- 1Department of Renal Medicine, Sir Charles Gairdner Hospital, Perth, WA, Australia
- 2School of Medicine, University of Wollongong, Wollongong, NSW, Australia
- 3Depatment of Nephrology, Wollongong Hospital, Wollongong, NSW, Australia
- 4School of Biomedical Sciences, University of Western Australia, Perth, WA, Australia
- 5Department of Renal Medicine, Cairns Hospital, Cairns, QLD, Australia
- 6Medical School, University of Western Australia, Perth, WA, Australia
The burden of type 2 diabetes and related complications has steadily increased over the last few decades and is one of the foremost global public health threats in the 21st century. Diabetes is one of the leading causes of chronic kidney disease and kidney failure and is an important contributor to the cardiovascular morbidity and mortality in this population. In addition, up to one in three patients who have received kidney transplants develop post-transplant diabetes, but the management of this common complication continues to pose a significant challenge for clinicians. In this review, we will describe the global prevalence and temporal trend of kidney failure attributed to diabetes mellitus in both developing and developed countries. We will examine the survival differences between treated kidney failure patients with and without type 2 diabetes, focusing on the survival differences in those on maintenance dialysis or have received kidney transplants. With the increased availability of novel hypoglycemic agents, we will address the potential impacts of these novel agents in patients with diabetes and kidney failure and in those who have developed post-transplant diabetes.
Introduction
The number of people with diabetes has more than doubled over the last two decades, and diabetes has become one of the predominant global health threats of the 21st century. It is estimated that more than 50 million people worldwide have diabetes, and diabetes-related complications and disability are associated with substantial economic healthcare cost and loss of productivity (1).
Type 2 diabetes is the leading cause of kidney failure globally. In Australia, the proportion of treated kidney failure patients with diabetes increased from 42% to 52% between 2005 and 2019, respectively, with diabetes as the primary cause of kidney failure in 39% of patients receiving kidney replacement therapy in 2019 (2, 3). Similar proportions have been reported in the United States (44% in 2012 and 48% in 2017), United Kingdom (20% in 2005 and 30% in 2018), and New Zealand (46% in 2005 and 59% in 2019) (2–7). The increasing prevalence of diabetes in many countries has further contributed to the expanding burden of kidney failure patients, heralding the development of an epidemic of diabetes-related complications worldwide (8).
Similar to the general population (9–11), the presence of type 2 diabetes in patients with kidney failure on maintenance dialysis or received kidney transplants was associated with over a twofold greater risk of cardiovascular disease (CVD) and all-cause mortality compared to patients without diabetes and kidney failure, reinforcing the negative health consequences of diabetes across the health spectrum (12, 13). Given the disproportionate increased risk of CVD and the reduced projected survival in patients with type 2 diabetes and kidney failure, understanding the short- and long-term health risks in this complex patient group, as well as the potential utility of novel anti-diabetic agents are essential when assessing and planning dialysis or kidney transplantation. Furthermore, up to 30% of patients develop post-transplant diabetes mellitus (PTDM) after kidney transplantation, but the diagnosis, risk factors, and the risk of adverse long-term health outcome of this common complication remain inadequately defined. There is considerable uncertainty as to whether the targets of PTDM and treatment options, particularly the availability of novel anti-diabetic agents, should be extrapolated from the general population, and clinicians will need to be cautious of the caveats of inferring findings from the general population to patients with PTDM where the pathophysiology of the disease process, potential drug–drug interactions, and competing comorbidities are vastly different.
This review focuses on the current understanding of the epidemiology and risk of adverse health outcomes of patients with type 2 diabetes and kidney failure and those who have developed PTDM, including the uncertainty in the management strategy and use of novel anti-diabetic agents in these patients.
Global Burden of Diabetic Kidney Disease: Definition and Incidence
The designation of diabetic kidney disease is to describe the development of chronic kidney disease and kidney failure attributable to the effects of diabetes. The pathogenesis of this disease process is generally conceptualized as the product of prolonged exposure to the toxic effects of hyperglycemia, but it is likely that this process is representative of several comparable and competing pathogenic processes (for example, concurrent hypertensive kidney damage), with the eventual consequence of progressive kidney function decline and ensuing kidney failure (14). The precise estimates of the incidence of chronic kidney disease or kidney failure attributed to diabetes is often underestimated because the diagnosis of diabetic nephropathy was previously established on clinical rather than histological findings. At present, the term diabetic nephropathy is often advocated only in patients with the characteristic lesions of diabetic glomerulopathy established on histology, and diabetic kidney disease occurs in patients with diabetes mellitus and reduced kidney function that can be from many diverse causes, including hypertensive nephrosclerosis and unresolved acute kidney failure (15–17). The biopsy rates to establish the presence of diabetic nephropathy as cause of chronic kidney disease or kidney failure is generally less than 15%, and the variation in the reported incidences of diabetic nephropathy is likely attributed to dissimilarities in clinical practice guidelines and healthcare resources between countries, lack of accurate biopsy data collection, and the heterogeneity of kidney biopsy indications in patients with diabetes (18–20). Misclassification bias of the underlying cause of chronic kidney disease or kidney failure in patients with diabetes is therefore possible, as several population cohort studies have shown that an alternative diagnosis such as glomerulonephritis or a mixed process (evidence of diabetic nephropathy with a second diagnosis) may be relatively common in patients with diabetes (12, 21). Given the availability of novel therapies for diabetes and glomerulonephritis, there is a renewed interest from clinicians and researchers to pursue kidney biopsy more aggressively in patients with diabetes and kidney disease to avoid undue delay recognition and subsequent treatment of the underlying disease. At present, the decision to undertake a kidney biopsy is often determined by the treating clinicians. Even though there is generally no agreement on the criteria for kidney biopsy in patients with diabetes, the presence of “atypical” clinical characteristics such as worsening of proteinuria, presence of active urine sediment, presence of systemic diseases, and absence of concurrent microvascular complications (such as retinopathy) may influence the decision for kidney biopsy (22–25). In addition, kidney biopsy is often useful to establish the presence of non-diabetic disease and quantify the extent of chronic damage in patients with diabetes who have experienced rapid and sustained drop in kidney function or unexplained kidney failure (26, 27). Future research aiming to investigate specific biomarkers (e.g., urine proteomic profile) or distinct clinical and patient phenotypes may assist clinicians to reliably identify patients likely to have diabetic kidney disease and those patients where kidney biopsy should be considered (28, 29).
The global incidence of treated kidney failure from diabetic kidney disease varies widely between countries, with the incidence ranging from as low as 10% reported in Romania up to almost 70% in Singapore and Malaysia (30). Figure 1 shows the incidence rate of patients with treated kidney failure secondary to diabetes by selected countries (2018 data) (31, 32). Although these differences may reflect the true disparities in the population rates of diabetes mellitus and diabetic kidney disease, it is likely that variations in the accuracy and completeness of data captured and the lack of diagnostic criteria for diabetic nephropathy or diabetic kidney disease contributed to these findings. With a greater understanding and potential accessibility and acceptance of conservative, non-dialysis pathway as a treatment option for kidney failure in many countries, the true total incidence of patients with chronic kidney disease or kidney failure from diabetic kidney disease is uncertain but is likely to be considerably underestimated as these patients are often not adequately captured by registries (30). For low- and low-middle-income countries such as Mexico where an estimated 90% of patients with kidney failure do not receive renal replacement therapy and data capture is unreliable, the incidences of untreated and treated kidney failure patients with diabetic kidney disease in these countries are essentially unknown (8). With the temporal change in the survival and pattern of cause-specific deaths in low- and middle-income countries, the burden of kidney failure has substantially increased in parallel with the high-income countries (33). As a way of illustration, several lower-middle- and upper-middle-income Southeast Asian nations have reported substantial increases in the incidence of kidney failure secondary to diabetic kidney disease, with Thailand and the Philippines reporting a respective 1,448% and 378% increase between 2001 and 2015. Other data indicate that this is not an isolated event, with yearly increases in the incidence of treated kidney failure due to diabetic kidney disease documented in many countries surveyed between 2003 and 2016 (33).
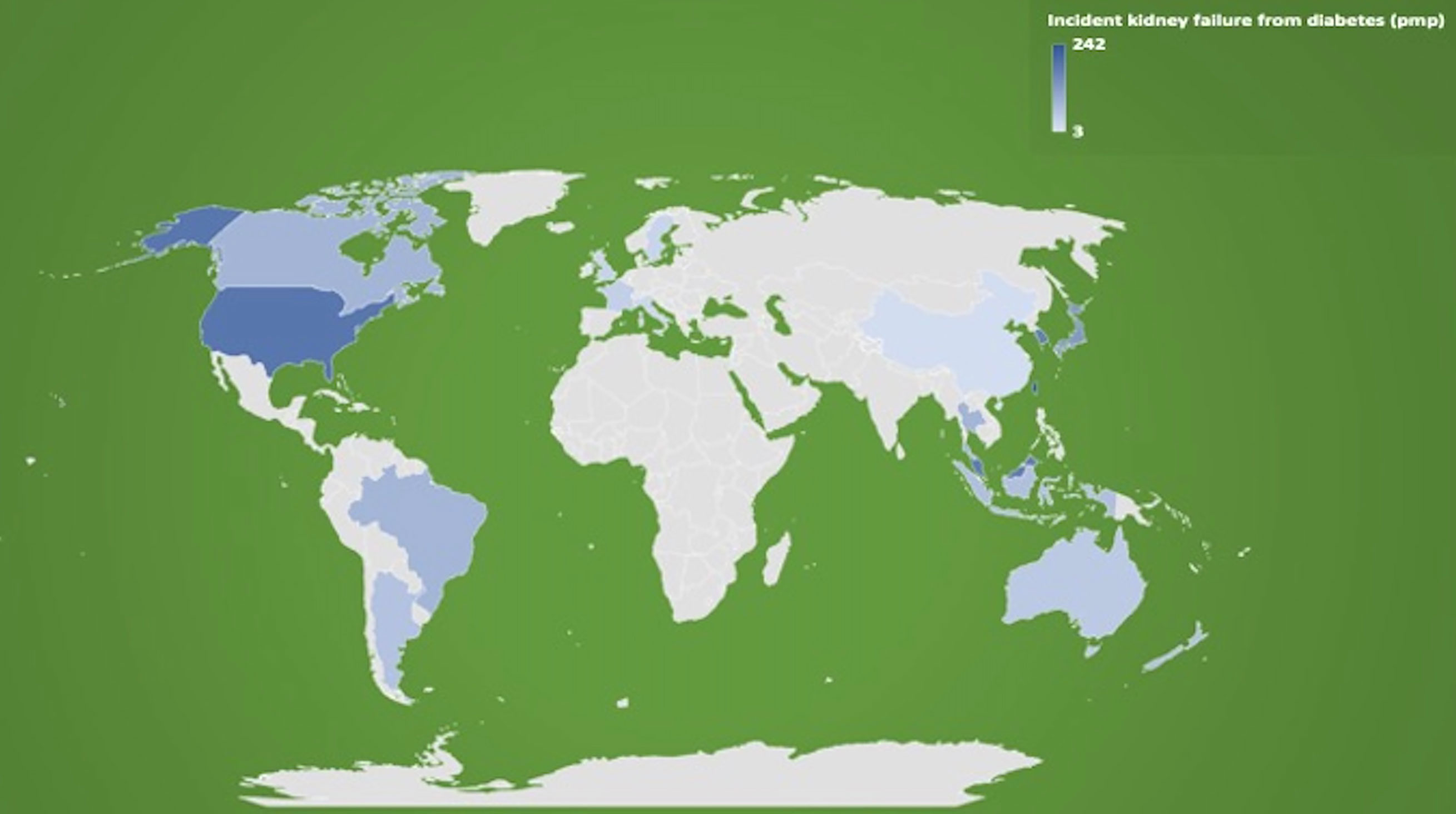
Figure 1 Incidence rates of treated kidney failure patients attributed to diabetes as primary cause of kidney failure (expressed as per million population [pmp] in 2018), by selected countries. Data extracted from the 2020 United States Renal Data System (USRDS) Annual Data Report and the Australia and New Zealand Dialysis and Transplant (ANZDATA) Registry 2019 Report (references 21, 22).
Patients on Dialysis With Type 2 Diabetes: Impact on Long-Term Outcomes
Population cohort studies have consistently shown that the presence of type 2 diabetes is associated with an excess risk of mortality in patients with chronic kidney disease and kidney failure compared to those without diabetes (12, 34–36). However, the association between diabetes status and mortality in kidney failure patients may be modified by several patient and disease characteristics. With the increasing recognition that diabetic nephropathy as the primary cause of kidney failure may represent a distinct clinical and prognostic phenotype compared to patients with type 2 diabetes and kidney failure attributed to non-diabetic nephropathy, re-classification of diabetic nephropathy according to the exact cause has been proposed (37, 38). In a study of 15,419 dialysis patients using data from the European Renal Association-European Dialysis and Transplant Association (ERA-EDTA) Registry, all-cause mortality was 20% higher in patients with diabetes as cause of kidney failure compared to patients with diabetes as a comorbid condition, independent of age and gender (39). These findings were corroborated in a contemporary study from Australia and New Zealand of 56,552 incident dialysis patients between 1980 and 2014, of which 15,829 (28%) had type 2 diabetes and diabetic nephropathy and 4993 (9%) had type 2 diabetes and non-diabetic nephropathy (12). In this study, patients with type 2 diabetes and kidney failure secondary to both diabetic nephropathy and non-diabetic nephropathy have higher risks of all-cause and CVD mortality in the competing risk analysis. Among patients with type 2 diabetes, all-cause (adjusted subdistributional hazard ratio 1.17, 95% confidence intervals 1.10–1.22) and CVD mortality (adjusted subdistributional hazard ratio 1.20, 95% confidence intervals 1.12–1.28) were significantly greater for patients with diabetic nephropathy than for those with non-diabetic nephropathy, emphasizing that these two disease processes may be clinically distinct. Similar to the observation in the general population (40), age modified the association between diabetes and mortality such that the magnitude of the risk of all-cause and CVD mortality was greater in younger patients, particularly those with diabetic nephropathy as cause of kidney failure (12). It is likely that kidney failure attributed to diabetic nephropathy is a surrogate metric of more severe and prolonged duration of diabetes, which may potentially explain the higher risk of mortality observed in this population. However, the possibility of misclassification bias cannot be disregarded as less than 15% of patients with diabetes have a diagnostic biopsy to confirm the underlying cause of kidney failure (12).
The prevalence of newly diagnosed diabetes following dialysis initiation varies between 4% and 16%, with the prevalence rate generally higher in patients maintained on peritoneal dialysis (41–45). However, the difference in the prevalence of newly diagnosed diabetes between peritoneal and hemodialysis patients remains inconsistent (46, 47). Similar to prevalent diabetes, the development of newly diagnosed diabetes is associated with higher mortality risk, although the magnitude of the survival disadvantage is less than those with pre-dialysis diabetes (44, 48).
Kidney Transplantation in Patients With Type 2 Diabetes: Impact on Long-Term Outcomes
There is often uncertainty with regard to the suitability of kidney transplantation in patients with diabetes and kidney failure, balancing between the expected improved health outcomes from transplantation versus the reduced life expectancy of this population compared to patients without diabetes. Nevertheless, clinicians must be cognizant of the projected incremental gains in survival and improved quality of life following successful kidney transplantation compared with maintenance dialysis treatment, with modeled data showing a substantial survival benefit for patients with diabetes.
In the United States Renal Data System study of 252,358 patients with treated kidney failure under the age of 70 years, kidney transplantation reduced the risk of mortality by over 70% compared to remaining on the waiting list (annual mortality rate of the cohort of 11%). The projected survival gain after transplantation was over 11 years among patients with diabetes, considerably higher than the survival gain in other kidney failure patients without diabetes (49). Similarly, modeled data from Australia and New Zealand showed that wait-listed and transplanted patients with diabetes aged 45 years and 60 years will achieve cumulative incremental survival gains of 1.5 and 0.5 life years, respectively. These modeled scenarios appeared to be cost-effective, with respective incremental cost-effectiveness ratio (ICER) of $8,965 and $21,506 per life years saved, and below the cost-effectiveness threshold of $50,000 for every life year saved (50, 51). These findings suggest that kidney transplantation should continue to be offered for appropriate patients with diabetes and kidney failure.
Despite the survival advantage from kidney transplantation in patients with type 2 diabetes and kidney failure, the long-term survival post-kidney transplantation remains inferior compared to kidney transplant recipients without diabetes. However, recent data from the United States showed a temporal improvement in the survival of kidney transplant recipients with diabetes over the last decade, achieving mortality rates comparable to recipients without diabetes in the most recent era (52). In this study of 1,688 kidney transplant recipients, 413 (24%) had pre-transplant diabetes, of which 75% had type 2 diabetes. The mortality of recipients with diabetes had declined by 12% between 1996 and 2007, which was not observed in those without diabetes. Consequently, the magnitude of the survival disadvantage experienced by recipients with diabetes reduced over time such that after 2004, there was no longer a statistically significant difference in 5-year mortality between kidney transplant recipients with and without diabetes [hazard ratio (HR) 1.45 (95% confidence intervals (95% CI) 0.74, 2.87; p = 0.28)] (52). These findings parallel the findings in the general population and suggest that improved management strategies combined with the availability of novel treatment options may have substantially reduced the survival disadvantage of patients with diabetes (53, 54). However, a more contemporaneous study from Australia and New Zealand challenged this initial observation. In this study of 10,714 kidney transplant recipients (9% with type 2 diabetes) spanning almost two decades between 1994 and 2012, the authors reported a significant survival disadvantage of recipients with pre-transplant type 2 diabetes compared to those without diabetes, with the magnitude of this difference more apparent in younger recipients (age <40 years: adjusted HR [95% CI] 5.16 [2.84, 9.35]; age 40–55 years: 2.08 [1.62, 2.66]; >55 years: 1.41 [1.17, 1.71]; referent: no diabetes). There was no temporal improvement in the survival disadvantage of recipients with type 2 diabetes such that the survival difference remains constant across the study time period. CVD and infection were the two main drivers of the higher mortality rates, with recipients with diabetes experiencing almost a threefold increased risk of these complications compared to those without diabetes (13). The disparity in the study findings may reflect the size of the population cohorts (single centre vs. bi-national registry study), inclusion of recipients with and without type 1 and 2 diabetes, and the potential for type II statistical error with a short follow-up time in the study from the United States. Nevertheless, these studies do highlight the continuing survival disadvantage of recipients with diabetes but do raise important questions about the uncertainty in the optimal pre- and post-transplant management strategy of these recipients and whether younger recipients with type 2 diabetes represent a distinct clinical phenotype that are associated with poorer long-term health outcomes. These findings should challenge clinicians and researchers to gain a better understanding of the pathophysiology and natural progression post-transplant of recipients with diabetes and how to integrate novel treatments into the management strategy for kidney transplant candidates and recipients with diabetes.
Post-Transplant Diabetes Mellitus
Incidence
PTDM is an established and frequent complication after kidney transplantation, occurring in between 10% and 50% of recipients in the first 12 months post-transplantation (55–60), with an annual incidence of approximately 6%–15% thereafter (47, 61). The observed large variations in the incidence of PTDM are likely related to the frequency and types of screening practices and diagnostic criteria to identify recipients with PTDM, as well as systematic differences in the management of hyperglycemia in the post-transplant period. Consistent with the reported natural history of abnormal glucose regulation in the general population (62), the occurrence of glucose dysregulation is a dynamic process after kidney transplantation, likely related to the progressive reduction in overall immunosuppression including corticosteroids. Several studies have shown substantial movements of kidney transplant recipients between states of normal glucose regulation, pre-diabetes and PTDM beyond 12-months post-transplant. Although a number of recipients with pre-diabetes will progress towards PTDM, there is generally a decline in the proportion of recipients with pre-diabetes and PTDM with up to 1 in 2 recipients with pre-diabetes or PTDM normalizing their glucose regulation on follow-up testing (63–65). Clinicians should be aware of these fluxes between pre-diabetes or PTDM and normal glucose regulation states and should consider re-screening and adapting the management strategy according to the dynamic glucose regulation status, therefore avoiding the unnecessary continuation of oral hypoglycemia treatment(s) or “misclassifying” recipients as having pre-diabetes or PTDM.
Screening for PTDM
The optimal screening method for PTDM remains unknown, with the Kidney Disease Improving Global Outcomes (KDIGO) 2009 guideline advocating fasting blood glucose, oral glucose tolerance test (OGTT), and/or glycated hemoglobin (HBA1c; level 1C evidence) but the frequency of screening remains unknown (level 2D evidence) (66). However, the screening and formal diagnosis of PTDM can be made from 6 weeks post-transplantation, thereby avoiding the incorrect classification of recipients with transient, postoperative hyperglycemia of little clinical significance. According to the British Clinical Diabetologist and Renal Association guidelines, OGTT is considered the current gold standard for the diagnosis of PTDM (grade 1B evidence: strong recommendation, moderate-quality evidence) (67). Even though the standard diagnostic criteria for diabetes described by the American Diabetes Association (ADA) are utilized (68), transplant clinicians will need to be cognizant of the limitations of when adapting these measures for kidney transplant recipients where a distinct diurnal variation of afternoon and evening hyperglycemia typically occurs (69). The diagnostic utility of a number of screening tests to identify kidney transplant recipients with pre-diabetes and PTDM remains unclear, with unknown thresholds of fasting blood glucose, OGTT, and HBA1c that would provide the best balance between sensitivity and specificity. To use a diagnostic test effectively in clinical practice, clinicians will need to recognize how well each diagnostic test (with the varying thresholds) distinguishes between those recipients with and without pre-diabetes and PTDM (70). However, cohort studies of diagnostic tests to identify kidney transplant recipients with and without pre-diabetes and PTDM are frequently methodologically imperfect, and the reported findings are often not generalizable to cohorts of differing characteristics and clinical practices. Utilization of a single threshold to inform practice may potentially misinform or misclassify recipients with and without pre-diabetes or PTDM (71). The diagnostic utility of other screening tests such as fructosamine, capillary blood glucose, and homeostasis model assessment-insulin resistance (HOMA-IR) score or the combination of a panel of screening tests may provide greater accuracy in identifying those with pre-diabetes or PTDM but will need to be validated in large external population cohorts (60, 72–74). The clinical utility of continuous glucose monitoring post-kidney transplant to identify PTDM or as a means to monitor response to treatment remains unknown.
Risk Factors
The pathogenesis of PTDM is likely to be multifactorial, with traditional (shared risk factors for type 2 diabetes in the general population) and transplant-related risk factors contributing to this high risk (Table 1). While many of the risk factors are non-modifiable, it does help to identify those patients who may benefit from closer monitoring and/or lifestyle intervention post-transplant. The chronic exposure to immunosuppressive agents represents a unique risk factor to kidney transplant recipients, attributed to the toxic or inhibitory (possibly reversible) effects of these drugs to pancreatic islet beta cells and manifesting as insulin resistance or impaired insulin secretion (75–80). There is likely a gradation variation between the different types of immunosuppressive agents and risk of PTDM, with tacrolimus consistently associated with a higher risk of PTDM compared to other agents (55, 81, 82). The associations between several chronic viral infections such as cytomegalovirus (CMV) and hepatitis C with increased risk of PTDM have been observed. The mechanistic pathway for this association remains unclear but may be related to the direct viral-induced toxic effect on beta cells, induction of insulin resistance secondary to hepatic steatosis, and/or excess production of pro-inflammatory cytokines (83–89). Other less recognized risk factors for PTDM include the presence of autosomal dominant polycystic kidney disease and pre- and post-transplant hypomagnesemia, but these associations remain inconsistent and may be related to the residual effects of post-transplant confounding factors (90–95). The failure of magnesium supplementation to improve insulin resistance and glucose metabolism in two small, randomized trials in kidney transplant recipients has challenged the potential causal relationship between hypomagnesemia and the development of PTDM (96, 97).
Impact of PTDM on Long-Term Health Outcomes
The association between abnormal glucose regulation and adverse long-term health outcomes in kidney transplant recipients is well established, with an incremental risk of allograft loss, all-cause mortality, fatal and non-fatal CVD, and/or reduced quality of life being apparent from the pre-diabetes stage to the onset of PTDM (56, 98–104). Furthermore, PTDM also contributes to the accelerated atherosclerotic vascular disease burden, particularly in transplant recipients with prevalent vascular disease burden (105, 106). As patients with PTDM share common metabolic CVD risk factors with those with pre-transplant diabetes, the mechanistic pathways of abnormalities in insulin sensitivity and insulin secretion resulting in adverse CVD outcomes are likely to be similar (107–111). A recent population cohort study from Canada showed that kidney transplant recipients with PTDM were 40% less likely to experience major adverse cardiovascular events (MACE) post-transplant but had exhibited similarly high rates of all-cause and CVD mortality compared to those with pre-transplant diabetes (112). In this study, the incidence rates (95% CI) of CVD and all-cause mortality between 1 and 3 years post-transplant for recipients with PTDM were 6.6 (2.5–17.6) and 31.4 (20.5–48.2) per 1000 person-years, respectively. These compared with respective 7.1 (4.7-10.7) and 25.9 (21.2, 31.7) per 1000 person-years for recipients with pre-transplant diabetes, suggesting the importance of early screening and identification of recipients with PTDM (112). Predictably, the cost associated with each new case of PTDM is in excess of USD$21,000 by 2-years post-transplant (47), likely related to the diagnosis and treatment of common diabetes-related complications typically observed in the general population including hospitalizations for severe hyper- and hypoglycemia, ophthalmic complications, neurological complications, CVD, and peripheral vascular disease (113–115).
Treatment
Prevention of Kidney Failure and Treatment of Diabetes in Patients With Diabetic Kidney Disease
The landscape of diabetic kidney disease treatment has changed significantly over the past decade, particularly with the emergent evidence of the cardiac and nephroprotective benefits of sodium-glucose cotransporter-2 (SGLT2) inhibitors. The availability of SGLT2 inhibitors and other novel agents may potentially slow the upward trend of the incidence of diabetic kidney disease and improvement in diabetes-related complications (Tables 2, 3). Figure 2 outlines the timeline of treatment for diabetic kidney disease and clinical trials suggesting positive nephroprotective outcomes (116, 119, 172–184).
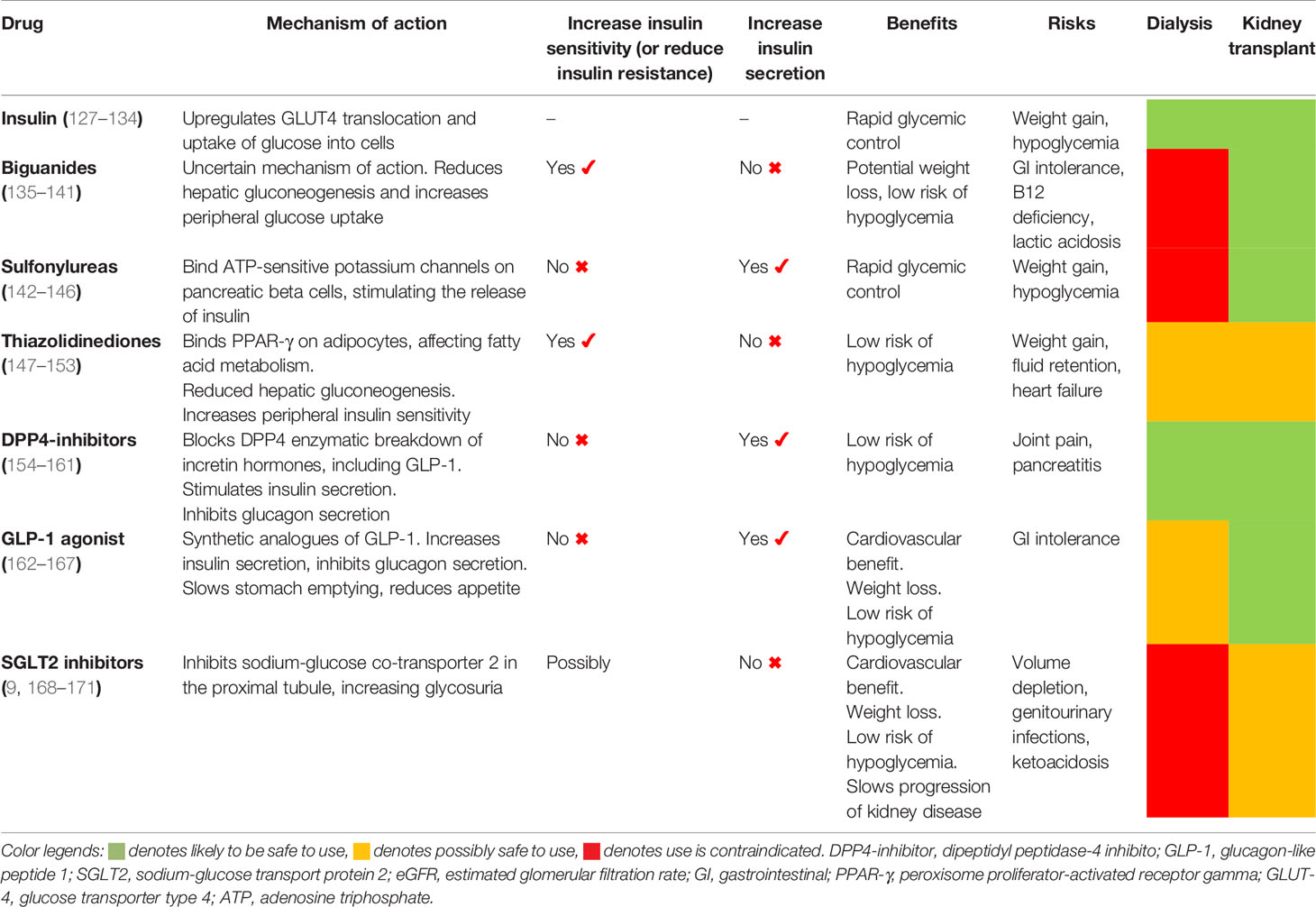
Table 3 Mechanism of actions and clinical concerns of potential diabetes treatment options in kidney failure patients maintained on dialysis or have received kidney transplants.
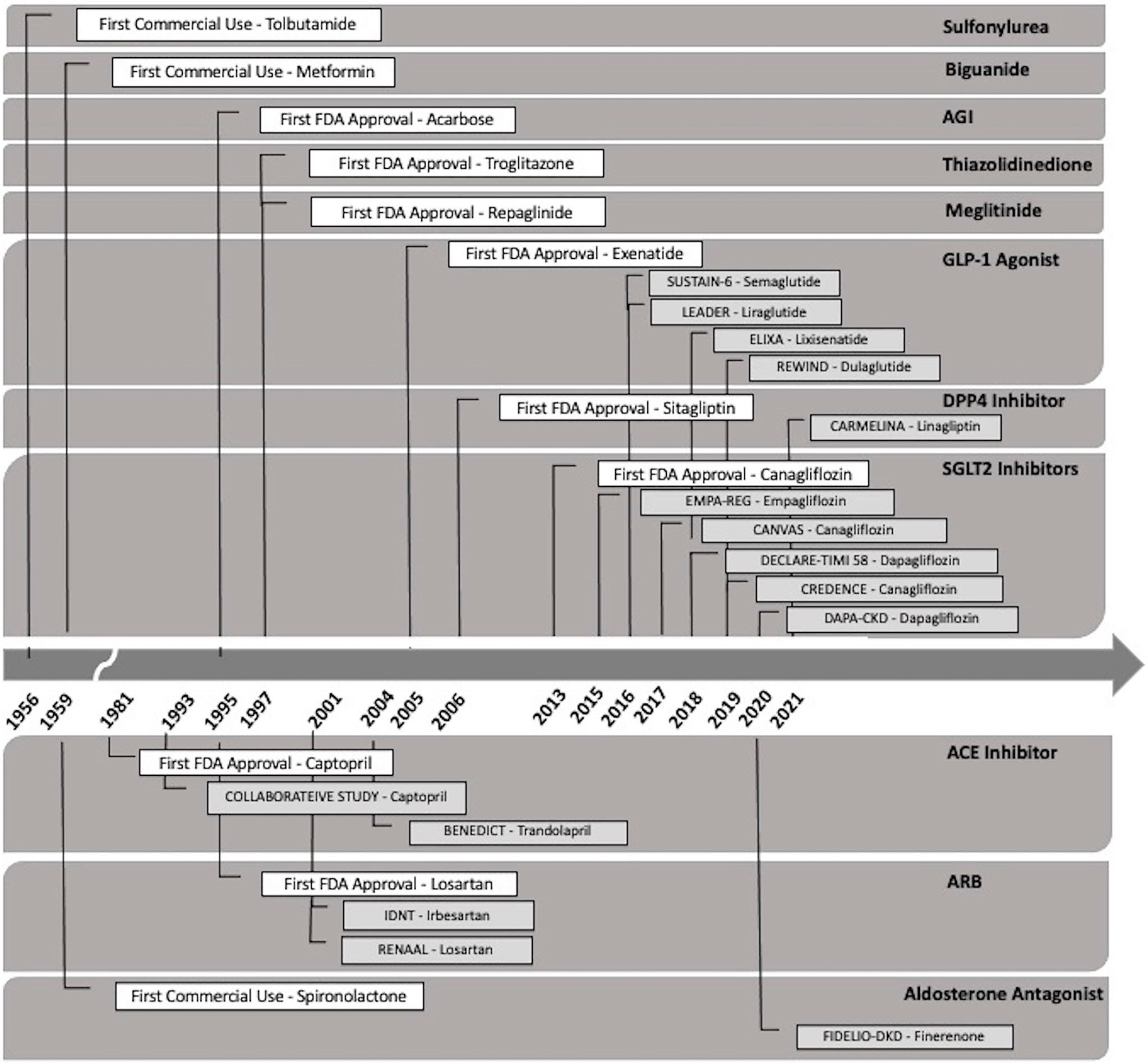
Figure 2 Timeline of the Food and Drug Administration (FDA) approval of the types of oral therapeutic options for the treatment of diabetic kidney disease. DPP4-inhibitor (dipeptidyl peptidase-4 inhibitor), GLP-1 agonist (glucagon-like peptide 1 agonist), SGLT2 inhibitor (sodium-glucose transport protein 2 inhibitor), AGI (alpha glucosidase inhibitor), ACE-inhibitor (angiotensin-converting enzyme inhibitor), and angiotensin II receptor blocker (ARB).
Diabetes Screening in Patients With Kidney Failure
The optimal HBA1c target associated with a reduction in the risk of hard clinical outcomes in kidney failure patients remains unclear. A systematic review published in 2017 showed a lack of superiority of more intensive (HbA1c < 7%) compared to liberal glycemic control (HbA1c > 7%) for the outcomes of kidney failure, death, or cardiovascular complications, whereas the Action to Control Cardiovascular Risk in Diabetes (ACCORD) post-hoc analysis showed that intensive glycemic control in high-risk patients with type 2 diabetes and mild/moderate chronic kidney disease was significantly associated with over 30% increased risk of cardiovascular and all-cause mortality (185, 186). These findings suggest that the extrapolation of general population data for HBA1c targets may not be appropriate for patients with kidney failure. Consequently, both the KDIGO and ADA guidelines have therefore advocated individualized glycemic target for patients with advanced chronic kidney disease (168). In addition, the clinicians should also be cognizant of the low reliability of HbA1c in this population (187, 188).
Prevention of Diabetic Kidney Disease
Prior to 2005, primary prevention was the main objective in the management of diabetic kidney disease with optimization of glycemic and hypertension treatment through multifactorial interventions such as lifestyle modification and initiation of pharmacological agents to recommended glycemic and blood pressure targets (189, 190). Metformin has been the first-line therapy recommended by international guidelines for patients with type 2 diabetes, but its use in patients with advanced chronic kidney disease and kidney failure is contraindicated due to the potential and real risk of lactic acidosis (168, 191). It is noteworthy that metformin may have nephroprotective effect via activity through glucagon-like peptide-1 (GLP1) receptor, improvement of sodium excretion; and reduction of tubular injury, oxidative stress, inflammation, fibrosis, and apoptosis (192). The practice of avoiding metformin in patients with advanced chronic kidney disease or those maintained on dialysis has been challenged recently with observational studies showing limited association between metformin and the risk of lactic acidosis (193–195). In a recent propensity-matched cohort study of 10,426 patients with type 2 diabetic kidney disease from South Korea, the use of metformin in advanced chronic kidney disease patients, especially those with stage 3B chronic kidney disease, decreased the risk of all-cause mortality and incident kidney failure by over 30%. An increased risk of lactic acidosis was not observed (196). Nevertheless, large randomized controlled trials (RCTs) are required to further evaluate the real-world safety, tolerability, and efficacy of metformin in the treatment of patients with diabetes and advanced chronic kidney disease.
Insulin therapy has been regarded as the default treatment for patients with type 2 diabetes and advanced chronic kidney disease, but weight gain is a relatively common side effect of treatment. Similar to the restricted use of metformin in patients with chronic kidney disease, use of sulfonylureas and meglitinides in patients with advanced chronic kidney disease is often complicated by hypoglycemia, with the use of thiazolidinediones frequently associated with an increased risk of fluid retention and congestive heart failure (197). Although these hypoglycemic agents have consistently shown to improve glycemic control with reduction in HbA1c, none of the traditional agents exhibited noticeable nephroprotective effects in patients with established diabetic kidney disease (168, 198).
Medical Therapy of Diabetic Kidney Disease
Previously, the only recommended secondary prevention strategy in patients with diabetes and hypertension or albuminuria to retard the progression of kidney disease was angiotensin-converting enzyme inhibitors or angiotensin receptor blockers (ARBs) (191). In 1993, the collaborative study group showed that captopril was associated with a 50% risk reduction of the composite endpoints of death, dialysis, and transplant in patients with insulin-dependent diabetes (181). However, management of diabetes and associated complications have changed markedly since. The Reduction of Endpoints in Non-Insulin-Dependent Diabetes Mellitus with the Angiotensin II Antagonist Losartan (RENAAL) and The Irbesartan Diabetic Nephropathy Trial (IDNT) trials in 2001 showed that ARBs were associated with over 20% risk reduction of doubling of serum creatinine or end-stage kidney disease, independent of the blood pressure-lowering effect (182, 183), but the residual risk remains high between 6 and 8 per 100 patient-years (199).
GLP-1 agonists and dipeptidyl peptidase 4 (DPP4) inhibitors were developed in the mid-2000s. Systematic reviews have shown that GLP-1 agonists improved cardiovascular outcomes with reduction in MACE (HR 0.88, 95% CI 0.82–0.94) and all-cause mortality (0.88, 0.83–0.95) (117), whereas DPP4 studies have failed to show cardiovascular benefits (116, 200). Even though both GLP-1 agonists and DPP4 inhibitors have exhibited minor nephroprotective effects (reduction of microalbuminuria), the impact of hard renal outcomes of delaying or preventing the development of kidney failure remains questionable [The Liraglutide Effect and Action in Diabetes: Evaluation of Cardiovascular Outcome Results (LEADER) and The Cardiovascular and Renal Microvascular Outcome Study With Linagliptin (CARMELINA)] (116, 174).
Novel Agents
Since 2013, SGLT2 inhibitors have been used in patients with diabetes with early trials establishing the potential cardio- and nephroprotective benefits of these drugs. In 2019, the Canagliflozin and Renal Events in Diabetes and Nephropathy Clinical Evaluation (CREDENCE) trial showed that the exposure to canagliflozin reduced the risk of the primary composite endpoint of end-stage kidney disease, doubling of serum creatinine level, and death from renal or cardiovascular causes by over 30% compared to placebo (180). This landmark study was soon followed by the Dapagliflozin and Prevention of Adverse Outcomes in Chronic Kidney Disease (DAPA-CKD) trial, which showed that the use of dapagliflozin reduced the risk of the composite sustained ≥50% decline in the estimated glomerular filtration rate (eGFR), progression to end-stage kidney disease, or death from renal or cardiovascular causes by almost 40% in patients with eGFR between 25 and 75 ml/min/1.73 m2, independent of diabetes status (119, 201). An Australian population prediction model suggested that SGLT2 inhibitors will effectively reduce the incidence of diabetes-related kidney failure in patients with type 2 diabetes by 12%–21% between 2020 and 2040. Nevertheless, the overall incidence rate was projected to trend upwards by 2040 (202). Despite the trial evidence of cardio- and nephroprotective effects of SGLT2 inhibitors, these agents are contraindicated in dialysis patients due to pharmacokinetic dependence of glomerular filtration of glucose for drug efficacy (203).
Several novel agents (aliskiren, bardoxolone, paricalcitol, and sulodexide) were assessed for the potential nephroprotective effect in patients with diabetic kidney disease, but these trials have been disappointing (204–207). Studies of newer agents (atrasentan, baricitinib, ruboxistaurin, pentoxifylline, and finerenone) are promising, with an apparent beneficial effect in reducing microalbuminuria and progression of kidney disease in patients with diabetes (Table 2) (118, 122–124, 126, 208, 209). However, further drug efficacy and safety studies are required before these newer agents are considered for clinical practice in patients with diabetic kidney disease.
Management of Kidney Transplant Recipients With PTDM
The optimal management strategies for the prevention or treatment PTDM remain unknown, with clinical practice guidelines generally informed by non-trial observational data (210). There is currently no trial evidence to support the use of pharmacological therapy to delay or prevent the development of PTDM in kidney transplant recipients, although a small proof-of-concept trial of 50 kidney transplant recipients without diabetes showed that the prescription of basal insulin in the immediate post-transplant period may reduce the incidence of PTDM, possibly by insulin-mediated protection of beta cells (127). Even though immunosuppressive agents have been shown to predispose to the development PTDM, it remains unclear whether the modification of immunosuppressive regimen (e.g., changing from tacrolimus to alternate agents; avoidance, minimization, or split dosing of corticosteroids) can reduce diabetogenic effect or consistently reverse the presence of established PTDM without resulting in a greater risk of other adverse allograft outcomes such as rejection and allograft loss (211–217). The benefit of lifestyle intervention (for weight reduction) or the use of “preventive” pharmacologic treatments including metformin, DPP4-inhibitor, and thiazolidinediones to reduce the risk of progression from a pre-diabetic state to type 2 diabetes in the general population has not been shown for kidney transplant recipients, but these approaches are currently being evaluated in this population group (218–223).
The approach to the treatment of kidney transplant recipients with PTDM is predictably extrapolated from the general population, with little clinical evidence to support the preferential use of specific oral hypoglycemic agents. The optimal HbA1c in kidney transplant recipients with PTDM is unclear, with the 2009 KDIGO guideline suggesting a target HbA1c of 7.0%–7.5% for recipients with PTDM (ungraded evidence) (66). However, the updated 2020 KDIGO diabetes management guideline recommended individualized HbA1c target ranging from less than 6.5% to less than 8.0% in patients with diabetes and chronic kidney disease not treated with dialysis (level 1C evidence), with this recommendation generalizable to kidney failure patients treated with kidney transplants (168). Given that the predominant underlying mechanisms resulting in PTDM are insulin resistance and/or diminished insulin secretion, the use of oral hypoglycemic agents known to target these pathways may be preferable. A systematic review of seven RCTs, quasi-RCTs, and crossover studies published in 2017 (n = 399 kidney transplant recipients with pre-transplant diabetes or PTDM) concluded that the studies were of poor quality and exhibited a high risk of bias, and the findings were unable to inform the superiority of any treatment options (128). Only three studies using DPP4-inhibitor (vildagliptin or sitagliptin vs. placebo or insulin) were undertaken in recipients with PTDM (n = 115), with no demonstrable benefit in improving glycemic control, allograft function, or outcome with DPP4-inhibitor (224–227). Table 4 summarizes the recent literature (case reports, series, and trials) of the use of novel oral hypoglycemic agents in recipients with PTDM. In the limited number of studies of interventions in kidney transplant recipients with PTDM, the efficacy of thiazolidinediones (total of four studies, n = 67) (228–230), repaglinide (one study, n = 23) (239), GLP1 agonist (four studies in 187 solid organ transplant recipients) (235–238), DPP4-inhibitor (five studies [1 with IGT only], n = 110) (224, 225, 231–233), and SGLT2 inhibitor (seven studies, n = 92) (227, 240–246) appears to be limited, confounded by the presence of a small number of RCTs and recipients and the difficulty in differentiating the response to intervention of recipients with pre-transplant diabetes compared to those with PTDM. Nevertheless, the limited data do give insight into the safety and tolerability of these novel oral hypoglycemic agents, and the observed lack of impact on the therapeutic drug levels of immunosuppressive agents such as calcineurin-inhibitors is reassuring in the ongoing utility and research of these agents in the treatment of PTDM. A proposed treatment algorithm for the prevention and treatment of PTDM is shown in Figure 3.
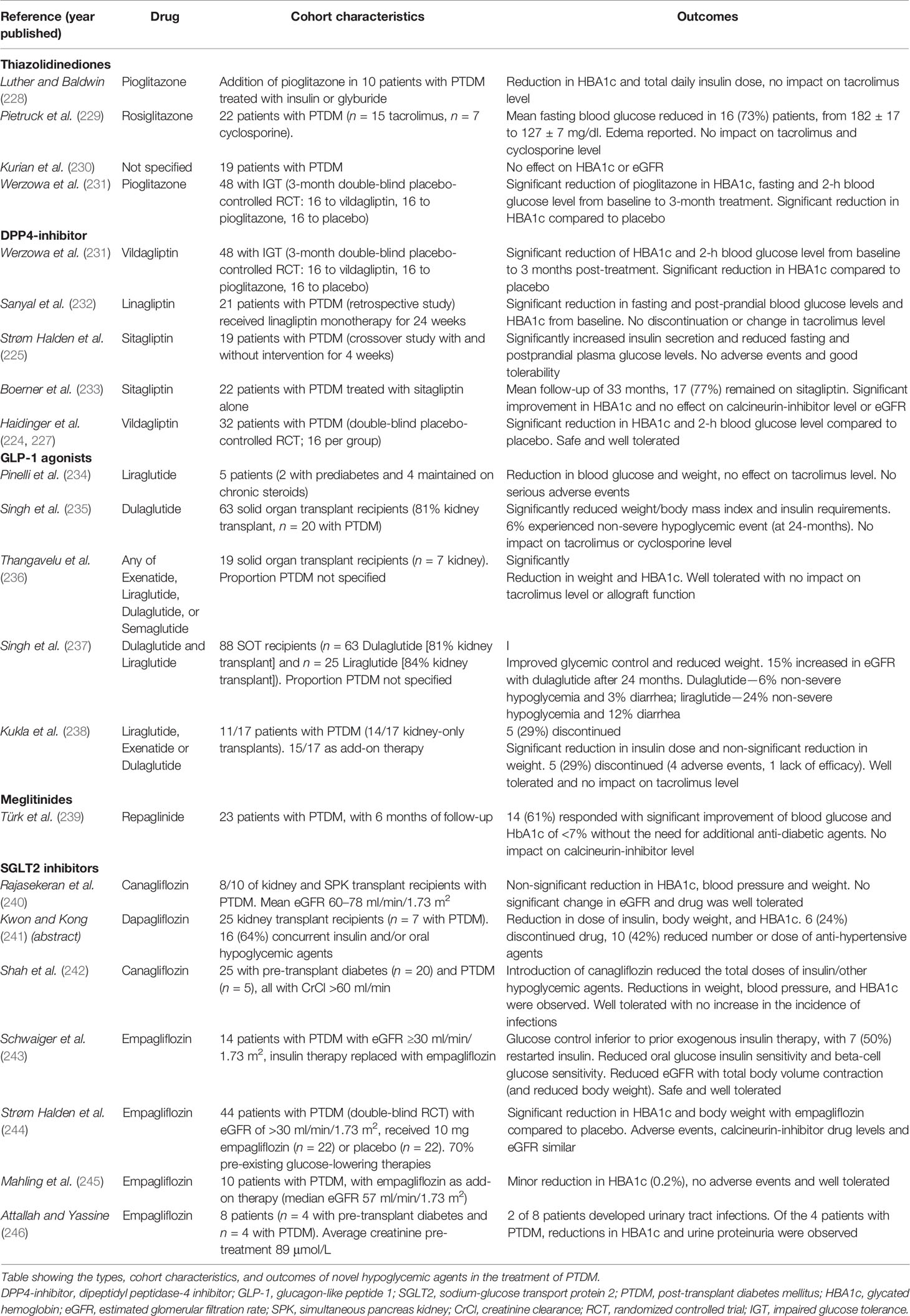
Table 4 Reports of the utilization of novel oral hypoglycemic agents in kidney transplant recipients with post-transplant diabetes mellitus.
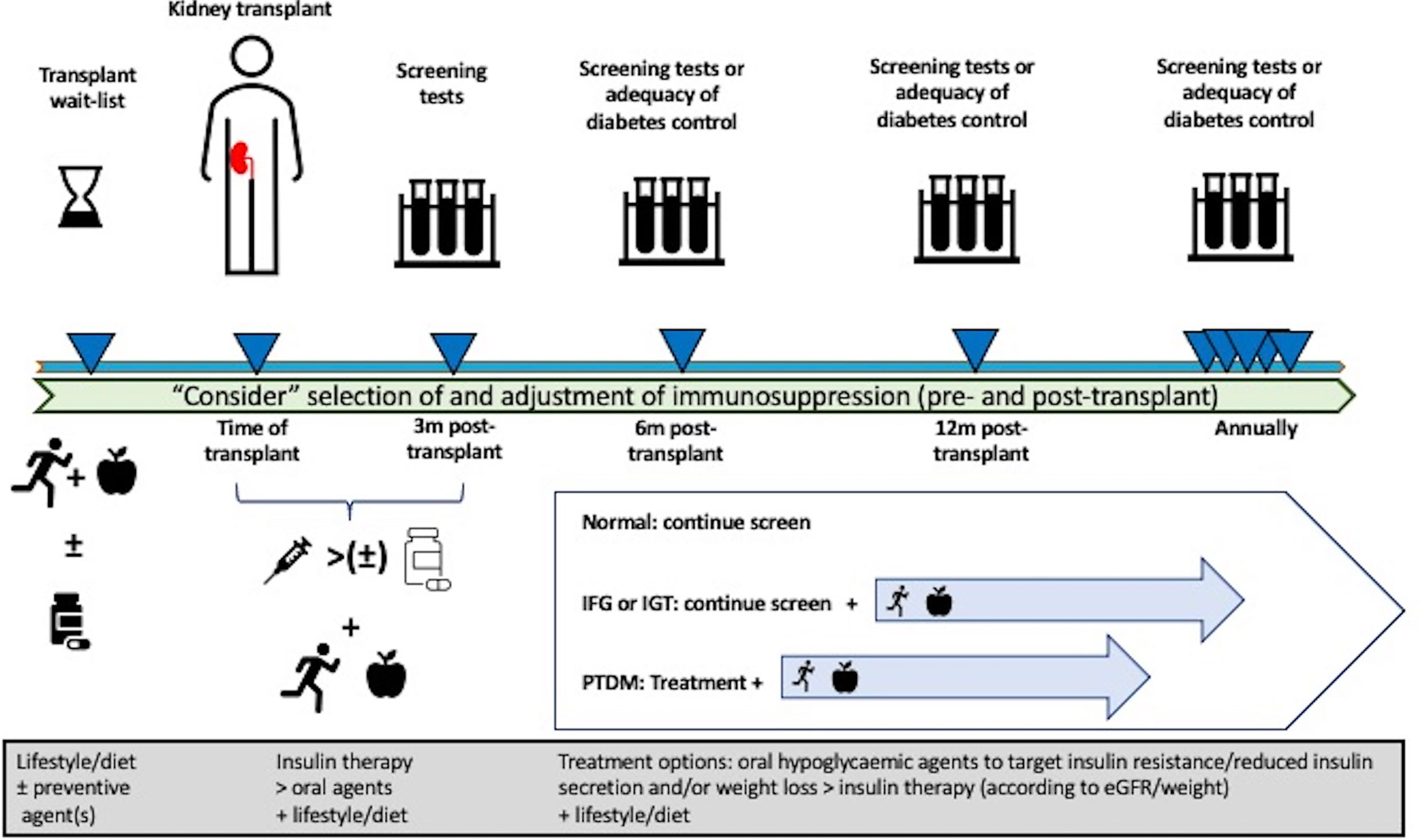
Figure 3 Proposed management algorithm pre- and post-transplantation in the prevention and treatment of post-transplant diabetes mellitus (PTDM) in kidney transplant recipients. Pre-transplant lifestyle intervention and/or preventive pharmacological therapy can be considered to reduce the risk of developing PTDM, but these approaches have not been established for potential kidney transplant candidates. Although the selection and modification of immunosuppression drugs can be considered, no specific immunosuppressive regimen(s) have been shown to prevent the risk of developing PTDM. Following kidney transplantation, consideration of early insulin therapy post-transplant, frequent monitoring for abnormal glucose regulation, lifestyle intervention, and aggressive management of metabolic complications should be undertaken. In kidney transplant recipients with PTDM, the initial treatment with appropriate oral hypoglycemic agents known to improve insulin sensitivity and secretion should be preferred over other hypoglycemic agents and/or insulin therapy.
Future Directions
Given the recent trial evidence on the efficacy of novel oral hypoglycemic agents in the treatment of type 2 diabetes in the general population, there is a considerable knowledge gap regarding the safety, tolerability, and efficacy of these newer agents in patients with advanced chronic kidney disease, including those maintained on dialysis or have received kidney transplants. A global collaborative effort composed of epidemiologists, clinicians, trialists, and basic science researchers is required to collect retrospective and prospective data reporting on the use of these and other novel agents in patients with kidney failure, in order to enhance our understanding of the similarities and differences in the pathogenesis of diabetes and related complications in those with kidney failure, identify and validate potential predictive biomarkers to guide evidence-based treatments, and coordinate adequately powered clinical trials to address the knowledge deficiency in the optimal treatment of patients with kidney failure and type 2 diabetes and those with PTDM.
Conclusion
Our understanding of the incidence and prevalence of type 2 diabetes and related complications among patients with treated kidney failure has significantly advanced with the availability and analysis of big data repositories that showed that diabetic nephropathy has become one of the dominant causes of treated kidney failure worldwide. Data from multiple major linkage projects have shown that treated kidney failure patients with type 2 diabetes have a survival disadvantage compared to those without diabetes, but the magnitude of these risks remains inconsistent. Even though important progress has been made in the understanding of the mechanistic insights into the pathogenesis and ensuing novel treatments to impede kidney disease progression and reduce the burden of vascular complications attributed to diabetes in the last decade, a similar understanding of these novel treatment strategies in patients with diabetes and kidney failure maintained on dialysis or having received kidney transplants remains an elusive goal. Future global collaborative efforts are urgently required to accurately map the disease incidence and prevalence, collating precise age-, gender-, and race-specific data to inform the risk of diabetes-related complications in patients with kidney failure and to provide evidence-based recommendations in the optimal treatment strategies for these patients, including those who have developed PTDM. These data will then inform the development of a global action plan to counteract diabetic kidney disease and provide meaningful knowledge to improve outcomes for the thousands of people with treated kidney failure and diabetes.
Author Contributions
All authors listed have made a substantial, direct, and intellectual contribution to the work and approved it for publication.
Conflict of Interest
The authors declare that the research was conducted in the absence of any commercial or financial relationships that could be construed as a potential conflict of interest.
Publisher’s Note
All claims expressed in this article are solely those of the authors and do not necessarily represent those of their affiliated organizations, or those of the publisher, the editors and the reviewers. Any product that may be evaluated in this article, or claim that may be made by its manufacturer, is not guaranteed or endorsed by the publisher.
References
1. American Diabetes Association. Economic Costs of Diabetes in the U.S. in 2017. Diabetes Care (2018) 41(5):917–28. doi: 10.2337/dci18-0007
2. McDonald S, Chang S, Excell L. New Patients Commencing Treatment in 2005. Australia and New Zealand Dialysis and Transplant Registry Annual Report. Adelaide, Australia: ANZDATA Registry (2006).
3. Australia and New Zealand Dialysis and Transplant Registry Annual Report. Chapter 1: Incidence of Renal Replacement Therapy for End Stage Kidney Disease. Adelaide, Australia: ANZDATA Registry (2020).
4. United States Renal Data System. 2019 Annual Data Report: Epidemiology of Kidney Disease in the United States. Bethesda, MD: National Institutes of Health, National Institute of Diabetes and Digestive and Kidney Diseases (2019).
5. United States Renal Data System. 2014 USRDS Annual Data Report Volume 2: End-Stage Renal Disease. Bethesda, MD: National Institutes of Health, National Institute of Diabetes and Digestive and Kidney Diseases (2014).
6. United Kingdom Renal Registry. UK Renal Registry 22nd Annual Report. Chapter 1: Adults Starting Renal Replacement Therapy (RRT) for End-Stage Kidney Disease (ESKD) in the UK in 2018. Bristol, United Kingdom (2018).
7. United Kingdom Renal Registry. UK Renal Registry 9th Annual Report. Chapter 3: New Adult Patients Starting Renal Replacement Therapy in the UK in 2005. Bristol, United Kingdom (2005).
8. GBD Chronic Kidney Disease Collaboration. Global, Regional, and National Burden of Chronic Kidney Disease, 1990-2017: A Systematic Analysis for the Global Burden of Disease Study 2017. Lancet (2020) 395(10225):709–33. doi: 10.1016/S0140-6736(19)32977-0
9. Xu G, You D, Wong L, Duan D, Kong F, Zhang X, et al. Risk of All-Cause and CHD Mortality in Women Versus Men With Type 2 Diabetes: A Systematic Review and Meta-Analysis. Eur J Endocrinol (2019) 180(4):243–55. doi: 10.1530/EJE-18-0792
10. Nwaneri C, Bowen-Jones D, Cooper H. Screening for Type 2 Diabetes and Population Mortality Over 10 Years. Lancet (2013) 381(9870):901–2. doi: 10.1016/S0140-6736(13)60665-0
11. Nanayakkara N, Curtis AJ, Heritier S, Gadowski AM, Pavkov ME, Kenealy T, et al. Impact of Age at Type 2 Diabetes Mellitus Diagnosis on Mortality and Vascular Complications: Systematic Review and Meta-Analyses. Diabetologia (2021) 64(2):275–87. doi: 10.1007/s00125-020-05319-w
12. Lim WH, Johnson DW, Hawley C, Lok C, Polkinghorne KR, Roberts MA, et al. Type 2 Diabetes in Patients With End-Stage Kidney Disease: Influence on Cardiovascular Disease-Related Mortality Risk. Med J Aust (2018) 209(10):440–6. doi: 10.5694/mja18.00195
13. Lim WH, Wong G, Pilmore HL, McDonald SP, Chadban SJ. Long-Term Outcomes of Kidney Transplantation in People With Type 2 Diabetes: A Population Cohort Study. Lancet Diabetes Endocrinol (2017) 5(1):26–33. doi: 10.1016/S2213-8587(16)30317-5
14. Vallon V, Komers R. Pathophysiology of the Diabetic Kidney. Compr Physiol (2011) 1(3):1175–232. doi: 10.1002/cphy.c100049
15. Nelson RG, Tuttle KR. The New KDOQI Clinical Practice Guidelines and Clinical Practice Recommendations for Diabetes and CKD. Blood Purif (2007) 25(1):112–4. doi: 10.1159/000096407
16. Umanath K, Lewis JB. Update on Diabetic Nephropathy: Core Curriculum 2018. Am J Kidney Dis (2018) 71(6):884–95. doi: 10.1053/j.ajkd.2017.10.026
17. Umanath K, Lewis JB. Diabetic Kidney Disease: The Tiger May Have New Stripes. Am J Kidney Dis (2018) 72(5):631–3. doi: 10.1053/j.ajkd.2018.06.033
18. Fiorentino M, Bolignano D, Tesar V, Pisano A, Van Biesen W, D'Arrigo G, et al. Renal Biopsy in 2015–From Epidemiology to Evidence-Based Indications. Am J Nephrol (2016) 43(1):1–19. doi: 10.1159/000444026
19. Cunningham A, Benediktsson H, Muruve DA, Hildebrand AM, Ravani P. Trends in Biopsy-Based Diagnosis of Kidney Disease: A Population Study. Can J Kidney Health Dis (2018) 5:2054358118799690. doi: 10.1177/2054358118799690
20. Tong X, Yu Q, Ankawi G, Pang B, Yang B, Yang H. Insights Into the Role of Renal Biopsy in Patients With T2DM: A Literature Review of Global Renal Biopsy Results. Diabetes Ther (2020) 11(9):1983–99. doi: 10.1007/s13300-020-00888-w
21. Fiorentino M, Bolignano D, Tesar V, Pisano A, Biesen WV, Tripepi G, et al. Renal Biopsy in Patients With Diabetes: A Pooled Meta-Analysis of 48 Studies. Nephrol Dial Transplant (2017) 32(1):97–110. doi: 10.1093/ndt/gfw070
22. Sharma SG, Bomback AS, Radhakrishnan J, Herlitz LC, Stokes MB, Markowitz GS, et al. The Modern Spectrum of Renal Biopsy Findings in Patients With Diabetes. Clin J Am Soc Nephrol (2013) 8(10):1718–24. doi: 10.2215/CJN.02510213
23. Zhuo L, Ren W, Li W, Zou G, Lu J. Evaluation of Renal Biopsies in Type 2 Diabetic Patients With Kidney Disease: A Clinicopathological Study of 216 Cases. Int Urol Nephrol (2013) 45(1):173–9. doi: 10.1007/s11255-012-0164-6
24. Wong TY, Choi PC, Szeto CC, To KF, Tang NL, Chan AW, et al. Renal Outcome in Type 2 Diabetic Patients With or Without Coexisting Nondiabetic Nephropathies. Diabetes Care (2002) 25(5):900–5. doi: 10.2337/diacare.25.5.900
25. He F, Xia X, Wu XF, Yu XQ, Huang FX. Diabetic Retinopathy in Predicting Diabetic Nephropathy in Patients With Type 2 Diabetes and Renal Disease: A Meta-Analysis. Diabetologia (2013) 56(3):457–66. doi: 10.1007/s00125-012-2796-6
26. Mazzucco G, Bertani T, Fortunato M, Bernardi M, Leutner M, Boldorini R, et al. Different Patterns of Renal Damage in Type 2 Diabetes Mellitus: A Multicentric Study on 393 Biopsies. Am J Kidney Dis (2002) 39(4):713–20. doi: 10.1053/ajkd.2002.31988
27. Kanwar YS, Sun L, Xie P, Liu FY, Chen S. A Glimpse of Various Pathogenetic Mechanisms of Diabetic Nephropathy. Annu Rev Pathol (2011) 6:395–423. doi: 10.1146/annurev.pathol.4.110807.092150
28. Papale M, Di Paolo S, Magistroni R, Lamacchia O, Di Palma AM, De Mattia A, et al. Urine Proteome Analysis may Allow Noninvasive Differential Diagnosis of Diabetic Nephropathy. Diabetes Care (2010) 33(11):2409–15. doi: 10.2337/dc10-0345
29. Gonzalez Suarez ML, Thomas DB, Barisoni L, Fornoni A. Diabetic Nephropathy: Is It Time Yet for Routine Kidney Biopsy? World J Diabetes (2013) 4(6):245–55. doi: 10.4239/wjd.v4.i6.245
30. United States Renal Data System. 2020 USRDS Annual Data Report: Epidemiology of Kidney Disease in the United States. Bethesda, MD, United States: National Institutes of Health, National Institute of Diabetes and Digestive and Kidney Diseases (2020).
31. United States Renal Data System. 2020 USRDS Annual Data Report: End Stage Renal Disease: Chapter 11 International Comparisons. Bethesda, MD, United States: National Institutes of Health, National Institute of Diabetes and Digestive and Kidney Diseases (2020).
32. Australia and New Zealand Dialysis and Transplant Registry Annual Report. Chapter 1: Incidence of Renal Replacement Therapy For End-Stage Kidney Disease. Adelaide, Australia: Australia and New Zealand Dialysis and Transplant Registry (2019).
33. Koye DN, Magliano DJ, Nelson RG, Pavkov ME. The Global Epidemiology of Diabetes and Kidney Disease. Adv Chronic Kidney Dis (2018) 25(2):121–32. doi: 10.1053/j.ackd.2017.10.011
34. van Diepen M, Schroijen MA, Dekkers OM, Rotmans JI, Krediet RT, Boeschoten EW, et al. Predicting Mortality in Patients With Diabetes Starting Dialysis. PloS One (2014) 9(3):e89744. doi: 10.1371/journal.pone.0089744
35. Chantrel F, Enache I, Bouiller M, Kolb I, Kunz K, Petitjean P, et al. Abysmal Prognosis of Patients With Type 2 Diabetes Entering Dialysis. Nephrol Dial Transplant (1999) 14(1):129–36. doi: 10.1093/ndt/14.1.129
36. Fox CS, Matsushita K, Woodward M, Bilo HJ, Chalmers J, Heerspink HJ, et al. Associations of Kidney Disease Measures With Mortality and End-Stage Renal Disease in Individuals With and Without Diabetes: A Meta-Analysis. Lancet (2012) 380(9854):1662–73. doi: 10.1016/S0140-6736(12)61350-6
37. Oh SW, Kim S, Na KY, Chae DW, Kim S, Jin DC, et al. Clinical Implications of Pathologic Diagnosis and Classification for Diabetic Nephropathy. Diabetes Res Clin Pract (2012) 97(3):418–24. doi: 10.1016/j.diabres.2012.03.016
38. Haneda M, Utsunomiya K, Koya D, Babazono T, Moriya T, Makino H, et al. A New Classification of Diabetic Nephropathy 2014: A Report From Joint Committee on Diabetic Nephropathy. J Diabetes Investig (2015) 6(2):242–6. doi: 10.1111/jdi.12319
39. Schroijen MA, van de Luijtgaarden MW, Noordzij M, Ravani P, Jarraya F, Collart F, et al. Survival in Dialysis Patients Is Different Between Patients With Diabetes as Primary Renal Disease and Patients With Diabetes as a Co-Morbid Condition. Diabetologia (2013) 56(9):1949–57. doi: 10.1007/s00125-013-2966-1
40. Huo L, Magliano DJ, Ranciere F, Harding JL, Nanayakkara N, Shaw JE, et al. Impact of Age at Diagnosis and Duration of Type 2 Diabetes on Mortality in Australia 1997-2011. Diabetologia (2018) 61(5):1055–63. doi: 10.1007/s00125-018-4544-z
41. Lameire N, Matthys D, Matthys E, Beheydt R. Effects of Long-Term CAPD on Carbohydrate and Lipid Metabolism. Clin Nephrol (1988) 30 Suppl 1:S53–8.
42. Liao CT, Kao TW, Chou YH, Wu MS, Chen YM, Chuang HF, et al. Associations of Metabolic Syndrome and Its Components With Cardiovascular Outcomes Among Non-Diabetic Patients Undergoing Maintenance Peritoneal Dialysis. Nephrol Dial Transplant (2011) 26(12):4047–54. doi: 10.1093/ndt/gfr175
43. Lambie M, Chess J, Do JY, Noh H, Lee HB, Kim YL, et al. Peritoneal Dialysate Glucose Load and Systemic Glucose Metabolism in Non-Diabetics: Results From the GLOBAL Fluid Cohort Study. PloS One (2016) 11(6):e0155564. doi: 10.1371/journal.pone.0155564
44. Tien KJ, Lin ZZ, Chio CC, Wang JJ, Chu CC, Sun YM, et al. Epidemiology and Mortality of New-Onset Diabetes After Dialysis: Taiwan National Cohort Study. Diabetes Care (2013) 36(10):3027–32. doi: 10.2337/dc12-2148
45. Wu PP, Kor CT, Hsieh MC, Hsieh YP. Association Between End-Stage Renal Disease and Incident Diabetes Mellitus-A Nationwide Population-Based Cohort Study. J Clin Med (2018) 7(10):343. doi: 10.3390/jcm7100343
46. Chou CY, Liang CC, Kuo HL, Chang CT, Liu JH, Lin HH, et al. Comparing Risk of New Onset Diabetes Mellitus in Chronic Kidney Disease Patients Receiving Peritoneal Dialysis and Hemodialysis Using Propensity Score Matching. PloS One (2014) 9(2):e87891. doi: 10.1371/journal.pone.0087891
47. Woodward RS, Schnitzler MA, Baty J, Lowell JA, Lopez-Rocafort L, Haider S, et al. Incidence and Cost of New Onset Diabetes Mellitus Among U.S. Wait-Listed and Transplanted Renal Allograft Recipients. Am J Transplant (2003) 3(5):590–8. doi: 10.1034/j.1600-6143.2003.00082.x
48. Szeto CC, Chow KM, Kwan BC, Chung KY, Leung CB, Li PK. New-Onset Hyperglycemia in Nondiabetic Chinese Patients Started on Peritoneal Dialysis. Am J Kidney Dis (2007) 49(4):524–32. doi: 10.1053/j.ajkd.2007.01.018
49. Wolfe RA, Ashby VB, Milford EL, Ojo AO, Ettenger RE, Agodoa LY, et al. Comparison of Mortality in All Patients on Dialysis, Patients on Dialysis Awaiting Transplantation, and Recipients of a First Cadaveric Transplant. N Engl J Med (1999) 341(23):1725–30. doi: 10.1056/NEJM199912023412303
50. Wong G, Howard K, Chapman JR, Chadban S, Cross N, Tong A, et al. Comparative Survival and Economic Benefits of Deceased Donor Kidney Transplantation and Dialysis in People With Varying Ages and Co-Morbidities. PloS One (2012) 7(1):e29591. doi: 10.1371/journal.pone.0029591
51. Quinn RR, Naimark DM, Oliver MJ, Bayoumi AM. Should Hemodialysis Patients With Atrial Fibrillation Undergo Systemic Anticoagulation? A Cost-Utility Analysis. Am J Kidney Dis (2007) 50(3):421–32. doi: 10.1053/j.ajkd.2007.05.019
52. Keddis MT, El Ters M, Rodrigo E, Dean P, Wohlfahrtova M, Kudva YC, et al. Enhanced Posttransplant Management of Patients With Diabetes Improves Patient Outcomes. Kidney Int (2014) 86(3):610–8. doi: 10.1038/ki.2014.70
53. Faerch K, Carstensen B, Almdal TP, Jorgensen ME. Improved Survival Among Patients With Complicated Type 2 Diabetes in Denmark: A Prospective Study (2002-2010). J Clin Endocrinol Metab (2014) 99(4):E642–6. doi: 10.1210/jc.2013-3210
54. Gregg EW, Cheng YJ, Saydah S, Cowie C, Garfield S, Geiss L, et al. Trends in Death Rates Among U.S. Adults With and Without Diabetes Between 1997 and 2006: Findings From the National Health Interview Survey. Diabetes Care (2012) 35(6):1252–7. doi: 10.2337/dc11-1162
55. Vincenti F, Friman S, Scheuermann E, Rostaing L, Jenssen T, Campistol JM, et al. Results of an International, Randomized Trial Comparing Glucose Metabolism Disorders and Outcome With Cyclosporine Versus Tacrolimus. Am J Transplant (2007) 7(6):1506–14. doi: 10.1111/j.1600-6143.2007.01749.x
56. Kasiske BL, Snyder JJ, Gilbertson D, Matas AJ. Diabetes Mellitus After Kidney Transplantation in the United States. Am J Transplant (2003) 3(2):178–85. doi: 10.1034/j.1600-6143.2003.00010.x
57. Hjelmesaeth J, Hartmann A, Kofstad J, Egeland T, Stenstrom J, Fauchald P. Tapering Off Prednisolone and Cyclosporin the First Year After Renal Transplantation: The Effect on Glucose Tolerance. Nephrol Dial Transplant (2001) 16(4):829–35. doi: 10.1093/ndt/16.4.829
58. Montori VM, Basu A, Erwin PJ, Velosa JA, Gabriel SE, Kudva YC. Posttransplantation Diabetes: A Systematic Review of the Literature. Diabetes Care (2002) 25(3):583–92. doi: 10.2337/diacare.25.3.583
59. Cosio FG, Pesavento TE, Osei K, Henry ML, Ferguson RM. Post-Transplant Diabetes Mellitus: Increasing Incidence in Renal Allograft Recipients Transplanted in Recent Years. Kidney Int (2001) 59(2):732–7. doi: 10.1046/j.1523-1755.2001.059002732.x
60. Armstrong KA, Prins JB, Beller EM, Campbell SB, Hawley CM, Johnson DW, et al. Should an Oral Glucose Tolerance Test Be Performed Routinely in All Renal Transplant Recipients? Clin J Am Soc Nephrol (2006) 1(1):100–8. doi: 10.2215/CJN.00090605
61. Friedman EA, Shyh TP, Beyer MM, Manis T, Butt KM. Posttransplant Diabetes in Kidney Transplant Recipients. Am J Nephrol (1985) 5(3):196–202. doi: 10.1159/000166932
62. Nathan DM, Davidson MB, DeFronzo RA, Heine RJ, Henry RR, Pratley R, et al. Impaired Fasting Glucose and Impaired Glucose Tolerance: Implications for Care. Diabetes Care (2007) 30(3):753–9. doi: 10.2337/dc07-9920
63. Viecelli A, Nguyen HT, Yong K, Chan D, Dogra G, Wong G, et al. The Impact of Abnormal Glucose Regulation on Arterial Stiffness at 3 and 15 Months After Kidney Transplantation. Diabetol Metab Syndr (2014) 6(1):52. doi: 10.1186/1758-5996-6-52
64. Guthoff M, Wagner R, Weichbrodt K, Nadalin S, Konigsrainer A, Haring HU, et al. Dynamics of Glucose Metabolism After Kidney Transplantation. Kidney Blood Press Res (2017) 42(3):598–607. doi: 10.1159/000481375
65. Porrini EL, Diaz JM, Moreso F, Delgado Mallen PI, Silva Torres I, Ibernon M, et al. Clinical Evolution of Post-Transplant Diabetes Mellitus. Nephrol Dial Transplant (2016) 31(3):495–505. doi: 10.1093/ndt/gfv368
66. Kasiske BL, Zeier MG, Chapman JR, Craig JC, Ekberg H, Garvey CA, et al. KDIGO Clinical Practice Guideline for the Care of Kidney Transplant Recipients: A Summary. Kidney Int (2010) 77(4):299–311. doi: 10.1038/ki.2009.377
67. Chowdhury T, Wahba M, Mallik R, Peracha J, Patel D, De P, et al. Association of British Clinical Diabetologist and Renal Association Guidelines on the Detection and Management of Diabetes Post Solid Organ Transplantation. Diabet Med (2021) 38(6):e14523. doi: 10.1111/dme.14523
68. American Diabetes Association. Classification and Diagnosis of Diabetes: Standards of Medical Care in Diabetes-2021. Diabetes Care (2021) 44(Suppl 1):S15–33. doi: 10.2337/dc21-S002
69. Aouad LJ, Clayton P, Wyburn KR, Gracey DM, Chadban SJ. Evolution of Glycemic Control and Variability After Kidney Transplant. Transplantation (2018) 102(9):1563–8. doi: 10.1097/TP.0000000000002155
70. Scott IA, Greenberg PB, Poole PJ. Cautionary Tales in the Clinical Interpretation of Studies of Diagnostic Tests. Intern Med J (2008) 38(2):120–9. doi: 10.1111/j.1445-5994.2007.01436.x
71. Montori VM, Wyer P, Newman TB, Keitz S, Guyatt G. Evidence-Based Medicine Teaching Tips Working G. Tips for Learners of Evidence-Based Medicine: 5. The Effect of Spectrum of Disease on the Performance of Diagnostic Tests. CMAJ (2005) 173(4):385–90. doi: 10.1503/cmaj.1031666
72. Rosettenstein K, Viecelli A, Yong K, Nguyen HD, Chakera A, Chan D, et al. Diagnostic Accuracies of Glycated Hemoglobin, Fructosamine, and Homeostasis Model Assessment of Insulin Resistance in Predicting Impaired Fasting Glucose, Impaired Glucose Tolerance, or New Onset Diabetes After Transplantation. Transplantation (2016) 100(7):1571–9. doi: 10.1097/TP.0000000000000949
73. Wojtusciszyn A, Mourad G, Bringer J, Renard E. Continuous Glucose Monitoring After Kidney Transplantation in Non-Diabetic Patients: Early Hyperglycaemia Is Frequent and may Herald Post-Transplantation Diabetes Mellitus and Graft Failure. Diabetes Metab (2013) 39(5):404–10. doi: 10.1016/j.diabet.2012.10.007
74. Ussif AM, Asberg A, Halden TAS, Nordheim E, Hartmann A, Jenssen T. Validation of Diagnostic Utility of Fasting Plasma Glucose and HbA1c in Stable Renal Transplant Recipients One Year After Transplantation. BMC Nephrol (2019) 20(1):12. doi: 10.1186/s12882-018-1171-3
75. Dong M, Parsaik AK, Eberhardt NL, Basu A, Cosio FG, Kudva YC. Cellular and Physiological Mechanisms of New-Onset Diabetes Mellitus After Solid Organ Transplantation. Diabetes Med (2012) 29(7):e1–12. doi: 10.1111/j.1464-5491.2012.03617.x
76. Yang SB, Lee HY, Young DM, Tien AC, Rowson-Baldwin A, Shu YY, et al. Rapamycin Induces Glucose Intolerance in Mice by Reducing Islet Mass, Insulin Content, and Insulin Sensitivity. J Mol Med (Berl) (2012) 90(5):575–85. doi: 10.1007/s00109-011-0834-3
77. Soleimanpour SA, Crutchlow MF, Ferrari AM, Raum JC, Groff DN, Rankin MM, et al. Calcineurin Signaling Regulates Human Islet {Beta}-Cell Survival. J Biol Chem (2010) 285(51):40050–9. doi: 10.1074/jbc.M110.154955
78. Johnston O, Rose CL, Webster AC, Gill JS. Sirolimus Is Associated With New-Onset Diabetes in Kidney Transplant Recipients. J Am Soc Nephrol (2008) 19(7):1411–8. doi: 10.1681/ASN.2007111202
79. Bugliani M, Masini M, Liechti R, Marselli L, Xenarios I, Boggi U, et al. The Direct Effects of Tacrolimus and Cyclosporin A on Isolated Human Islets: A Functional, Survival and Gene Expression Study. Islets (2009) 1(2):106–10. doi: 10.4161/isl.1.2.9142
80. Dai C, Walker JT, Shostak A, Padgett A, Spears E, Wisniewski S, et al. Tacrolimus- and Sirolimus-Induced Human Beta Cell Dysfunction Is Reversible and Preventable. JCI Insight (2020) 5(1):1–12. doi: 10.1172/jci.insight.130770
81. Ekberg H, Bernasconi C, Noldeke J, Yussim A, Mjornstedt L, Erken U, et al. Cyclosporine, Tacrolimus and Sirolimus Retain Their Distinct Toxicity Profiles Despite Low Doses in the Symphony Study. Nephrol Dial Transplant (2010) 25(6):2004–10. doi: 10.1093/ndt/gfp778
82. Ekberg H, Tedesco-Silva H, Demirbas A, Vitko S, Nashan B, Gurkan A, et al. Reduced Exposure to Calcineurin Inhibitors in Renal Transplantation. N Engl J Med (2007) 357(25):2562–75. doi: 10.1056/NEJMoa067411
83. Einollahi B, Motalebi M, Salesi M, Ebrahimi M, Taghipour M. The Impact of Cytomegalovirus Infection on New-Onset Diabetes Mellitus After Kidney Transplantation: A Review on Current Findings. J Nephropathol (2014) 3(4):139–48. doi: 10.12860/jnp.2014.27
84. Fabrizi F, Messa P, Martin P, Takkouche B. Hepatitis C Virus Infection and Post-Transplant Diabetes Mellitus Among Renal Transplant Patients: A Meta-Analysis. Int J Artif Organs (2008) 31(8):675–82. doi: 10.1177/039139880803100801
85. Fabrizi F, Lampertico P, Lunghi G, Mangano S, Aucella F, Martin P. Review Article: Hepatitis C Virus Infection and Type-2 Diabetes Mellitus in Renal Diseases and Transplantation. Aliment Pharmacol Ther (2005) 21(6):623–32. doi: 10.1111/j.1365-2036.2005.02389.x
86. Hjelmesaeth J, Muller F, Jenssen T, Rollag H, Sagedal S, Hartmann A. Is There a Link Between Cytomegalovirus Infection and New-Onset Posttransplantation Diabetes Mellitus? Potential Mechanisms of Virus Induced Beta-Cell Damage. Nephrol Dial Transplant (2005) 20(11):2311–5. doi: 10.1093/ndt/gfi033
87. Jaeckel E, Manns M, Von Herrath M. Viruses and Diabetes. Ann N Y Acad Sci (2002) 958:7–25. doi: 10.1111/j.1749-6632.2002.tb02943.x
88. Lohr JM, Oldstone MB. Detection of Cytomegalovirus Nucleic Acid Sequences in Pancreas in Type 2 Diabetes. Lancet (1990) 336(8716):644–8. doi: 10.1016/0140-6736(90)92145-8
89. Butler AE, Janson J, Bonner-Weir S, Ritzel R, Rizza RA, Butler PC. Beta-Cell Deficit and Increased Beta-Cell Apoptosis in Humans With Type 2 Diabetes. Diabetes (2003) 52(1):102–10. doi: 10.2337/diabetes.52.1.102
90. Culliford A, Phagura N, Sharif A. Autosomal Dominant Polycystic Kidney Disease Is a Risk Factor for Posttransplantation Diabetes Mellitus: An Updated Systematic Review and Meta-Analysis. Transplant Direct (2020) 6(5):e553. doi: 10.1097/TXD.0000000000000989
91. Cheungpasitporn W, Thongprayoon C, Vijayvargiya P, Anthanont P, Erickson SB. The Risk for New-Onset Diabetes Mellitus After Kidney Transplantation in Patients With Autosomal Dominant Polycystic Kidney Disease: A Systematic Review and Meta-Analysis. Can J Diabetes (2016) 40(6):521–8. doi: 10.1016/j.jcjd.2016.03.001
92. Ruderman I, Masterson R, Yates C, Gorelik A, Cohney SJ, Walker RG. New Onset Diabetes After Kidney Transplantation in Autosomal Dominant Polycystic Kidney Disease: A Retrospective Cohort Study. Nephrology (Carlton) (2012) 17(1):89–96. doi: 10.1111/j.1440-1797.2011.01507.x
93. Garnier AS, Duveau A, Planchais M, Subra JF, Sayegh J, Augusto JF. Serum Magnesium After Kidney Transplantation: A Systematic Review. Nutrients (2018) 10(6):729. doi: 10.3390/nu10060729
94. Cheungpasitporn W, Thongprayoon C, Harindhanavudhi T, Edmonds PJ, Erickson SB. Hypomagnesemia Linked to New-Onset Diabetes Mellitus After Kidney Transplantation: A Systematic Review and Meta-Analysis. Endocr Res (2016) 41(2):142–7. doi: 10.3109/07435800.2015.1094088
95. Augusto JF, Subra JF, Duveau A, Rakotonjanahary J, Dussaussoy C, Picquet J, et al. Relation Between Pretransplant Magnesemia and the Risk of New Onset Diabetes After Transplantation Within the First Year of Kidney Transplantation. Transplantation (2014) 97(11):1155–60. doi: 10.1097/01.TP.0000440950.22133.a1
96. Van Laecke S, Caluwe R, Huybrechts I, Nagler EV, Vanholder R, Peeters P, et al. Effect of Magnesium Supplements on Insulin Secretion After Kidney Transplantation: A Randomized Controlled Trial. Ann Transplant (2017) 22:524–31. doi: 10.12659/AOT.903439
97. Van Laecke S, Nagler EV, Taes Y, Van Biesen W, Peeters P, Vanholder R. The Effect of Magnesium Supplements on Early Post-Transplantation Glucose Metabolism: A Randomized Controlled Trial. Transpl Int (2014) 27(9):895–902. doi: 10.1111/tri.12287
98. Hjelmesaeth J, Hartmann A, Leivestad T, Holdaas H, Sagedal S, Olstad M, et al. The Impact of Early-Diagnosed New-Onset Post-Transplantation Diabetes Mellitus on Survival and Major Cardiac Events. Kidney Int (2006) 69(3):588–95. doi: 10.1038/sj.ki.5000116
99. Cosio FG, Kudva Y, van der Velde M, Larson TS, Textor SC, Griffin MD, et al. New Onset Hyperglycemia and Diabetes Are Associated With Increased Cardiovascular Risk After Kidney Transplantation. Kidney Int (2005) 67(6):2415–21. doi: 10.1111/j.1523-1755.2005.00349.x
100. Porrini E, Diaz JM, Moreso F, Lauzurrica R, Ibernon M, Torres IS, et al. Prediabetes Is a Risk Factor for Cardiovascular Disease Following Renal Transplantation. Kidney Int (2019) 96(6):1374–80. doi: 10.1016/j.kint.2019.06.026
101. Valderhaug TG, Hjelmesaeth J, Hartmann A, Roislien J, Bergrem HA, Leivestad T, et al. The Association of Early Post-Transplant Glucose Levels With Long-Term Mortality. Diabetologia (2011) 54(6):1341–9. doi: 10.1007/s00125-011-2105-9
102. Eide IA, Halden TA, Hartmann A, Asberg A, Dahle DO, Reisaeter AV, et al. Mortality Risk in Post-Transplantation Diabetes Mellitus Based on Glucose and HbA1c Diagnostic Criteria. Transpl Int (2016) 29(5):568–78. doi: 10.1111/tri.12757
103. Seoane-Pillado MT, Pita-Fernandez S, Valdes-Canedo F, Seijo-Bestilleiro R, Pertega-Diaz S, Fernandez-Rivera C, et al. Incidence of Cardiovascular Events and Associated Risk Factors in Kidney Transplant Patients: A Competing Risks Survival Analysis. BMC Cardiovasc Disord (2017) 17(1):72. doi: 10.1186/s12872-017-0505-6
104. Sharif A, Baboolal K. Complications Associated With New-Onset Diabetes After Kidney Transplantation. Nat Rev Nephrol (2011) 8(1):34–42. doi: 10.1038/nrneph.2011.174
105. Wauters RP, Cosio FG, Suarez Fernandez ML, Kudva Y, Shah P, Torres VE. Cardiovascular Consequences of New-Onset Hyperglycemia After Kidney Transplantation. Transplantation (2012) 94(4):377–82. doi: 10.1097/TP.0b013e3182584831
106. Lim WH, Johnson DW, Hawley CM, Pascoe E, Wong G. Impact of Diabetes Mellitus on the Association of Vascular Disease Before Transplantation With Long-Term Transplant and Patient Outcomes After Kidney Transplantation: A Population Cohort Study. Am J Kidney Dis (2018) 71(1):102–11. doi: 10.1053/j.ajkd.2017.08.018
107. Ghisdal L, Van Laecke S, Abramowicz MJ, Vanholder R, Abramowicz D. New-Onset Diabetes After Renal Transplantation: Risk Assessment and Management. Diabetes Care (2012) 35(1):181–8. doi: 10.2337/dc11-1230
108. Pham PT, Pham PM, Pham SV, Pham PA, Pham PC. New Onset Diabetes After Transplantation (NODAT): An Overview. Diabetes Metab Syndr Obes (2011) 4:175–86. doi: 10.2147/DMSO.S19027
109. Bayes B, Granada ML, Pastor MC, Lauzurica R, Salinas I, Sanmarti A, et al. Obesity, Adiponectin and Inflammation as Predictors of New-Onset Diabetes Mellitus After Kidney Transplantation. Am J Transplant (2007) 7(2):416–22. doi: 10.1111/j.1600-6143.2006.01646.x
110. Midtvedt K, Hartmann A, Hjelmesaeth J, Lund K, Bjerkely BL. Insulin Resistance Is a Common Denominator of Post-Transplant Diabetes Mellitus and Impaired Glucose Tolerance in Renal Transplant Recipients. Nephrol Dial Transplant (1998) 13(2):427–31. doi: 10.1093/oxfordjournals.ndt.a027841
111. Chakkera HA, Weil EJ, Pham PT, Pomeroy J, Knowler WC. Can New-Onset Diabetes After Kidney Transplant be Prevented? Diabetes Care (2013) 36(5):1406–12. doi: 10.2337/dc12-2067
112. Lim WH, Lok CE, Kim SJ, Knoll G, Shah BR, Naylor K, et al. Impact of Pretransplant and New-Onset Diabetes After Transplantation on the Risk of Major Adverse Cardiovascular Events in Kidney Transplant Recipients: A Population-Based Cohort Study. Transplantation (2021). doi: 10.1097/TP.0000000000003639
113. Burroughs TE, Swindle J, Takemoto S, Lentine KL, Machnicki G, Irish WD, et al. Diabetic Complications Associated With New-Onset Diabetes Mellitus in Renal Transplant Recipients. Transplantation (2007) 83(8):1027–34. doi: 10.1097/01.tp.0000259617.21741.95
114. Peev V, Reiser J, Alachkar N. Diabetes Mellitus in the Transplanted Kidney. Front Endocrinol (Lausanne) (2014) 5:141. doi: 10.3389/fendo.2014.00141
115. Ponticelli C, Favi E, Ferraresso M. New-Onset Diabetes After Kidney Transplantation. Medicina (Kaunas) (2021) 57(3):250. doi: 10.3390/medicina57030250
116. Wanner C, Cooper ME, Johansen OE, Toto R, Rosenstock J, McGuire DK, et al. Effect of Linagliptin Versus Placebo on Cardiovascular and Kidney Outcomes in Nephrotic-Range Proteinuria and Type 2 Diabetes: The CARMELINA Randomized Controlled Trial. Clin Kidney J (2021) 14(1):226–36. doi: 10.1093/ckj/sfaa225
117. Kristensen SL, Rørth R, Jhund PS, Docherty KF, Sattar N, Preiss D, et al. Cardiovascular, Mortality, and Kidney Outcomes With GLP-1 Receptor Agonists in Patients With Type 2 Diabetes: A Systematic Review and Meta-Analysis of Cardiovascular Outcome Trials. Lancet Diabetes Endocrinol (2019) 7(10):776–85. doi: 10.1016/S2213-8587(19)30249-9
118. Neuen BL, Young T, Heerspink HJL, Neal B, Perkovic V, Billot L, et al. SGLT2 Inhibitors for the Prevention of Kidney Failure in Patients With Type 2 Diabetes: A Systematic Review and Meta-Analysis. Lancet Diabetes Endocrinol (2019) 7(11):845–54. doi: 10.1016/S2213-8587(19)30256-6
119. Heerspink HJL, Stefánsson BV, Correa-Rotter R, Chertow GM, Greene T, Hou FF, et al. Dapagliflozin in Patients With Chronic Kidney Disease. N Engl J Med (2020) 383(15):1436–46. doi: 10.1056/NEJMoa2024816
120. Strippoli GF, Bonifati C, Craig M, Navaneethan SD, Craig JC. Angiotensin Converting Enzyme Inhibitors and Angiotensin II Receptor Antagonists for Preventing the Progression of Diabetic Kidney Disease. Cochrane Database Syst Rev (2006) 2006(4):Cd006257. doi: 10.1002/14651858.CD006257
121. Currie G, Taylor AH, Fujita T, Ohtsu H, Lindhardt M, Rossing P, et al. Effect of Mineralocorticoid Receptor Antagonists on Proteinuria and Progression of Chronic Kidney Disease: A Systematic Review and Meta-Analysis. BMC Nephrol (2016) 17(1):127. doi: 10.1186/s12882-016-0337-0
122. Bakris GL, Agarwal R, Anker SD, Pitt B, Ruilope LM, Rossing P, et al. Effect of Finerenone on Chronic Kidney Disease Outcomes in Type 2 Diabetes. N Engl J Med (2020) 383(23):2219–29. doi: 10.1056/NEJMoa2025845
123. Heerspink HJL, Parving HH, Andress DL, Bakris G, Correa-Rotter R, Hou FF, et al. Atrasentan and Renal Events in Patients With Type 2 Diabetes and Chronic Kidney Disease (SONAR): A Double-Blind, Randomised, Placebo-Controlled Trial. Lancet (2019) 393(10184):1937–47. doi: 10.1016/s0140-6736(19)30772-x
124. Tuttle KR, Bakris GL, Toto RD, McGill JB, Hu K, Anderson PW. The Effect of Ruboxistaurin on Nephropathy in Type 2 Diabetes. Diabetes Care (2005) 28(11):2686–90. doi: 10.2337/diacare.28.11.2686
125. Tuttle KR, Brosius FC 3rd, Adler SG, Kretzler M, Mehta RL, Tumlin JA, et al. JAK1/JAK2 Inhibition by Baricitinib in Diabetic Kidney Disease: Results From a Phase 2 Randomized Controlled Clinical Trial. Nephrol Dial Transplant (2018) 33(11):1950–9. doi: 10.1093/ndt/gfx377
126. Navarro-González JF, Mora-Fernández C, Muros de Fuentes M, Chahin J, Méndez ML, Gallego E, et al. Effect of Pentoxifylline on Renal Function and Urinary Albumin Excretion in Patients With Diabetic Kidney Disease: The PREDIAN Trial. J Am Soc Nephrol (2015) 26(1):220–9. doi: 10.1681/ASN.2014010012
127. Hecking M, Haidinger M, Doller D, et al. Early Basal Insulin Therapy Decreases New-Onset Diabetes After Renal Transplantation. J Am Soc Nephrol (2012) 23(4):739–49. doi: 10.1681/ASN.2011080835
128. Lo C, Jun M, Badve SV, Pilmore H, White SL, Hawley c, et al. Glucose-Lowering Agents for Treating Pre-Existing and New-Onset Diabetes in Kidney Transplant Recipients. Cochrane Database Syst Rev (2017) 2:CD009966. doi: 10.1002/14651858.CD009966.pub2
129. Chang L, Chiang SH, Saltiel AR. Insulin Signaling and the Regulation of Glucose Transport. Mol Med (2004) 10(7-12):65–71. doi: 10.2119/2005-00029.Saltiel
130. Leto D, Saltiel AR. Regulation of Glucose Transport by Insulin: Traffic Control of GLUT4. Nat Rev Mol Cell Biol (2012) 13(6):383–96. doi: 10.1038/nrm3351
131. Sobngwi E, Enoru S, Ashuntantang G, Azabji-Kenfack M, Dehayem M, Onana A, et al. Day-To-Day Variation of Insulin Requirements of Patients With Type 2 Diabetes and End-Stage Renal Disease Undergoing Maintenance Hemodialysis. Diabetes Care (2010) 33(7):1409–12. doi: 10.2337/dc09-2176
132. Sudha MJ, Salam HS, Viveka S, Udupa AL. Assessment of Changes in Insulin Requirement in Patients of Type 2 Diabetes Mellitus on Maintenance Hemodialysis. J Nat Sci Biol Med (2017) 8(1):64–8. doi: 10.4103/0976-9668.198348
133. Nakao T, Inaba M, Abe M, Kaizu K, Shima K, Babazono T, et al. Best Practice for Diabetic Patients on Hemodialysis 2012. Ther Apher Dial (2015) 19 Suppl 1:40–66. doi: 10.1111/1744-9987.12299
134. Abe M, Kaizu K, Matsumoto K. Plasma Insulin Is Removed by Hemodialysis: Evaluation of the Relation Between Plasma Insulin and Glucose by Using a Dialysate With or Without Glucose. Ther Apher Dial (2007) 11(4):280–7. doi: 10.1111/j.1744-9987.2007.00491.x
135. Cameron AR, Logie L, Patel K, Erhardt S, Bacon S, Middleton P, et al. Metformin Selectively Targets Redox Control of Complex I Energy Transduction. Redox Biol (2018) 14:187–97. doi: 10.1016/j.redox.2017.08.018
136. Rena G, Hardie DG, Pearson ER. The Mechanisms of Action of Metformin. Diabetologia (2017) 60(9):1577–85. doi: 10.1007/s00125-017-4342-z
137. Sirtori CR, Pasik C. Re-Evaluation of a Biguanide, Metformin: Mechanism of Action and Tolerability. Pharmacol Res (1994) 30(3):187–228. doi: 10.1016/1043-6618(94)80104-5
138. Inzucchi SE, Lipska KJ, Mayo H, Bailey CJ, McGuire DK. Metformin in Patients With Type 2 Diabetes and Kidney Disease: A Systematic Review. JAMA (2014) 312(24):2668–75. doi: 10.1001/jama.2014.15298
139. Tanner C, Wang G, Liu N, Andrikopoulos S, Zajac JD, Ekinci EI. Metformin: Time to Review Its Role and Safety in Chronic Kidney Disease. Med J Aust (2019) 211(1):37–42. doi: 10.5694/mja2.50239
140. Kalantar-Zadeh K, Kovesdy CP. Should Restrictions Be Relaxed for Metformin Use in Chronic Kidney Disease? No, We Should Never Again Compromise Safety! Diabetes Care (2016) 39(7):1281–6. doi: 10.2337/dc15-2327
141. Williams ME, Garg R. Glycemic Management in ESRD and Earlier Stages of CKD. Am J Kidney Dis (2014) 63(2 Suppl 2):S22–38. doi: 10.1053/j.ajkd.2013.10.049
142. Ashcroft FM. Mechanisms of the Glycaemic Effects of Sulfonylureas. Horm Metab Res (1996) 28(9):456–63. doi: 10.1055/s-2007-979837
143. Sola D, Rossi L, Schianca GP, Maffioli P, Bigliocca M, Mella R, et al. Sulfonylureas and Their Use in Clinical Practice. Arch Med Sci (2015) 11(4):840–8. doi: 10.5114/aoms.2015.53304
144. Proks P, Reimann F, Green N, Gribble F, Ashcroft F. Sulfonylurea Stimulation of Insulin Secretion. Diabetes (2002) 51 Suppl 3:S368–76. doi: 10.2337/diabetes.51.2007.S368
145. Bloom RD, Crutchlow MF. New-Onset Diabetes Mellitus in the Kidney Recipient: Diagnosis and Management Strategies. Clin J Am Soc Nephrol (2008) 3 Suppl 2:S38–48. doi: 10.2215/CJN.02650707
146. Krepinsky J, Ingram AJ, Clase CM. Prolonged Sulfonylurea-Induced Hypoglycemia in Diabetic Patients With End-Stage Renal Disease. Am J Kidney Dis (2000) 35(3):500–5. doi: 10.1016/S0272-6386(00)70204-6
147. Kahn CR, Chen L, Cohen SE. Unraveling the Mechanism of Action of Thiazolidinediones. J Clin Invest (2000) 106(11):1305–7. doi: 10.1172/JCI11705
148. Hauner H. The Mode of Action of Thiazolidinediones. Diabetes Metab Res Rev (2002) 18 Suppl 2:S10–5. doi: 10.1002/dmrr.249
149. Saltiel AR, Olefsky JM. Thiazolidinediones in the Treatment of Insulin Resistance and Type II Diabetes. Diabetes (1996) 45(12):1661–9. doi: 10.2337/diabetes.45.12.1661
150. Kim H, Haluzik M, Gavrilova O, Yakar S, Portas J, Sun H, et al. Thiazolidinediones Improve Insulin Sensitivity in Adipose Tissue and Reduce the Hyperlipidaemia Without Affecting the Hyperglycaemia in a Transgenic Model of Type 2 Diabetes. Diabetologia (2004) 47(12):2215–25. doi: 10.1007/s00125-004-1581-6
151. Quinn CE, Hamilton PK, Lockhart CJ, McVeigh GE. Thiazolidinediones: Effects on Insulin Resistance and the Cardiovascular System. Br J Pharmacol (2008) 153(4):636–45. doi: 10.1038/sj.bjp.0707452
152. Soccio RE, Chen ER, Lazar MA. Thiazolidinediones and the Promise of Insulin Sensitization in Type 2 Diabetes. Cell Metab (2014) 20(4):573–91. doi: 10.1016/j.cmet.2014.08.005
153. Ribon V, Johnson JH, Camp HS, Saltiel AR. Thiazolidinediones and Insulin Resistance: Peroxisome Proliferatoractivated Receptor Gamma Activation Stimulates Expression of the CAP Gene. Proc Natl Acad Sci USA (1998) 95(25):14751–6. doi: 10.1073/pnas.95.25.14751
154. Zheng W, Zhou J, Song S, Kong W, Xia W, Chen L, et al. Dipeptidyl-Peptidase 4 Inhibitor Sitagliptin Ameliorates Hepatic Insulin Resistance by Modulating Inflammation and Autophagy in Ob/Ob Mice. Int J Endocrinol (2018) 2018:8309723. doi: 10.1155/2018/8309723
155. Foley JE, Jordan J. Weight Neutrality With the DPP-4 Inhibitor, Vildagliptin: Mechanistic Basis and Clinical Experience. Vasc Health Risk Manag (2010) 6:541–8. doi: 10.2147/VHRM.S10952
156. Ahren B. DPP-4 Inhibitors. Best Pract Res Clin Endocrinol Metab (2007) 21(4):517–33. doi: 10.1016/j.beem.2007.07.005
157. Ahren B. Dipeptidyl Peptidase-4 Inhibitors: Clinical Data and Clinical Implications. Diabetes Care (2007) 30(6):1344–50. doi: 10.2337/dc07-0233
158. Lyu X, Zhu X, Zhao B, Du L, Chen D, Wang C, et al. Effects of Dipeptidyl Peptidase-4 Inhibitors on Beta-Cell Function and Insulin Resistance in Type 2 Diabetes: Meta-Analysis of Randomized Controlled Trials. Sci Rep (2017) 7:44865. doi: 10.1038/srep44865
159. Drucker DJ. Dipeptidyl Peptidase-4 Inhibition and the Treatment of Type 2 Diabetes: Preclinical Biology and Mechanisms of Action. Diabetes Care (2007) 30(6):1335–43. doi: 10.2337/dc07-0228
160. Vella A. Mechanism of Action of DPP-4 Inhibitors–New Insights. J Clin Endocrinol Metab (2012) 97(8):2626–8. doi: 10.1210/jc.2012-2396
161. Thornberry NA, Gallwitz B. Mechanism of Action of Inhibitors of Dipeptidyl-Peptidase-4 (DPP-4). Best Pract Res Clin Endocrinol Metab (2009) 23(4):479–86. doi: 10.1016/j.beem.2009.03.004
162. Drucker DJ. Mechanisms of Action and Therapeutic Application of Glucagon-Like Peptide-1. Cell Metab (2018) 27(4):740–56. doi: 10.1016/j.cmet.2018.03.001
163. Hinnen D. Glucagon-Like Peptide 1 Receptor Agonists for Type 2 Diabetes. Diabetes Spectr (2017) 30(3):202–10. doi: 10.2337/ds16-0026
164. Doyle ME, Egan JM. Mechanisms of Action of Glucagon-Like Peptide 1 in the Pancreas. Pharmacol Ther (2007) 113(3):546–93. doi: 10.1016/j.pharmthera.2006.11.007
165. Collins L, Costello RA. Glucagon-Like Peptide-1 Receptor Agonists. Treasure Island (FL: StatPearls (2021).
166. Guo C, Huang T, Chen A, Chen X, Wang L, Shen F, et al. Glucagon-Like Peptide 1 Improves Insulin Resistance In Vitro Through Anti-Inflammation of Macrophages. Braz J Med Biol Res (2016) 49(12):e5826. doi: 10.1590/1414-431x20165826
167. Jiang Y, Wang Z, Ma B, Fan L, Yi N, Lu B, et al. GLP-1 Improves Adipocyte Insulin Sensitivity Following Induction of Endoplasmic Reticulum Stress. Front Pharmacol (2018) 9:1168. doi: 10.3389/fphar.2018.01168
168. KDIGO 2020 Clinical Practice Guideline for Diabetes Management in Chronic Kidney Disease. Kidney Int (2020) 98(4s):S1–115. doi: 10.1016/j.kint.2020.06.019
169. Waseda M, Satoh H, Yoshida C, Ikeda F, Kanazawa A, Watada H. Effects of SGLT2 Inhibitors on Insulin Secretion and Insulin Resistance—Results From a Cross-Sectional Study. Diabetes (2018) 67(supplement 1). doi: 10.2337/db18-1187-P
170. Sano R, Shinozaki Y, Ohta T. Sodium-Glucose Cotransporters: Functional Properties and Pharmaceutical Potential. J Diabetes Investig (2020) 11(4):770–82. doi: 10.1111/jdi.13255
171. Ghezzi C, Loo DDF, Wright EM. Physiology of Renal Glucose Handling via SGLT1, SGLT2 and GLUT2. Diabetologia (2018) 61(10):2087–97. doi: 10.1007/s00125-018-4656-5
172. White JR Jr. A Brief History of the Development of Diabetes Medications. Diabetes Spectr (2014) 27(2):82–6. doi: 10.2337/diaspect.27.2.82
173. Marso SP, Bain SC, Consoli A, Eliaschewitz FG, Jódar E, Leiter LA, et al. Semaglutide and Cardiovascular Outcomes in Patients With Type 2 Diabetes. N Engl J Med (2016) 375(19):1834–44. doi: 10.1056/NEJMoa1607141
174. Mann JFE, Ørsted DD, Brown-Frandsen K, Marso SP, Poulter NR, Rasmussen S, et al. Liraglutide and Renal Outcomes in Type 2 Diabetes. N Engl J Med (2017) 377(9):839–48. doi: 10.1056/NEJMoa1616011
175. Muskiet MHA, Tonneijck L, Huang Y, Liu M, Saremi A, Heerspink HJL, et al. Lixisenatide and Renal Outcomes in Patients With Type 2 Diabetes and Acute Coronary Syndrome: An Exploratory Analysis of the ELIXA Randomised, Placebo-Controlled Trial. Lancet Diabetes Endocrinol (2018) 6(11):859–69. doi: 10.1016/S2213-8587(18)30268-7
176. Gerstein HC, Colhoun HM, Dagenais GR, Diaz R, Lakshmanan M, Pais P, et al. Dulaglutide and Renal Outcomes in Type 2 Diabetes: An Exploratory Analysis of the REWIND Randomised, Placebo-Controlled Trial. Lancet (2019) 394(10193):131–8. doi: 10.1016/s0140-6736(19)31150-x
177. Zinman B, Wanner C, Lachin JM, Fitchett D, Bluhmki E, Hantel S, et al. Empagliflozin, Cardiovascular Outcomes, and Mortality in Type 2 Diabetes. N Engl J Med (2015) 373(22):2117–28. doi: 10.1056/NEJMoa1504720
178. Neal B, Perkovic V, Mahaffey KW, de Zeeuw D, Fulcher G, Erondu N, et al. Canagliflozin and Cardiovascular and Renal Events in Type 2 Diabetes. N Engl J Med (2017) 377(7):644–57. doi: 10.1056/NEJMoa1611925
179. Wiviott SD, Raz I, Bonaca MP, Mosenzon O, Kato ET, Cahn A, et al. Dapagliflozin and Cardiovascular Outcomes in Type 2 Diabetes. N Engl J Med (2019) 380(4):347–57. doi: 10.1056/NEJMc1902837
180. Perkovic V, Jardine MJ, Neal B, Bompoint S, Heerspink HJL, Charytan DM, et al. Canagliflozin and Renal Outcomes in Type 2 Diabetes and Nephropathy. N Engl J Med (2019) 380(24):2295–306. doi: 10.1056/NEJMoa1811744
181. Lewis EJ, Hunsicker LG, Bain RP, Rohde RD. The Effect of Angiotensin-Converting-Enzyme Inhibition on Diabetic Nephropathy. The Collaborative Study Group. N Engl J Med (1993) 329(20):1456–62. doi: 10.1056/NEJM199311113292004
182. Brenner BM, Cooper ME, de Zeeuw D, Keane WF, Mitch WE, Parving HH, et al. Effects of Losartan on Renal and Cardiovascular Outcomes in Patients With Type 2 Diabetes and Nephropathy. N Engl J Med (2001) 345(12):861–9. doi: 10.1056/NEJMoa011161
183. Lewis EJ, Hunsicker LG, Clarke WR, Berl T, Pohl MA, Lewis JB, et al. Renoprotective Effect of the Angiotensin-Receptor Antagonist Irbesartan in Patients With Nephropathy Due to Type 2 Diabetes. N Engl J Med (2001) 345(12):851–60. doi: 10.1056/NEJMoa011303
184. Ruggenenti P, Fassi A, Ilieva AP, Bruno S, Iliev IP, Brusegan V, et al. Preventing Microalbuminuria in Type 2 Diabetes. N Engl J Med (2004) 351(19):1941–51. doi: 10.1056/NEJMoa042167
185. Ruospo M, Saglimbene VM, Palmer SC, De Cosmo S, Pacilli A, Lamacchia O, et al. Glucose Targets for Preventing Diabetic Kidney Disease and Its Progression. Cochrane Database Syst Rev (2017) 6(6):Cd010137. doi: 10.1002/14651858.CD010137.pub2
186. Papademetriou V, Lovato L, Doumas M, Nylen E, Mottl A, Cohen RM, et al. Chronic Kidney Disease and Intensive Glycemic Control Increase Cardiovascular Risk in Patients With Type 2 Diabetes. Kidney Int (2015) 87(3):649–59. doi: 10.1038/ki.2014.296
187. Freedman BI, Shenoy RN, Planer JA, Clay KD, Shihabi ZK, Burkart JM, et al. Comparison of Glycated Albumin and Hemoglobin A1c Concentrations in Diabetic Subjects on Peritoneal and Hemodialysis. Perit Dial Int (2010) 30(1):72–9. doi: 10.3747/pdi.2008.00243
188. Peacock TP, Shihabi ZK, Bleyer AJ, Dolbare EL, Byers JR, Knovich MA, et al. Comparison of Glycated Albumin and Hemoglobin A(1c) Levels in Diabetic Subjects on Hemodialysis. Kidney Int (2008) 73(9):1062–8. doi: 10.1038/ki.2008.25
189. Friedman EA, Friedman AL, Eggers P. End-Stage Renal Disease in Diabetic Persons: Is the Pandemic Subsiding? Kidney Int Suppl (2006) 104:S51–4. doi: 10.1038/sj.ki.5001978
190. Nathan DM, Genuth S, Lachin J, Cleary P, Crofford O, Davis M, et al. The Effect of Intensive Treatment of Diabetes on the Development and Progression of Long-Term Complications in Insulin-Dependent Diabetes Mellitus. N Engl J Med (1993) 329(14):977–86. doi: 10.1056/NEJM199309303291401
191. Microvascular Complications and Foot Care: Standards of Medical Care in Diabetes-2020. Diabetes Care (2020) 43(Suppl 1):S135–51. doi: 10.2337/dc20-S011
192. Kawanami D, Takashi Y, Tanabe M. Significance of Metformin Use in Diabetic Kidney Disease. Int J Mol Sci (2020) 21(12):4239. doi: 10.3390/ijms21124239
193. Heaf J. Metformin in Chronic Kidney Disease: Time for a Rethink. Perit Dial Int (2014) 34(4):353–7. doi: 10.3747/pdi.2013.00344
194. Al-Hwiesh AK, Abdul-Rahman IS, Noor AS, Nasr-El-Deen MA, Abdelrahman A, El-Salamoni TS, et al. The Phantom of Metformin-Induced Lactic Acidosis in End-Stage Renal Disease Patients: Time to Reconsider With Peritoneal Dialysis Treatment. Perit Dial Int (2017) 37(1):56–62. doi: 10.3747/pdi.2015.00309
195. Duong JK, Roberts DM, Furlong TJ, Kumar SS, Greenfield JR, Kirkpatrick CM, et al. Metformin Therapy in Patients With Chronic Kidney Disease. Diabetes Obes Metab (2012) 14(10):963–5. doi: 10.1111/j.1463-1326.2012.01617.x
196. Kwon S, Kim YC, Park JY, Lee J, An JN, Kim CT, et al. The Long-Term Effects of Metformin on Patients With Type 2 Diabetic Kidney Disease. Diabetes Care (2020) 43(5):948–55. doi: 10.2337/dc19-0936
197. Yale JF. Oral Antihyperglycemic Agents and Renal Disease: New Agents, New Concepts. J Am Soc Nephrol (2005) 16 Suppl 1:S7–10. doi: 10.1681/ASN.2004110974
198. Alicic RZ, Rooney MT, Tuttle KR. Diabetic Kidney Disease: Challenges, Progress, and Possibilities. Clin J Am Soc Nephrol (2017) 12(12):2032–45. doi: 10.2215/CJN.11491116
199. Fernandez-Fernandez B, Fernandez-Prado R, Górriz JL, Martinez-Castelao A, Navarro-González JF, Porrini A, et al. Canagliflozin and Renal Events in Diabetes With Established Nephropathy Clinical Evaluation and Study of Diabetic Nephropathy With Atrasentan: What Was Learned About the Treatment of Diabetic Kidney Disease With Canagliflozin and Atrasentan? Clin Kidney J (2019) 12(3):313–21. doi: 10.1093/ckj/sfz070
200. Scirica BM, Bhatt DL, Braunwald E, Steg PG, Davidson J, Hirshberg B, et al. Saxagliptin and Cardiovascular Outcomes in Patients With Type 2 Diabetes Mellitus. N Engl J Med (2013) 369(14):1317–26. doi: 10.1056/NEJMoa1307684
201. Wheeler DC, Stefánsson BV, Jongs N, Chertow GM, Greene T, Hou FF, et al. Effects of Dapagliflozin on Major Adverse Kidney and Cardiovascular Events in Patients With Diabetic and Non-Diabetic Chronic Kidney Disease: A Prespecified Analysis From the DAPA-CKD Trial. Lancet Diabetes Endocrinol (2021) 9(1):22–31. doi: 10.1016/S2213-8587(20)30369-7
202. Morton JI, McDonald SP, Salim A, Liew D, Shaw JE, Magliano DJ. Projecting the Incidence of Type 2 Diabetes-Related End-Stage Kidney Disease Until 2040: A Comparison Between the Effects of Diabetes Prevention and the Effects of Diabetes Treatment. Diabetes Care (2021) dc210220. doi: 10.2337/dc21-0220
203. Moses RG, Colagiuri S, Pollock C. SGLT2 Inhibitors: New Medicines for Addressing Unmet Needs in Type 2 Diabetes. Australas Med J (2014) 7(10):405–15. doi: 10.4066/AMJ.2014.2181
204. Parving HH, Brenner BM, McMurray JJ, de Zeeuw D, Haffner SM, Solomon DS, et al. Cardiorenal End Points in a Trial of Aliskiren for Type 2 Diabetes. N Engl J Med (2012) 367(23):2204–13. doi: 10.1056/NEJMoa1208799
205. de Zeeuw D, Akizawa T, Agarwal R, Audhya P, Bakris GL, Chin M, et al. Rationale and Trial Design of Bardoxolone Methyl Evaluation in Patients With Chronic Kidney Disease and Type 2 Diabetes: The Occurrence of Renal Events (BEACON). Am J Nephrol (2013) 37(3):212–22. doi: 10.1159/000346948
206. de Zeeuw D, Agarwal R, Amdahl M, Audhya P, Coyne D, Garimella T, et al. Selective Vitamin D Receptor Activation With Paricalcitol for Reduction of Albuminuria in Patients With Type 2 Diabetes (VITAL Study): A Randomised Controlled Trial. Lancet (2010) 376(9752):1543–51. doi: 10.1016/S0140-6736(10)61032-X
207. Packham DK, Wolfe R, Reutens AT, Berl T, Heerspink HL, Rohde R, et al. Sulodexide Fails to Demonstrate Renoprotection in Overt Type 2 Diabetic Nephropathy. J Am Soc Nephrol (2012) 23(1):123–30. doi: 10.1681/ASN.2011040378
208. Brosius FC, Tuttle KR, Kretzler M. JAK Inhibition in the Treatment of Diabetic Kidney Disease. Diabetologia (2016) 59(8):1624–7. doi: 10.1007/s00125-016-4021-5
209. Chaudhury A, Duvoor C, Reddy Dendi VS, Kraleti S, Chada A, Ravilla R, et al. Clinical Review of Antidiabetic Drugs: Implications for Type 2 Diabetes Mellitus Management. Front Endocrinol (Lausanne) (2017) 8:6. doi: 10.3389/fendo.2017.00006
210. Sharif A, Hecking M, de Vries AP, Porrini E, Hornum M, Rasoul-Rockenschaub S, et al. Proceedings From an International Consensus Meeting on Posttransplantation Diabetes Mellitus: Recommendations and Future Directions. Am J Transplant (2014) 14(9):1992–2000. doi: 10.1111/ajt.12850
211. Teutonico A, Schena PF, Di Paolo S. Glucose Metabolism in Renal Transplant Recipients: Effect of Calcineurin Inhibitor Withdrawal and Conversion to Sirolimus. J Am Soc Nephrol (2005) 16(10):3128–35. doi: 10.1681/ASN.2005050487
212. Haller MC, Royuela A, Nagler EV, Pascual J, Webster AC. Steroid Avoidance or Withdrawal for Kidney Transplant Recipients. Cochrane Database Syst Rev (2016) 8:CD005632. doi: 10.1002/14651858.CD005632.pub3
213. Pascual J, Zamora J, Galeano C, Royuela A, Quereda C. Steroid Avoidance or Withdrawal for Kidney Transplant Recipients. Cochrane Database Syst Rev (2009) 1:CD005632. doi: 10.1002/14651858.CD005632.pub2
214. Augustine JJ, Hricik DE. Steroid Sparing in Kidney Transplantation: Changing Paradigms, Improving Outcomes, and Remaining Questions. Clin J Am Soc Nephrol (2006) 1(5):1080–9. doi: 10.2215/CJN.01800506
215. Yates CJ, Fourlanos S, Colman PG, Cohney SJ. Divided Dosing Reduces Prednisolone-Induced Hyperglycaemia and Glycaemic Variability: A Randomized Trial After Kidney Transplantation. Nephrol Dial Transplant (2014) 29(3):698–705. doi: 10.1093/ndt/gft377
216. Decker SO, Keller F, Mayer J, Stracke S. Twice Daily Fractionated Dose Administration of Prednisolone Compared to Standard Once Daily Administration to Patients With Glomerulonephritis or With Kidney Transplants. Med Klin (Munich) (2009) 104(6):429–33. doi: 10.1007/s00063-009-1091-x
217. Kramer BK, Montagnino G, Del Castillo D, Margreiter R, Sperschneider H, Olbricht CJ, et al. Efficacy and Safety of Tacrolimus Compared With Cyclosporin A Microemulsion in Renal Transplantation: 2 Year Follow-Up Results. Nephrol Dial Transplant (2005) 20(5):968–73. doi: 10.1093/ndt/gfh739
218. Hostalek U, Gwilt M, Hildemann S. Therapeutic Use of Metformin in Prediabetes and Diabetes Prevention. Drugs (2015) 75(10):1071–94. doi: 10.1007/s40265-015-0416-8
219. Alnasrallah B, Pilmore H, Manley P. Protocol for a Pilot Randomised Controlled Trial of Metformin in Pre-Diabetes After Kidney Transplantation: The Transplantation and Diabetes (Transdiab) Study. BMJ Open (2017) 7(8):e016813. doi: 10.1136/bmjopen-2017-016813
220. Alnasrallah B, Goh TL, Chan LW, Manley P, Pilmore H. Transplantation and Diabetes (Transdiab): A Pilot Randomised Controlled Trial of Metformin in Impaired Glucose Tolerance After Kidney Transplantation. BMC Nephrol (2019) 20(1):147. doi: 10.1186/s12882-019-1321-2
221. Gaiffe E, Crepin T, Bamoulid J, Courivaud C, Buchler M, Cassuto E, et al. PRODIG (Prevention of New Onset Diabetes After Transplantation by a Short Term Treatment of Vildagliptin in the Early Renal Post-Transplant Period) Study: Study Protocol for a Randomized Controlled Study. Trials (2019) 20(1):375. doi: 10.1186/s13063-019-3392-6
222. Warrilow A, Somerset S, Pumpa K, Fleet R. Metformin Use in Prediabetes: Is Earlier Intervention Better? Acta Diabetol (2020) 57(11):1359–66. doi: 10.1007/s00592-020-01559-9
223. Zinman B, Harris SB, Neuman J, Gerstein HC, Retnakaran RR, Raboud J, et al. Low-Dose Combination Therapy With Rosiglitazone and Metformin to Prevent Type 2 Diabetes Mellitus (CANOE Trial): A Double-Blind Randomised Controlled Study. Lancet (2010) 376(9735):103–11. doi: 10.1016/S0140-6736(10)60746-5
224. Haidinger M, Werzowa J, Voigt HC, Pleiner J, Stemer G, Hecking M, et al. A Randomized, Placebo-Controlled, Double-Blind, Prospective Trial to Evaluate the Effect of Vildagliptin in New-Onset Diabetes Mellitus After Kidney Transplantation. Trials (2010) 11:91. doi: 10.1186/1745-6215-11-91
225. Strom Halden TA, Asberg A, Vik K, Hartmann A, Jenssen T. Short-Term Efficacy and Safety of Sitagliptin Treatment in Long-Term Stable Renal Recipients With New-Onset Diabetes After Transplantation. Nephrol Dial Transplant (2014) 29(4):926–33. doi: 10.1093/ndt/gft536
226. Soliman AR, Fathy A, Khashab S, Shaheen N, Soliman MA. Sitagliptin Might be a Favorable Antiobesity Drug for New Onset Diabetes After a Renal Transplant. Exp Clin Transplant (2013) 11(6):494–8. doi: 10.6002/ect.2013.0018
227. Haidinger M, Werzowa J, Hecking M, et al. Efficacy and Safety of Vildagliptin in New-Onset Diabetes After Kidney Transplantation–a Randomized, Double-Blind, Placebo-Controlled Trial. Am J Transplant (2014) 14(1):115–23. doi: 10.1111/ajt.12518
228. Luther P, Baldwin D Jr. Pioglitazone in the Management of Diabetes Mellitus After Transplantation. Am J Transplant (2004) 4(12):2135–8. doi: 10.1111/j.1600-6143.2004.00613.x
229. Pietruck F, Kribben A, Van TN, Patschan D, Herget-Rosenthal S, Janssen O, et al. Rosiglitazone Is a Safe and Effective Treatment Option of New-Onset Diabetes Mellitus After Renal Transplantation. Transpl Int (2005) 18(4):483–6. doi: 10.1111/j.1432-2277.2004.00076.x
230. Kurian B, Joshi R, Helmuth A. Effectiveness and Long-Term Safety of Thiazolidinediones and Metformin in Renal Transplant Recipients. Endocr Pract (2008) 14(8):979–84. doi: 10.4158/EP.14.8.979
231. Werzowa J, Hecking M, Haidinger M, Lechner F, Doller D, Pacini G, et al. Vildagliptin and Pioglitazone in Patients With Impaired Glucose Tolerance After Kidney Transplantation: A Randomized, Placebo-Controlled Clinical Trial. Transplantation (2013) 95(3):456–62. doi: 10.1097/TP.0b013e318276a20e
232. Sanyal D, Gupta S, Das P. A Retrospective Study Evaluating Efficacy and Safety of Linagliptin in Treatment of NODAT (in Renal Transplant Recipients) in a Real World Setting. Indian J Endocrinol Metab (2013) 17(Suppl 1):S203–5. doi: 10.4103/2230-8210.119572
233. Boerner BP, Miles CD, Shivaswamy V. Efficacy and Safety of Sitagliptin for the Treatment of New-Onset Diabetes After Renal Transplantation. Int J Endocrinol (2014) 2014:617638. doi: 10.1155/2014/617638
234. Pinelli NR, Patel A, Salinitri FD. Coadministration of Liraglutide With Tacrolimus in Kidney Transplant Recipients: A Case Series. Diabetes Care (2013) 36(10):e171–2. doi: 10.2337/dc13-1066
235. Singh P, Pesavento TE, Washburn K, Walsh D, Meng S. Largest Single-Centre Experience of Dulaglutide for Management of Diabetes Mellitus in Solid Organ Transplant Recipients. Diabetes Obes Metab (2019) 21(4):1061–5. doi: 10.1111/dom.13619
236. Thangavelu T, Lyden E, Shivaswamy V. A Retrospective Study of Glucagon-Like Peptide 1 Receptor Agonists for the Management of Diabetes After Transplantation. Diabetes Ther (2020) 11(4):987–94. doi: 10.1007/s13300-020-00786-1
237. Singh P, Taufeeq M, Pesavento TE, Washburn K, Walsh D, Meng S. Comparison of the Glucagon-Like-Peptide-1 Receptor Agonists Dulaglutide and Liraglutide for the Management of Diabetes in Solid Organ Transplant: A Retrospective Study. Diabetes Obes Metab (2020) 22(5):879–84. doi: 10.1111/dom.13964
238. Kukla A, Hill J, Merzkani M, Bentall A, Lorenz EC, Park WD, et al. The Use of GLP1R Agonists for the Treatment of Type 2 Diabetes in Kidney Transplant Recipients. Transplant Direct (2020) 6(2):e524. doi: 10.1097/TXD.0000000000000971
239. Turk T, Pietruck F, Dolff S, Kribben A, Janssen OE, Mann K, et al. Repaglinide in the Management of New-Onset Diabetes Mellitus After Renal Transplantation. Am J Transplant (2006) 6(4):842–6. doi: 10.1111/j.1600-6143.2006.01250.x
240. Rajasekeran H, Kim SJ, Cardella CJ, Schiff J, Cattral M, Cherney DZI, et al. Use of Canagliflozin in Kidney Transplant Recipients for the Treatment of Type 2 Diabetes: A Case Series. Diabetes Care (2017) 40(7):e75–6. doi: 10.2337/dc17-0237
241. Kwon H, Kong J, editors. Sodium/Glucose Co-Transporter 2 (SGLT2) Inhibitor for Diabetic Kidney Transplant (KT) Patients. vol. 2017. New Orleans: ASN Kidney Week 2017 (2017).
242. Shah M, Virani Z, Rajput P, Shah B. Efficacy and Safety of Canagliflozin in Kidney Transplant Patients. Indian J Nephrol (2019) 29(4):278–81. doi: 10.4103/ijn.IJN_2_18
243. Schwaiger E, Burghart L, Signorini L, Ristl R, Kopecky C, Tura A, et al. Empagliflozin in Posttransplantation Diabetes Mellitus: A Prospective, Interventional Pilot Study on Glucose Metabolism, Fluid Volume, and Patient Safety. Am J Transplant (2019) 19(3):907–19. doi: 10.1111/ajt.15223
244. Halden TAS, Kvitne KE, Midtvedt K, Rajakumar L, Robertsen I, Brox J, et al. Efficacy and Safety of Empagliflozin in Renal Transplant Recipients With Posttransplant Diabetes Mellitus. Diabetes Care (2019) 42(6):1067–74. doi: 10.2337/dc19-0093
245. Mahling M, Schork A, Nadalin S, Fritsche A, Heyne N, Guthoff M. Sodium-Glucose Cotransporter 2 (SGLT2) Inhibition in Kidney Transplant Recipients With Diabetes Mellitus. Kidney Blood Press Res (2019) 44(5):984–92. doi: 10.1159/000501854
Keywords: diabetes, type 2 diabetes, kidney failure, dialysis, kidney transplant, mortality, post-transplant diabetes, oral hypoglycemic agents
Citation: Phillips J, Chen JHC, Ooi E, Prunster J and Lim WH (2021) Global Epidemiology, Health Outcomes, and Treatment Options for Patients With Type 2 Diabetes and Kidney Failure. Front. Clin. Diabetes Healthc. 2:731574. doi: 10.3389/fcdhc.2021.731574
Received: 27 June 2021; Accepted: 29 July 2021;
Published: 23 August 2021.
Edited by:
Tamara Poljicanin, Croatian Institute of Public Health, CroatiaReviewed by:
Charilaos Dimosthenopoulos, Laiko General Hospital of Athens, GreeceGeorgia Samakidou, National and Kapodistrian University of Athens, Greece
Copyright © 2021 Phillips, Chen, Ooi, Prunster and Lim. This is an open-access article distributed under the terms of the Creative Commons Attribution License (CC BY). The use, distribution or reproduction in other forums is permitted, provided the original author(s) and the copyright owner(s) are credited and that the original publication in this journal is cited, in accordance with accepted academic practice. No use, distribution or reproduction is permitted which does not comply with these terms.
*Correspondence: Jessica Phillips, jessica.phillips@health.wa.gov.au