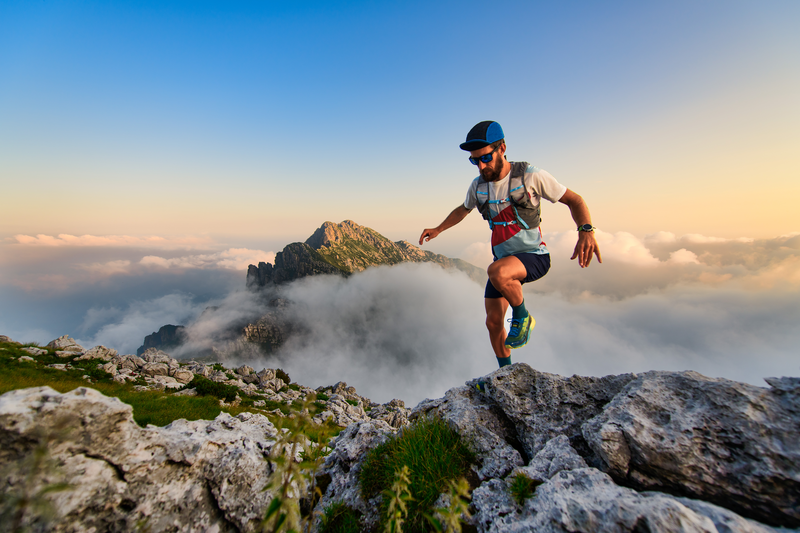
94% of researchers rate our articles as excellent or good
Learn more about the work of our research integrity team to safeguard the quality of each article we publish.
Find out more
MINI REVIEW article
Front. Commun. , 05 February 2025
Sec. Science and Environmental Communication
Volume 10 - 2025 | https://doi.org/10.3389/fcomm.2025.1518768
The global climate is changing rapidly, with cascading impacts across the world. Even though the modern instrument-based record of Earth observations reflects decades of critical work, multi-century time series may be required to understand and forecast key elements of Earth system dynamics. Here, we review the potential uses of non-traditional climate data records—observations reported without using modern instruments or standardized measurement protocols—to identify climate and ecosystem dynamics that predate modern methodologies and tools. We compile a list of diverse datasets collected over more than 500 years, including landscape paintings, sea lore, and animal migration data. This initial review presents opportunities for further investigation to reconstruct past climate or to use non-traditional records to complement modern instrument methods.
Modern Earth system instrumentation and recordkeeping began in the late 1800s, with the Metre Convention in Paris on May 20, 1875 that established a standardized set of international weights and measures (Ortiz and Jackson, 2022). The 1700s and 1800s were crucial for European naturalists and emerging paleoclimatologists as they characterized the ice ages, evolution, and speciation (Stone, 1913; Hankins, 2015). Instruments to directly measure atmospheric gases, plant respiration, light refraction, and many other dynamics began to emerge, as scientists tracked precipitation, temperature, circulation, vegetation ranges, and animal movement utilizing standardized methodology (Edwards, 2011).
Today, as forecasting the range of potential climate extremes becomes critical, scientists must understand both the baselines and boundaries of physical and ecological dynamics. In many cases, long-term historical data is required as inputs to predictive models for forecasting climate change. For example, over 300 years of regional ecological data may be required to characterize critical global Earth system tipping points (Lenton et al., 2019), and ongoing system dynamics (Lenton et al., 2024; Miner et al., 2024), informing models on topics as diverse as regional temperature trends, sea level rise impacts, and glacier retreat timing. To forecast these physical dynamics, baselining data that predate the 19th-century scientific instrumentation revolution are required.
To date, the scientific community has often relied on global paleoclimate proxies including ice cores, tree rings, and isotopes as historical inputs for climate and ecosystem models (Winski et al., 2017; Christensen, 1993; Putnam et al., 2016). While these paleoclimate datasets store critical information, multi-decadal trends are often the easiest to derive. For example, while glacial ice cores may provide information about a particular glacier in addition to larger regional snowfall and temperature trends, larger-scale trends over multiple years in the global climate are most pronounced. Many proxies do not provide seasonal data, particularly for specific regions of interest, and there are substantial complexities in preserving and analyzing the records (Putnam et al., 2016; Pelletier et al., 2019; Birkel et al., 2011; Gajurel et al., 2020; Huhtamaa and Helama, 2017; Ungar et al., 2021).
Further, while the diversity of data available from modern satellite, airborne, drone, and in-situ measurements continues to grow (Bartsch et al., 2023; Schimel and Schneider, 2019), these data cannot capture changes predating the technological instrument expansion of the 1950’s (Miner et al., 2023). This dearth of information for baselining Earth system dynamics past the mid 1900’s increases uncertainty in forecasting the impacts of climate change.
As ecosystem baselines shift on even regional and seasonal scales (Lenton et al., 2019; Turner et al., 2020), there is increased urgency to fill the gaps between modern records and lower-resolution paleoclimate data. Expanding Earth system records with non-traditional data that spans centuries could support a greater understanding of current environmental change (Turner et al., 2020; Keeley, 2002). Therefore, data that can supplement and extend the instrument records past the last few centuries are necessary to support climate change planning.
Though not typically regarded as scientific data, non-traditional climate data including oral histories, personal written accounts, and art may inadvertently or directly record key aspects of long-term climate dynamics. From sea ice boundary observations to the seasonality of vernal flowering, pre-industrial lore and art documented weather, climate, and animal movement. Records of seafarers, land trade routes, crop surpluses and failures, and fishing hauls proliferate across cultures (Table 1; Figure 1). Though our understanding of the drivers of natural change has evolved, important data may underlie oral tradition and historical records.
Figure 1. Non-traditional climate data records are found in the oral and written traditions of cultures worldwide. This map marks the locations of the records listed throughout this manuscript.
While these records are often considered more a part of cultural than scientific tradition, folk or non-traditional data that predate modern scientific methods may be able to extend the current climate and ecosystem records through specific and careful application (Canavera, 2021; Bartlett et al., 2012; Canavera, 2023). For example, while traditional climate proxies tell us that our current rate of atmospheric warming is the most rapid in planetary history (Winski et al., 2017; Santibáñez et al., 2018), could non-traditional data help us validate or falsify assumptions, point us toward additional key research areas, or fill knowledge gaps?
Observations from British ship logs have already filled gaps in the Indian Ocean record for sea surface temperatures (Ingold, 2010). Style and color changes in Monet and Turner’s paintings have been used to infer trends in air pollution during the Industrial Revolution (Martin and Barboza, 2020). Glacier landscapes in paintings have been used to extend photographic records of long-term glacier retreat (Lacina and Halas, 2015; Winiger et al., 2019). Despite these successes, non-traditional climate data have not been applied to many critical climate change questions due to the challenges in confirming the authenticity and accuracy of the records. For example, it may be difficult to ascertain the influence of key artistic choices or elements driven by imagination or cultural expectations. As a result, the applied uses of oral, written and artistic records may be often overlooked.
This review is a first effort to identify and catalog a wide range of non-traditional climate and ecosystem data from diverse sources that could be applied to extend the instrument record or pre-screen modern remote sensing retrievals. The compiled records span fine art (Lacina and Halas, 2015; McLoughlin, 1999; Farag-Miller et al., 2013; Metzger, 2020; Sillasoo, 2014; Rees, 2008; Rudolph, 1966; Ingold, 2010), shipping manifests (Christensen, 1993; D’Alto, 2007; García-Herrera et al., 2018; Catchpole and Faurer, 1985; Teillet, 1988), oral and written compendia, species movement records (Stone, 1913; Stejneger, 1905; Arnott, 2007; Linglin et al., 2020; Reeb et al., 2020; Martin et al., 2018; Martin and Barboza, 2020), and trade reports (Sahrhage and Lundbeck, 1992; Wilcox, 1982; Jurabek, 2022). While not geographically or temporally comprehensive, the non-traditional data reviewed here offer a perspective of the possible climate applications in specific regions. We highlight these records as an example of diverse non-traditional data that could be useful in establishing regional ecological and climate baselines that predate instrument records (Miner et al., 2023).
While each non-traditional dataset will need to be assessed individually for utility and cross-checked for accuracy, any opportunity to extend the climate record cannot be overlooked. If historical non-traditional data sources can provide an additional index for climate change, the observations and stories of diverse naturalists, healers, artists, historians and explorers across the globe could supplement modern observations.
Starting with the Voyages of Discovery in the 1500s and transitioning into global commerce in the 1600s, European merchant and naval vessels regularly recorded weather information, including precipitation, atmospheric conditions, sea ice extent, and sea surface temperature (D’Alto, 2007). These records contain numerous observations across the global oceans and are of great potential value. To that end, several international projects, such as the Climatological Database for the World’s Oceans (CLIWOC) and the Recovery of Logbooks and International Marine Data (RECLAIM), have digitized thousands of shipboard logbooks. Most of these records are now stored in the International Comprehensive Ocean–Atmosphere Data Set (ICOADS), providing surface marine data from as early as 1,662 (García-Herrera et al., 2018; Brohan et al., 2009). Together, these programs extracted millions of observations on sea surface temperature, sea level pressure, wind force, atmospheric circulation indices, and weather conditions (García-Herrera et al., 2018; Brohan et al., 2009; Woodruff et al., 2005). The application of these logbooks has so far included reconstructions of sea ice conditions in the Arctic (Catchpole and Faurer, 1985; Teillet, 1988), understanding baseline ice and snow cover thickness in the Antarctic (Worby et al., 2008), and knowledge of historical hurricanes and monsoons (García-Herrera et al., 2018; Zooniverse, n.d.).
However, investigations have also revealed data inconsistencies due to variations in observational methods across time and between ships (Woodruff et al., 2005). Early ship observations primarily consisted of subjective meteorological descriptions. The Beaufort wind scale was not commonly used until the 1840s, and instrumental data did not become widespread until barometer and thermometer reporting practices were standardized in 1853 (Woodruff et al., 2005). As a result, digitizing subjective records remains extremely labor-intensive, in some cases requiring the support of citizen science, (Zooniverse, n.d.) or can require expertise in deciphering archaic terminology across numerous languages. Despite these limitations, ship logbooks have already provided critical observations that predate modern ocean observations. With extensive collections of logbooks still unprocessed, future efforts will continue to expand the range and usefulness of this climate record.
Landscape paintings can also provide snapshots of the natural world before modern instrumental records, and recent analysis has illustrated their utility in reconstructing past environments (Lacina and Halas, 2015; Farag-Miller et al., 2013; Metzger, 2020). Many paintings, especially from artists with topographical landscape training, may display consistent and accurate observations of surface conditions, vegetation, species, and habitat (Lacina and Halas, 2015; McLoughlin, 1999; Farag-Miller et al., 2013).
Researchers have identified reliable representations of cloud formation, weather conditions, atmospheric phenomena, land cover and ice extent which can be used to draw inferences about long-term change and seasonal trends (Ossing and Brauer, 2006; Robinson, 2005). For example, impressionist paintings in London and Paris over the 19th century accurately captured changes to the optical environment due to anthropogenic aerosol emissions, recently providing evidence for historical trends in air pollution before quantitative measurements (Albright and Huybers, 2023). In another example, the color of snow, ice, and watercolor in polar paintings may reveal information about glaciers’ reflectance and health (Gapp, 2021). Yet, analyses also indicate that some paintings do not depict the landscape entirely faithfully (Ossing and Brauer, 2006), overrepresent certain climatic conditions (Metzger, 2020), or romanticize natural features like fjords and glaciers (Gapp, 2021). The artist’s subjective interpretation or depiction of the scene may also influence the painting’s color, shape, or state, reflecting a stylistic interpretation rather than a direct copy of the landscape at the time of capture. Although more work is needed to assess how art can inform quantitative science, paintings and other art forms may provide critical insights into ecosystems, land use, and ecosystem change through the centuries.
Archival records and oral knowledge may also have considerable scientific value. For example, observations from old diaries of residents and colonists have been used to characterize the vegetation and landscape of the Mexican Bajío in the 16th century (Butzer and Butzer, 1997; Hill, 1992), to track the first arrivals of migrating birds (Stone, 1913; Stejneger, 1905; Arnott, 2007), and to reconstruct changes in spring mean temperatures using phenological data deduced from cherry blossom records and viewing parties in Japan (Aono, 2015).
Many historical accounts could also provide continuous environmental records over decades to centuries. For instance, the high demand for timber for shipbuilding worldwide in the 1500–1900s led to detailed national records and surveys of trees, records which could be used to assess ecological forest changes (Carroll, 1981; Eliasson and Nilsson, 2002). Similarly, centuries of fishery catch data could provide insights into fish population abundances or shifts in aquatic ecosystems (Wilcox, 1982; Izzo et al., 2016). Beyond these written sources, ethical incorporation of Indigenous community knowledge provides a baseline for understanding regional ecosystems across scales (Feinberg et al., 2003; Harden, 2022).
Finally, archeological and paleoclimate studies are critical in understanding ancient ecosystem structure, human history, and responses to environmental change. For example, archeological research on prehistoric coastal settlements has provided evidence of anthropogenic influence on marine ecosystems, improving the accuracy of baselines for fisheries management (Izzo et al., 2016; Erlandson and Rick, 2010). Historical trends in crop cultivation and forestry could be supplemented with studies of tree ring density to determine weather and climate controls on growth at hyper-local scales (Christensen, 1993; Huhtamaa and Helama, 2017).
Paleoclimate methods provide excellent proxy data for precipitation, water availability, humidity, and seasonal temperatures (Putnam et al., 2016; Douglas et al., 2015; Oh, 2013; Patterson et al., 2010). Combined with historical documentation, these data can reveal vital insights into the impact of the climate on ancient societies. Studies have shown that during wet phases, the Maya experienced rapid growth, while multi-decadal droughts corresponded with social instability, depopulation, and collapse (Douglas et al., 2015; Oh, 2013; Ebert et al., 2017; Scarborough and Gallopin, 1991). Similarly, the failure of Norse colonies and Northern European communities often coincided with a prolonged period of low temperatures (Huhtamaa and Helama, 2017; Patterson et al., 2010; Worth, 1990; Guarin et al., 2020; Osmaston, 1985), or sea level rise (Borreggine et al., 2023), and the Mongol Empire’s expansion followed the spread of steppe grassland after the wet conditions of the Little Ice Age (Putnam et al., 2016).
Adding relevant non-traditional data to modern instrumental records could considerably expand the baseline of documented climate conditions or provide an index for identifying hyper-local changes. To continue to expand the breadth of records available, identifying potential non-traditional records and integrating them with modern tools will prove essential.
To forecast the impacts of climate change, we must fully understand the recent and distant history of physical and ecosystem processes. Reconstructing the climate signal at spatiotemporal scales between the paleo record and modern instrument science has been an ongoing challenge, increasing uncertainty. It is possible that with the careful application of non-traditional data records, key information could be extracted to reconstruct past climate and provide context for contemporary observations.
To successfully apply these non-traditional data, it will be critical to develop methodologies for standardizing and scaling records across regions and observers. For example, using industrial fish haul records together with seasonal fishing lore could help illuminate the ecosystem dynamics of a specific place and time. In some cases, these records may reflect the cultural traditions or colonialism of a specific time and must be taken within the context of a greater historical perspective. In this regard, pre-colonial written or oral records from many cultures could be compiled through additional effort within specific local archives.
A methodology incorporating cross-verification could strengthen the applicability of non-traditional data and help identify patterns of bias or subjectivity, using stories and measurements from one discipline to understand the veracity of another. To accomplish this, diverse non-traditional data from the same region could be applied to research questions, informing the context and characterizing data gaps. Similar normalizing techniques for standardizing non-traditional data have been used for specific use cases (Martin and Barboza, 2020; Robinson, 2005; Albright and Huybers, 2023; Erlandson and Rick, 2010) and could be applied more broadly.
The use of artificial intelligence and image extraction tools would also be of specific benefit when characterizing both the availability and content of non-traditional data. Applying AI tools would allow the extraction of content from paintings and records recorded as copies or images. While citizen science projects have successfully implemented volunteers to identify difficult to read data including sea records of monsoons and fish hauls (Zooniverse, n.d.), identifying gaps and trends across the resulting large datasets is an ideal application for AI (Gay et al., 2023). As AI becomes increasingly useful for interpreting large datasets, the most modern tools help us understand records from the distant past.
Folklore endures. Though records have been lost to fires, plagues, violence, and colonization, many stories passed down through generations persist. Non-traditional data could fill gaps in understanding biodiversity and landcover change, provide a longer record to strengthen model projections, and baseline changing ecosystems (Lenton et al., 2019; Heinze et al., 2021). In this time of unprecedented environmental change, it is more important than ever to elevate and utilize all the ways of knowing and understanding the Earth, allowing the distant past to help us prepare for the future.
KM: Conceptualization, Data curation, Formal analysis, Funding acquisition, Investigation, Methodology, Project administration, Resources, Software, Supervision, Validation, Visualization, Writing – original draft, Writing – review & editing. EW: Formal analysis, Investigation, Writing – original draft, Writing – review & editing. BG: Conceptualization, Data curation, Formal analysis, Funding acquisition, Investigation, Methodology, Writing – original draft, Writing – review & editing. CM: Conceptualization, Funding acquisition, Project administration, Resources, Software, Supervision, Validation, Visualization, Writing – review & editing.
The author(s) declare that financial support was received for the research, authorship, and/or publication of this article. A portion of this work was carried out at the Jet Propulsion Laboratory, California Institute of Technology, under a contract with the National Aeronautics and Space Administration (80NM0018D0004). JPL is within the unceded land of the people known as the Tongva (Gabrielieño) within the limits of the Kizh Nation. © 2024. All rights reserved.
The authors declare that the research was conducted in the absence of any commercial or financial relationships that could be construed as a potential conflict of interest.
The authors declare that no Gen AI was used in the creation of this manuscript.
All claims expressed in this article are solely those of the authors and do not necessarily represent those of their affiliated organizations, or those of the publisher, the editors and the reviewers. Any product that may be evaluated in this article, or claim that may be made by its manufacturer, is not guaranteed or endorsed by the publisher.
Albright, A. L., and Huybers, P. (2023). Paintings by Turner and Monet depict trends in 19th century air pollution. Proc. Natl. Acad. Sci. 120:e2219118120. doi: 10.1073/pnas.2219118120
Aono, Y. (2015). Cherry blossom phenological data since the seventeenth century for Edo (Tokyo), Japan, and their application to estimation of march temperatures. Int. J. Biometeorol. 59, 427–434. doi: 10.1007/s00484-014-0854-0
Bartlett, C., Marshall, M., and Marshall, A. (2012). Two-eyed seeing and other lessons learned within a co-learning journey of bringing together indigenous and mainstream knowledges and ways of knowing. J. Environ. Stud. Sci. 2, 331–340. doi: 10.1007/s13412-012-0086-8
Bartsch, A., Strozzi, T., Ingmar Nitze, I., and De Nitze, I. N. (2023). Permafrost monitoring from space. Surv. Geophys. 44, 1579–1613. doi: 10.1007/s10712-023-09770-3
Birkel, S. D., Putnam, A. E., Denton, G. H., Koons, P. O., Fastook, J. L., Maasch, K. A., et al. (2011). Climate inferences from a glaciological reconstruction of the late pleistocene wind river ice cap, wind river range, Wyoming. Arctic Antarctic Alpine Res. 44, 265–276. doi: 10.1657/1938-4246-44.3.265
Borreggine, M., Latychev, K., Coulson, S., Powell, E. M., Mitrovica, J. X., Milne, G. A., et al. (2023). Sea-level rise in Southwest Greenland as a contributor to Viking abandonment. Proc. Natl. Acad. Sci. 120:e2209615120. doi: 10.1073/pnas.2209615120
Brohan, P., Allan, R., Freeman, J. E., Waple, A. M., Wheeler, D., Wilkinson, C., et al. (2009). Marine observations of old weather. Bull. Am. Meteorol. Soc. 90, 219–230. doi: 10.1175/2008BAMS2522.1
Butzer, K. W., and Butzer, E. K. (1997). The ‘natural’ vegetation of the Mexican Bajío: archival documentation of a 16th-century savanna environment. Quat. Int. 43-44, 161–172. doi: 10.1016/S1040-6182(97)00032-3
Canavera, L. (2021). Blue economy solutions to the challenges in our food system. Arctic Circle Assembly. Available at: https://www.youtube.com/watch?v=iWwkv96CCPk (Accessed August 2, 2024).
Canavera, L. (2023). Blending indigenous knowledge and artificial intelligence to enable adaptation. Circle 12–13.
Carroll, C. F. (1981). Wooden ships and American forests. J. For. Hist. 25, 213–215. doi: 10.2307/4004612
Catchpole, A. J. W., and Faurer, M.-A. (1985). Ships’ log-books, sea ice and the cold summer of 1816 in Hudson Bay and its approaches. Arctic 38, 121–128. doi: 10.14430/arctic2121
Christensen, A. E. (1993). Dendrochronological dating of the VIKING Age ship burials at Oseberg, Gokstad and Tune, Norway. Antiquity 67, 575–583.
D’Alto, N. (2007). 100 years before the mast: in the hands of climatologists and historians, centuries-old ships’ logs are revealing past—and maybe future—weather. Weatherwise 60, 26–33. doi: 10.3200/WEWI.60.3.26-33
Douglas, P. M. J., Pagani, M., Canuto, M. A., Brenner, M., Hodell, D. A., Eglinton, T. I., et al. (2015). Drought, agricultural adaptation, and sociopolitical collapse in the Maya lowlands. Proc. Natl. Acad. Sci. 112, 5607–5612. doi: 10.1073/pnas.1419133112
Ebert, C. E., Peniche May, N., Culleton, B. J., Awe, J. J., and Kennett, D. J. (2017). Regional response to drought during the formation and decline of Preclassic Maya societies. Quat. Sci. Rev. 173, 211–235. doi: 10.1016/j.quascirev.2017.08.020
Edwards, P. N. (2011). History of climate modeling. Wiley Interdiscip. Rev. Clim. Chang. 2, 128–139. doi: 10.1002/wcc.95
Eliasson, P., and Nilsson, S. G. (2002). ‘You should hate young oaks and young noblemen’: the environmental history of oaks in eighteenth-and nineteenth-century Sweden. Environ. Hist. 7, 659–677. doi: 10.2307/3986064
Erlandson, J. M., and Rick, T. C. (2010). Archaeology meets marine ecology: the antiquity of maritime cultures and human impacts on marine fisheries and ecosystems. Annu. Rev. Mar. Sci. 2, 231–251. doi: 10.1146/annurev.marine.010908.163749
Farag-Miller, M., Miller, K., and Kirkpatrick, J. B. (2013). Determining the accuracy of historical landscape paintings. Geogr. Res. 51, 49–58. doi: 10.1111/j.1745-5871.2012.00763.x
Feinberg, R., Dymon, U. J., Pu, P., Pu, R., Pu, N., and Rollins, M. (2003). “Drawing the coral heads”: mental mapping and its physical representation in a Polynesian community. Cartogr. J. 40, 243–253. doi: 10.1179/000870403225012943
Gajurel, A. P., Hubbard, M., Giri, B., Maka, S., Rafter, P. A., Putnam, A. E., et al. (2020). Sedimentology of glacial lake core from Gokyo Valley, Everest Region. Nepal: The AGU Fall meeting.
Gapp, I. (2021). A woman in the far north: Anna Boberg and the Norwegian glacial landscape. Kunst og Kultur 104, 82–96. doi: 10.18261/issn.1504-3029-2021-02-02
García-Herrera, R., Barriopedro, D., Gallego, D., Mellado-Cano, J., Wheeler, D., and Wilkinson, C. (2018). Understanding weather and climate of the last 300 years from ships’ logbooks. Wiley Interdiscip. Rev. Clim. Chang. 9:e544. doi: 10.1002/wcc.544
Gay, B. A., Pastick, N. J., Züfle, A. E., Armstrong, A. H., Miner, K. R., and Qu, J. J. (2023). Investigating permafrost carbon dynamics in Alaska with artificial intelligence. Environ. Res. Lett. 18:125001. doi: 10.1088/1748-9326/ad0607
Guarin, J. R., Asseng, S., Martre, P., and Bliznyuk, N. (2020). Testing a crop model with extreme low yields from historical district records. Field Crop Res. 249:107269. doi: 10.1016/j.fcr.2018.03.006
Hankins, T. L. (2015). Science and the enlightenment. Cambridge University Press. Available at: https://www.cambridge.org/core/books/science-and-the-enlightenment/A3ABF37F83B590987A47C099797EF4D7
Harden, M. (2022). Reawakened: traditional navigators of Te Moana-nui-a-Kiwa. Contemp. Pac. 34, 514–516. doi: 10.1353/cp.2022.0053
Heinze, C., Blenckner, T., Martins, H., Rusiecka, D., Döscher, R., Gehlen, M., et al. (2021). The quiet crossing of ocean tipping points. Proc. Natl. Acad. Sci. 118:e2008478118. doi: 10.1073/pnas.2008478118
Hill, J. H. (1992). The flower world of old Uto-Aztecan. J. Anthropol. Res. 48, 117–144. doi: 10.1086/jar.48.2.3630407
Huhtamaa, H., and Helama, S. (2017). Reconstructing crop yield variability in Finland: long-term perspective of the cultivation history on the agricultural periphery since ad 760. The Holocene 27, 3–11. doi: 10.1177/0959683616646188
Ingold, T. (2010). The temporality of the landscape. World Archaeol. 25, 152–174. doi: 10.1080/00438243.1993.9980235
Izzo, C., Doubleday, Z. A., Grammer, G. L., Gilmore, K. L., Alleway, H. K., Barnes, T. C., et al. (2016). Fish as proxies of ecological and environmental change. Rev. Fish Biol. Fish. 26, 265–286. doi: 10.1007/s11160-016-9424-3
Jurabek, K. (2022). Ancient crafts and household occupations of the in habitants of Jizzakh oasis. Eur. J. Interdis. Res. Dev. 5, 12–19.
Keeley, J. E. (2002). Native American impacts on fire regimes of the California coastal ranges. J. Biogeogr. 29, 303–320. doi: 10.1046/j.1365-2699.2002.00676.x
Lacina, J., and Halas, P. (2015). Landscape painting in evaluation of changes in landscape. J. Landscape Ecol. (Czech Republic) 8, 60–68. doi: 10.1515/jlecol-2015-0009
Lenton, T. M., Abrams, J. F., Bartsch, A., Bathiany, S., Boulton, C. A., Buxton, J. E., et al. (2024). Remotely sensing potential climate change tipping points across scales. Nat. Commun. 15:343. doi: 10.1038/s41467-023-44609-w
Lenton, T. M., Rockström, J., Gaffney, O., Rahmstorf, S., Richardson, K., Steffen, W., et al. (2019). Climate tipping points — too risky to bet against. Nature 575, 592–595. doi: 10.1038/d41586-019-03595-0
Linglin, M., Amiot, R., Richardin, P., Porcier, S., Antheaume, I., Berthet, D., et al. (2020). Isotopic systematics point to wild origin of mummified birds in ancient Egypt. Sci. Rep. 10:15463. doi: 10.1038/s41598-020-72326-7
Martin, J. M., and Barboza, P. S. (2020). Thermal biology and growth of bison (Bison bison) along the Great Plains: examining four theories of endotherm body size. Ecosphere 11:e03176. doi: 10.1002/ecs2.3176
Martin, J. M., Mead, J. I., and Barboza, P. S. (2018). Bison body size and climate change. Ecol. Evol. 8, 4564–4574. doi: 10.1002/ece3.4019
McLoughlin, L. C. (1999). Vegetation in the early landscape art of the Sydney region, Australia: accurate record or artistic licence? Landsc. Res. 24, 25–47. doi: 10.1080/01426399908706549
Metzger, A. (2020). Enjoying the ice. Dutch winter landscapes, weather and climate in the Golden age, 17th century. Climate Past Discuss., 1–14.
Miner, K. R., Braghiere, R. K., Miller, C. E., Schlegel, N., and Schimel, D. (2024). A decadal survey without analogs: earth observation needs for a warming world. AGU Adv. 5:e2023AV001148. doi: 10.1029/2023AV001148
Miner, K., Canavera, L., Gonet, J., Luis, K., Maddox, M., McCarney, P., et al. (2023). The co-production of knowledge for climate science. Nat. Clim. Chang. 13, 307–308. doi: 10.1038/s41558-023-01633-4
Ortiz, J. D., and Jackson, R. (2022). Understanding Eunice Foote’s 1856 experiments: heat absorption by atmospheric gases. Notes Rec. 76, 67–84. doi: 10.1098/rsnr.2020.0031
Osmaston, H. (1985). Crop failures on the Winchester manors 1232-1349 A. D. Trans. Inst. Br. Geogr. 10:495. doi: 10.2307/621894
Ossing, F., and Brauer, A. (2006). Contrived reality: weather and geology in Jacob van Ruisdael’s painting “view of Ootmarsum”, 1–5. Available at: http://bib.gfz-potsdam.de/pub/wegezurkunst/ootmarsum/ootmarsum_en.pdf
Patterson, W. P., Dietrich, K. A., Holmden, C., and Andrews, J. T. (2010). Two millennia of North Atlantic seasonality and implications for Norse colonies. Proc. Natl. Acad. Sci. USA 107, 5306–5310. doi: 10.1073/pnas.0902522107
Pelletier, M., Allard, M., and Levesque, E. (2019). Ecosystem changes across a gradient of permafrost degradation in subarctic Québec (Tasiapik Valley, Nunavik, Canada). Arctic Sci. 5, 1–26. doi: 10.1139/as-2016-0049
Putnam, A. E., Putnam, D. E., Andreu-Hayles, L., Cook, E. R., Palmer, J. G., Clark, E. H., et al. (2016). Little ice age wetting of interior Asian deserts and the rise of the Mongol empire. Quat. Sci. Rev. 131, 33–50. doi: 10.1016/j.quascirev.2015.10.033
Reeb, R. A., Acevedo, I., Heberling, J. M., Isaac, B., and Kuebbing, S. E. (2020). Nonnative old-field species inhabit early season phenological niches and exhibit unique sensitivity to climate. Ecosphere 11:e03217. doi: 10.1002/ecs2.3217
Rees, R. (2008). Geography and landscape painting: an introduction to a neglected field. Scott. Geogr. Mag. 89, 147–157. doi: 10.1080/00369227308736255
Robinson, P. J. (2005). Ice and snow in paintings of little ice age winters. Weather 60, 37–41. doi: 10.1256/wea.164.03
Sahrhage, D., and Lundbeck, J. (1992). A history of fishing : Springer Science & Business Media. Available at: https://link.springer.com/book/10.1007/978-3-642-77411-9
Santibáñez, P. A., Maselli, O. J., Greenwood, M. C., Grieman, M. M., Saltzman, E. S., McConnell, J. R., et al. (2018). Prokaryotes in the WAIS divide ice core reflect source and transport changes between last glacial maximum and the early Holocene. Glob. Chang. Biol. 24, 2182–2197. doi: 10.1111/gcb.14042
Scarborough, V. L., and Gallopin, G. G. (1991). A water storage adaptation in the Maya lowlands. Science 251, 658–662. doi: 10.1126/science.251.4994.658
Schimel, D., and Schneider, F. D. (2019). Flux towers in the sky: global ecology from space. New Phytol. 224, 570–584. doi: 10.1111/nph.15934
Sillasoo, Ü. (2014). Landscapes, vegetation, and folklore in late medieval art: an iconographic study based on selected Austrian and south German panel paintings. Landsc. Res. 39, 455–479. doi: 10.1080/01426397.2012.761188
Stejneger, L. (1905). Do birds migrate along their ancient immigration routes? Condor 7, 36–38. doi: 10.2307/1361687
Stone, W. (1913). Bird migration records of William Bartram, 1802-1822. Auk 30, 325–358. doi: 10.2307/4071664
Teillet, J. V. (1988). A reconstruction of summer sea ice conditions in the Labrador Sea using Hudson’s bay company ships’ log-books, 1751 to 1870, (M.A. thesis), 1–161. Available at: https://mspace.lib.umanitoba.ca/items/990f5539-62e9-432f-a717-8c5030a9db3a
Turner, M. G., Calder, W. J., Cumming, G. S., Hughes, T. P., Jentsch, A., LaDeau, S. L., et al. (2020). Climate change, ecosystems and abrupt change: science priorities. Philos. Trans. Roy. Soc. B Biol. Sci. 375:20190105. doi: 10.1098/rstb.2019.0105
Ungar, P. S., Van Valkenburgh, B., Peterson, A. S., Sokolov, A. A., Sokolova, N. A., Ehrich, D., et al. (2021). Dental evidence for variation in diet over time and space in the Arctic fox, Vulpes lagopus. Polar Biol. 44, 509–523. doi: 10.1007/s00300-021-02821-8
Winiger, P., Barrett, T. E., Sheesley, R. J., Huang, L., Sharma, S., Barrie, L. A., et al. (2019). Gustafsson, source apportionment of circum-arctic atmospheric black carbon from isotopes and modeling. Sci. Adv. 5:eaau8052. doi: 10.1126/sciadv.aau8052
Winski, D., Osterberg, E., Ferris, D., Kreutz, K., Wake, C., Campbell, S., et al. (2017). Industrial-age doubling of snow accumulation in the Alaska range linked to tropical ocean warming. Sci. Rep. 7:17869. doi: 10.1038/s41598-017-18022-5
Woodruff, S. D., Diaz, H. F., Worley, S. J., Reynolds, R. W., and Lubker, S. J. (2005). Early ship observational data and ICOADS. Clim. Chang. 73, 169–194. doi: 10.1007/s10584-005-3456-3
Worby, A. P., Geiger, C. A., Paget, M. J., Van Woert, M. L., Ackley, S. F., and DeLiberty, T. L. (2008). Thickness distribution of Antarctic Sea ice. J. Geophys. Res. Oceans 113. doi: 10.1029/2007JC004254
Worth, R. (1990). The "hungry gap," crop failure, and famine: the fourteenth-century agricultural crisis and Piers plowman. Yearbook Langland Stud. 4, 87–104. doi: 10.1484/J.YLS.2.302914
Zooniverse. Available at: https://www.zooniverse.org/projects/p-teleti/monsoon-voyages (Accessed October 10, 2024).
Keywords: Arctic, climate change, proxies, observation, measurements
Citation: Miner KR, Wong E, Gay BA and Miller CE (2025) Non-traditional data to inform modern climate science. Front. Commun. 10:1518768. doi: 10.3389/fcomm.2025.1518768
Received: 28 October 2024; Accepted: 16 January 2025;
Published: 05 February 2025.
Edited by:
Ataharul Chowdhury, University of Guelph, CanadaReviewed by:
Nasir Khan, University of Guelph, CanadaCopyright © 2025 Miner, Wong, Gay and Miller. This is an open-access article distributed under the terms of the Creative Commons Attribution License (CC BY). The use, distribution or reproduction in other forums is permitted, provided the original author(s) and the copyright owner(s) are credited and that the original publication in this journal is cited, in accordance with accepted academic practice. No use, distribution or reproduction is permitted which does not comply with these terms.
*Correspondence: Kimberley R. Miner, a2ltYmVybGV5Lm1pbmVyQG1haW5lLmVkdQ==
Disclaimer: All claims expressed in this article are solely those of the authors and do not necessarily represent those of their affiliated organizations, or those of the publisher, the editors and the reviewers. Any product that may be evaluated in this article or claim that may be made by its manufacturer is not guaranteed or endorsed by the publisher.
Research integrity at Frontiers
Learn more about the work of our research integrity team to safeguard the quality of each article we publish.