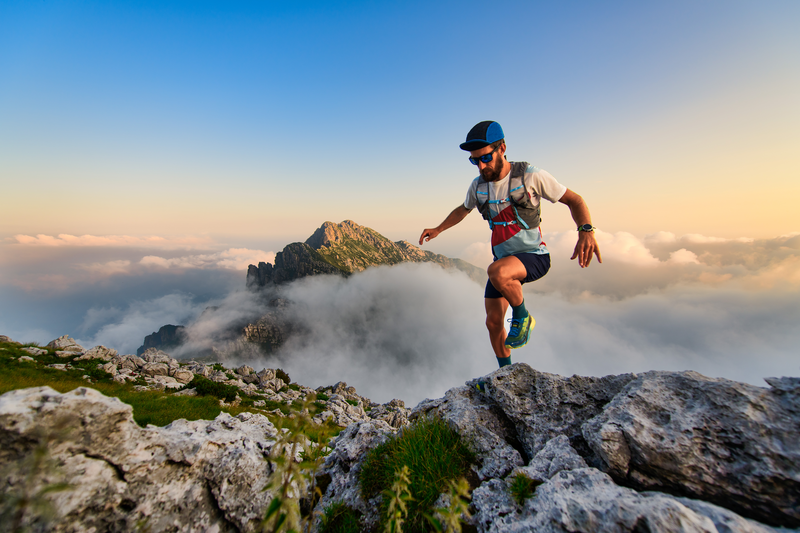
94% of researchers rate our articles as excellent or good
Learn more about the work of our research integrity team to safeguard the quality of each article we publish.
Find out more
CLINICAL TRIAL article
Front. Clin. Diabetes Healthc.
Sec. Diabetes Cardiovascular Complications
Volume 6 - 2025 | doi: 10.3389/fcdhc.2025.1537005
The final, formatted version of the article will be published soon.
You have multiple emails registered with Frontiers:
Please enter your email address:
If you already have an account, please login
You don't have a Frontiers account ? You can register here
Background: Type 2 diabetes (T2DM) is a chronic metabolic disorder that affects approximately 10.5% of the world's population and is an independent risk factor for cardiovascular complications, including sudden cardiac death (SCD). Inhibitors of sodium-glucose co-transporter type 2 (iSGLT2), particularly dapagliflozin, have emerged as promising treatments in patients with T2DM and with heart failure and chronic kidney disease, demonstrating the ability to significantly reduce major cardiovascular events.However, the exact mechanisms that promote the observed benefits are still not fully understood. Objective: In this study, we sought to understand the mechanisms associated with the benefits of dapagliflozin by evaluating various electrophysiological parameters of the electrocardiogram (ECG) in patients with T2DM. A randomized, multicenter, prospective study with 174 patients with T2DM divided into two groups: one receiving dapagliflozin plus optimized guideline directed medical therapy (GDMT) and the other optimized GDMT without SGLT2 inhibitors. Clinical, electrocardiographic, laboratory, and echocardiographic evaluations were performed initially and after three months.Descriptive and inferential statistics were used, with a significance level of 0.05. Result:This study shows that in patients treated with dapagliflozin plus GDMT, a significant reduction in the duration of the interval from the peak of the T wave to the end of the T wave (TpTe), the QTc interval, and the ratio between the TpTe/QT intervals was observed, with no change in other electrocardiographic variables such as QT interval dispersion, JT peak interval, or changes in the QRS complex and T wave axes (QRS-T angle). Conclusion: In patients with T2DM, dapagliflozin significantly shortened the TpTe and QTc intervals, as well as the TpTe/QT ratio. These results suggest a reduction in ventricular electrical remodeling, highlighting a potential cardioprotective effect of dapagliflozin.
Keywords: type 2 diabetes, dapagliflozin, ventricular repolarization, electrocardiogram, cardiac arrhythmias
Received: 29 Nov 2024; Accepted: 11 Mar 2025.
Copyright: © 2025 Campos, Moreira and da Fonseca. This is an open-access article distributed under the terms of the Creative Commons Attribution License (CC BY). The use, distribution or reproduction in other forums is permitted, provided the original author(s) or licensor are credited and that the original publication in this journal is cited, in accordance with accepted academic practice. No use, distribution or reproduction is permitted which does not comply with these terms.
* Correspondence:
Rodrigo Noronha Campos, Brazilian Society of Cardiology, São Paulo, Brazil
Disclaimer: All claims expressed in this article are solely those of the authors and do not necessarily represent those of their affiliated organizations, or those of the publisher, the editors and the reviewers. Any product that may be evaluated in this article or claim that may be made by its manufacturer is not guaranteed or endorsed by the publisher.
Research integrity at Frontiers
Learn more about the work of our research integrity team to safeguard the quality of each article we publish.