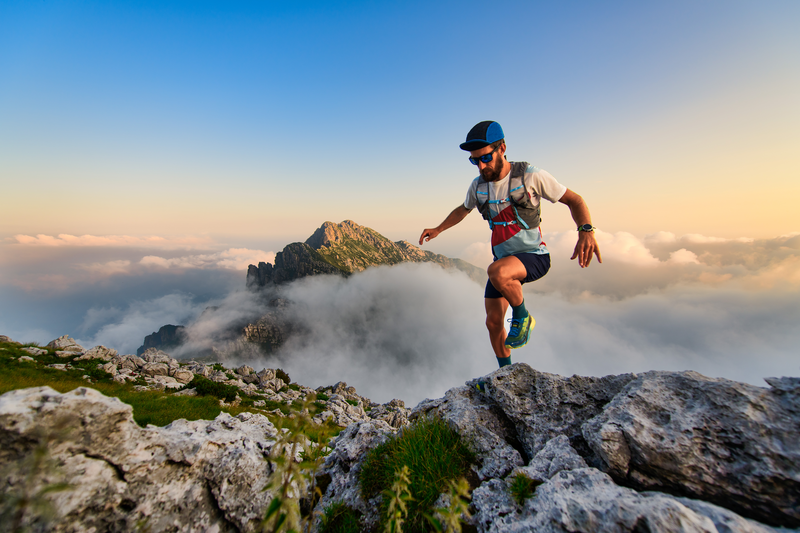
95% of researchers rate our articles as excellent or good
Learn more about the work of our research integrity team to safeguard the quality of each article we publish.
Find out more
REVIEW article
Front. Cognit. , 06 November 2023
Sec. Attention
Volume 2 - 2023 | https://doi.org/10.3389/fcogn.2023.1205618
This article is part of the Research Topic Insights in Attention: 2022 View all 15 articles
Attention is the ability to focus one's awareness on relevant events and objects while ignoring distracting ones. Laboratory studies of top-down voluntary attention commonly use predictive or instructional cues to direct attention. However, in real world scenarios, voluntary attention is not necessarily externally cued, but may be focused by internal, self-generated processes. The voluntary focusing of attention in the absence of external guidance has been referred to as “willed attention,” a term borrowed from the literature on willed motor actions. In a fashion similar to studies of willed (self-initiated) actions, during willed attention, participants are given the freedom to deploy attention based on their own free choices. Electrophysiological studies have shown that during willed attention, ongoing neural activity biases willed attention decisions on a moment-to-moment basis as reflected in transient patterns of brain electrical activity that predict where participants will later choose to focus their attention. Brain imaging studies have revealed that compared to cued attention, willed attention involves additional frontal cortical structures, which interact with the classic attentional control networks of the human brain to produce a modified network organization for willed attention control. In this introduction to willed attention, we briefly review the fields of voluntary attention and self-initiated motor actions, in order to describe willed attention and its neural correlates as they relate to the broader concepts of attention and volition.
“You can take a road that takes you to the stars now, I can take a road that will see me through.”
Nick Drake, 1972
Lyrics from Road
Pink Moon (Island Records, U.K.)
To navigate the world, one must select relevant information from the senses based on behavioral goals, weigh options given prior experience, make decisions, and take actions. Selective attention has an essential role in this cascade of mental events by supporting the selection of salient and/or behaviorally-relevant inputs and outputs. Voluntary attention allows one to focus their mental effort on some sensory stimuli, while suppressing irrelevant or distracting information. In vision, this influence on sensory processing is manifested as improved detection, discrimination and perception of attended visual target stimuli (Posner et al., 1980; Luck et al., 1994; Liu et al., 2009; Carrasco, 2018; Jigo and Carrasco, 2018; Carrasco and Barbot, 2019)—for a review, see Carrasco (2011)—and has been shown to result from neural changes early in visual cortical processing (Van Voorhis and Hillyard, 1977; Moran and Desimone, 1985; Corbetta et al., 1990; Mangun and Hillyard, 1991; Motter, 1993; Heinze et al., 1994; Kastner and Ungerleider, 2001; Briggs et al., 2013; Ghosh and Maunsell, 2021); for a review, see Battistoni et al. (2017).
Voluntary attention is a conscious process that is generally considered to be self-generated or “volitional.” In laboratory studies of voluntary attention, it is common, however, to provide incentives or instructions that lead observers to voluntarily focus their attention. For example, in studies of spatial attention, it is common to use an arrow cue, or other symbolic cue, to indicate a to-be-attended location in space where behaviorally relevant stimuli might be presented, requiring a response (Posner, 1980, 2016). Such a cue carries meaning in the context of the task, and therefore the observer makes a voluntary decision in using the cue information to perform the task. But it has been appreciated since at least the 19th century writings of Helmholtz (1867) and James (1890) that attention can be allocated voluntarily without being externally cued, such as in real world settings where internal goals may be pursued volitionally without explicit external instruction.
In recent years, new experimental paradigms have been developed to investigate how voluntary attention can be self-generated by observers in the absence of external cues provided by investigators. These new paradigms permit free choices that hand over the decision about where to attend to the observers rather than via investigator provided attention-directing cues (Taylor et al., 2008; Hopfinger et al., 2010; Bengson et al., 2014). We termed this self-generated form of voluntary attention, willed attention, a terminology proposed in our 2015 paper (Bengson et al., 2015), and which is derived in part from prior work on intention and action that used the term “willed action” to describe self-generated motor acts (Lau et al., 2004b).
The goal of this review is to describe the current state of research on willed attention and to place it in context with the extant literature on voluntary attention and willed action. First, we will set the stage by briefly introducing current models of attention. Then, we will focus on voluntary attention, describing the experimental methods that have been developed to investigate voluntary attention. The body of the review will explain the innovations that have led to the investigation of willed attention, and how cued (instructed) attention and willed attention are both related and different from each other. We will also contrast willed attention to the literature on willed actions, a field which has dominated studies of intention, volition and free will (Passingham et al., 2010; Haggard, 2019; Seghezzi and Haggard, 2022; Uithol et al., 2023). Finally, we will offer some new directions for studies to further understand the mechanisms of willed attention.
Attentional control processes are commonly categorized as either voluntary, implying top-down (internally-generated or endogenous) cognitive control, or involuntary or reflexive, indicating they are driven by bottom-up (external or exogenous) sensory inputs (Jonides, 1981; Muller and Rabbitt, 1989; Posner and Petersen, 1990; Corbetta and Shulman, 2002; Petersen and Posner, 2012; Katsuki and Constantinidis, 2014). Voluntary attention is said to be goal driven (Behrmann and Haimson, 1999; Fecteau et al., 2004), while reflexive attention is thought of as automatic (Jonides, 1981; Yantis and Jonides, 1984; Koch and Ullman, 1985; Muller and Rabbitt, 1989; Hopfinger and Mangun, 1998; Lupianez et al., 2004; Hopfinger and Maxwell, 2005; Hopfinger and Ries, 2005; Wyble et al., 2020). These processes, the top-down and the bottom-up, interact in everyday vision to provide humans with sophisticated abilities to pursue behavioral goals while also retaining the ability to orient and respond to novel events (Gaspelin and Luck, 2018; Theeuwes, 2018a).
Behavioral research has shown that voluntary and reflexive attention differ in important ways. Reflexive attention results in faster shifts of attention, which are shorter lived, and often followed by a period of inhibition, known as inhibition of return, whereas voluntary attention takes slightly longer to engage, and is more resistant to decay, interference and inhibition (Jonides, 1981; Tassinari et al., 1987; Muller and Rabbitt, 1989; Lupianez et al., 2001). Electrophysiological studies have shown that the effects of both voluntary and reflexive visual attention result in spatial-selective modulations of visual inputs that occur early in visual cortical processing (Mangun and Hillyard, 1991; Eimer, 1994; Hopfinger and Mangun, 1998, 2001; Hopfinger and Maxwell, 2005; Hopfinger and Ries, 2005; Hopfinger and West, 2006), but as the result of different neural control mechanisms.
Neuroimaging research has shown that voluntary and reflexive attention rely on distinguishable brain control systems (Mesulam, 1981; Corbetta and Shulman, 2002; Chica et al., 2013; Vossel et al., 2014). Corbetta and Shulman (2002) articulated the concept of two distinct attentional systems for voluntary and reflexive attention, the dorsal and ventral attention networks, respectively. The dorsal attention network (DAN) controls top-down spatial, feature, and object attention (Corbetta et al., 2000, 2005; Hopfinger et al., 2000; Giesbrecht et al., 2003; Liu and Hou, 2013), while the ventral attention network (VAN) is involved in bottom-up attention, detects salient events, and supports shifting attention from currently attended stimuli to potentially relevant rare events (Fox et al., 2006; Indovina and Macaluso, 2007; Corbetta et al., 2008; Geng and Mangun, 2011; Allan et al., 2020). How precisely the interplay between these two systems occurs from moment-to-moment remains to be fully understood, but most models argue for a close association in support of everyday behavior (Vossel et al., 2014).
The overarching framework of most voluntary attention models is the idea that voluntary attention is controlled by the DAN (Hopfinger et al., 2000; Wager et al., 2004; Woldorff et al., 2004; Slagter et al., 2006; Armstrong and Moore, 2007; Corbetta et al., 2008; Sylvester et al., 2009; Weissman et al., 2009; Asplund et al., 2010; Szczepanski et al., 2010, 2013; Gazzaley and Nobre, 2011; Tamber-Rosenau et al., 2011; Gregoriou et al., 2014; Battistoni et al., 2017; Zink et al., 2021); for reviews see Corbetta and Shulman (2002) and Miller and Buschman (2013). The DAN issues control signals (Corbetta and Shulman, 2002; Rajan et al., 2021), transmitted via top-down neural pathways, that bias activity in visual cortex (Luck et al., 1997; Chawla et al., 1999; Kastner et al., 1999; Hopfinger et al., 2000; McMains et al., 2007). This biasing leads to modulations of sensory inputs based on their task relevance, which ultimately results in selective perception (Moran and Desimone, 1985; Mangun and Hillyard, 1991; Treue and Maunsell, 1996; Buschman and Kastner, 2015; Battistoni et al., 2017).
The strong dichotomy of top-down control vs. bottom-up as reflecting volitional and non-volitional/automatic processes, respectively, is a useful heuristic, but we hasten to point out that current models are more complex, and consider situations where the context, past reward and other factors influence attentional selection independent of immediate goals, and sometimes in contradiction to them (Awh et al., 2012; King et al., 2012; Egner, 2014); for a review, see Baluch and Itti (2011). In recent years, the concept of selection history in attentional control and selection has been quite influential in this regard (Theeuwes, 2018a), and has generated much discussion in the literature (Egeth, 2018; Failing and Theeuwes, 2018; Gaspelin and Luck, 2018; Theeuwes, 2018b). We wish to simply highlight this point here and will return to it later.
Voluntary attention has been studied using various experimental paradigms, including visual search (Treisman and Gelade, 1980; Luck and Hillyard, 1995; Lamy et al., 2004), flanker interference methods (Eriksen and Eriksen, 1974; Lavie, 2011; Gaspelin et al., 2014), Stroop interference tasks (Treisman and Fearnley, 1969; Aine and Harter, 1984; Egner and Hirsch, 2005), and cuing paradigms (Posner, 1978; Klein, 1980; Donovan et al., 2020). In particular, trial-by-trial attention cuing has proven to be a powerful method for investigating voluntary attention (Posner, 2016), because it enables the experimental and theoretical dissection of attentional control from attention selection of task relevant target stimuli (Hopfinger et al., 2001).
In typical cuing studies, an observer is presented with an attention-directing cue, which instructs or biases the person to prepare to process an upcoming task-relevant stimulus. In theory, cognitive-neural events following the attention-directing cue but occurring prior to the appearance of task-relevant target stimuli (and/or distractors), can be related to the various mental processes necessary for the top-down voluntary control of attention. In contrast, following the appearance of the target stimuli, processes related to sensory-perceptual processing, and any potential modulation of them by top-down attention, are expected. Thus, because the cue and target are separated in time by hundreds or thousands of milliseconds, so are the temporally-associated cognitive, sensory, and motor processes. In spite of some important constraints about the measures employed, especially for functional imaging studies (e.g., Das et al., 2023), this temporal segregation allows one to measure the cognitive-neural events for top-down attentional control separately from subsequent stimulus selection (and task-related motor processes).
In experimental psychology studies, for the most part, the activity of the cue-induced top-down attentional control activity must be inferred from the performance differences observed in the behavioral measures obtained in the task. These behavioral measures are the responses (speed, accuracy, etc.) to the target stimuli as a function of attention (Posner et al., 1980). Cognitive neuroscience methods, however, provide an additional opportunity to measure attentional control separately from stimulus selection because the brain activity to the attention-directing cues can be measured even though there are typically no behavioral responses to the cues themselves.
Using event-related potentials (ERPs) to capitalize on the temporal separation of cues and targets in an attentional cuing study, Harter et al. (1989) derived measures of brain electrical activity following attention-directing cues separately from the subsequent target stimuli. They found characteristic ERP waveforms over the scalp following the cue and preceding the target. The cue-related responses included the sensory-evoked activity evoked by the physical features of the cues themselves, of course, but also included non-sensory cognitive responses that were related to the attention instructions. They interpreted the non-sensory cognitive ERPs as being related to the top-down (voluntary) control of attention, which included both the top-down signals themselves, and the differential biasing of cortical excitability in sensory-specific cortex. This work was replicated and extended in many studies (Hopf and Mangun, 2000; Eimer et al., 2004; Kelly et al., 2009; Seiss et al., 2009; Green and McDonald, 2010; Hong et al., 2015). Following this logic, fMRI studies have been able to isolate cue-related from target-evoked activity. In a pair of papers published side-by-side in the journal Nature Neuroscience, Corbetta et al. (2000) and Hopfinger et al. (2000) investigated the neural correlates of top-down voluntary visual spatial attention, showing distinct brain activity for voluntary control and stimulus selection and motor action (see also, Kastner et al., 1999). These ERP and fMRI studies, and the many to follow using this logic, punctuate the theoretical distinction between voluntary control and stimulus selection and how they can be separately measured and studied. Studies using cuing methods to investigate voluntary attentional control have flourished over the past 25 years, and the paradigm has become a gold standard methodology (Corbetta and Shulman, 2011; Fiebelkorn and Kastner, 2020; Posner and Rothbart, 2023).
The use of attention-directing cues to study voluntary attention is a widely accepted method that is rarely questioned. Indeed, it is clear that within the context of these types of laboratory studies, subjects do exert voluntary attention in response to the cue, because such cues alone do not necessarily direct attention reflexively, but instead require the participant to focus attention as specified in the instructions provided by the investigator with respect to the cues and task goals (see, however, Ristic et al., 2007). Nonetheless, some have asked what it means to be voluntary if the act to be undertaken is somehow signaled (cued) from outside the individual observer/actor. We take the position that there is a spectrum of volition, such that depending on the circumstances, an individual may rely fully on self-generated decisions and action, or may biased by external circumstances, such as cues. So, being instructed to pay attention to a stimulus by an external cue certainly requires volition, but if it does not involve a free choice by the observer, should it be considered fully voluntary? This question prompted the first studies of what we now refer to as willed attention.
Taylor et al. (2008) investigated willed spatial attention by using fMRI to reveal the underlying brain activity when observers were either cued or were permitted to choose where to attend. The study was otherwise a standard visual-spatial cued attention task, but on some trials the subjects were simply prompted to make a free choice between attending a left visual field location or a right one in order to discriminate a target stimulus at the attended (chosen) location. Hopfinger et al. (2010) used a similar experimental design, including a condition which eliminated attention directing cues, allowing free attention choices by the observers. Both studies found key differences between the brain regions activated for free choice and cued attention. While there was significant overlap in the brain activity engaged for cued vs. willed attention, crucial differences, largely in frontal cortex, made clear that during willed attention there were additional brain regions engaged. These seminal studies are highly relevant to our understanding of purely voluntary (willed) attention, which is arguably critical in natural settings where internally-generated cognitive and motor acts are fundamental to goal-driven behavior (Pezzulo et al., 2018; Turner et al., 2019); for a review, see Tsotsos et al. (2021).
The foregoing fMRI studies focused on the brain activity patterns related to attention decisions and attentional control by investigating activity related to the attention-directing cues or prompts to choose where to attend; that is, time locked to the cues/prompts. However, because fMRI relies on measures of brain hemodynamics, which are sluggish and indirect measures of neural activity (Burock et al., 1998; Glover, 2011; Marxen et al., 2023), it is difficult to know the precise time course of the observed activity, and therefore, when it occurs with respect to decisions about how to focus attention. In order to measure the rapidly unfolding neural activity associated with willed attention, we entered the fray by using EEG, ERPs, and behavioral measures (Bengson et al., 2014).
In the EEG study of Bengson et al. (2014), we used a paradigm similar to those by Taylor et al. (2008) and Hopfinger et al. (2010), where we contrasted the brain signals recorded between conditions in which cues instructed subjects where to selectively attend on a trial-by-trial basis (left or right), or in which a prompt signaled that the participant was to spontaneously choose where (left or right) to attend on that trial (i.e., choose trials). That is, on the choose trials, although the time point of the focusing of attention was specified by the appearance of the prompt, whether to focus attention selectively on the left or right visual hemifield location was determined by the participants' free choices. The paradigm is illustrated in Figure 1: there were three stimuli used as instructive cues or prompts: one stimulus (cue) instructed the participant to attend left, another instructed then to attend right, and a third (the prompt) signaled the participant to choose whether to attend left or right on that trial. Following the cue or prompt, a sine wave grating stimulus would appear in the left or right hemifield location, and subjects would have to discriminate the spatial frequency of the grating. The task was a 100% attentional allocation task, meaning that participants would attend to and respond only to the target if it was in the cued (or chosen) hemifield; otherwise, they ignored the grating stimulus.
Figure 1. Example trial from the willed attention study of Bengson et al. (2014). Three different cues/prompts were used, and these are shown at the top of the figure. In this example, the trial is a willed attention trial, where the subject had to choose whether to attend right or attend left upon receiving the somewhat unexpected prompt (circle). Shown is a target presented to the right visual hemifield, but targets could be in either visual hemifield with 50/50 probability. If the subject would have chosen to covertly attend right on this trial, then they would have been required to discriminate the spatial frequency of the target grating (thick vs. thin bars) and to report that with the appropriate button press. At the end of the trial, the Report query appeared (?SIDE?), and if they had chosen to attend right, then they would push a button indicating their choice as “attended right.” If on this trial they had chosen to attend left, then they would have ignored the right visual hemifield target entirely and would only press a button to report “attended left” to the query (adapted from Bengson et al., 2014 with permission from MIT Press).
Before turning to the EEG results, we must take a quick detour to describe a behavioral control study critical for this work. Because the task required subjects to only attend and respond to the cued or chosen-location targets, no standard behavioral measures of selective attention were available in this design; that is, there were no reaction time measures for the uncued/unchosen-location targets (e.g., Posner et al., 1980). This was deliberate because we wanted to avoid inducing the subjects from dividing their attention in the task. Thus, in order to validate the paradigm and determine whether the behavioral attention effects for cued and willed attention were similar or different, we ran a behavioral-only version of this study where participants were told to respond to targets in both the cued/chosen location and in the uncued/unchosen location. To avoid this being simply a divided attention task, they were told to focus covert attention fully on the cued/chosen location on each trial, but to quickly switch to and respond to the uncued/unchosen targets when they appeared. Note, that there was no probability manipulation of cue-target expectancy—a common method in such designs (Posner, 1978; Klein, 1994; Chen et al., 2023)—because such a manipulation was not logically possible in the choose condition. We observed highly significant attention effects in this task, where overall, the cued/instructed and chosen location targets were responded to significantly faster than were targets at the uncued/unchosen location (p < 0.01; Figure 2). This reaction time spatial attention effect was not statistically different for cued and willed attention. This is similar to the result of Taylor et al. (2008), who compared cued/chosen-location target performance with performance in a separate divided attention condition, finding no differences in the benefits of focal attention between cued and willed attention conditions. These effects are in line with the large literature on attention's effects on reaction times (for a review, see Posner, 2016), and demonstrate that at the level of behavioral performance, cued and willed attention both result in selective processing of target stimuli, which imparts an advantage to attended-location events.
Figure 2. Reaction time attention effects for cued vs. willed spatial attention. Reaction time data plotted as bar graphs from the behavioral validation experiment of Bengson et al. (2014). The bar graphs show RTs for cued trials (blue) and willed trials (red). The effect of spatial attention (attended vs. unattended) was significant overall at p < 0.01 (as represented by ‘**’ on the figure). There was no significant interaction between attention type (cued vs. willed) and the attention effect (attended vs. unattended), which demonstrated that at the level of behavioral performance, willed attention and cued attention produce the same performance outcomes for the target stimuli.
Now, we return to describe the novel EEG findings in Bengson et al. (2014) study. Using electrophysiology, we had a unique opportunity to investigate not only the post-cue/prompt brain activity during cued vs. willed attention, but also the brain activity patterns prior to the appearance of the prompts. This permitted us to investigate the antecedent brain states associated with different free choices made by the participants in response to the prompts, and to reveal the time course of any activity related specifically to willed attention; this analysis was inspired by the literature on motor intention and self-initiated movement that we will review in a later section.
Our analyses focused on EEG oscillatory activity, especially in the alpha band (8–13 Hz), because these signals had previously been shown to be related to focused spatial attention (e.g., Worden et al., 2000). Quantifying EEG alpha backwards in time from the onset of the prompts, we looked for differences in alpha power as a function of whether the subjects later chose to attend left or attend right. We found that willed attention was associated with a unique predictive pattern of alpha-band power immediately preceding the unpredictable onset of a choose prompt. This alpha-band activity was localized primarily over left parietal-occipital scalp, and predicted the upcoming decision to attend before the subject could be aware that they had to make a decision (see more on this point in the next paragraph). The predictive EEG alpha pattern was restricted to approximately 1 second prior to the unexpected appearance of the prompts (Figure 3A, left). These results indicated that ongoing neural variability in the alpha-band was correlated with attentional decision making on a trial-by-trial basis. The interpretation we offered in the Bengson et al. (2014) paper was that there were stochastic fluctuations in the patterns of brain activity that were correlated with, and therefore predicted, whether a person would choose to attend left or attend right; we wrote, “…the appearance of free will, as manifested through seemingly arbitrary cognitive decisions, may be a consequence of the role that inherent variability in brain activity plays in biasing momentary behavior.” This pre-prompt neural activity is reminiscent of that observed in studies of free choice decision making in motor actions (Libet et al., 1983b; Haggard and Eimer, 1999; Lau et al., 2006; Soon et al., 2008), but differs in that it is preceding decisions regarding covert cognitive functions; i.e., the decision about how to selectively focus covert visual spatial attention. The relationship of our willed attention work to motor intention studies will be discussed in a later section.
Figure 3. EEG and fMRI measures of willed attention. (A) Pre-prompt and post-prompt alpha power topographic maps for choose left vs. choose right trials (adapted from Bengson et al., 2014 with permission from MIT Press). Time = 0 msec is the onset of the choose prompt, indicating that the participants should choose whether to attend left or attend right for that trial. Predictive patterns of EEG alpha power were only observed during the 800 msec prior to the onset of the prompt, while earlier in time there was no significant EEG signals predicting the subsequent choice. After the prompt, in the prompt-to-target interval, the decision to attend left vs. right led to the well-known post attentional orienting occipital alpha lateralization (+1,000–1,800 msec). (B) Contrast of willed to cued (instructed attention) (adapted from Bengson et al., 2015, dataset; see text).
Important design characteristics need to be acknowledged in the Bengson et al. (2014) study in order to make clear why we asserted that the pre-prompt predictive EEG alpha activity was not a reflection of a predetermined decision by the subject, but rather a stochastic brain state. That is, we argue that the subjects made a spontaneous decision about where to attend at the time they perceived the choose prompt. First, there was only a 33% chance that the prompt would appear as opposed to an instructive cue, and thus the subjects would not have strong motivation to adopt a strategy of preparing for a choose trial and picking a side to attend in advance. Put another way, two-thirds of the time they were going to receive an instructive cue, which would render any predetermined decision about where to attend moot; we verified this by post-experimental debriefing of the subjects who reported that they did not decide in advance where to attend because most of the time they received an explicit instructional cue. Second, and more critically, the experimental design also made it impossible for the participants to estimate the time of arrival of the cue or prompt with any precision because of the highly variable inter-trial interval, which varied randomly over the range from 2 to 8 seconds. Essentially, the subjects would have had to have been clairvoyant in order to make an advance decision that was restricted to the 1,000 msec just prior to the unexpected prompts.
In addition to the pre-prompt predictive EEG activity, we also observed post-prompt ERP and EEG activity that differed from that during cued attention (reported in Bengson et al., 2015). Immediately following the prompts (compared to the instructive cues) we observed two unique ERPs associated with willed attention. The first had a frontal scalp distribution in the time period of 250 to 350 msec post-prompt; we called this the Early Willed Attention Component (EWAC). A second ERP sign of willed attention occurred between 400 and 800 msec post-prompt over central scalp sites, which we called simply the Willed Attention Component (WAC). Thus, willed attention was associated with unique signs of brain activity both before choose prompts (the predictive EEG alpha) and after (the EWAC and WAC), as subjects made decisions about where to attend. Note that the pre-prompt activity is the predictive EEG alpha with respect to the subsequent decision, not a contrast of cued and willed attention, while the post cue/prompt ERPs are revealed by the difference between willed and cued attention trials.
In addition to these unique electrophysiological signs of willed attention, we also observed EEG and ERP effects that were the same for cued and willed attention. Several 100 msec after the cues and prompts, the well-known occipital alpha asymmetry with spatial attention was observed (Figure 3A, right); i.e., there was a reduction of alpha power over the occipital scalp contralateral to the direction of spatial attention. Finally, the subsequent target-evoked visual ERPs showed standard spatial attention modulations in the amplitudes of early sensory ERP components (Bengson et al., 2014). We will not discuss these EEG/ERP findings further here, and instead refer the reader to the published studies (Bengson et al., 2014, 2015); however, the bottom line is that cued and willed attention result in significantly different attention-related neural activity in and around the time of cue/prompt appearance, while later in time, the brain activity for cued and willed attention are virtually identical, suggesting that the final neural outcome of cued and willed attention on brain activity and behavior (as described above) are the same.
In the foregoing, our aim was to establish the experimental and conceptual framework for the concept of willed attention, and to describe the electrophysiological evidence for the similarities and differences between cued and willed attention. In this section we will review what is known about the underlying neural mechanisms of willed attention and how they may differ from cued (instructed) attention. Several reports have helped to elucidate the underlying neural correlates of willed vs. cued attention. The literature on willed attention remains rather small, and much of the work comes from our group, which we will make clear as we survey the available evidence. In the following, we will focus primarily on the activity following the prompts and cues that elicited attentional orienting, rather than the antecedent brain states described in the study by Bengson et al. (2014). The primary reason for this is because, unlike the EEG/ERP measures, fMRI data has poor temporal resolution, making it challenging to know whether cue/prompt-related activity occurs immediately prior to or after the cues or prompts. Although we believe there are methods that may be applied to imaging data to help mitigate this limitation, and other methods such as magnetoencephalography (MEG) could be helpful, the studies to date have not applied such tools to investigate willed attention.
In an fMRI version of the study of Bengson et al. (2014), using the same experimental paradigm in the same volunteers, we investigated the brain networks supporting willed attention (Bengson et al., 2015). This multimodal method permitted us to relate the EEG/ERP data described above to the underlying brain functional anatomy (e.g., Mangun et al., 2000). By contrasting instructed and willed attention, this work showed that during willed attention, additional neural activity could be detected in the frontal cortex in the dorsal anterior cingulate cortex (dACC), middle frontal gyrus (MFG), and the anterior insula (AI) (c.f., Taylor et al., 2008). These were in addition to activity in the DAN, which were common to willed and instructed attention (Taylor et al., 2008; Hopfinger et al., 2010). In related work from the lab of the late Steve Yantis (Gmeindl et al., 2016), frontal activations, especially in dACC and MFG were again shown for willed attention in a different task (rapid serial visual search paradigm), and using multivoxel pattern analysis (MVPA) decoding methods. All these studies point to key frontal brain regions that are activated when subjects orient attention in a self-generated manner, and these include the dACC, MFG and anterior insula.
We subsequently replicated the fMRI study of Bengson et al. (2015) with our colleagues at the University of Florida. Using both datasets, we investigated new questions about the mechanisms of willed attention using graph-theoretic analysis (Liu et al., 2017). In this work we investigated possible functional network differences between instructed and willed attention. Three well known cortical networks were identified as supporting willed attention: the cingulo-opercular network, the dorsal attention network (DAN) and the frontoparietal network (He et al., 2007; Dosenbach et al., 2008). By comparing the network architecture for willed and instructed attention, we discovered that the dorsal anterior cingulate cortex (dACC) changed its network allegiance from being clustered with the dorsal attention network during instructed attention to becoming associated with the cingulo-opercular network during willed attention. This shift in dACC alignment for instructed and willed attention demonstrates its central role in both forms of attention, but via different functional network associations. That is, during willed attention, the cingulo-opercular network acts to mediate communications between the frontoparietal network and the DAN. This rather remarkable finding of a change in the dACC network allegiance for willed attention suggests a key neurobiological difference between instructed and willed attention. What does this network organization suggest about willed attention?
The dACC has long been implicated in attention (Mesulam, 1981; Bench et al., 1993; Nobre et al., 1997; Posner and Rothbart, 1998; Weissman et al., 2005; Walsh et al., 2011), cognitive control (Botvinick et al., 2001; van Veen et al., 2001; Kerns et al., 2004; Schulz et al., 2011), action initiation (Srinivasan et al., 2013), motivational value (Yee et al., 2021), and volition (Winterer et al., 2002; Nitschke and Mackiewicz, 2005). While many have bemoaned the fact that the dACC activates in many tasks, its role in attention, cognitive control, action initiation, motivational value, and volition form a common thread that suggests the dACC is relevant when an organism must make task-relevant decisions and actions. Willed attention fits well within this framework, as the subjects in willed attention tasks are asked to make free choice decisions and then act on them by selectively focusing their attention. The graph-theoretic network analysis demonstrates how the dACC may play a role in self-generated acts like our willed attention task by serving as a mediating influence on executive functions of the frontoparietal network and the top-down control ultimately exerted on sensory processes by the DAN (Meehan et al., 2017; Ray et al., 2020).
Further evidence for this network-level organization comes from analysis of the EEG data gathered in our willed attention design, which again included two datasets (UC Davis and University of Florida). Analyzing the spectral context of the EEG signals following the instructive cues vs. the choose prompts revealed significant difference in frontal theta signals (3–7 Hz) such that there was increased theta power during willed attention, arising 500 msec after the free choice about where to attend (Rajan et al., 2018); see also Bengson et al. (2020) for a replication of the increase frontal theta with willed attention. Using Granger causality analyses (Ding et al., 2006; Dhamala et al., 2008a,b)—a method to quantify the strength and directionality of brain signals—we showed that the increase in frontal theta power was paired with increased frontal-to-parietal scalp theta-band coherence, and bidirectional Granger causality. We interpret this enhanced bidirectional influence reflected in theta EEG to mean that during willed attention, frontal cortical structures and associated networks transmit information regarding the decision about where to attend to key nodes in the attentional control system (DAN), but also receive information back, perhaps to update representations of the current state of focused attention (Heekeren et al., 2008).
In addition to the increased frontal theta following free choice decisions during willed attention, as noted earlier (see Figure 3, right), we have also observed the well-known occipital EEG alpha (8–13 Hz) asymmetry with lateralized spatial attention (Worden et al., 2000). In standard cuing studies, this alpha asymmetry takes the form of reduced alpha power over the occipital scalp contralateral to attended visual hemifield, and a relative increase in the hemisphere ipsilateral to the attended field (Thut et al., 2006; Rihs et al., 2007; Romei et al., 2010; Popov et al., 2019), and is hypothesized to reflect inhibition of upcoming unattended inputs in the Gating by Inhibition Model of alpha (Jensen and Mazaheri, 2010). We demonstrated that the occipital attention-related alpha asymmetry was also present following willed attention decisions (Bengson et al., 2014), indicating similarities in the downstream effects of orienting and focusing spatial attention, which might be expected theoretically (see also, Trachel et al., 2015). That is, once a voluntary decision to focus spatial attention has been taken and initiated, the chain of cognitive-brain processes for focused spatial attention are largely the same following instructive cues and prompts to make free choices; as described earlier, this involves activity in the DAN (reflecting the top-down issuing of control signals for selective attention), biasing of sensory cortex by this control (reflected in baseline shifts of neuronal activity, and the occipital EEG alpha asymmetry), and subsequent selective sensory processing of attended inputs (seen as increased firing rates and ERP amplitudes for attended stimuli, we well as facilitated behavioral performance). However, as might be expected, the post-prompt occipital alpha asymmetry is delayed for willed attention compared to cued attention hundreds of milliseconds, and it appears after the frontal theta power increase that occurs post prompt (Rajan et al., 2018; Bengson et al., 2020). So, during willed attention, volitional free choice decisions about where to attend (willed attention)—arising (presumably) from frontal cortex and correlated with increased frontal theta and increased theta coherence and connectivity—result in top-down control signals from the DAN that bias the visual cortex, as reflected by the occipital alpha asymmetry, leading to selective visual processing. This cascade takes longer than for cued (instructed) attention, where the free choice decision component is largely eliminated, thus allowing for more expeditious implementation of attention-related biasing of visual cortex. Or to put it more simply, the free choice decision takes more time than acting in response to an attention-directing cue.
Finally, one may ask whether the prompts to make free choice decisions themselves might also introduce artificial or confounding circumstances, further concealing or distorting the operations of purely voluntary (self-generated) attention. Indeed, as an aside, this may be a more general concern when using cues or prompts. For example, some cuing studies on voluntary attention have come to different results when different types of cues were used (Antonov et al., 2020; Gundlach et al., 2020). Moreover, even rather simple arrow cues have been shown to engage attention in a fashion more akin to reflexive attention, due to their overlearned nature in humans (e.g., Ristic et al., 2007). So, in a recent study of willed attention, we took a further step by completely eliminating any cues or prompts (Nadra et al., 2023). The idea was that if willed attention reflects purely volitional processes, then its properties and mechanisms should be similar even when allocating attention without any cues or prompt, as may sometimes occur in natural vision (c.f., Gmeindl et al., 2016). Thence, with the goal of developing a laboratory-based experimental paradigm that was a step in the direction of natural vision, we left the decision about both when and where to focus attention to the participants. The experimental display contained two dot motion arrays (one on each side of fixation) consisting of red dots in the center and blue dots in the surround (Figure 4). The red dots gradually increased and decreased over time, with both dot motion fields uncorrelated with each other. Participants were instructed to keep their eyes on a central fixation cross for the duration of each trial. On each trial, the dot motion arrays would appear, and the subjects' task was to wait a moment, and then at a time of their own choosing, select a side to attend and then immediately voluntarily shift and focus covert attention on the left or right stimulus patch. They were then to covertly monitor the attended patch (ignoring the opposite field patch) in order to detect when the proportion of red dots in that patch reached its maximum, pushing a button in response. As in all our studies of willed attention, they were told not to use any explicit strategy or develop any pattern for choosing when or which side to deploy covert attention (such as alternating sides on each trial), and to not decide prior to trial onset which hemifield patch to attend. In other words, once the bilateral array appeared, the subjects were requested to make a spontaneous decision about when and to which side to focus covert spatial attention. Responses were made with their right hands, pushing the left arrow on a keyboard with their index finger if they had chosen to attend left on that trial, or pushing the right arrow with their middle finger if they had chosen to attend right. Thus, the button responses not only signaled the time of their detection of the target, but also provided evidence as to where they attended on that trial. Using machine learning MVPA decoding of EEG alpha power, we could identify the attended hemifield, using the button response as a time stamp and indication of the side attended. We found that the occipital attention-related alpha power asymmetry observed in cued and willed attention was also present in this uncued study, and that it preceded the motor responses by about 1 second. By eliminating explicit cues or prompts that influence the allocation of voluntary attention, this study helps to advance our understanding of willed attention, and more generally of voluntary attentional control.
Figure 4. Uncued/unprompted willed attention paradigm. Participants were told to fixate the central cross, and then to spontaneously choose whether to covertly attend left or right at a time of their choosing (adapted from Nadra et al., 2023). See text for description.
Intention, volition and free will are concepts with long histories, sometimes involving contentious debate. So, before using these terms in interpreting willed attention in a larger framework, we will begin with some simple, well-accepted definitions in order to clarify how we will use the terms. Intention can be defined as the mental state in which a future action (cognitive and/or motor) is represented in the mind and brain. Here, we consider only immediate intentions, not distant intentions (e.g., such as planning to earn a Ph.D.), and with this in mind, turn to some helpful thoughts by Pacherie and Haggard (2011). They suggested that there are two characteristics of immediate intentions, and that they are: “…accessible to consciousness,” and “…they bear some relation to subsequent action.” Essentially, intentions are the mental representations of a behavioral goal that are activated to execute the goal about which we are aware. Volition can be operationalized as a process that: (i) is internally generated (as opposed to stimulus driven), (ii) involves decisional control (as opposed to being reflexive or habitual actions), (iii) is goal directed, and (iv) is accompanied by the conscious experience of intending to take the action (Haggard, 2019; Seghezzi and Haggard, 2022). Free will is often used synonymously with the term volition, and therefore the preceding characteristics of volition would apply to free will as well (Lavazza, 2016). Although the literature on free will is expansive, we will stop here, and thus, in the context of our review of willed attention, we will use the terms volition and free will synonymously. One might ask why use the term free will at all (Gazzaniga, 2012; Shadlen and Roskies, 2012), but given that in our tasks, subjects are asked to make free choices (and we have used the term willed attention), it would seem natural to refer to free will. However, in doing so, we are most decidedly not suggesting that volition or free will are brain-independent mental functions. Rather, we hope to make clear the opposite, that willed attention relies on brain mechanisms, mechanisms that can be studied and understood. Our goal, therefore, in the following is to relate willed attention to the larger psychology and neuroscience literature on willed actions, where considerations of intention, volition and free will are commonplace. We will focus on prominent studies that have identified neural correlates of free choices, beginning with the work of Benjamin Libet.
Libet approached the concepts of intention, volition and free will by recording brain electrical activity preceding voluntary motor actions. He found that motor readiness potentials (ERPs) preceded conscious intentions to make a self-generated movement by up to half of a second (Libet et al., 1983a; Libet, 1985); how did he accomplish this? Libet's highly influential studies employed a novel paradigm where subjects were facing a clock which moved once every 2.56 seconds. The experimenters collected both EEG data as a measures of brain activity, and EMG data to track their muscle activity as well as self-reports about the time of their intentions to move (from the clock face). Subjects were told to make a spontaneous movement while looking at the clock, flexing their wrist at a time of their own choosing. The subjects were also instructed to report the position of the clock when they first became aware of their decision to move, and this reported time was used as a measure of the onset of conscious intention. The onset of the willed action was signaled by the onset of electromyographic (EMG) activity in the wrist flexor muscles (as measured by electrodes on the forearm), and the onset of the intention to act was pinpointed by the onset of the ERP known as the readiness potential (RP) (Kornhuber and Deecke, 1964, 2016). Libet's key finding was that the RP preceded conscious awareness of the intention to move (indexed by the clock time reported by the subjects) by 500 to 1,000 msec, which suggested that the conscious decision to move one's hand had a neural antecedent that preceded the conscious intention to move. Libet's breakthrough work opened the floodgates on free will and agency as a research topic in neuroscience (David et al., 2008; Moore and Obhi, 2012; Wolpe and Rowe, 2014; Braun et al., 2021).
Libet's studies, were however, often challenged and criticized. For example, the nature of the RP itself, and therefore what it tells us about volition in Libet's paradigm has been questioned. There is evidence that the RP may not be the driving force behind the decision to act, and therefore whether it is involved in volitional decision making, or only related to the act of simply being presented with a decision, is at issue (Balaguer, 2014). Haggard and Eimer (1999), for example, investigated the RP and a derived ERP measure known as the lateralized readiness potential (LRP), which is computed by subtracting the RP recorded at electrodes contralateral and ipsilateral to the moving hand. They concluded that it is the LRP, which develops later in time, rather than RP, that is an index of conscious motor intention. Subsequently, in a joint article, Haggard and Libet (2001) proposed that the RP was an index of the general preparation to act, whereas the LRP reflected the onset of an intention to make a specific action in the brain. Thus, the original model of Libet was refined, but the key underlying patterns of brain activity in the Libet model were nonetheless replicated, supporting his original interpretation that brain activity preceded the conscious intention to act.
There also arose a notable hypothesis that perhaps free will does not exist in the way humans intuitively believe, but that it is more related to a veto mechanism that can override an action that was initiated unconsciously in the brain. Libet referred to this mechanism as “free won't,” postulating that the brain acts automatically in the generation of actions, but our will acts as an inhibitory process that guides our decisions based on what not to do, rather than by initiating a voluntary action (Libet, 1985). Our power to inhibit processes that may be underway can be implemented up until a point of no return, where actions are unable to be vetoed. Decisions to act, therefore, are hypothesized to have three separable components, what action to take, when to make the action, and whether to make the action—the concept of “free won't” affects whether a decision is made or not, and acts as Libet's primary argument against determinism: the idea that neural events, rather than free will, determine our actions (Lavazza, 2019). However, there has also been some evidence against this idea. EEG activity immediately (−150 msec) preceding decisions to act or not to act have similar patterns in the EEG, thus suggesting that similar antecedent brain states are involved in both cases (Filevich et al., 2013). This argues against the idea offered by Libet and others that free will may better be conceived of as the voluntary inhibition of motor intentions that arise by unconscious brain activity. Filevich et al. (2013) wrote: “the cause of our ‘free decisions’ may at least in part, be simply the background stochastic fluctuations of cortical excitability… free won't may be no more free than free will” (see also Shadlen and Roskies, 2012).
Measures of the unconscious determinants of free decisions in humans is not limited to EEG. In fMRI work, brain activity in the anterior medial prefrontal and medial posterior parietal cortex were shown to encode a decision to make a button press (left or right) up to 10 seconds before the subjects were aware of the intention to move (Soon et al., 2008). Their paradigm consisted of a stream of letters presented on a computer monitor (one every 500 msec), and the subject's task was to report which letter was on the screen at the time they made the decision to move. As in Libet's original studies, the time of the self-report of awareness to move was taken as the onset of motor intention, and significant brain activity built up in the time prior to this awareness of motor intention. Thus, there is converging neuroscience evidence for predictive brain activity during self-generated motor actions.
Additional evidence also comes from studies of free choices in both humans and animals (for a review, see Funahashi, 2017). Free choice decision making studies in humans commonly cite the prefrontal cortex as the primary driver in intentional actions, but not instructed actions (Lau et al., 2004a). Primate studies have consistently shown that prefrontal neurons can predict an upcoming decision to act when the animals are presented with a free choice decision (Marcos and Genovesio, 2016; Mione et al., 2019). This neural activity that is selectively appearing before free choice decisions is termed “choice-predictive activity,” and is hypothesized to be a transient active state preceding a decision that is caused by spontaneous fluctuations in the baseline activity of prefrontal neurons (Funahashi, 2017).
Although tasks involving motor actions are the primary method of studying intention (Haggard, 2005), free choices for abstract intentions have also been investigated in human (Soon et al., 2013). Soon et al. (2013) gave participants the freedom to add or subtract numbers under the constraints of an arithmetic task in an fMRI study. They trained independent classifiers to distinguish the spatial patterns of fMRI signals related to the two choices of adding and subtracting. They found that in medial prefrontal and parietal cortex they could accurately decode the decision up to four seconds before the participant reported their conscious awareness of their decision. This study reinforces that the neuronal signals preceding a decision to attend generalize beyond merely motor signals. Thus, studying willed cognitive acts may provide additional insights into whether or not there is a unified decision network for volition that spans the domains of cognition and action.
The forgoing selective review of the willed action literature provides the context in which we wish to place the work on willed attention. First, like the studies of neural activity preceding willed actions, willed attention can also be predicted by brain activity preceding the orienting of attention; i.e., as described above, Bengson et al. (2014) used EEG to demonstrate that in the approximately 1,000 msec prior to free choice decisions (but not earlier), EEG alpha power over parietal-occipital cortex predicted subsequent decisions to attend left vs. right. Thus, the emerging literature on willed attention is moving the discussion of intention, volition and free will from primarily the domain of motor actions to the broader domain of cognitive “acts” (c.f., Soon et al., 2013). But studies of cognitive acts present great challenges because there is typically no outwardly visible sign of when a covert cognitive function has occurred. Rather, as we are hoping to accomplish in our research program (e.g., Nadra et al., 2023), high temporal resolution non-invasive neural measures like EEG (and MEG) will have to be used to identify signs of the initiation of cognitive processes like attentional orienting in order to reveal the time course of willed acts of cognition. Such high-temporal resolution measures of brain activity permit the precursor brain activity and thus the antecedent brain states of willed attention to be identified separately from decision, post-decision and orienting-related brain activity. With such measures in hand, it will then be possible to understand the common and separable processes involved in willed attention and action, as well as willed cognition more generally.
While there are similarities in the investigation of willed attention and willed actions, there are also some differences. For example, in our original EEG studies of willed attention (Bengson et al., 2014, 2015), there were prompts indicating when subjects should decide where to attend. This experimental design differs from, for example, the work of Libet et al. (1983b) where subjects simply had to wait and then decide when to move; that is, they received no prompt. Because in our designs the subjects were uncertain as to whether (or when) they might be prompted to make a free choice about where to attend, the predictive EEG alpha signal during willed attention differs from the readiness potential in self-initiated motor actions. That is, our pre-prompt predictive EEG alpha pattern (Bengson et al., 2014) does not resemble the build-up of neural activity that is seen in the readiness potential in the studies of Libet, but rather is a more temporally circumscribed activity.
As noted above, one critique of Libet's research on willed action was the idea that the readiness potential is a general activator of intent, rather than being related to a specific decision vs. another (Haggard and Eimer, 1999; Haggard and Libet, 2001; Balaguer, 2014). In our research of willed attention, we distinguish the neural antecedents of a decision to shift attention to the left vs. the right before the onset of a prompt, and thus the predictive neural activity encodes a specific cognitive outcome and is not reflecting a generalized preparatory activity for simply making any decision (Bengson et al., 2014). This neural pre-prompt EEG alpha pattern may be a form of neural “noise” (stochastic neural activity) that biases free choice decisions (Shadlen and Roskies, 2012), or may reflect variations in specific attentional sets that are task and goal specific (e.g., attend left vs. attend right); the answer is not known.
The goal of the mini review was to introduce willed attention, and to place it in context by describing how it has been defined relative to the standard meaning of voluntary attention, as well as how it relates to the literature on self-initiated motor actions. Although there is now behavioral electrophysiological and neuroimaging evidence for the idea that willed attention involves (i) front-end decision processes in frontal cortex, (ii) changes in the network organization and interactions of decision and attention systems, and (iii) that these decision stages may be biased by ongoing stochastic brain states (i.e., the pre-prompt EEG alpha activity), there is much we do not understand about willed attention both theoretically and neuroscientifically.
One such area of uncertainty was presaged at the end of the last section. We do not know whether the pre-prompt predictive EEG alpha power changes observed in Bengson et al. (2014) reflect stochastic brain states (e.g., variations in general left vs. right hemisphere activation states) or are instead manifestations of task set states of the brain related to the specific task presented to the subjects. In our work on spatial attention, for example, the subjects know that they will either be cued to the left or right visual field location or be required to choose to attend to the left or right, which is a highly constrained task set. One might hypothesize that with such a task set in mind, the subjects were not in some sort of neutral brain states during the intertrial intervals, but were instead alternating or randomly varying between attending-left and attending-right brain states (unconsciously), and this might even have occurred in a systematic alternating pattern; by way of analogy, like a soccer goalie rocking back and forth prior to a penalty kick because they will only either jump left or right to defend the goal when the kick is made. If so, the predictive EEG pattern would be reflections of these varying brain states at different times, and would therefore tend to predict what the subjects would choose, even though the subjects were not consciously deciding in advance. The idea that subject invoke free will to make the decisions about whether to attend to the left or right is rather challenged by the idea that they might merely be going with a bias induced by a limited set of alternatives in a highly rarified laboratory setting. It is important to get clarity on this and related issues. So, experiments that are more naturalistic and involve fewer experimentally-induced constraints on potential free willed decisions, are needed to take this research forward. A first step might simply be to have several possible locations to choose to attend to, which would introduce more opportunities for free willed choices.
Another area of investigation that will be important in understanding willed attention will be to remove the experimenter control over when attention can be focused as well as over only where. The introduction of the prompt is an innovation over presenting instructive cues, but is still a highly constrained laboratory situation. As described above, we have taken a step in this direction by developing a task where subjects can exert a free choice over both where and when to allocation spatial attention (Nadra et al., 2023). More studies that investigate willed attention in more naturalistic contexts where free will choices are constrained less by the designs of the laboratory tasks are needed.
Investigating whether the pre-prompt alpha signals observed in willed attention reflect a mechanism that generalizes across different domains of willed attention (e.g., visual spatial, visual non-spatial, auditory, etc.) would provide insight into the nature of willed attention. Such a step will be important if willed attention is to prove useful for understanding intention, volition and free will (Norman and Shallice, 1986; Lau et al., 2004a,b; Humphreys et al., 2010).
Since it is known that selection history and unconscious influences can alter how we allocate attention (Theeuwes, 2018a), the neural antecedents of attentional decision making likely vary with different perceptual, cognitive and motor contexts (Mudrik et al., 2022). Thus, in the future, the neural activity associated with free choice decisions should be considered in the context that the decisions are being made (Bode et al., 2014), perhaps like a spectrum of volition that is engaged as needed by the presented scenario (Dresler et al., 2014). This could be investigated by considering how selection history in more naturalistic experimental designs could interact with willed attention decisions. Similarly, how reward and reinforcement influence willed attention will be important for understanding the constraints place on free will by the ongoing environmental and behavioral contexts.
Direct investigations of the interactions of willed attention and willed action in terms of both the underlying computational and neural of each will be an important step in understanding volition and free will. Put another way, willed attention and willed action should fit into a common theoretical framework and this needs to be elaborated. For example, integrating these domains as in the classic model of attention and action proposed by Norman and Shallice (1986), where controlled (willed) actions are contrasted with automatic actions (Shallice and Burgess, 1996). In this case, automatic is defined as a situation where an action is routine and does not require any explicit attention to complete, while controlled actions are those requiring deliberate conscious control to execute (Shiffrin and Schneider, 1984; Rubinstein et al., 2001; Carlisle and Woodman, 2011). For controlled actions, the model proposes a supervisory attentional system (SAS) which biases the activations of specific schemas, inhibiting those which are not relevant and activating those which are the most relevant out of all presented options (Shallice and Burgess, 1996). The necessity of attention varies based on the experience of the human executing the action. In the field of voluntary attention, most studies of attention using instructional cues engage some level of volition (allowing subjects to follow the experimenter's instructions), but have not adequately studied self-generated (“willed”) shifts of attention, which require more volition than instructed attention, while involuntary shifts of attention are more akin to automatic actions in the Norman and Shallice model, where they require very little to no volition to carry out. Because willed attention has the potential to allows us to study volition in a variety of scenarios that are more akin to real life (shifting attention with or without the use of cues and prompts), future research on willed attention may provide a new view on the nature of attention, intention, volition and free will that is different from the dominant approaches in research on voluntary attention or free choice actions.
To advance our theoretical models of voluntary attention, future work should consider willed attention research. Insights from the study of willed attention may also contribute to the ongoing debate about the terminology we use as a field (e.g., Buzsaki, 2020; Poeppel and Adolfi, 2020) and allow the field to revise our collective understanding of what constitutes truly voluntary attention. Finally, the study of willed attention may also provide insights into the mechanisms of intention, volition and free will, by expanding the work beyond the dominant paradigms of willed actions to enable the study of willed cognition, which is a path toward understanding what we mean by free will and what it relates to in the brain.
Willed attention is a form of top-down voluntary attention that is characterized by participants having the ability to choose where, what and/or when to selectively attend. It is focused attention that is self-generated in the absence of external cues or external control, as is found in many laboratory studies where cues are used to guide what we call voluntary attention. The study of willed attention thus aims to understand fully volitional shifts of attention, with a goal of revealing the underlying neural mechanisms involved, as well as how they might be the same or different from those revealed in cued (instructed) attention studies. But much beyond these proximal goals, studies of willed attention address the very nature of intention, volition and free will by providing additional methods and insights that can help us advance understanding of agency empirically and theoretically. Such advances will be necessary for a more complete description of human cognition, but also, and importantly, to support efforts to translate basic science knowledge into interventions that can help ameliorate losses of normal functioning from damage or disease that limit humans from converting their intentions to everyday actions (Nicolelis, 2022; Metzger et al., 2023).
JN and GM wrote and edited the review. All authors contributed to the article and approved the submitted version.
This work was supported by an NEI T32 EY015387 traineeship to JN and grants NIMH MH117991 and NSF 2318886 to GM and Mingzhou Ding.
We would like to thank Natalia Khodayari for her advice and insights and to our colleagues at UC Davis and the University of Florida who have conducted much of the work reviewed here. With respect to the latter, we often use terms like we in referring to published work, and in so doing, mean we, the Mangun Lab at UC Davis and the Ding Lab at the University of Florida. We also thank our collaborator Mingzhou Ding at the University of Florida for his insights and assistance with this mini-review. Dr. Ding is a PI on the grants that supported much of the research reviewed here.
The authors declare that the research was conducted in the absence of any commercial or financial relationships that could be construed as a potential conflict of interest.
The author(s) GM declared that they were an editorial board member of Frontiers, at the time of submission. This had no impact on the peer review process and the final decision.
All claims expressed in this article are solely those of the authors and do not necessarily represent those of their affiliated organizations, or those of the publisher, the editors and the reviewers. Any product that may be evaluated in this article, or claim that may be made by its manufacturer, is not guaranteed or endorsed by the publisher.
Aine, C. J., and Harter, M. R. (1984). Hemispheric differences in event-related potentials to stroop stimuli. Attention and color-word processing. Ann. NY. Acad. Sci. 425, 154–156. doi: 10.1111/j.1749-6632.1984.tb23525.x
Allan, P. G., Briggs, R. G., Conner, A. K., O'Neal, C. M., Bonney, P. A., Maxwell, B. D., et al. (2020). Parcellation-based tractographic modeling of the ventral attention network. J. Neurol. Sci. 408, 116548. doi: 10.1016/j.jns.2019.116548
Antonov, P. A., Chakravarthi, R., and Andersen, S. K. (2020). Too little, too late, and in the wrong place: Alpha band activity does not reflect an active mechanism of selective attention. NeuroImage 219, 117006. doi: 10.1016/j.neuroimage.2020.117006
Armstrong, K. M., and Moore, T. (2007). Rapid enhancement of visual cortical response discriminability by microstimulation of the frontal eye field. Proc. Natl. Acad. Sci. U S A. 104, 9499–9504. doi: 10.1073/pnas.0701104104
Asplund, C. L., Todd, J. J., Snyder, A. P., and Marois, R. (2010). A central role for the lateral prefrontal cortex in goal-directed and stimulus-driven attention. Nat. Neurosci. 13, 507–512. doi: 10.1038/nn.2509
Awh, E., Belopolsky, A. V., and Theeuwes, J. (2012). Top-down versus bottom-up attentional control: a failed theoretical dichotomy. Trends Cogn. Sci. 16, 437–443. doi: 10.1016/j.tics.2012.06.010
Baluch, F., and Itti, L. (2011). Mechanisms of top-down attention. Trends Neurosci. 34, 210–224. doi: 10.1016/j.tins.2011.02.003
Battistoni, E., Stein, T., and Peelen, M. V. (2017). Preparatory attention in visual cortex. Ann. N Y. Acad. Sci. 1396, 92–107. doi: 10.1111/nyas.13320
Behrmann, M., and Haimson, C. (1999). The cognitive neuroscience of visual attention. Curr. Opin. Neurobiol. 9, 158–163. doi: 10.1016/S0959-4388(99)80021-X
Bench, C. J., Frith, C. D., Grasby, P. M., Friston, K. J., Paulesu, E., Frackowiak, R. S., et al. (1993). Investigations of the functional anatomy of attention using the Stroop test. Neuropsychologia 31, 907–922. doi: 10.1016/0028-3932(93)90147-R
Bengson, J. J., Kelley, T., and Mangun, G. R. (2015). The neural correlates of volitional attention: a combined fMRI and ERP study. Hum. Brain Mapp. 36, 2443–2454. doi: 10.1002/hbm.22783
Bengson, J. J., Kelley, T. A., Zhang, X., Wang, J. L., and Mangun, G. R. (2014). Spontaneous neural fluctuations predict decisions to attend. J. Cogn. Neurosci. 26, 2578–2584. doi: 10.1162/jocn_a_00650
Bengson, J. J., Liu, Y., Khodayari, N., and Mangun, G. R. (2020). Gating by inhibition during top-down control of willed attention. Cogn. Neurosci. 11, 60–70. doi: 10.1080/17588928.2019.1648405
Bode, S., Murawski, C., Soon, C. S., Bode, P., Stahl, J., and Smith, P. L. (2014). Demystifying “free will”: the role of contextual information and evidence accumulation for predictive brain activity. Neurosci. Biobehav. Rev. 47, 636–645. doi: 10.1016/j.neubiorev.2014.10.017
Botvinick, M. M., Braver, T. S., Barch, D. M., Carter, C. S., and Cohen, J. D. (2001). Conflict monitoring and cognitive control. Psychol. Rev. 108, 624–652. doi: 10.1037/0033-295X.108.3.624
Braun, M. N., Wessler, J., and Friese, M. (2021). A meta-analysis of Libet-style experiments. Neurosci. Biobehav. Rev. 128, 182–198. doi: 10.1016/j.neubiorev.2021.06.018
Briggs, F., Mangun, G. R., and Usrey, W. M. (2013). Attention enhances synaptic efficacy and the signal-to-noise ratio in neural circuits. Nature 499, 476–480. doi: 10.1038/nature12276
Burock, M. A., Buckner, R. L., Woldorff, M. G., Rosen, B. R., and Dale, A. M. (1998). Randomized event-related experimental designs allow for extremely rapid presentation rates using functional MRI. Neuroreport 9, 3735–3739. doi: 10.1097/00001756-199811160-00030
Buschman, T. J., and Kastner, S. (2015). From behavior to neural dynamics: an integrated theory of attention. Neuron 88, 127–144. doi: 10.1016/j.neuron.2015.09.017
Buzsaki, G. (2020). The brain-cognitive behavior problem: a retrospective. eNeuro 7, e69. doi: 10.1523/ENEURO.0069-20.2020
Carlisle, N. B., and Woodman, G. F. (2011). Automatic and strategic effects in the guidance of attention by working memory representations. Acta Psychol. 137, 217–225. doi: 10.1016/j.actpsy.2010.06.012
Carrasco, M. (2011). Visual attention: the past 25 years. Vision Res. 51, 1484–1525. doi: 10.1016/j.visres.2011.04.012
Carrasco, M. (2018). How visual spatial attention alters perception. Cogn. Process. 19, 77–88. doi: 10.1007/s10339-018-0883-4
Carrasco, M., and Barbot, A. (2019). Spatial attention alters visual appearance. Curr. Opin. Psychol. 29, 56–64. doi: 10.1016/j.copsyc.2018.10.010
Chawla, D., Rees, G., and Friston, K. J. (1999). The physiological basis of attentional modulation in extrastriate visual areas. Nat. Neurosci. 2, 671–676. doi: 10.1038/10230
Chen, L., Zhu, P., Li, J., Song, H., Liu, H., Shen, M., et al. (2023). The modulation of expectation violation on attention: Evidence from the spatial cueing effects. Cognition 238, 105488. doi: 10.1016/j.cognition.2023.105488
Chica, A. B., Bartolomeo, P., and Lupianez, J. (2013). Two cognitive and neural systems for endogenous and exogenous spatial attention. Behav. Brain Res. 237, 107–123. doi: 10.1016/j.bbr.2012.09.027
Corbetta, M., Kincade, J. M., Ollinger, J. M., McAvoy, M. P., and Shulman, G. L. (2000). Voluntary orienting is dissociated from target detection in human posterior parietal cortex. Nat. Neurosci. 3, 292–297. doi: 10.1038/73009
Corbetta, M., Miezin, F. M., Dobmeyer, S., Shulman, G. L., and Petersen, S. E. (1990). Attentional modulation of neural processing of shape, color, and velocity in humans. Science 248, 1556–1559. doi: 10.1126/science.2360050
Corbetta, M., Patel, G., and Shulman, G. L. (2008). The reorienting system of the human brain: from environment to theory of mind. Neuron 58, 306–324. doi: 10.1016/j.neuron.2008.04.017
Corbetta, M., and Shulman, G. L. (2002). Control of goal-directed and stimulus-driven attention in the brain. Nat. Rev. Neurosci. 3, 201–215. doi: 10.1038/nrn755
Corbetta, M., and Shulman, G. L. (2011). Spatial neglect and attention networks. Ann. Rev. Neurosci. 34, 569–599. doi: 10.1146/annurev-neuro-061010-113731
Corbetta, M., Tansy, A. P., Stanley, C. M., Astafiev, S. V., Snyder, A. Z., and Shulman, G. L. (2005). A functional MRI study of preparatory signals for spatial location and objects. Neuropsychologia 43, 2041–2056. doi: 10.1016/j.neuropsychologia.2005.03.020
Das, S., Ding, M., Yi, W., and Mangun, G. R. (2023). Optimizing cognitive neuroscience experiments for separating event- related fMRI BOLD responses in non-randomized alternating designs. Front. Neuroimag. 2, 1068616. doi: 10.3389/fnimg.2023.1068616
David, N., Newen, A., and Vogeley, K. (2008). The “sense of agency” and its underlying cognitive and neural mechanisms. Consc. Cogn. 17, 523–534. doi: 10.1016/j.concog.2008.03.004
Dhamala, M., Rangarajan, G., and Ding, M. (2008a). Analyzing information flow in brain networks with nonparametric Granger causality. Neuroimage 41, 354–362. doi: 10.1016/j.neuroimage.2008.02.020
Dhamala, M., Rangarajan, G., and Ding, M. (2008b). Estimating Granger causality from fourier and wavelet transforms of time series data. Phys. Rev. Lett. 100, 018701. doi: 10.1103/PhysRevLett.100.018701
Ding, M., Chen, Y., and Bressler, S. L. (2006). “Granger causality: basic theory and application to neuroscience,” in Handbook of Time Series Analysis, eds. M. W. B. Schelter, and J. Timmer (London: Wiley-VCH Verlag), 437–460. doi: 10.1002/9783527609970.ch17
Donovan, I., Zhou, Y. J., and Carrasco, M. (2020). In search of exogenous feature-based attention. Atten. Percept. Psychophys. 82, 312–329. doi: 10.3758/s13414-019-01815-3
Dosenbach, N. U., Fair, D. A., Cohen, A. L., Schlaggar, B. L., and Petersen, S. E. (2008). A dual-networks architecture of top-down control. Trends Cogn. Sci. 12, 99–105. doi: 10.1016/j.tics.2008.01.001
Dresler, M., Eibl, L., Fischer, C. F., Wehrle, R., Spoormaker, V. I., Steiger, A., et al. (2014). Volitional components of consciousness vary across wakefulness, dreaming and lucid dreaming. Front. Psychol. 4, 987. doi: 10.3389/fpsyg.2013.00987
Egeth, H. (2018). Comment on theeuwes's characterization of visual selection. J. Cogn. 1, 26. doi: 10.5334/joc.29
Egner, T. (2014). CreatuRes. of habit (and control): a multi-level learning perspective on the modulation of congruency effects. Front. Psychol. 5, 1247. doi: 10.3389/fpsyg.2014.01247
Egner, T., and Hirsch, J. (2005). The neural correlates and functional integration of cognitive control in a Stroop task. Neuroimage 24, 539–547. doi: 10.1016/j.neuroimage.2004.09.007
Eimer, M. (1994). An erp study on visual-spatial priming with peripheral onsets. Psychophysiology 31, 154–163. doi: 10.1111/j.1469-8986.1994.tb01035.x
Eimer, M., Forster, B., Fieger, A., and Harbich, S. (2004). Effects of hand posture on preparatory control processes and sensory modulations in tactile-spatial attention. Clin. Neurophysiol. 115, 596–608. doi: 10.1016/j.clinph.2003.10.015
Eriksen, B. A., and Eriksen, C. W. (1974). Effects of noise letters upon the identification of a target letter in a nonsearch task. Percep. Psychophys. 16, 143–149. doi: 10.3758/BF03203267
Failing, M., and Theeuwes, J. (2018). Selection history: how reward modulates selectivity of visual attention. Psychon Bull Rev. 25, 514–538. doi: 10.3758/s13423-017-1380-y
Fecteau, J. H., Bell, A. H., and Munoz, D. P. (2004). Neural correlates of the automatic and goal-driven biases in orienting spatial attention. J. Neurophysiol. 92, 1728–1737. doi: 10.1152/jn.00184.2004
Fiebelkorn, I. C., and Kastner, S. (2020). Functional specialization in the attention network. Ann. Rev. Psychol. 71, 221–249. doi: 10.1146/annurev-psych-010418-103429
Filevich, E., Kuhn, S., and Haggard, P. (2013). There is no free won't: antecedent brain activity predicts decisions to inhibit. PLoS ONE 8, e53053. doi: 10.1371/journal.pone.0053053
Fox, M. D., Corbetta, M., Snyder, A. Z., Vincent, J. L., and Raichle, M. E. (2006). Spontaneous neuronal activity distinguishes human dorsal and ventral attention systems. Proc. Natl. Acad. Sci. U S A. 103, 10046–10051. doi: 10.1073/pnas.0604187103
Funahashi, S. (2017). Prefrontal contribution to decision-making under free-choice conditions. Front. Neurosci. 11, 431. doi: 10.3389/fnins.2017.00431
Gaspelin, N., and Luck, S. J. (2018). “Top-down” does not mean “voluntary”. J. Cogn. 1, 25. doi: 10.5334/joc.28
Gaspelin, N., Ruthruff, E., and Jung, K. (2014). Slippage theory and the flanker paradigm: an early-selection account of selective attention failures. J. Exp. Psychol. Hum. Percept. Perform. 40, 1257–1273. doi: 10.1037/a0036179
Gazzaley, A., and Nobre, A. C. (2011). Top-down modulation: bridging selective attention and working memory. Trends Cogn. Sci. 16, 129–135. doi: 10.1016/j.tics.2011.11.014
Gazzaniga, M. (2012). Who's in Charge? Free Will and the Science of the Brain. New York, NY: HarperCollins.
Geng, J. J., and Mangun, G. R. (2011). Right temporoparietal junction activation by a salient contextual cue facilitates target discrimination. Neuroimage 54, 594–601. doi: 10.1016/j.neuroimage.2010.08.025
Ghosh, S., and Maunsell, J. H. R. (2021). Single trial neuronal activity dynamics of attentional intensity in monkey visual area V4. Nat. Commun. 12, 2003. doi: 10.1038/s41467-021-22281-2
Giesbrecht, B., Woldorff, M. G., Song, A. W., and Mangun, G. R. (2003). Neural mechanisms of top-down control during spatial and feature attention. Neuroimage 19, 496–512. doi: 10.1016/S1053-8119(03)00162-9
Glover, G. H. (2011). Overview of functional magnetic resonance imaging. Neurosurg. Clin. N Am. 22, 133–139. doi: 10.1016/j.nec.2010.11.001
Gmeindl, L., Chiu, Y. C., Esterman, M. S., Greenberg, A. S., Courtney, S. M., and Yantis, S. (2016). Tracking the will to attend: Cortical activity indexes self-generated, voluntary shifts of attention. Atten. Percept. Psychophys. 78, 2176–2184. doi: 10.3758/s13414-016-1159-7
Green, J. J., and McDonald, J. J. (2010). The role of temporal predictability in the anticipatory biasing of sensory cortex during visuospatial shifts of attention. Psychophysiology 47, 1057–1065. doi: 10.1111/j.1469-8986.2010.01025.x
Gregoriou, G. G., Rossi, A. F., Ungerleider, L. G., and Desimone, R. (2014). Lesions of prefrontal cortex reduce attentional modulation of neuronal responses and synchrony in V4. Nat. Neurosci. 17, 1003–1011. doi: 10.1038/nn.3742
Gundlach, C., Moratti, S., Forschack, N., and Müller, M. M. (2020). Spatial attentional selection modulates early visual stimulus processing independently of visual alpha modulations. Cereb. Cortex 30, 3686–3703. doi: 10.1093/cercor/bhz335
Haggard, P. (2005). Conscious intention and motor cognition. Trends Cogn. Sci. 9, 290–295. doi: 10.1016/j.tics.2005.04.012
Haggard, P. (2019). The neurocognitive bases of human volition. Ann. Rev. Psychol. 70, 9–28. doi: 10.1146/annurev-psych-010418-103348
Haggard, P., and Eimer, M. (1999). On the relation between brain potentials and the awareness of voluntary movements. Exper. Brain Res. 126, 128–133. doi: 10.1007/s002210050722
Haggard, P., and Libet, B. (2001). Conscious intention and brain activity. J. Consc. Stud. 11, 47–63.
Harter, M. R., Miller, S. L., Price, N. J., Lalonde, M. E., and Keyes, A. L. (1989). Neural processes involved in directing attention. J. Cogn. Neurosci. 1, 223–237. doi: 10.1162/jocn.1989.1.3.223
He, B. J., Snyder, A. Z., Vincent, J. L., Epstein, A., Shulman, G. L., and Corbetta, M. (2007). Breakdown of functional connectivity in frontoparietal networks underlies behavioral deficits in spatial neglect. Neuron 53, 905–918. doi: 10.1016/j.neuron.2007.02.013
Heekeren, H. R., Marrett, S., and Ungerleider, L. G. (2008). The neural systems that mediate human perceptual decision making. Nat. Rev. Neurosci. 9, 467–479. doi: 10.1038/nrn2374
Heinze, H. J., Mangun, G. R., Burchert, W., Hinrichs, H., Scholz, M., Munte, T. F., et al. (1994). Combined spatial and temporal imaging of brain activity during visual selective attention in humans. Nature 372, 543–546. doi: 10.1038/372543a0
Helmholtz, V. H. (1867). “Handbuch der physiologischen Optik,” in den Text eingedruckten Holzschnitten und 11 Tafeln (Voss).
Hong, X., Sun, J., Bengson, J. J., Mangun, G. R., and Tong, S. (2015). Normal aging selectively diminishes alpha lateralization in visual spatial attention. Neuroimage 106, 353–363. doi: 10.1016/j.neuroimage.2014.11.019
Hopf, J. M., and Mangun, G. R. (2000). Shifting visual attention in space: an electrophysiological analysis using high spatial resolution mapping. Clin. Neurophysiol. 111, 1241–1257. doi: 10.1016/S1388-2457(00)00313-8
Hopfinger, J. B., Buonocore, M. H., and Mangun, G. R. (2000). The neural mechanisms of top-down attentional control. Nat. Neurosci. 3, 284–291. doi: 10.1038/72999
Hopfinger, J. B., Camblin, C. C., and Parks, E. L. (2010). Isolating the internal in endogenous attention. Psychophysiology 47, 739–747. doi: 10.1111/j.1469-8986.2010.00981.x
Hopfinger, J. B., and Mangun, G. R. (1998). Reflexive attention modulates processing of visual stimuli in human extrastriate cortex. Psychol. Sci. 9, 441–447. doi: 10.1111/1467-9280.00083
Hopfinger, J. B., and Mangun, G. R. (2001). Tracking the influence of reflexive attention on sensory and cognitive processing. Cogn. Affect. Behav. Neurosci. 1, 56–65. doi: 10.3758/CABN.1.1.56
Hopfinger, J. B., and Maxwell, J. S. (2005). Appearing and disappearing stimuli trigger a reflexive modulation of visual cortical activity. Brain Res. Cogn. Brain Res. 25, 48–56. doi: 10.1016/j.cogbrainres.2005.04.010
Hopfinger, J. B., and Ries, A. J. (2005). Automatic versus contingent mechanisms of sensory-driven neural biasing and reflexive attention. J. Cogn. Neurosci. 17, 1341–1352. doi: 10.1162/0898929055002445
Hopfinger, J. B., and West, V. M. (2006). Interactions between endogenous and exogenous attention on cortical visual processing. Neuroimage 31, 774–789. doi: 10.1016/j.neuroimage.2005.12.049
Hopfinger, J. B., Woldorff, M. G., Fletcher, E. M., and Mangun, G. R. (2001). Dissociating top-down attentional control from selective perception and action. Neuropsychologia 39, 1277–1291. doi: 10.1016/S0028-3932(01)00117-8
Humphreys, G. W., Yoon, E. Y., Kumar, S., Lestou, V., Kitadono, K., Roberts, K. L., et al. (2010). The interaction of attention and action: from seeing action to acting on perception. Br. J. Psychol. 101, 185–206. doi: 10.1348/000712609X458927
Indovina, I., and Macaluso, E. (2007). Dissociation of stimulus relevance and saliency factors during shifts of visuospatial attention. Cereb. Cortex 17, 1701–1711. doi: 10.1093/cercor/bhl081
James, W. (1890). Principles of Psychology. Washington, DC: Henry Holt and Co. doi: 10.1037/10538-000
Jensen, O., and Mazaheri, A. (2010). Shaping functional architecture by oscillatory alpha activity: gating by inhibition. Front. Hum. Neurosci. 4, 186. doi: 10.3389/fnhum.2010.00186
Jigo, M., and Carrasco, M. (2018). Attention alters spatial resolution by modulating second-order processing. J. Vis. 18, 2. doi: 10.1167/18.7.2
Jonides, J. (1981). “Voluntary versus automatic control over the mind's eye,” in Attention and Performance IX, eds. J. Long and A. D. Baddeley (Mahwah, NJ: Erlbaum), 187–203.
Kastner, S., Pinsk, M. A., De Weerd, P., Desimone, R., and Ungerleider, L. G. (1999). Increased activity in human visual cortex during directed attention in the absence of visual stimulation. Neuron 22, 751–761. doi: 10.1016/S0896-6273(00)80734-5
Kastner, S., and Ungerleider, L. G. (2001). The neural basis of biased competition in human visual cortex. Neuropsychologia 39, 1263–1276. doi: 10.1016/S0028-3932(01)00116-6
Katsuki, F., and Constantinidis, C. (2014). Bottom-up and top-down attention: different processes and overlapping neural systems. Neuroscientist 20, 509–521. doi: 10.1177/1073858413514136
Kelly, S. P., Gomez-Ramirez, M., and Foxe, J. J. (2009). The strength of anticipatory spatial biasing predicts target discrimination at attended locations: a high-density EEG study. Eur. J. Neurosci. 30, 2224–2234. doi: 10.1111/j.1460-9568.2009.06980.x
Kerns, J. G., Cohen, J. D., MacDonald, A. W. 3rd, Cho, R. Y., Stenger, V. A., and Carter, C. S. (2004). Anterior cingulate conflict monitoring and adjustments in control. Science 303, 1023–1026. doi: 10.1126/science.1089910
King, J. A., Korb, F. M., and Egner, T. (2012). Priming of control: implicit contextual cuing of top-down attentional set. J. Neurosci. 32, 8192–8200. doi: 10.1523/JNEUROSCI.0934-12.2012
Klein, R. M. (1980). “Does oculomotor readiness mediate cognitive control of visual attention?” in Attention and Performance VIII, ed. R. S. Nickerson (Mahwah, NJ: Erlbaum), 250–276.
Klein, R. M. (1994). Perceptual-motor expectancies interact with covert visual orienting under conditions of endogenous but not exogenous control. Can. J. Exp. Psychol. 48, 167–181. doi: 10.1037/1196-1961.48.2.167
Koch, C., and Ullman, S. (1985). Shifts in selective visual attention: towards the underlying neural circuitry. Hum. Neurobiol. 4, 219–227.
Kornhuber, H. H., and Deecke, L. (1964). Hirnpotentialänderungen beim Menschen vor und nach Willkürbewegungen, dargestellt mit Magnetbandspeicherung und Rückwärtsanalyse. Arch. Eur. J. Physiol. 281, 52.
Kornhuber, H. H., and Deecke, L. (2016). Brain potential changes in voluntary and passive movements in humans: readiness potential and reafferent potentials. Pflugers Arch. 468, 1115–1124. doi: 10.1007/s00424-016-1852-3
Lamy, D., Leber, A., and Egeth, H. E. (2004). Effects of task relevance and stimulus-driven salience in feature-search mode. J. Exp. Psychol. Hum. Percept. Perform. 30, 1019–1031. doi: 10.1037/0096-1523.30.6.1019
Lau, H. C., Rogers, R. D., Haggard, P., and Passingham, R. E. (2004a). Attention to intention. Science 303, 1208–1210. doi: 10.1126/science.1090973
Lau, H. C., Rogers, R. D., and Passingham, R. E. (2006). On measuring the perceived onsets of spontaneous actions. J. Neurosci. 26, 7265–7271. doi: 10.1523/JNEUROSCI.1138-06.2006
Lau, H. C., Rogers, R. D., Ramnani, N., and Passingham, R. E. (2004b). Willed action and attention to the selection of action. Neuroimage 21, 1407–1415. doi: 10.1016/j.neuroimage.2003.10.034
Lavazza, A. (2016). Free will and neuroscience: from explaining freedom away to new ways of operationalizing and measuring it. Front. Hum. Neurosci. 10, 262. doi: 10.3389/fnhum.2016.00262
Lavazza, A. (2019). Why cognitive sciences do not prove that free will is an epiphenomenon. Front. Psychol. 10, 326. doi: 10.3389/fpsyg.2019.00326
Lavie, N. (2011). ‘Load theory’ of attention. Nilli Lavie. Curr. Biol. 21, R645–647. doi: 10.1016/j.cub.2011.05.051
Libet, B. (1985). Unconscious cerebral initiative and the role of conscious will in voluntary action. Behav. Brain Sci. 8, 529–566. doi: 10.1017/S0140525X00044903
Libet, B., Gleason, C. A., Wright, E. W., and Pearl, D. K. (1983a). Time of conscious intention to act in relation to onset of cerebral activity (readiness-potential). The unconscious initiation of a freely voluntary act. Brain 106, 623–642. doi: 10.1093/brain/106.3.623
Libet, B., Wright, E. W. Jr., and Gleason, C. A. (1983b). Preparation- or intention-to-act, in relation to pre-event potentials recorded at the vertex. Electroencephalogr. Clin. Neurophysiol. 56, 367–372. doi: 10.1016/0013-4694(83)90262-6
Liu, T., Abrams, J., and Carrasco, M. (2009). Voluntary attention enhances contrast appearance. Psychol. Sci. 20, 354–362. doi: 10.1111/j.1467-9280.2009.02300.x
Liu, T., and Hou, Y. (2013). A hierarchy of attentional priority signals in human frontoparietal cortex. J. Neurosci. 33, 16606–16616. doi: 10.1523/JNEUROSCI.1780-13.2013
Liu, Y., Hong, X., Bengson, J. J., Kelley, T. A., Ding, M., and Mangun, G. R. (2017). Deciding where to attend: Large-scale network mechanisms underlying attention and intention revealed by graph-theoretic analysis. Neuroimage 157, 45–60. doi: 10.1016/j.neuroimage.2017.05.048
Luck, S. J., Chelazzi, L., Hillyard, S. A., and Desimone, R. (1997). Neural mechanisms of spatial selective attention in areas V1, V2, and V4 of macaque visual cortex. J. Neurophysiol. 77, 24–42. doi: 10.1152/jn.1997.77.1.24
Luck, S. J., and Hillyard, S. A. (1995). The role of attention in feature detection and conjunction discrimination: an electrophysiological analysis. Int. J. Neurosci. 80, 281–297. doi: 10.3109/00207459508986105
Luck, S. J., Hillyard, S. A., Mouloua, M., Woldorff, M. G., Clark, V. P., and Hawkins, H. L. (1994). Effects of spatial cuing on luminance detectability: psychophysical and electrophysiological evidence for early selection. J. Exp. Psychol. Hum. Percept. Perform. 20, 887–904. doi: 10.1037/0096-1523.20.4.887
Lupianez, J., Decaix, C., Sieroff, E., Chokron, S., Milliken, B., and Bartolomeo, P. (2004). Independent effects of endogenous and exogenous spatial cueing: inhibition of return at endogenously attended target locations. Exp. Brain Res. 159, 447–457. doi: 10.1007/s00221-004-1963-5
Lupianez, J., Milliken, B., Solano, C., Weaver, B., and Tipper, S. P. (2001). On the strategic modulation of the time course of facilitation and inhibition of return. Q J. Exp. Psychol. A. 54, 753–773. doi: 10.1080/713755990
Mangun, G. R., and Hillyard, S. A. (1991). Modulations of sensory-evoked brain potentials indicate changes in perceptual processing during visual-spatial priming. J. Exp. Psychol. Hum. Percept. Perform. 17, 1057–1074. doi: 10.1037/0096-1523.17.4.1057
Mangun, G. R., Hopfinger, J. B., and Jha, A. P. (2000). Integrating electrophysiology and neuroimaging in the study of brain function. Adv. Neurol. 84, 35–49.
Marcos, E., and Genovesio, A. (2016). Determining monkey free choice long before the choice is made: the principal role of prefrontal neurons involved in both decision and motor processes. Front. Neural. Circ. 10, 75. doi: 10.3389/fncir.2016.00075
Marxen, M., Graff, J. E., Riedel, P., and Smolka, M. N. (2023). Observing cognitive processes in time through functional MRI model comparison. Hum. Brain Mapp. 44, 1359–1370. doi: 10.1002/hbm.26114
McMains, S. A., Fehd, H. M., Emmanouil, T. A., and Kastner, S. (2007). Mechanisms of feature- and space-based attention: response modulation and baseline increases. J. Neurophysiol. 98, 2110–2121. doi: 10.1152/jn.00538.2007
Meehan, T. P., Bressler, S. L., Tang, W., Astafiev, S. V., Sylvester, C. M., Shulman, G. L., et al. (2017). Top-down cortical interactions in visuospatial attention. Brain Struct. Funct. 222, 3127–3145. doi: 10.1007/s00429-017-1390-6
Mesulam, M. M. (1981). A cortical network for directed attention and unilateral neglect. Ann. Neurol. 10, 309–325. doi: 10.1002/ana.410100402
Metzger, S. L., Littlejohn, K. T., Silva, A. B., Moses, D. A., Seaton, M. P., Wang, R., et al. (2023). A high-performance neuroprosthesis for speech decoding and avatar control. Nature 620, 1037–1046. doi: 10.1038/s41586-023-06443-4
Miller, E. K., and Buschman, T. J. (2013). Cortical circuits for the control of attention. Curr. Opin. Neurobiol. 23, 216–222. doi: 10.1016/j.conb.2012.11.011
Mione, V., Tsujimoto, S., and Genovesio, A. (2019). Neural correlations underlying self-generated decision in the frontal pole cortex during a cued strategy task. Neuroscience 404, 519–528. doi: 10.1016/j.neuroscience.2019.02.023
Moore, J. W., and Obhi, S. S. (2012). Intentional binding and the sense of agency: a review. Consc. Cogn. 21, 546–561. doi: 10.1016/j.concog.2011.12.002
Moran, J., and Desimone, R. (1985). Selective attention gates visual processing in the extrastriate cortex. Science 229, 782–784. doi: 10.1126/science.4023713
Motter, B. C. (1993). Focal attention produces spatially selective processing in visual cortical areas V1, V2, and V4 in the presence of competing stimuli. J. Neurophysiol. 70, 909–919. doi: 10.1152/jn.1993.70.3.909
Mudrik, L., Arie, I. G., Amir, Y., Shir, Y., Hieronymi, P., Maoz, U., et al. (2022). Free will without consciousness? Trends Cogn. Sci. 26, 555–566. doi: 10.1016/j.tics.2022.03.005
Muller, H. J., and Rabbitt, P. M. (1989). Reflexive and voluntary orienting of visual attention: time course of activation and resistance to interruption. J. Exp. Psychol. Hum. Percept. Perform. 15, 315–330. doi: 10.1037/0096-1523.15.2.315
Nadra, J. G., Bengson, J. J., Morales, A. B., and Mangun, G. R. (2023). Attention without constraint: alpha lateralization in uncued willed attention. eNeuro 10, e258. doi: 10.1523/ENEURO.0258-22.2023
Nicolelis, M. A. L. (2022). Brain-machine-brain interfaces as the foundation for the next generation of neuroprostheses. Natl. Sci. Rev. 9, nwab206. doi: 10.1093/nsr/nwab206
Nitschke, J. B., and Mackiewicz, K. L. (2005). Prefrontal and anterior cingulate contributions to volition in depression. Int. Rev. Neurobiol. 67, 73–94. doi: 10.1016/S0074-7742(05)67003-1
Nobre, A. C., Sebestyen, G. N., Gitelman, D. R., Mesulam, M. M., Frackowiak, R. S., and Frith, C. D. (1997). Functional localization of the system for visuospatial attention using positron emission tomography. Brain 120, 515–533. doi: 10.1093/brain/120.3.515
Norman, D. A., and Shallice, T. (1986). “Attention to action: willed and automatic control of behavior,” in Consciousness and Self-Regulation, eds. R. J. Davidson, G. E. Schwartz, and D. Shapiro (New York, NY: Springer), 1–18. doi: 10.1007/978-1-4757-0629-1_1
Pacherie, E., and Haggard, P. (2011). “What are intentions?” in Conscious Will and Responsibility: A Tribute to Benjamin Libet, eds. W. Sinnott-Armstrong and L. Nadel (Oxford, Oxford Academic press), 70–84. doi: 10.1093/acprof:oso/9780195381641.003.0008
Passingham, R. E., Bengtsson, S. L., and Lau, H. C. (2010). Medial frontal cortex: from self-generated action to reflection on one's own performance. Trends Cogn. Sci. 14, 16–21. doi: 10.1016/j.tics.2009.11.001
Petersen, S. E., and Posner, M. I. (2012). The attention system of the human brain: 20 years after. Annu. Rev. Neurosci. 35, 73–89. doi: 10.1146/annurev-neuro-062111-150525
Pezzulo, G., Rigoli, F., and Friston, K. J. (2018). Hierarchical active inference: a theory of motivated control. Trends Cogn. Sci. 22, 294–306. doi: 10.1016/j.tics.2018.01.009
Poeppel, D., and Adolfi, F. (2020). Against the epistemological primacy of the hardware: the brain from inside out, turned upside down. eNeuro 7, e215. doi: 10.1523/ENEURO.0215-20.2020
Popov, T., Gips, B., Kastner, S., and Jensen, O. (2019). Spatial specificity of alpha oscillations in the human visual system. Hum. Brain Mapp. 40, 4432–4440. doi: 10.1002/hbm.24712
Posner, M. I. (1980). Orienting of attention. Q J. Exp. Psychol. 32, 3–25. doi: 10.1080/00335558008248231
Posner, M. I. (2016). Orienting of attention: Then and now. Q J. Exp. Psychol. 69, 1864–1875. doi: 10.1080/17470218.2014.937446
Posner, M. I., and Petersen, S. E. (1990). The attention system of the human brain. Annu. Rev. Neurosci. 13, 25–42. doi: 10.1146/annurev.ne.13.030190.000325
Posner, M. I., and Rothbart, M. K. (1998). Attention, self-regulation and consciousness. Philos. Trans. R. Soc. Lond. B Biol. Sci. 353, 1915–1927. doi: 10.1098/rstb.1998.0344
Posner, M. I., and Rothbart, M. K. (2023). Fifty years integrating neurobiology and psychology to study attention. Biol. Psychol. 180, 108574. doi: 10.1016/j.biopsycho.2023.108574
Posner, M. I., Snyder, C. R., and Davidson, B. J. (1980). Attention and the detection of signals. J. Exp. Psychol. 109, 160–174. doi: 10.1037/0096-3445.109.2.160
Rajan, A., Meyyappan, S., Liu, Y., Samuel, I. B. H., Nandi, B., Mangun, G. R., et al. (2021). The microstructure of attentional control in the dorsal attention network. J. Cogn. Neurosci. 33, 965–983. doi: 10.1162/jocn_a_01710
Rajan, A., Siegel, S. N., Liu, Y., Bengson, J., Mangun, G. R., and Ding, M. (2018). Theta oscillations index frontal decision-making and mediate reciprocal frontal-parietal interactions in willed attention. Cereb. Cortex. 29, 2832–2843. doi: 10.1093/cercor/bhy149
Ray, K. L., Ragland, J. D., MacDonald, A. W., Gold, J. M., Silverstein, S. M., Barch, D. M., et al. (2020). Dynamic reorganization of the frontal parietal network during cognitive control and episodic memory. Cogn. Affect. Behav. Neurosci. 20, 76–90. doi: 10.3758/s13415-019-00753-9
Rihs, T. A., Michel, C. M., and Thut, G. (2007). Mechanisms of selective inhibition in visual spatial attention are indexed by alpha-band EEG synchronization. Eur. J. Neurosci. 25, 603–610. doi: 10.1111/j.1460-9568.2007.05278.x
Ristic, J., Wright, A., and Kingstone, A. (2007). Attentional control and reflexive orienting to gaze and arrow cues. Psychon. Bull. Rev. 14, 964–969. doi: 10.3758/BF03194129
Romei, V., Gross, J., and Thut, G. (2010). On the role of prestimulus alpha rhythms over occipito-parietal areas in visual input regulation: correlation or causation? J. Neurosci. 30, 8692–8697. doi: 10.1523/JNEUROSCI.0160-10.2010
Rubinstein, J. S., Meyer, D. E., and Evans, J. E. (2001). Executive control of cognitive processes in task switching. J. Exp. Psychol. Hum. Percept. Perform. 27, 763–797. doi: 10.1037/0096-1523.27.4.763
Schulz, K. P., Bedard, A. V., Czarnecki, R., and Fan, J. (2011). Preparatory activity and connectivity in dorsal anterior cingulate cortex for cognitive control. Neuroimage 57, 242–250. doi: 10.1016/j.neuroimage.2011.04.023
Seghezzi, S., and Haggard, P. (2022). Volition and “free will”. PsyArXiv. doi: 10.31234/osf.io/e46pb
Seiss, E., Driver, J., and Eimer, M. (2009). Effects of attentional filtering demands on preparatory ERPs elicited in a spatial cueing task. Clin. Neurophysiol. 120, 1087–1095. doi: 10.1016/j.clinph.2009.03.016
Shadlen, M. N., and Roskies, A. L. (2012). The neurobiology of decision-making and responsibility: reconciling mechanism and mindedness. Front. Neurosci. 6, 56. doi: 10.3389/fnins.2012.00056
Shallice, T., and Burgess, P. (1996). The domain of supervisory processes and temporal organization of behaviour. Philos. Trans. R Soc. Lond. B Biol. Sci. 351, 1405–1411. doi: 10.1098/rstb.1996.0124
Shiffrin, R. M., and Schneider, W. (1984). Automatic and controlled processing revisited. Psychol. Rev. 91, 269–276. doi: 10.1037/0033-295X.91.2.269
Slagter, H. A., Weissman, D. H., Giesbrecht, B., Kenemans, J. L., Mangun, G. R., Kok, A., et al. (2006). Brain regions activated by endogenous preparatory set shifting as revealed by fMRI. Cogn. Affect. Behav. Neurosci. 6, 175–189. doi: 10.3758/CABN.6.3.175
Soon, C. S., Brass, M., Heinze, H. J., and Haynes, J. D. (2008). Unconscious determinants of free decisions in the human brain. Nat. Neurosci. 11, 543–545. doi: 10.1038/nn.2112
Soon, C. S., He, A. H., Bode, S., and Haynes, J. D. (2013). Predicting free choices for abstract intentions. Proc. Natl. Acad. Sci. U S A. 110, 6217–6222. doi: 10.1073/pnas.1212218110
Srinivasan, L., Asaad, W. F., Ginat, D. T., Gale, J. T., Dougherty, D. D., Williams, Z. M., et al. (2013). Action initiation in the human dorsal anterior cingulate cortex. PLoS ONE 8, e55247. doi: 10.1371/journal.pone.0055247
Sylvester, C. M., Shulman, G. L., Jack, A. I., and Corbetta, M. (2009). Anticipatory and stimulus-evoked blood oxygenation level-dependent modulations related to spatial attention reflect a common additive signal. J. Neurosci. 29, 10671–10682. doi: 10.1523/JNEUROSCI.1141-09.2009
Szczepanski, S. M., Konen, C. S., and Kastner, S. (2010). Mechanisms of spatial attention control in frontal and parietal cortex. J. Neurosci. 30, 148–160. doi: 10.1523/JNEUROSCI.3862-09.2010
Szczepanski, S. M., Pinsk, M. A., Douglas, M. M., Kastner, S., and Saalmann, Y. B. (2013). Functional and structural architecture of the human dorsal frontoparietal attention network. Proc. Natl. Acad. Sci. U S A. 110, 15806–15811. doi: 10.1073/pnas.1313903110
Tamber-Rosenau, B. J., Esterman, M., Chiu, Y. C., and Yantis, S. (2011). Cortical mechanisms of cognitive control for shifting attention in vision and working memory. J. Cogn. Neurosci. 23, 2905–2919. doi: 10.1162/jocn.2011.21608
Tassinari, G., Aglioti, S., Chelazzi, L., Marzi, C. A., and Berlucchi, G. (1987). Distribution in the visual field of the costs of voluntarily allocated attention and of the inhibitory after-effects of covert orienting. Neuropsychologia 25, 55–71. doi: 10.1016/0028-3932(87)90043-1
Taylor, P. C., Rushworth, M. F., and Nobre, A. C. (2008). Choosing where to attend and the medial frontal cortex: an FMRI study. J. Neurophysiol. 100, 1397–1406. doi: 10.1152/jn.90241.2008
Theeuwes, J. (2018a). Visual selection: usually fast and automatic; seldom slow and volitional. J. Cogn. 1, 29. doi: 10.5334/joc.13
Theeuwes, J. (2018b). Visual selection: usually fast and automatic; seldom slow and volitional; a reply to commentaries. J. Cogn. 1, 21. doi: 10.5334/joc.32
Thut, G., Nietzel, A., Brandt, S. A., and Pascual-Leone, A. (2006). Alpha-band electroencephalographic activity over occipital cortex indexes visuospatial attention bias and predicts visual target detection. J. Neurosci. 26, 9494–9502. doi: 10.1523/JNEUROSCI.0875-06.2006
Trachel, R. E., Clerc, M., and Brochier, T. G. (2015). Decoding covert shifts of attention induced by ambiguous visuospatial cues. Front. Hum. Neurosci. 9, 358. doi: 10.3389/fnhum.2015.00358
Treisman, A., and Fearnley, S. (1969). The Stroop test: selective attention to colours and words. Nature 222, 437–439. doi: 10.1038/222437a0
Treisman, A. M., and Gelade, G. (1980). A feature-integration theory of attention. Cogn. Psychol. 12, 97–136. doi: 10.1016/0010-0285(80)90005-5
Treue, S., and Maunsell, J. H. (1996). Attentional modulation of visual motion processing in cortical areas MT and MST. Nature 382, 539–541. doi: 10.1038/382539a0
Tsotsos, J. K., Abid, O., Kotseruba, I., and Solbach, M. D. (2021). On the control of attentional processes in vision. Cortex 137, 305–329. doi: 10.1016/j.cortex.2021.01.001
Turner, M. H., Sanchez Giraldo, L. G., Schwartz, O., and Rieke, F. (2019). Stimulus- and goal-oriented frameworks for understanding natural vision. Nat. Neurosci. 22, 15–24. doi: 10.1038/s41593-018-0284-0
Uithol, S., Gorgen, K., Pischedda, D., Toni, I., and Haynes, J. D. (2023). The effect of context and reason on the neural correlates of intentions. Heliyon 9, e17231. doi: 10.1016/j.heliyon.2023.e17231
van Veen, V., Cohen, J. D., Botvinick, M. M., Stenger, V. A., and Carter, C. S. (2001). Anterior cingulate cortex, conflict monitoring, and levels of processing. Neuroimage 14, 1302–1308. doi: 10.1006/nimg.2001.0923
Van Voorhis, S., and Hillyard, S. A. (1977). Visual evoked potentials and selective attention to points in space. Percept. Psychophys. 22, 54–62. doi: 10.3758/BF03206080
Vossel, S., Geng, J., and Fink, G. R. (2014). Dorsal and ventral attention systems: Distinct neural circuits but collaborative roles. Neuroscientist 20, 150–159. doi: 10.1177/1073858413494269
Wager, T. D., Jonides, J., and Reading, S. (2004). Neuroimaging studies of shifting attention: a meta-analysis. Neuroimage 22, 1679–1693. doi: 10.1016/j.neuroimage.2004.03.052
Walsh, B. J., Buonocore, M. H., Carter, C. S., and Mangun, G. R. (2011). Integrating conflict detection and attentional control mechanisms. J. Cogn. Neurosci. 23, 2211–2221. doi: 10.1162/jocn.2010.21595
Weissman, D. H., Gopalakrishnan, A., Hazlett, C. J., and Woldorff, M. G. (2005). Dorsal anterior cingulate cortex resolves conflict from distracting stimuli by boosting attention toward relevant events. Cereb. Cortex 15, 229–237. doi: 10.1093/cercor/bhh125
Weissman, D. H., Warner, L. M., and Woldorff, M. G. (2009). Momentary reductions of attention permit greater processing of irrelevant stimuli. Neuroimage 48, 609–615. doi: 10.1016/j.neuroimage.2009.06.081
Winterer, G., Adams, C. M., Jones, D. W., and Knutson, B. (2002). Volition to action–an event-related fMRI study. Neuroimage 17, 851–858. doi: 10.1006/nimg.2002.1232
Woldorff, M. G., Hazlett, C. J., Fichtenholtz, H. M., Weissman, D. H., Dale, A. M., and Song, A. W. (2004). Functional parcellation of attentional control regions of the brain. J. Cogn. Neurosci. 16, 149–165. doi: 10.1162/089892904322755638
Wolpe, N., and Rowe, J. B. (2014). Beyond the “urge to move”: objective measures for the study of agency in the post-Libet era. Front. Hum. Neurosci. 8, 450. doi: 10.3389/fnhum.2014.00450
Worden, M. S., Foxe, J. J., Wang, N., and Simpson, G. V. (2000). Anticipatory biasing of visuospatial attention indexed by retinotopically specific alpha-band electroencephalography increases over occipital cortex. J. Neurosci. 20, RC63. doi: 10.1523/JNEUROSCI.20-06-j0002.2000
Wyble, B., Callahan-Flintoft, C., Chen, H., Marinov, T., Sarkar, A., and Bowman, H. (2020). Understanding visual attention with RAGNAROC: A reflexive attention gradient through neural AttRactOr competition. Psychol. Rev. 127, 1163–1198. doi: 10.1037/rev0000245
Yantis, S., and Jonides, J. (1984). Abrupt visual onsets and selective attention: evidence from visual search. J. Exp. Psychol. Hum. Percept. Perform. 10, 601–621. doi: 10.1037/0096-1523.10.5.601
Yee, D. M., Crawford, J. L., Lamichhane, B., and Braver, T. S. (2021). Dorsal anterior cingulate cortex encodes the integrated incentive motivational value of cognitive task performance. J. Neurosci. 41, 3707–3720. doi: 10.1523/JNEUROSCI.2550-20.2021
Keywords: attention, volition, willed action, willed attention, free will
Citation: Nadra JG and Mangun GR (2023) Placing willed attention in context: a review of attention and free will. Front. Cognit. 2:1205618. doi: 10.3389/fcogn.2023.1205618
Received: 14 April 2023; Accepted: 10 October 2023;
Published: 06 November 2023.
Edited by:
Dariusz Asanowicz, Jagiellonian University, PolandReviewed by:
Alfredo Spagna, Columbia University, United StatesCopyright © 2023 Nadra and Mangun. This is an open-access article distributed under the terms of the Creative Commons Attribution License (CC BY). The use, distribution or reproduction in other forums is permitted, provided the original author(s) and the copyright owner(s) are credited and that the original publication in this journal is cited, in accordance with accepted academic practice. No use, distribution or reproduction is permitted which does not comply with these terms.
*Correspondence: John G. Nadra, am5hZHJhQHVjZGF2aXMuZWR1
Disclaimer: All claims expressed in this article are solely those of the authors and do not necessarily represent those of their affiliated organizations, or those of the publisher, the editors and the reviewers. Any product that may be evaluated in this article or claim that may be made by its manufacturer is not guaranteed or endorsed by the publisher.
Research integrity at Frontiers
Learn more about the work of our research integrity team to safeguard the quality of each article we publish.