on systems of collaboration big data, Internet of Things & Security (SysCoBIoTS), Casablanca, MR, December 12–13, 2019 (New York, NY: IEEE), 1–5.
Khan, F. A., Malik, Z. A., Nasir, A. A., and Masood, M. (2020). Relay selection & power allocation for maximizing sum-throughput of a buffered relay network. IEEE Commun. Lett. 24, 1318–1322. doi:10.1109/lcomm.2020.2981882
Kim, J-H., Choi, S-J., and Jeong, J-W. (2019). Watch & do: a smart IoT interaction system with object detection and gaze estimation. IEEE Trans. Consumer Electron. 65, 195–204. doi:10.1109/tce.2019.2897758
Komagata, H., Hattori, T., Ohshima, R., Kakinuma, E., Ishikawa, M., Shinoda, K., et al. (2019). “Development of human behavior monitoring system around bed using infrared depth sensor,” in 2019 IEEE 1st global conference on life sciences and technologies (LifeTech), Osaka, JP, March 12–14, 2019 (New York, NY: IEEE), 117–118.
Kshirsagar, S., Sachdev, S., Singh, N., Tiwari, A., and Sahu, S. (2020). “Iot enabled gesture-controlled home automation for disabled and elderly,” in 2020 fourth international conference on computing methodologies and communication (ICCMC), Erode, India, March 11–13, 2020 (New York, NY: IEEE), 821–826.
Lin, B., and Wu, S. (2020). Covid-19 (coronavirus disease 2019): opportunities and challenges for digital health and the internet of medical things in China. OMICS: A J. Integr. Biol. 24, 231–232. doi:10.1089/omi.2020.0047
Liu, X., Trieu, N., Kornaropoulos, E. M., and Song, D. (2020). Beetrace: a unified platform for secure contact tracing that breaks data silos. arXiv preprint arXiv:2007.02285 (Accessed July 5, 2020).
Lokman, A., and Ramasamy, R. K. (2019). “Smart toilet: threats and challenges identifying human presence using iot sensors,” in Proceedings of the 3rd international conference on big data and internet of things, Melbourn Australia, August 13–15, 2019 (New York, NY: Association for Computing Machinery), 56–60.
Lopez, S. C., Tan, S. S., Villasin, W., Zarzoso, A. J., and Torregaza, M. L. (2018). Raspberry pi 3 as a smart stand-alone pos system in a small restaurant business. J. Telecommunication, Electron. Comput. Eng. (JTEC) 10, 47–53. doi:10.13189/ujeee.2015.030403
Lotfi, A., and Elmisery, A. M. (2019). Sensor network in automated hand hygiene systems using iot for public building. Emerging Trends Intell. Comput. Inform. Data Sci. Intell. Inf. Syst. Smart Comput. 1073, 463.
Luvisotto, M., Pang, Z., Dzung, D., Zhan, M., and Jiang, X. (2017). Physical layer design of high-performance wireless transmission for critical control applications. IEEE Trans. Ind. Inf. 13, 2844–2854. doi:10.1109/tii.2017.2703116
Ma, Y., Feng, X., Jiao, J., Peng, Z., Qian, S., Xue, H., et al. (2020). “Smart fire alarm system with person detection and thermal camera,” in International conference on computational science, Krakow, PL, June 6–18, 2021 (New York, NY: Springer), 353–366.
Mahajan, S. (2020). Smart home appliances market 2020: industry growth, trends analysis, covid-19 impact and forecast to 2026. Available at: https://works.bepress.com/sayali-mahajan/98/download/ (Accessed May 13, 2020).
Malasinghe, L., Katsigiannis, S., Ramzan, N., and Dahal, K. (2018). “Remote heart rate extraction using microsoft kinecttm v2. 0,” in Proceedings of the 2018 10th international conference on bioinformatics and biomedical technology, Amsterdam, NL, May 16, 2018 (New York, NY: Association for Computing Machinery), 1–6.
Malathy, S., Porkodi, V., Sampathkumar, A., Hindia, M. N., Dimyati, K., Tilwari, V., et al. (2020). An optimal network coding based backpressure routing approach for massive iot network. WLAN. 26, 3657–3674. doi:10.1007/s11276-020-02284-5
Manikandan, T., Selvan, D. T., Vaidhyanathan, R., Vigneshvaran, B., and Nandalal, V. (2020). Home groceries management system using IoT. Int. J. Psychosocial Rehabil. 24, 2741–2746. doi:10.37200/IJPR/V24I5/PR201977
Manoj, R., Kumarasami, R., Joseph, J., George, B., and Sivaprakasam, M. (2019). “Continuous weight monitoring system for icu beds using air-filled mattresses/pads: a proof of concept,” in 2019 IEEE international symposium on medical measurements and applications (MeMeA), Istanbul, TR, June 26–28, 2019 (New York, NY: IEEE), 1–5.
Marques, G. (2019). “Ambient assisted living and internet of things,” In Harnessing the Internet of everything (IoE) for accelerated innovation opportunities. (Hershey, US: IGI Global), 100–115.
Marr, B. (2020). Robots and drones are now used to fight covid-19. Available at: https://www.forbes.com/sites/bernardmarr/2020/03/18/how-robots-and-drones-are-helping-to-fight-coronavirus/***4d7abf662a12 (Accessed May 05, 2020).
McArthur, R.. (2020). Bahrain launches electronic bracelets to keep track of active covid-19 cases. Available at: https://www.mobihealthnews.com/news/europe/bahrain-launches-electronic-bracelets-keep-track-active-covid-19-cases (Accessed May 09, 2020).
Mekruksavanich, S. (2019). “The smart shopping basket based on iot applications,” in 2019 IEEE 10th International Conference on Software Engineering and Service Science (ICSESS), Beijing, China, Octomber 18–20, 2019 (New York, NY: IEEE), 714–717.
Mirzaeinia, A., Mirzaeinia, M., Bradfield, Q. A., Bradley, S., and Hassanalian, M. (2019). “Particle swarm optimization for wireless charging of swarming drones through ambient radio frequencies,” in AIAA propulsion and energy 2019 forum, Indianapolis, IN, August 19–22, 2019 4463.
Misra, S., Bishoyi, P. K., and Sarkar, S. (2020). i-mac: in-body sensor mac in wireless body area networks for healthcare iot. IEEE Syst. J. 25, 1–8. doi:10.1109/JSYST.2020.3020306
Mohamed, M., Gomaa, H., and El-Sherif, N. (2018). Evaluation of current smart airport technologies implemented in cairo international airport. Int. J. Heritage, Tourism Hospitality 12, 130–140. doi:10.21608/ijhth.2019.31985
Mohammed, M. N., Desyansah, S., Al-Zubaidi, S., and Yusuf, E. (2020a). An internet of things-based smart homes and healthcare monitoring and management system. J. Phys. Conf. Ser. 1450, 012079. doi:10.1088/1742-6596/1450/1/012079
Mohammed, M. N., Hazairin, N. A., Syamsudin, H., Al-Zubaidi, S., Sairah, A., Mustapha, S., et al. (2020b). 2019 novel coronavirus disease (covid-19): detection and diagnosis system using iot based smart glasses. Int. J. Adv. Sci. Tech. 29, 954–960.
Mohammed, M. N., Syamsudin, H., Al-Zubaidi, S., Aks, R. R., and Yusuf, E. (2020c). Novel covid-19 detection and diagnosis system using iot based smart helmet. Int. J. Psychosocial Rehabil. 24, 2296–2303. doi:10.37200/IJPR/V24I7/PR270221
Mohr, D. C., Zhang, M., and Schueller, S. M. (2017). Personal sensing: understanding mental health using ubiquitous sensors and machine learning. Annu. Rev. Clin. Psychol. 13, 23–47. doi:10.1146/annurev-clinpsy-032816-044949
Morales, L. V. M., Piratova, K. X. C., and Martinez, M. A. R. (2019). Real-time temperature and audio baby monitoring using iot technologies,” in Congreso Internacional de innovación y Tendencias en ingenieria (CONIITI), Bogota, Colombia, Octomber 2–4, 2017 (New York, NY: IEEE), 1–6.
Muzny, M., Henriksen, A., Giordanengo, A., Muzik, J., Grøttland, A., Blixgård, H., et al. (2020). Wearable sensors with possibilities for data exchange: analyzing status and needs of different actors in mobile health monitoring systems. Int. J. Med. Inform. 133, 104017. doi:10.1016/j.ijmedinf.2019.104017
Hartvigsen, C. B., Malik, H., Mahtab Alam, M., Le Moullec, Y., Parand, S., and Mumtaz, S. (2019). Narrowband internet of things (nb-iot): from physical (phy) and media access control (mac) layers perspectives. Sensors 19, 2613. doi:10.3390/s19112613
Naik, N. (2017). “Choice of effective messaging protocols for iot systems: mqtt, coap, amqp and http,” in 2017 IEEE international systems engineering symposium (ISSE), Vienna, AT, Octomber 11–13, 2017 (New York, NY: IEEE), 1–6.
Nappi, I., and de Campos Ribeiro, G. (2020). Internet of things technology applications in the workplace environment: a critical review. J. Corporate Real Estate [Epub ahead of print]. doi:10.1108/JCRE-06-2019-0028
Nasajpour, M., Pouriyeh, S., Parizi, R. M., Dorodchi, M., Valero, M., and Arabnia, H. R. (2020). Internet of things for current covid-19 and future pandemics: an exploratory study. J. Healthc Inform. Res. 12, 1–40. [Epub ahead of print]. doi:10.1007/s41666-020-00080-6
Nitu, A. M., Hasan, M. J., and Alom, M. S. (2019). “Wireless home automation system using iot and paas,” in 2019 1st international conference on advances in science, engineering and robotics technology(ICASERT), Dhaka, Bangladesh, May 3–5, 2019 (New York, NY: IEEE), 1–6.
Olatinwo, D. D., Abu-Mahfou, A. M., and Hancke, G. P. (2020). A hybrid multi-class mac protocol for iot-enabled wban systems. IEEE Sensors J. 21, 6761–6774. doi:10.1109/JSEN.2020.3037788
Omoyibo, M. O., Al-Hadhrami, T., Olajide, F., Lotfi, A., and Elmisery, A. M. (2019). “Sensor network in automated hand hygiene systems using iot for public building,” in International conference of reliable information and communication technology, Johor, MA, September 22–23, 2019 (New York, NY: Springer), 463–476.
Osisanwo, F., Kuyoro, S., and Awodele, O. (2015). “Internet refrigerator–a typical internet of things (iot),” in 3rd international conference on advances in engineering sciences & applied mathematics (ICAESAM’2015), London, United Kingdom, March 23–24, 2015 (London United Kingdom: International Institute of Engineers).
Park, A. (2020). As the first coronavirus vaccine human trials begin, manufacturer is already preparing to scale production to millions. Available at: https://time.com/5807669/coronavirus-vaccine-moderna/ (Accessed June 16, 2020
Peak, C. M., Kahn, R., Grad, Y. H., Childs, L. M., Li, R., Lipsitch, M., et al. (2020). Comparative impact of individual quarantine vs. active monitoring of contacts for the mitigation of covid-19: a modelling study. medRxiv[Preprint] (Accessed April 26, 2020).
Plagg, B., Engl, A., Piccoliori, G., and Eisendle, K. (2020). Prolonged social isolation of the elderly during covid-19: between benefit and damage. Arch. Gerontol. Geriatr. 89, 104086. doi:10.1016/j.archger.2020.104086
Rahman, N. A., Saad, N., Abdullah, A., and Wahab, F. A. (2018). The internet of things beverages bottle shape defect detection using na;;ıve bayes classifier. Int. J. Hum. Tech. Interaction (Ijhati) 2, 71–76.
Ralevski, M., and Stojkoska, B. R. (2019). “Iot based system for detection of gas leakage and house fire in smart kitchen environments,” in 2019 27th telecommunications forum (TELFOR), Belgrade, SRB, November 26–27, 2019 (New York, NY: IEEE), 1–4.
Ramalho, J. F., Correia, S. F., Fu, L., Dias, L. M., Adão, P., Mateus, P., et al. (2020). Super modules-based active qr codes for smart trackability and iot: a responsive-banknotes case study. npj Flexible Electron. 4, 1–9. doi:10.1038/s41528-020-0073-1
Ramírez-Faz, J., Fernández-Ahumada, L. M., Fernández-Ahumada, E., and López-Luque, R. (2020). Monitoring of temperature in retail refrigerated cabinets applying iot over open-source hardware and software. Sensors 20, 846. doi:10.3390/s20030846
Ramson, S. J., Vishnu, S., and Shanmugam, M. (2020). “Applications of internet of things (iot)–an overview,” in 2020 5th international conference on devices, circuits and systems(ICDCS), Coimbatore, India, March 5–6, 2020 (New York, NY: IEEE), 92–95.
Ravekar, U., and Singh, S. (2019). Data centric smart restaurant management system. Int. Res. J. Eng. Tech. (Irjet). 6, 1425.
Ray, P. P., Thapa, N., Dash, D., and De, D. (2019). Novel implementation of iot based non-invasive sensor system for real-time monitoring of intravenous fluid level for assistive e-healthcare. Circuit World 45, 109–123. doi:10.1108/CW-01-2019-0008
Ren, H., Pan, C., Deng, Y., Elkashlan, M., and Nallanathan, A. (2020). Resource allocation for secure urllc in mission-critical iot scenarios. IEEE Trans. Commun. 68, 5793–5807. doi:10.1109/tcomm.2020.2999628
Rincon, J. A., Costa, A., Carrascosa, C., Novais, P., and Julian, V. (2019). EMERALD-exercise monitoring emotional assistant. Sensors 19, 1953. doi:10.3390/s19081953
Rohan, A., Rabah, M., Asghar, F., Talha, M., and Kim, S-H. (2019). Advanced drone battery charging system. J. Electr. Eng. Technol. 14, 1395–1405. doi:10.1007/s42835-019-00119-8
Rubio-Drosdov, E., Díaz-Sánchez, D., Almenárez, F., Arias-Cabarcos, P., and Marín, A. (2017). Seamless human-device interaction in the internet of things. IEEE Trans. Consumer Electron. 63, 490–498. doi:10.1109/tce.2017.015076
Saeed, H., Shouman, A., Elfar, M., Shabka, M., Majumdar, S., and Horng-Lung, C. (2016). “Near-field communication sensors and cloud-based smart restaurant management system,” in IEEE 3rd World Forum on Internet of Things (WF-IoT), Reston, United States, December 12–14, 2016 (New York, NY: IEEE), 686–691.
Saez, M., Maturana, F. P., Barton, K., and Tilbury, D. M. (2018). Real-time manufacturing machine and system performance monitoring using internet of things. IEEE Trans. Automat. Sci. Eng. 15, 1735–1748. doi:10.1109/tase.2017.2784826
Sahoo, K. C., and Pati, U. C. (2017). “Iot based intrusion detection system using pir sensor,” in 2017 2nd IEEE international conference on recent trends in electronics, information & Communication Technology (RTEICT, Bangalore, India, May 19–20, 2017 (New York, NY: IEEE), 1641–1645.
Salah Uddin, M., Khan, A. D, and Khan, N. (2019). Kitchen grocery items monitoring system based on internet of things. Int. J. Comput. Netw. Tech. 7, 47–52. 10.12785/ijcnt/070202
Salvo Rossi, P., Ciuonzo, D., and Romano, G. (2013). Orthogonality and cooperation in collaborative spectrum sensing through MIMO decision fusion. IEEE Trans. Wireless Commun. 12, 5826–5836. doi:10.1109/twc.2013.092013.130279
Siddiqui, S., Khan, A. A., Farid, N., and Dey, I. (2021). “Anxiety and depression management for elderly using internet of things and symphonic melodies.” in IEEE ICC 2021 - SAC-03 EH track, 14th–23rd June, 2021, Montreal, Canada. (New York, NY: IEEE), 6.
Singh, R. P., Javaid, M., Haleem, A., and Suman, R. (2020). Internet of things (iot) applications to fight against covid-19 pandemic. Diabetes Metab Syndr. 14, 521–524. doi:10.1016/j.dsx.2020.04.041
Sinha, D., Cottur, K., Bhat, K., Guruprasad, C., and Badari, N. (2019). “Automated billing system using rfid and cloud,” in 2019 Innovations in power and advanced computing technologies (i-PACT), Vellore, India, March 22–23, 2019 (New York, NY: IEEE), 1–6.
Siriwardhana, Y., De Alwis, C., Gür, G., Ylianttila, M., and Liyanage, M. (2020). The fight against the covid-19 pandemic with 5g technologies. IEEE Eng. Manag. Rev. 48, 72–84. doi:10.1109/emr.2020.3017451
Song, Y., Jiang, J., Wang, X., Yang, D., and Bai, C. (2020). Prospect and application of internet of things technology for prevention of saris. Clin. eHealth 3, 1–4. doi:10.1016/j.ceh.2020.02.001
Spice, B. (2020). COVID-19 should be wake-up call for robotics research. Available at: https://www.cmu.edu/news/stories/archives/2020/march/coronavirus-a-wakeup-call-for-robotics-research.html (Accessed May 09, 2020).
Sruthi, M. S. (2019). Iot based real time people counting system for smart buildings. Int. J. Emerging Tech. Innovative Eng. 5, 83–86.
Sunchu, R., Palli, S., Datta, V. S. R., and Shanmugasundaram, M. (2019). “Intelligent system for office environment using internet of things,” in 2019 3rd international conference on trends in electronics and informatics (ICOEI), Tirunelveli, India, April 23–25, 2019 (New York, NY: IEEE), 717–721.
Sung, W. T., and Lu, C. Y. (2018). “Smart warehouse management based on iot architecture,” in 2018 International symposium on computer, consumer and control (IS3C), Taichung, TW, December 6–8, 2018 (New York, NY: IEEE), 169–172.
Sunyaev, A. (2020). “The internet of things,” In Internet Computing. (New York, NY: Springer), 301–337.
Suryaatmadja, S., and Maulani, N. (2020). Contributions of space technology to global health in the context of covid-19. Jaki 8, 60–73. doi:10.20473/jaki.v8i2.2020.60-73
TechRadar (2020). Indian government launches aarogya setu app to track coronavirus infections. Available at: https://www.techradar.com/news/indian-government-launches-aarogya-setu-app-to-crack-coronavirus-infections (Accessed May 03, 2020).
Thantharate, A., Paropkari, R., Walunj, V., Beard, C., and Kankariya, P. (2020). “Secure5g: a deep learning framework towards a secure network slicing in 5g and beyond,” in 2020 10th annual computing and communication workshop and conference (CCWC), Las Vegas, United States, January 6–8, 2020 (New York, NY: IEEE), 0852–0857.
Thiagarajan, G., and Murthy, C. R. (2015). Novel transmit precoding methods for Rayleigh fading multiuser TDD-MIMO systems with CSIT and no CSIR. IEEE Trans. Veh. Technol. 64, 973–984. doi:10.1109/tvt.2014.2325778
Thienemann, F., Pinto, F., Grobbee, D. E., Boehm, M., Bazargani, N., Ge, J., et al. (2020). World heart federation briefing on prevention: coronavirus disease 2019 (covid-19) in low-income countries. Glob. Heart 15, 31. doi:10.5334/gh.778
Ting, D. S. W., Carin, L., Dzau, V., and Wong, T. Y. (2020). Digital technology and covid-19. Nat. Med. 26, 459–461. doi:10.1038/s41591-020-0824-5
Utomo, B., Luthfiyah, S., Mudjiono, U., and Hamzah, T. (2019). The real-time vital sign monitor for heart rate and spo2 parameter using internet of things technology. J. Phys. Conf. Ser., 1373, 012028. doi:10.1088/1742-6596/1373/1/012028
Vaishya, R., Javaid, M., Khan, I. H., and Haleem, A. (2020). Artificial intelligence (ai) applications for covid-19 pandemic. Diabetes Metab. Syndr. 14, 337–339. doi:10.1016/j.dsx.2020.04.012
Vijayaraghavan, V., and Leevinson, J. R. (2019). “Internet of things applications and use cases in the era of industry 4.0,” in The internet of things in the industrial sector. (New York, NY: Springer), 279–298.
WHO (2020a). 10th ebola outbreak in the democratic republic of the congo declared over; vigilance against flare-ups and support for survivors must continue. Available at: https://www.who.int/news-room/detail/25-06-2020-10th-ebola-outbreak-in-the-democratic-republic-of-the-congo-declared-over-vigilance-against-flare-ups-and-support-for-survivors-must-continue (Accessed July 13, 2020).
WHO (2020b). Coronavirus disease 2019 (COVID-19) situation report 87. Available at: https://www.who.int/docs/default-source/coronaviruse/situation-reports/20200416-sitrep-87-covid-19.pdf?sfvrsn=9523115a2 (Accessed June 14, 2020).
WHO (2020c). Coronavirus disease (COVID-19) advice for the public: when and how to use masks. Available at: https://www.who.int/emergencies/diseases/novel-coronavirus-2019/advice-for-public/when-and-how-to-use-mask (Accessed June 20, 2020).
WHO (2020d). Draft landscape of COVID-19 candidate vaccines. Available at: https://www.who.int/publications/m/item/draft-landscape-of-covid-19-candidate-vaccines (Accessed June 16, 2020).
WHO (2020e). Laboratory testing for coronavirus disease 2019 (COVID-19) in suspected human cases. Available at: https://www.who.int/publications/i/item/10665-331501 (Accessed June 14, 2020).
WHO (2020f). Report who-China joint mission coronavirus disease 2019 (covid-19). Available at: https://www.who.int/docs/default-source/coronaviruse/who-china-joint-mission-on-covid-19-final-report.pdf (Accessed June 25, 2020).
WHO (2020g). Welcome to the WHO ICTRP. Available at: https://www.who.int/ictrp/en/ (Accessed June 16, 2020).
Worldometer (2020). Covid-19 coronavirus pandemic. Available at: https://www.worldometers.info/coronavirus/ (Accessed May 13, 2020).
Xia, Y., and Lee, G. (2020). How to return to normalcy: fast and comprehensive contact tracing of covid-19 through proximity sensing using mobile devices. arXiv preprint arXiv:2004.12576 (Accessed April 27, 2020).
Xu, X., Schroeder, F., Gevrekce, B., Kutrolli, G., Ni, M., and Mathar, R. (2013). A physical layer simulator based on radio wave propagation for lte cellular networks,” in 2013 7th European Conference on Antennas and propagation (EuCAP), Gothenburg, SE, 8–12 April 2013 (New York, NY: IEEE), 1007–1010.
Xu, H., Zhang, L., Onireti, O., Fang, Y., Buchanan, W. B., and Imran, M. A. (2020). Beeptrace: blockchain-enabled privacy-preserving contact tracing for covid-19 pandemic and beyond. IEEE Internet Things J. 8, 3915–3929. doi:10.1109/smartiot49966.2020.00036
Ye, Q., Zhou, J., and Wu, H. (2020). Using information technology to manage the covid-19 pandemic: development of a technical framework based on practical experience in China. JMIR Med. Inform. 8, e19515. doi:10.2196/19515
Yew, H. T., Ng, M. F., Ping, S. Z., Chung, S. K., Chekima, A., and Dargham, J. A. (2020). “Iot based real-time remote patient monitoring system,” in 2020 16th IEEE international colloquium on signal processing & its applications (CSPA), Langkawi, MY, February 28–29, 2020 (New York, NY: IEEE), 176–179.
Zallio, M., McGrory, J., and Berry, D. (2020). “How to democratize internet of things devices: a participatory design study to improve digital literacy,” in International conference on applied human factors and ergonomics, 16th–20th July, 2020, San Diego, CA, (New York, NY: Springer), 139–150.
Zhong, X., Li, L., Zhang, S., and Lu, R. (2020). Ecor: an energy aware coded opportunistic routing for cognitive radio social internet of things. Wireless Pers Commun. 110, 1–20. doi:10.1007/s11277-019-06708-0
Zou, Y., Zhu, J., and Jiang, X. (2019). Joint power splitting and relay selection in energy-harvesting communications for IoT networks. IEEE Internet Things J. 7, 584–597. 10.1109/JIOT.2019.2946651
Keywords: pandemic, architecture, IoT, sensors, network, COVID-19
Citation: Siddiqui S, Shakir MZ, Khan AA and Dey I (2021) Internet of Things (IoT) Enabled Architecture for Social Distancing During Pandemic. Front. Comms. Net 2:614166. doi: 10.3389/frcmn.2021.614166
Received: 05 October 2020; Accepted: 15 February 2021;
Published: 15 April 2021.
Edited by:
Akram Alomainy, Queen Mary University of London, United KingdomReviewed by:
Masood Ur Rehman, University of Glasgow, United KingdomZia Ullah Khan, Queen Mary University of London, United Kingdom
Copyright © 2021 Siddiqui, Shakir, Khan and Dey. This is an open-access article distributed under the terms of the Creative Commons Attribution License (CC BY). The use, distribution or reproduction in other forums is permitted, provided the original author(s) and the copyright owner(s) are credited and that the original publication in this journal is cited, in accordance with accepted academic practice. No use, distribution or reproduction is permitted which does not comply with these terms.
*Correspondence: Shama Siddiqui, shamasid@hotmail.com