- 1Department of Energy and Technology, Swedish University of Agricultural Sciences (SLU), Uppsala, Sweden
- 2Department of Chemistry, Umeå University, Umeå, Sweden
Arctic melting is an effect of climate change; the use of fossil fuels in marine shipping emits large amounts of air emissions that impact climate change, and Arctic aquatic and human life. Swedish pulp and paper mills generate large amounts of waste and side streams that could be utilized. The production of forest-based biofuel may be a promising solution to achieve sustainable Arctic marine shipping. This review highlights the socio-economic impacts associated with the production of forest-based biofuel in Sweden, the related opportunities, challenges, knowledge gaps, and further need of research. From the economic perspective, it was found that the production and use of forest-based biofuel have short and long-term economic sustainability benefits: (a) short-term benefits, the use of the waste and side streams of the pulp and paper industry is a low-cost available feedstock, unlike first-generation biofuel from crops like corn forest-based biofuels neither require additional land use, water resources nor compete with food. (b) Long-term benefits: (i) the Swedish shipping sector depends on imported fossil fuels, these new biofuels can replace partly those imported fossil fuels that will reduce shipping costs, and generate economic benefits for local consumers. (ii) Usage of forest-based biofuels as blends with conventional fuels in existing engines will reduce greenhouse gas emissions from the Arctic shipping to the set limits in the region. (iii) One of the important socio-economic impacts of forest-based biofuel production and use is the new job creation and employment opportunities that will impact the local communities and livelihoods of indigenous people in the area. From a societal perspective, stakeholder involvement is essential to address the sustainability challenges of biofuel production: EU policymakers need to encourage the production and use of biofuels by developing policies that promote biofuel use. Further studies are needed to develop more efficient and low-cost biofuel production routes, more investments in related research and development are required as well. Local indigenous communities must be involved in the decision-making process through surveys, local dialogues, and research studies. The production of forest-based biofuels has great potential and many social-economic impacts alongside the environmental benefits.
1 Introduction
Climate change impacts affect us on a daily basis all around the world, mitigation and adaptation strategies for global change have to be developed and implemented as soon as possible in the different regions. In order to limit global warming to well below two temperature degrees “2°C” and preferably one and half degrees “1.5°C” as per the Paris Agreement, several countries have investigated their carbon-neutral pathways within these objectives (Akkermans et al., 2023). Also, The Intergovernmental Panel on Climate Change (IPCC) states that all the considered pathways to limit global warming to one and half degree centigrade 1.5°C integrate the use of carbon dioxide removal (IPCC, 2018), and the adoption of negative emission technologies (Anderson, 2015).
The Paris Agreement encourages different parties to prepare and submit development strategies of long-term low greenhouse gas emission (GHG) to the United Framework Convention on Climate Change (UNFCCC). Furthermore, the United Nations (UN) approved 17 Sustainable Development Goals (SDGs), two linked to climate change and its mitigation strategies: energy access and use: ensuring access to affordable, safe, sustainable, and modern energy for all (SDG7) and climate action (SDG13). The European Climate Pact in the EU, is committed to encouraging and engaging citizens in climate action (European Commission, 2022a, 2022b), achieving these SDGs and mitigating climate change requires empowering communities to participate in energy transformation (Coy et al., 2021; Gjorgievski et al., 2021). Recently, Sweden updated its Climate policy framework aiming for a net zero GHG emissions in 2045 (BECC, 2024), this is in line with the recommendations of the last IPPC report 2023 that highlights the importance of effective climate action (IPCC, 2023).
The Arctic region is one of the global regions significantly affected by climate change. Global warming has led to the thinning of the polar ice to the point where increasing numbers of ships are using Arctic shipping routes. The decline of the extent and thickness of Arctic sea ice is projected to continue. Shipping transportation in the Arctic is thus growing significantly, and it has been estimated that the overall shipping activity in the Arctic will increase by more than 50% by 2050 due to climate change (Fridell, 2019). The prospect of an ice-free Arctic Ocean during summer periods is likely at some point in the next 30–40 years, increasing the shipping activities in the Arctic and expanding the related impacts. The main impacts of an increase in shipping in the Arctic region are: (a) an increase of black carbon (BC) and GHG emissions, consequently increasing their climate change impacts; and (b) Greater risks when carrying and burning heavy fuel oil (the main fuel used for marine shipping), risks which are associated with impacts on both marine and human life (Fridell, 2019). Currently, international shipping transportation is one of the biggest contributors to air emissions. Heavy fuel oil (HFO), marine diesel oil (MDO), and liquefied natural gas (LNG) account for 79, 16, and 5%, respectively, of the total fuel energy content consumed by international shipping (IMO, 2020). Their usage in international shipping generates 3% of the total global GHG emissions and 2% of the global BC emissions. Forecasts predict a doubling of the emissions from this sector by 2050 (Fridell, 2019; Government of Sweden (GOS), 2020).
In 2023, the International Maritime Organization (IMO) set a new ambitious target in its 2023 GHG strategy, the target is to reach 100% reduction in GHG emissions from international shipping by 2050 (IMO, 2023). Biofuels are a promising solution for marine fuel because of their capacity to reduce life cycle emissions, high energy density, compatibility with existing marine engines and bunkering infrastructure. Biofuels, which are generally low-sulfur, provide the short-term potential of meeting IMO fuel sulfur regulations, as they are already commercially available and can be used with existing engine technologies with minor modifications (Hsieh and Felby, 2017; Tyrovola et al., 2017). Furthermore, the commercialization of biofuel production to support the marine sector could foster the development of a domestic bio-economy, promoting regional job creation and economic growth. However, further investments in research and innovation development for biofuels for the maritime industry are needed (Fossil free Sweden, 2021a).
Sustainable biofuel production was an issue of interest to many scholars, several studies used life cycle assessment (LCA) methodology to assess the environmental impacts associated with biofuel production and use. LCA methodology is globally used to assess the environmental impacts along the entire life cycle of the product by the identification and quantification of consumed energy, materials, and wastes released to the environment (ISO14044, 2006). Most of the LCA studies focused on cli change impacts and related GHG emission reduction from biofuel production in comparison to conventional fuels from crude oil for transportation. Few studies took into consideration a more holistic approach taking into consideration the “disputed environmental categories,” like acidification, eutrophication, photochemical smog, human ecotoxicity, land use, and biodiversity. It was noted the emerging need to develop regional-specific characterization factors for accurate environmental assessment of biofuel production in counties of the Arctic region (Yacout et al., 2021).
At the same time, limited studies considered the socio-economic impacts related to biofuel production for shipping. Duer and Ovre Christensen (2010) considered the socio-economic impacts related to biofuel production taking into account: GHG emission reduction value from biofuel feedstock production, transport and processing, the effect on the value of substituted fossil fuels, the effect on security of energy supply and the employment effects in agriculture and industry. They used a cost–benefit analysis for the economic assessments of different biofuel production scenarios. Mvelase et al. (2023) analyzed the socio-economic studies conducted for biofuels production in South Africa focusing on assessing the economic viability and environmental impacts. They stated that “48% of the published empirical studies reviewed integrated the socio-economic and environmental impact assessment, followed by 26% that only examined the social impacts of biofuel, 15% of the studies examined economic impact only, and 11.54% examined the socio-economic impact.”
It was noted that the conducted studies so far related to biofuel production focus mainly on the environmental, social, and economic impacts of the production processes. As for the author’s knowledge, no studies take into consideration the different impacts of the usage phase. Also, most of the studies were related to biofuel production for aviation and vehicles, however, limited studies addressed the biofuel production for shipping in Arctic.
Recently, the replacement of fossil fuels by forest biomass is gaining a lot of attention due to its potential impacts to mitigate climate change. Forest biomass represents a valuable feedstock which can be used to produce various biofuels for marine shipping (Røyne, 2016). The overall environmental impacts including GHG emissions, BC and Sulphur emissions emitted by shipping in Arctic can be reduced by using forest-based biofuels as blends with conventional fuels. These new blends can help in reducing the GHGs emissions from Arctic shipping to reach the limits set by IMO (Yacout et al., 2021). Shiqi and Liming (2020) assessed the economic, environmental, and social sustainability of two forest-based biodiesels produced from palm oil and jatropha oil, based on 50 studies from 2010 till 2020. They found that the forest-based biodiesel from these oils is economically infeasible due to the low yield, high cost, high price of feedstock, global change in the price of crude oil and low fiscal subsidies. The environmental performances vary across locations and cultivation systems, most studies indicated significant reduction of 49–85% in GHG emissions compared to the use of fossil diesel. At the same time transforming high-carbon reserve lands such as rainforests into palm or jatropha plantations will cause huge GHG emissions as energy is mainly consumed during farm and mill stages for forest-based biodiesel. Compared with fossil diesel, over 70% of the non-renewable energy consumed can be saved. Also, they stated that the social benefit of forest-based biodiesel is not obvious, at present very few studies quantify the social impact of forest-based biodiesel comprehensively.
One of the main sources of forest biomass is the pulp and paper industry which represents one of the largest industries worldwide. Europe is the major producing region of paper and pulp globally, it produced approximately 90 million tons which represented around 25% of the global paper and pulp production in 2022 (Hussain, 2019). At the same time, Sweden is the top pulp-producing country in Europe with a total paper and pulp production of 11.3 million tons in 2022 (Statista, 2024a). Yacout et al. (2021) and Hussain (2019) stated that “The production and expansion of this industry is associated with several environmental and sustainability concerns. The side streams generated from this industry can be a valuable resource if used properly.” Ogner Jåstad et al. (2021) forecasted the impacts of biofuel production for road transport in the Nordic countries in the upcoming years 2023 and 2050. They predicted a strong electrification of the transport sector, resulting in a demand for biofuels of approximately 2.5 billion liters in 2035 and 1 billion liters in 2050. This will be reflected in an increased demand for biofuels from the pulp and paper industries.
Few research works investigated the potential environmental impacts of biofuel production from the pulp and paper industry. In a recent study, our research team investigated the potential environmental impacts of biodiesel and bioethanol from side streams of the pulp and paper industry and compared it to the environmental impacts of conventional fuels from crude oil used currently for marine shipping marine gas oil, and HFO. Future projection scenarios for the upcoming years 2030 and 2050 for estimating the environmental impacts of a transition from fossil fuels to biofuels in Arctic shipping were studied as well (Yacout et al., 2021). The study results indicated that a holistic view is very important for biofuel use. The production and use of forest-based bioethanol had a significantly lower impact on climate change potential, but had a higher impact on human toxicity, acidification, and eutrophication. Replacing HFO with forest-based biodiesel reduced the potential acidification by 55%, and had a lower impact on other environmental categories like climate change, acidification, and eutrophication. In order to produce forest-based biofuels in a sustainable way, we recommend further investigation of the related socio-economic impacts (Yacout et al., 2021).
This work highlights the socio-economic impacts associated with the production of forest-based biofuels for Arctic marine shipping, the related opportunities, challenges, knowledge gaps and further need for research. The production and use of waste and side streams of the pulp and paper industry for the production of biofuels for marine shipping, will not only reduce the GHG emissions from this sector and related environmental impacts on the Arctic region, but also it will save: (1) feedstock production and transportation costs, (2) costs of imported fossil fuels for marine shipping and (3) future employment opportunities will be created, which will impact the local livelihoods of indigenous people. This review provides valuable insights for policymakers interested in Sweden & the Arctic region as well as decision-makers in the shipping sector for adapting strategies that achieve Arctic sustainability and improve marine and human life.
2 Study methodology and analysis
In this study, a rapid literature review search was performed for identifying the economic and social impacts of biofuels for Arctic marine shipping. The study was done following the systematic literature review procedure by Xiao and Watson (2019) on how to conduct systematic reviews, and employed by several similar studies like Jeswani et al. (2020) and Mvelase et al. (2023). The approach includes the following two main steps:
i. Literature search and evaluation (literature identification, inclusion criterion, quality and eligibility assessment)
ii. Data extrapolation, analysis, and results.
2.1 Scoping
This review aims to assess the socio-economic impacts of biofuel production for Arctic Shipping. The reason for assessing the socio-economic impacts is the upscaling need to use biofuels in maritime shipping in the Arctic, in order to reduce the GHG emissions from this industry and related impacts on climate change, human and aquatic life in the Arctic. Martin et al. (2017) reviewed and documented the conducted studies related to the environmental and socio-economic benefits of biofuel production in Sweden. The review included the application of LCA to assess the environmental performance of biofuel production processes. The socio-economic assessment involved a screening of job creation and economic assessments. They confirmed the large environmental potential and socioeconomic benefits from biofuel production which will add value to Swedish biofuel production. However, they clarified that more work is needed in this area. This review takes into consideration further socio-economic aspects that were not addressed before like stakeholders engagement (industry, research and local indigenous communities), climate policy in EU and its role in promoting the transaction to biofuels usage. The review provides valuable insights for biofuel producers and policymakers interested in Sweden & the Arctic region, as well as local indigenous communities and the public.
The main questions addressed by this review were:
– To what extent research was done on the socio-economic impacts of biofuel production for shipping in the Arctic? in the EU? and in Sweden?
– Which aspects were investigated?
– What are the challenges, knowledge gaps, and further need for research?
2.2 Literature search
The search was conducted in different databases (Science Direct, Web of Science, Scopus, and relevant academic journals) to identify reports, academic, peer-reviewed studies on the economic and social impacts of biofuels for Arctic marine shipping. Special focus was given to literature related to forest-based biofuels production and use for marine shipping in Scandinavian countries and the case study country of Sweden. Used keywords and synonyms (socio-economic OR economic OR social AND impact AND assessment AND biofuel OR forest-based biofuels AND Arctic AND/OR shipping AND/OR Sweden). The search was restricted to articles published using the English language.
Governmental and international reports related to Arctic region were also incorporated due to their importance and relevance to the topic. To avoid outdated information, the review of the literature focused on the articles published in the period from 2014 to 2024. One important publication was also taken into account “Sweden’s strategy for the Arctic region” published by Swedish Ministry for Foreign Affairs (Government of Sweden (GOS), 2011). Presented in Figure 1 is an overview of publications considered in the study based on topic wise and geographic location. In total, 158 articles were reviewed, 21% related to the economic impacts, 31% social impacts, and 49% covered biofuels and maritime shipping. Geographically, 48% of the studies were based in Europe, 28% in Arctic and 25% in Sweden.
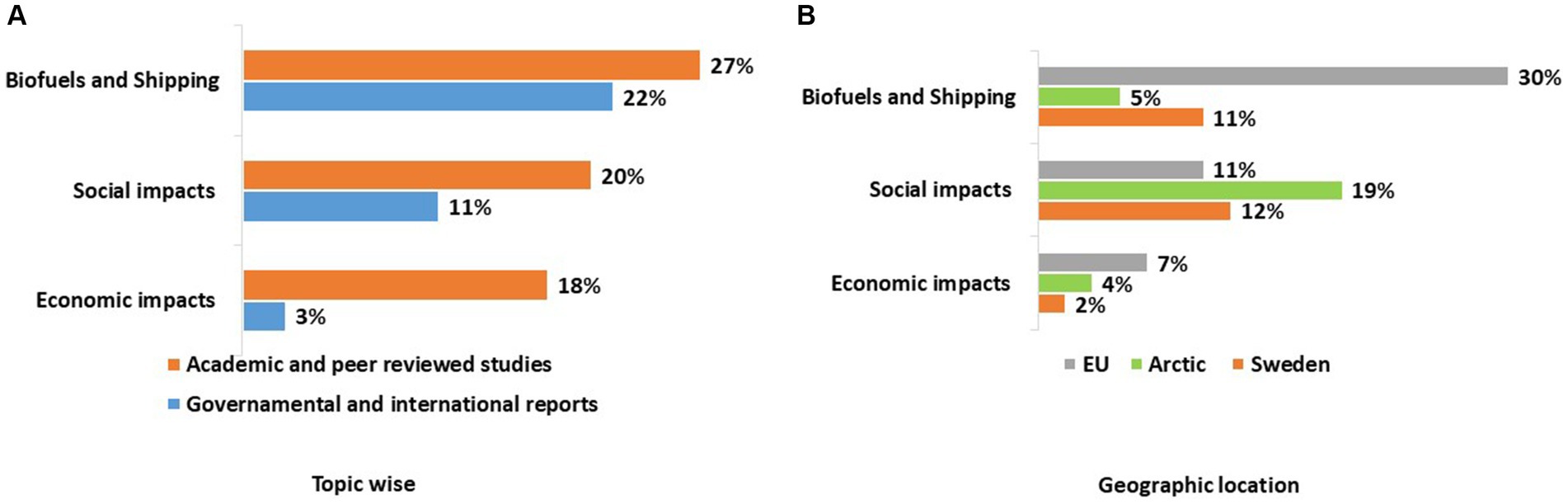
Figure 1. An overview of publications considered the study based on (A) topic and (B) geographic location.
3 International collaboration in the Arctic
The Arctic faces several sustainability challenges from inside the region, like increased engineering and technological activities, and from outside, due to the effects of global change phenomena (Figure 2). The change in the Arctic and related challenges has led to increased international interest in the region. In 1996, the Ottawa Declaration established the Arctic Council as a high-level intergovernmental forum for promoting cooperation, coordination, and interaction among the Arctic states. This council includes 8 countries (the United States, Canada, Norway, Denmark, Sweden, Finland, Iceland and Russia). The Arctic Council is the main multilateral regional format that constitutes the core cooperation in the Arctic region. Its activities focus mainly on environmental issues and sustainable development. With the increased interest in the Arctic, several countries in Europe and Asia have become observers of the Arctic Council (Government of Sweden (GOS), 2020).
4 Sweden’s strategy for the Arctic region and marine transportation
Sweden has been internationally involved with other Arctic countries in many ways. In 2011, Sweden established its own strategy for the Arctic region and became the chair of the Arctic Council for two cycles in a row. It also became the chair of the Conservation of Arctic Flora and Fauna (CAFF), Arctic Monitoring and Assessment Programme (AMAP) in 2019–2021, and Protection of the Arctic Marine Environment (PAME) in 2022–2024 (Government of Sweden (GOS), 2020). The Swedish strategy for the Arctic region was updated in 2020, with more focus on internationalization and the different impacts of climate change on the region. Sweden has emphasized three main priorities: “climate and the environment,” “economic development” and “the human dimension” (Government of Sweden (GOS), 2020) (Figure 3).
Recent efforts have been made to strengthen Sweden’s Arctic profile by making use of the full range of knowledge and resources in Sweden. Today, the Arctic region is facing severe challenges due to dramatic climate and environmental changes. Global warming has impacted the Arctic severely, causing the reduction of ice and permafrost cover, affecting biodiversity and local animals. Social impacts have been reported in the living conditions of indigenous people, the main inhabitants of the Arctic. Several economic impacts have arisen due to global warming as well. The economic importance of the Arctic region has increased, as reduced ice cover has created new conditions for the use of natural resources in the region and increased access for the exploitation of oil, gas, and minerals (Government of Sweden (GOS), 2020). Shipping traffic has increased as well due to the new shipping routes and trade destinations. Today, approximately 90% of Swedish imports and exports are transported via marine shipping. The associated impacts on local air pollution and climate change will increase with the expansion of this sector unless this issue is properly addressed (Government of Sweden (GOS), 2020).
4.1 Climate and the environment
Changes in the Arctic’s climate affect the entire world, and the area is vulnerable to climate change. The area is warming more than twice as fast as the rest of the globe, causing the melting of sea ice during the summer months as well as the melting of the permafrost, a layer that protects polar systems and reduces carbon emissions. This has severe impacts on biodiversity in the Arctic region, its ecosystem, and Indigenous people, who have inhabited the Arctic for thousands of years (Government of Sweden (GOS), 2020).
These changes in the Arctic climate have been impacting the traditional activities of Indigenous people and have threatened the livelihoods connected with activities such as hunting, fishing, and herding in the area. Hunting has become more dangerous due to fast weather changes, melting ice, thin ice, and severe weather conditions, including stronger winds and storms. Sea ice melting has impacted the biodiversity of the area, affecting many species that were harvested by Indigenous people, such as polar bears, seals, whales, and some fish stocks. Indigenous peoples have an especially strong bond with nature, meaning that the changes in harvesting activities have a series of implications on their economic, social, cultural and health lifestyles (Larsen and Fondahl, 2015).
According to the latest Swedish strategy for the Arctic region, Sweden is committed to achieving the global Sustainable Development Goals in the 2030 Agenda in the Arctic. Also, it is committed to implementing the Paris Agreement to keep the increase in global average temperature to well below 2°C above pre-industrial levels. Preserving Arctic ice and permafrost can only be achieved by limiting global warming in the Arctic region. Additionally, Sweden aims to be the world’s first fossil-free nation by 2045 (Government of Sweden (GOS), 2020; BECC, 2024).
4.2 Economic development in the Arctic
According to economic development is one of the most crucial factors for Swedish foreign affairs interests in the Arctic. Many economic activities are available in the area including mining, petroleum, land transport & infrastructure, maritime security, maritime commercial transportation, sea & air rescue, ice-breaking, energy production, tourism, hunting, fishing, and reindeer husbandry. Figure 4 presents a summary of the economic activities and geopolitical interests in the Arctic, including Sweden as one of the Arctic countries. Figure 5 shows how the different economic activities impact Arctic sustainability.
Due to climate change and related polar ice melt, new sea areas have been opened up for activities like shipping, fishing and natural resource extraction. These changes have caused the development of the Northern Sea Route (NSR) as an alternative maritime route connecting the Atlantic and Pacific Oceans. This new Arctic route has the potential to be an alternative to the Suez Canal, saving shipping time and the related costs. However, the increased traffic in this area will bring with it higher GHG emissions from shipping, higher underwater noise levels, and impacts on aquatic animals in the area (Government of Sweden (GOS), 2020).
Currently, Nordic countries, including Sweden, are looking at the Arctic passages as trade routes with great potential and importance. As Sweden is interested in the sustainable economic development of the Arctic, Sweden is actively working on promoting sustainable transportation systems in the Arctic and supporting the actions of the International Maritime Organization (IMO) in reducing GHG emissions from shipping traffic in the area. The development of the Arctic routes, including the Transpolar Sea Route (TSR), a non-coastal sea lane across the Arctic Ocean, is of special interest to Sweden, as it may convert Sweden into the most important shipping hub for Europe, due to its growing economic relationship with China (Government of Sweden (GOS), 2020).
5 Challenges and research gaps related to biofuel production in Sweden for marine transportation in the Arctic
5.1 Technology and price development
Currently, in Sweden, the total biofuel production capacity is 5 TWh/year, and projections estimate that the need for biofuel for transportation will reach 30–50 TWh/year by 2030 (SEA, 2019). Sweden has the potential to increase domestic production of renewable fuels from the forest industry, its residues, and by-products. However, alternative biofuel production technologies that can use feedstock from the forest industry are not competitive, due to high investment costs and technology immaturity. The economic risk is a factor that needs to be reduced to scale up several of these technological advances. One of the potential solutions is to investigate policy instruments to promote the Swedish production of biofuels using new and innovative technologies (Mossberg et al., 2019; Government of Sweden (GOS), 2020).
5.2 Feedstock availability
Biomass as feedstock can be produced from several types of lignocellulosic biomass, including forest wastes, and waste from the pulp and paper industry. Its availability may depend on local waste collection systems and the capital in place for extraction. These waste supply streams could be converted into valuable maritime fuels (Ramirez et al., 2015; Tyrovola et al., 2017; Jeswani et al., 2020).
Today, in Sweden, most of the forest residues, waste, and by-products from the pulp and paper industry are used for internal energy supply, and only 20 TWh/year is used outside of Sweden, mainly for electricity and heat production. Residues and by-products from forestry consist of about 10 TWh/year of branches and tops, 5 TWh/year of damaged wood, and 10 TWh/year of firewood, which is also mostly used for electricity and heat production (Fossil free Sweden, 2021b).
Most of the biofuel production that takes place in Sweden today from forest feedstock is based on tall oil formed during pulp production. The production of tall oil currently amounts to 1 TWh/year. This, together with a small production of methanol at the Monsterås pulp mill and ethanol at Domsjö Fabriker, is the only forest-based biofuel production currently taking place in Sweden (Fossil free Sweden, 2021b).
5.3 Cold weather performance
Biofuels used for ships in the Arctic will be subjected to low temperatures, meaning that they must be able to perform in cold weather. Some biofuels, however, may have consistency and stability issues in these conditions. It is important to perform long-term biofuel engine testing and to take fuel storage and its oxidation stability into account (Mohd Noor et al., 2018). Stated that “Understanding the downstream impacts of fuel stability across the value chain could avert potential technical bottlenecks and thus increase fuel adoption.” Mohd Noor et al. (2018) suggested using biofuels with marine ships in shorter routes, allowing for more frequent bunkering. This kind of ship can reach its bunkering destination in less than eight weeks and is not likely to develop any stability issues in that time, especially if the fuel is stored between 14°C and 43°C.
6 Discussion
6.1 Sweden and the potential of biofuel production for marine transportation
Sweden has been working towards implementing its strategy for the Arctic region 2020. When it comes to marine transportation in the Arctic, the maritime industry in Sweden enables the transportation of passengers and goods between continents and countries, making it a vital tool for Sweden’s imports and exports. Domestic shipping emits approximately 700,000 tonnes of CO2 equivalents a year, amounting to approximately 4% of emissions from domestic transport (Fossil free Sweden, 2021a).
Biofuels are a promising solution for climate change mitigation via GHG emissions reduction, they are considered to be carbon-neutral, accounting for the direct CO2 emissions associated with their combustion on the grounds that the carbon dioxide absorbed by the plants through photosynthesis is equivalent to the CO2 released during their combustion. In spite of that, it is worth mentioning that part of biofuels carbon may have a fossil origin and is responsible for “non-neutral” CO2 emissions (Sebos et al., 2020; Sebos, 2022).
The supply of biofuels in Sweden has tripled over the last 40 years, according to the Swedish Energy Agency in 2023. The total energy supply from biofuel was 141 TWh, but only 21 TWh (14.8% of total biofuel) was used in the transportation sector (SEA, 2023). The maritime shipping sector in Sweden still relies on fossil fuels as the main source of energy, with shipping consuming about 2.2% of the total energy use in the transportation sector (SEA, 2022).
Biofuel production for shipping has great potential, and some biofuels, like biodiesel from Rapeseed oil (1st generation) and from Lignocellulose “forest–based from wood” (2nd generation), are commercially available. These fuels can be used with the existing infrastructure without the need for major technical modifications. They can be used as drop-in fuels or blended with conventional fuels; they are completely compatible with the existing engines (Smith et al., 2014; Hsieh and Felby, 2017; PAME, 2019). Biodiesel can restore the lubricity of the engine, reduce smoke, soot and burnt diesel odour from engine exhaust, and protect against wear in the fuel and injector pumps (Hsieh and Felby, 2017). Additionally, these biofuels have fuel properties and combustion characteristics similar to those of the conventional fuels used in shipping, like Heave fuel oil (HFO) and Marine gas oil (MGO), but with an important addition, the potential of reducing GHG emissions (Mohd Noor et al., 2018; Kesieme et al., 2019). Marine fuel products are manufactured according to ISO 8217 (2017) and ASTM D975 standards, the latter allowing blends of 5% biodiesel, known as B5 fuels, which are currently available in most countries (Mohd Noor et al., 2018).
Industrial waste from pulp and paper mills can be used for biofuel production. In Sweden, the pulp and paper industry generates approximately 380,000 tons of waste sludge, which is co-combusted in on-site boilers or disposed of to landfill. This waste stream could be utilized and used as a valuable resource to produce “Forest-based biofuel.” However, the production and use of forest-based biofuels for marine shipping in the Arctic region must be done in a sustainable way, ensuring the preservation of the local environment, and considering the impacts on marine life and the health risks for local communities, especially Indigenous peoples in the North of Sweden (Yacout et al., 2021).
Recently, Martin et al. (2017) studied the conducted research works related to the environmental and socio-economic impacts of biofuel production in Sweden. They found that from an environmental perspective, there is a large potential for GHG reduction by replacing conventional fuels with biofuels like ethanol, biogas, and lignocellulosic fuel production. They stated that “With the current Swedish biofuel production portfolio, consideration of non-fuel related benefits could lead to 50% greater GHG emission savings, compared to when only considering the replaced fossil fuels.” At the same time, biofuel production may lead to other negative environmental impacts, especially on acidification and eutrophication potentials. A holistic view needs to be taken into consideration in such cases (Martin et al., 2017; Yacout et al., 2021). Furthermore, the collected information about the socio-economic impacts of biofuel production revealed that the opportunities for job creation are equal to one full-time employment per GWh, and the regional domestic product gains is around 1 MSEK per GWh of fuel (Martin et al., 2017).
6.2 Economic benefits
In agreement with Ogner Jåstad et al. (2021) wood biomass has an important role in Nordic countries due to the large resource base, long history of wood processing industries, and forest resources that represent the main potential for biofuel production in these countries. Sweden as one of the Nordic countries, owns large amounts of biomass, and it has highly developed bioenergy systems, including systems to produce biofuels for transportation, which gives Sweden the potential opportunity to expand local biofuel production based on domestic feedstock (Peck, 2017). This expansion will create various economic benefits. As suggested by Ogner Jåstad et al. (2021) and Tavera-Ruiz et al. (2023), a critical factor for sustainable biofuel production is economic viability. Biofuels have the benefit of reducing GHG emissions and the reliance on fossil fuels. However, their economic sustainability depends on many factors, including feedstock accessibility, production expenses, used technology, and efficiency of the production process. From an economic perspective, the production of forest-based biofuels for marine transportation has several benefits (Figure 6). These benefits can be divided into short-term and long-term economic sustainability benefits as follows:
6.2.1 Short term benefits
6.2.1.1 Low cost available feedstock
Some of the concerns related to biofuels production are the upscaling pressure on food availability, the risk of increasing GHG emissions through direct and indirect land-use change from production of biofuel feedstock, the risks of land degradation, reduction of water resources and changes in the ecosystems. For example, the use of first-generation feedstock like corn, sugarcane and wheat become a global concern due to its competition with food production and diversion of agricultural land into fuel production land (UNEP, 2009; Jeswani et al., 2020). In agreement with Kumar (2023) biofuels have to be both energy and water-efficient to ensure overall sustainability. These issues can be mitigated when using second-generation biofuels from wood biomass, the production of forest-based biofuels depends on using the surplus waste and by-products of the pulp and paper industry. It is a biofuel that does not require new land use or any feedstock production costs, unlike other biofuels that require land use and may compete with food production.
6.2.2 Long term benefits
6.2.2.1 Production and use costs
Nowadays in Sweden, the maritime shipping sector depends on imported fossil fuels as the main source of energy. If forest-based biofuels are produced domestically at a larger scale and used in maritime shipping replacing conventional fuels this could lead to lower fossil fuel imports and reduce local vulnerability to the adverse impacts of supply disruptions. Additionally, reducing local demand for imported petroleum could reduce shipping costs, and generate economic benefits for local consumers (US EPA, 2010, 2024; Huang et al., 2013).
At the same time, reusing the generated waste from the pulp and paper industry as biomass feedstock saves the costs of their handling and disposal. This waste is either used onsite for heating or needs to be transported and disposed of in large dumps or incinerated in proper facilities. When using this waste for onsite biofuel production, the cost of both the transportation and the disposal or incineration of waste used for biofuel production will be avoided.
6.2.2.2 Reduction of GHG emissions
To reduce GHG emissions from maritime shipping in the Arctic, the Maritime Environment Protection Committee (MEPC) under the International Maritime Organization (IMO) set a number of regulation among them:
– Staring from 1 of January 2020, Sulphur content of any fuel oil used in Arctic shipping must be below 0.50% (Artic council, 2024).
– As on 1 July 2024, it will be prohibited the use of HFO by ships in Arctic (Artic council, 2024).
MEPC asked member states of the Arctic council including Sweden and ship operators in the Arctic to use cleaner alternative fuels or methods of propulsion that are safe for ships and could contribute to the reduction of Black Carbon emissions from ships when operating in or near the Arctic. As a result, the Arctic ships started using distil fuels and scrubbers to meet the new regulations and reduce their related GHG emission. Forest-based biofuels have the potential to be used as drop-in fuels (blends) in combination with conventional fuels in existing engines of maritime shipping in Arctic, that will reduce GHG emissions to the desired levels (Yacout et al., 2021). In this case, the cost of emission reduction through the use of scrubbers in ships that use conventional fuels from crude oil, which generates large amounts of GHG emissions, will be avoided.
6.2.2.3 Job creation and employment
The total EU employment in the biofuels industry provide more than 248,200 new jobs in 2018, 283,000 jobs in 2020, these number fluctuated in the next years reaching 149,700 people in 2022 (European Commission, 2022a,b; Statista, 2024b). The production of forest-based biofuel is considered a new opportunity for creating new jobs and provide new employment. Martin et al. (2017) studied the economic benefits biofuel production in Sweden, by modeling an ambitious scenario where domestic biofuel production in Sweden generates 5 TWh per year, the total number of newly created employment opportunities can range between 450–1,100 and 1,000–1,200 per TWh from bioethanol and biodiesel production, respectively.
Similarly, Lechón et al. (2019) studied the socio-economic impacts of biofuel production in Uruguay, they considered the local production of bioethanol from sugarcane and bioethanol from sorghum. They found high impacts on economic activity in the form of job creation and employment opportunities. They reported that around 34,000 full-time new jobs can be created as a result of bioethanol production from sugarcane, and half this number of new jobs can be created due to biodiesel production from sorghum.
6.3 Social aspects
6.3.1 Local indigenous communities in Arctic
Shipping transportation in the Arctic is growing significantly, exacerbating climate change, endangering delicate ecosystems, and causing marine and human health risks, especially for Indigenous peoples in the Arctic. Biofuel production may provide the potential to reduce the related impacts of conventional fuel use in Arctic shipping. However, biofuel production can cause land use changes, the displacement of local communities, and the violation of human rights in the form of unsuitable labor conditions (Mendieta et al., 2021).
Indigenous communities, including the Sámi people, who live in parts of Norway, Sweden, Finland, and Russia, are major players in the fight against climate change. These communities own or manage about 18% of the Earth’s land surface. Moreover, up to 80% of global biodiversity occurs on land managed by Indigenous communities. The displacement of these local communities is one of the most alarming social impacts of biofuels. The conversion of natural habitats like forests into bioenergy farms forces indigenous and traditional communities to leave their homes, disrupting their livelihoods and way of life (Mendieta et al., 2021).
Obaideen et al. (2022) argued that to ensure that biofuels are socially sustainable, policies and regulations must be put in place to protect the rights and well-being of local communities and promote fair labor practices. Utilizing sustainable feedstock, such as waste and residues, and avoiding the conversion of natural ecosystems into bioenergy crops are crucial steps in this direction. The production of forest-based biofuels from the waste and side streams of existing pulp and paper mills creates new employment opportunities which allow for the improvement of the livelihoods of Indigenous people without the risk of displacement of local communities.
6.3.2 European Union (EU) policies to mitigate CO2 and local communities
The recent IPCC report (2023) addressed the importance of climate policies and the increasing need for international collaboration to reduce and mitigate climate impacts. Nowadays, the Arctic is becoming of strategic importance to the EU for its climate, energy and space-related possibilities (Hanaček et al., 2022; Broek, 2023). The risks of climate change extend to many social aspects as well as environmental and economic, as a result, the EU is continuously promoting important institutional initiatives to protect agricultural production, ensure food security, social stability and protect the environment in different areas (Kyriakopoulos et al., 2023).
The EU provides the opportunity to improve resource efficiency performance and sustainable economic, social, environmental, and climate growth via European Innovation Partnership (EIP) operational groups. In Greece for example, they assisted young farmers, investing in the processing, and marketing of agricultural products, as well as in forestry technologies and the marketing of forest products, more than 70,000 new employment positions will be created in rural areas (Kyriakopoulos et al., 2023). Similar approaches can be considered for improving the livelihoods of Indigenous people by investing in the production of forest-based biofuels in local communities of the Arctic region.
In agreement with Martin et al. (2015, 2017) biofuel production is linked to climate policy, some biofuel producers have suggested that policies and regulations have limited the development and investments in biofuel production due to limits on biofuels produced from “food crops” and associated land use concerns (Lantmännen, 2014; Martin et al., 2015). Therefore, policies and guidelines on quantitative and qualitative measures should take into account an array of impact categories to ensure biofuels are produced sustainably. EU policy-makers can assist more in the transitions to biofuel production and use by: providing data used for sustainability assessments of biofuels under the Renewable Energy Directive program to improve data availability and transparency, encourage dialogues and collaborations with researchers and biofuel producers to create and monitor methods for the environmental, economic and social impact assessment of biofuels. They should be also more involved in identifying the potential impacts of biofuels and raising awareness about it among the public including local communities (Martin et al., 2015).
6.3.3 Stakeholders engagement
Stakeholders engagement is crucial for the success of climate policies and adaptation of climate strategies to reduce GHG emissions including production and use of biofuels. Stakeholders can be engaged at different levels, for example:
a) Local Indigenous communities: The EU upholds the rights of local Indigenous communities in the Arctic, granting the right to free, prior and informed consent, enabling Indigenous peoples to give or withhold their consent to projects in their areas (Broek, 2023; Nystø Keskitalo and Götze, 2023). In order to ensure efficient climate adaptation of different mitigation strategies, awareness raising and involvement among a variety of key stakeholders is a must. Local stakeholders like executives and staff in both the public and private sectors have to be engaged actively (Ioanna et al., 2022; Sebos et al., 2023). Furthermore, Indigenous peoples have a time-tested understanding of their Arctic environments and living sustainably, their involvement and inputs can help in preventing unsustainable and conflictual projects (Westeson, 2022; Broek et al., 2023). They can be involved in many ways like through surveys, local dialogues, and research studies.
b) Biofuel industry and decision-makers: these stakeholders should increase their collaboration with both the research community and policy-makers to co-develop assessment methods that improve LCA methodologies used for assessing the environmental impacts of biofuel production and use (Martin et al., 2015). They should also be involved in both: increasing knowledge dissemination to the public on the potential benefits of producing sustainable biofuels, and improving awareness of the related environmental, economic and social impacts.
c) Research community: researchers have a great responsibility and a major role in promoting the use of biofuels by conducting more research and development to produce biofuels more efficiently and economically, improving the availability and access to LCA data to improve transparency and reduce uncertainty, include datasets in the publication of biofuel LCAs, employ holistic approaches and improving availability of assessments (Von Blottnitz and Curran, 2007; Martin et al., 2015), finally, development of regional specific characterization factors for the Arctic region (Yacout et al., 2021).
6.3.4 COVID-19 and the Arctic
The Arctic is considered particularly vulnerable to pandemics due the limited infrastructure and healthcare options, remoteness, difficult socioeconomic conditions, and painful histories of colonization and neglect from central governments in the past (Huot et al., 2019; Petrov et al., 2020; Adams and Dorough, 2022). The COVID-19 pandemic declared by the World Health Organization on 11 March 2020 has had a profound global impact on everyone around the world, including all Nordic countries in the Arctic region. COVID-19 had a substantial role in the economic growth and employment levels (Gassen and Penje, 2021; Papadogiannaki et al., 2023).
Furthermore, the Covid-19 pandemic revealed a shortage in energy and critical materials in Europe. It revealed that the EU is currently dependent on imports of critical materials, almost 70% of EU critical materials are imported from Russia and China (Broek, 2023). These critical materials are available in many local communities in the Arctic such as Kiruna in Northern Sweden, which opens the opportunity for further exploration, investments and employment opportunities in these areas. However, this needs to be done in a sustainable way taking into consideration the involvement of the local indigenous people in the area (Broek, 2023).
7 Further considerations for Arctic sustainability
Arctic sustainability is an important issue that is gaining more and more attention. Further research is required to identify sustainable products for Arctic transportation and settlements. The framework for socio-economic benefits (presented in Figure 6) can be applied to the different potential products of Arctic concern produced from the by-products and waste from the forest industry. Potential products of Arctic concern can be divided into three categories:
1) Petroleum fuels (fuels like diesel, HFO, MGO, etc.) and potential replaceable biofuels (wood-based biodiesel, etc.)
2) Other materials from the forest industry, such as biochemicals and biobased composites, biobased paints, which, like the currently used products (e.g., copper coatings and biocides), are used to prevent hull fouling, can lead to problems like ecotoxicity, etc.
3) Remote heating scenarios, taking into consideration the cost of heating houses in remote areas which use waste like wood chips and are not connected to the district heating network.
8 Conclusion
The need for developing mitigation solutions for climate change adaptation in the Arctic region became a must, reducing GHG emissions using biofuels for Arctic shipping is a potential solution with many benefits. This work highlighted the potential impacts of sustainable production of forest-based biofuels from waste and side streams of the pulp and paper industry for Arctic shipping. These biofuels can replace the most used conventional fuels for marine shipping from crude oil. In addition to the environmental benefits of reducing GHG emissions from the socio-economic perspective, the feedstock is considered a low-cost one, and its production will create new employment opportunities that will impact the local livelihoods of indigenous people.
However, further efforts are required to address the sustainable challenges associated with biofuels production. The involvement of stakeholders at different levels is essential, EU policy makers need to lead the conversation with researchers, biofuel producers, and local communities to develop policies that encourage the production and use of biofuels. Investments in related research and development are required to ensure efficient and sustainable biofuels production. Local indigenous communities can be involved in many ways through surveys, local dialogues, and research studies. They have local knowledge of the Arctic environments and living sustainably, their inputs can develop sustainable and natural-based solutions for biofuel production in the region. The biofuel industry and decision-makers have to be involved with researchers in developing methods for sustainable assessment of biofuel production, also their engagement is needed to raise awareness about the positive impacts of biofuels among the public including local communities. Researchers have a great role in developing sustainable biofuels by employing holistic approaches taking into consideration the different environmental impact categories, along with the socio-economic impacts and development of regional-specific characterization factors for the Arctic region.
Arctic sustainability is a major issue that is gaining more and more attention, further research is needed for the sustainable development of products for Arctic transportation and settlements like wood-based biodiesel, biochemical, biobased composites, and biobased paints.
Author contributions
DY: Conceptualization, Data curation, Funding acquisition, Investigation, Methodology, Project administration, Resources, Visualization, Writing – original draft, Writing – review & editing. MT: Conceptualization, Visualization, Writing – original draft, Writing – review & editing. VU: Visualization, Writing – original draft, Writing – review & editing.
Funding
The author(s) declare financial support was received for the research, authorship, and/or publication of this article. This work was supported by the Swedish Research Council for Sustainable Development FORMAS grant 2020-00879.
Acknowledgments
The authors gratefully acknowledge the FORMAS grant 2020-00879 and the Strategic Research Programme Bio4Energy for funding this research work.
Conflict of interest
The authors declare that the research was conducted in the absence of any commercial or financial relationships that could be construed as a potential conflict of interest.
Publisher’s note
All claims expressed in this article are solely those of the authors and do not necessarily represent those of their affiliated organizations, or those of the publisher, the editors and the reviewers. Any product that may be evaluated in this article, or claim that may be made by its manufacturer, is not guaranteed or endorsed by the publisher.
References
Adams, L. V., and Dorough, D. S. (2022). Accelerating indigenous health and wellbeing: the lancet commission on Arctic and northern health. Lancet (London, England) 399, 613–614. doi: 10.1016/S0140-6736(22)00153-2
Akkermans, S., Martín-Ortega, J. L., Sebos, I., and López-Blanco, M. J. (2023). Exploring long-term mitigation pathways for a net zero Tajikistan. Mitig. Adapt. Strateg. Glob. Chang. 28, 1–26. doi: 10.1007/s11027-023-10053-w
BECC (2024). Annual report 2022/23. Published by Lund University, Lund, Sweden. Available at: https://www.becc.lu.se/world-leading-research-biodiversity-and-ecosystem-services-changing-climate/becc-annual-report-20222023
Broek, E. (2023) The Arctic is hot: addressing the social and environmental implications. doi: 10.55163/VJEE5200
Broek, E., Olczak, N., and Dellmuth, L. (2023) The involvement of civil society organizations in Arctic governance. doi: 10.55163/NKQM8574
Coy, D., Malekpour, S., Saeri, A. K., and Dargaville, R. (2021). Rethinking community empowerment in the energy transformation: a critical review of the definitions, drivers and outcomes. Energy Res. Soc. Sci. 72:101871. doi: 10.1016/j.erss.2020.101871
Duer, H., and Ovre Christensen, P. (2010). Socio-economic aspects of different biofuel development pathways. Biomass Bioenergy 34, 237–243. doi: 10.1016/j.biombioe.2009.07.010
European Commission (2022a) In focus: employment in EU’s renewable energy sector. Available at: https://commission.europa.eu/news/focus-employment-eus-renewable-energy-sector-2022-05-16_en (Accessed June 12, 2024)
European Commission. (2022b) European climate pact. Communication from the commission to the European Parliament, the council, the European economic and social committee and the Committee of the Regions; (inform no. COM 2020 788); European Commission: Brussels, Belgium. Available at: https://europa.eu/climate-pact/system/files/2020-12/20201209%20European%20Climate%20Pact%20Communication.pdf (Accessed May 12, 2024).
Fossil free Sweden (2021a). Roadmaps for fossil free competitiveness – follow up 2021. Available at: https://fossilfrittsverige.se/wp-content/uploads/2022/01/Roadmaps_follow_up_2021_ENG.pdf
Fossil free Sweden (2021b). Strategy for fossil-free competitiveness – bioenergy and bio-based feedstock in industry transition.
Fridell, E. (2019). “Chapter 2 – Emissions and fuel use in the shipping sector” in Green ports. eds. R. Bergqvist and J. Monios (Amsterdam: Elsevier), 19–33.
Gassen, N., and Penje, O. (2021) Integrating immigrants into the Nordic labour markets. The impact of the COVID-19 pandemic. Available at: https://pub.norden.org/nord2021-050/
Gjorgievski, V. Z., Cundeva, S., and Georghiou, G. E. (2021). Social arrangements, technical designs and impacts of energy communities: a review. Renew. Energy 169, 1138–1156. doi: 10.1016/j.renene.2021.01.078
Government of Sweden (GOS) (2011). Sweden’s strategy for the Arctic region. Stockholm, Sweden: Swedish Ministry for Foreign Affairs.
Government of Sweden (GOS) (2020) Ministry of Infrastructure, Uppdrag att utreda behovet av ytterligare styrmedel för att främja ökad inhemsk produktion av biodrivmedel.
Hanaček, K., Kröger, M., Scheidel, A., Rojas, F., and Martinez-Alier, J. (2022). On thin ice: the Arctic commodity extraction frontier and environmental conflicts. Ecol. Econ. 191:107247. doi: 10.1016/j.ecolecon.2021.107247
Hsieh, C. C., and Felby, C. (2017). Biofuels for the marine shipping sector. University of Copenhagen, IEA Bioenergy Task. 39.
Huang, H., Khanna, M., Onal, H., and Chen, X. (2013). Stacking low carbon policies on the renewable fuels standard: economic and greenhouse gas implications. Energy Policy 56, 5–15. doi: 10.1016/j.enpol.2012.06.002
Huot, S., Ho, H., Ko, A., Lam, S., Tactay, P., MacLachlan, J., et al. (2019). Identifying barriers to healthcare delivery and access in the circumpolar north: important insights for health professionals. Int. J. Circumpolar Health 78:1571385. doi: 10.1080/22423982.2019.1571385
Hussain, S. (2019) Top 10 Pulp & Paper Producing Countries in World (2022). World Paper Mill. Available at: https://worldpapermill.com/top-pulp-paper-producing-countries/ (Accessed June 09, 2024)
IMO. (2023) Revised GHG reduction strategy for global shipping adopted. Available at: https://www.imo.org/en/MediaCentre/PressBriefings/pages/Revised-GHG-reduction-strategy-for-global-shipping-adopted-.aspx (Accessed March 21, 2024).
Ioanna, N., Pipina, K., Despina, C., Ioannis, S., and Dionysis, A. (2022). Stakeholder mapping and analysis for climate change adaptation in Greece. Euro-Mediterr. J. Environ. Integr. 7, 339–346. doi: 10.1007/s41207-022-00317-3
IPCC (2018). “Summary for policymakers” in Global warming of 1.5 °C. An IPCC special report on the impacts of global warming of 1.5 °C above pre-industrial levels and related global greenhouse gas emission pathways, in the context of strengthening the global response to the threat of climate change, sustainable development, and efforts to eradicate poverty. eds. V. Masson-Delmotte, P. Zhai, and H.-O. Pörtner, et al. (Geneva: World Meteorological Organization).
IPCC (2023) AR6 synthesis report climate change 2023. Available at: https://www.ipcc.ch/report/ar6/syr/
ISO 8217 (2017). Petroleum products — Fuels (class F) — Specifications of marine fuels, Geneva, Switzerland
ISO14044 (2006) International Organization for Standardizations: Environmental management—Life cycle assessment—Requirements and guidelines, Geneva, Switzerland.
Jeswani, H. K., Chilvers, A., and Azapagic, A. (2020). Environmental sustainability of biofuels: a review. Proc. R. Soc. A 476:20200351. doi: 10.1098/rspa.2020.0351
Kesieme, U., Pazouki, K., Murphy, A., and Chrysanthou, A. (2019). Biofuel as an alternative shipping fuel: technological, environmental and economic assessment. Sustain. Energy Fuel. 3, 899–909. doi: 10.1039/C8SE00466H
Kumar, A. (2023) Biofuels: short-term goals versus long-term sustainability. ETEnergyWorld. Available at: https://energy.economictimes.indiatimes.com/news/oil-and-gas/biofuels-short-term-goals-versus-long-term-sustainability/106078935
Kyriakopoulos, G. L., Sebos, I., Triantafyllou, E., Stamopoulos, D., and Dimas, P. (2023). Benefits and synergies in addressing climate change via the implementation of the common agricultural policy in Greece. Appl. Sci. 13:2216. doi: 10.3390/app13042216
Lantmännen (2014). Lantmännen Energis remissyttrande avseende Utredningen om en fossilfri fordonstrafik (SOU 2013:84) (Dnr N2014-7434-E)
Larsen, J. N., and Fondahl, G. (2015). Arctic human development report: regional processes and global linkages. Copenhagen: Nordisk Ministerrådet.
Lechón, Y., de la Rúa, C., Rodríguez, I., and Caldés, N. (2019). Socioeconomic implications of biofuels deployment through an input-output approach. A case study in Uruguay. Renew. Sustain. Energy Rev. 104, 178–191. doi: 10.1016/j.rser.2019.01.029
Martin, M., Lazarevic, D., Larsson, M., and Aid, G. (2015). Carbon vision? Reviewing environmental systems analyses of biofuel production in Sweden. F3 project research report manuscript, 2015
Martin, M., Wetterlund, E., Peck, P., Hackl, R., and Holmgren, K. (2017) Environmental and socio-economic benefits of Swedish biofuel production. Report no 2017:01, f3 the Swedish knowledge Centre for Renewable Transportation Fuels, Sweden. Available at: www.f3centre.se.
Mendieta, O., Castro, L., Vera, E., Rodríguez, J., and Escalante, H. (2021). Toward the adoption of anaerobic digestion technology through low-cost biodigesters: a case study of non-centrifugal cane sugar producers in Colombia. Water 13:2566. doi: 10.3390/w13182566
Mohd Noor, C. W., Noor, M. M., and Mamat, R. (2018). Biodiesel as alternative fuel for marine diesel engine applications: a review. Renew. Sustain. Energy Rev. 94, 127–142. doi: 10.1016/j.rser.2018.05.031
Mossberg, J., Pettersson, K., Furusjö, E., Baky, A., and Klintbom, P. (2019). Perspektiv på svenska förnybara drivmedel. Uppsala, Sweden: BioDriv Öst.
Mvelase, L. M., Ferrer, S. R. D., and Mustapha, N. (2023). The socio-economic impact assessment of biofuels production in South Africa: a rapid structured review of literature. Cogent. Cogent Eng. 10, 1–29. doi: 10.1080/23311916.2023.2192328
Nystø Keskitalo, A., and Götze, J., (2023) ‘A rights-based approach for implementing the European green Deal’, German Institute of Development and Sustainability (IDOS).
Obaideen, K., Abdelkareem, M. A., Wilberforce, T., Elsaid, K., Sayed, E. T., Maghrabie, H. M., et al. (2022). Biogas role in achievement of the sustainable development goals: evaluation, challenges, and guidelines. J. Taiwan Inst. Chem. Eng. 131:104207. doi: 10.1016/j.jtice.2022.104207
Ogner Jåstad, E., Folsland Bolkesjø, T., Rørstad, P. K., Midttun, A., Sandquist, J., and Trømborg, E. (2021). The future role of forest-based biofuels: industrial impacts in the Nordic countries. Energies 14:2073. doi: 10.3390/en14082073
PAME (2019). Alternative fuels in the Arctic. Maritime Environment Advisory, Høvik, Norway. Report No.: 2019-0226, Rev. 0.
Papadogiannaki, S., Liora, N., Parliari, D., Cheristanidis, S., Poupkou, A., Sebos, I., et al. (2023). Evaluating the impact of COVID-19 on the carbon footprint of two research projects: a comparative analysis. Atmos. 14:1365. doi: 10.3390/atmos14091365
Peck, P. (2017) Socio-economic metrics for transport biofuels: a review. Report no 2017:09, f3 the Swedish knowledge Centre for Renewable Transportation Fuels, Sweden. Available at: www.f3centre.se.
Petrov, A. N., Welford, M., Golosov, N., DeGroote, J., Degai, T., and Savelyev, A. (2020). Spatiotemporal dynamics of the COVID-19 pandemic in the arctic: early data and emerging trends. Int. J. Circumpolar Health 79:1835251. doi: 10.1080/22423982.2020.1835251
Ramirez, J., Brown, R., and Rainey, T. (2015). A review of hydrothermal liquefaction bio-crude properties and prospects for upgrading to transportation fuels. Energies 8, 6765–6794. doi: 10.3390/en8076765
Røyne, F. (2016). Exploring the relevance of uncertainty in the life cycle assessment of Forest products. Umeå, Sweden: Umeå University.
SEA (2019). Kontrollstation 2019 för reduktionsplikten – Reduktionspliktens utveckling 2021–2030. Eskilstuna: Swedish Energy Agency.
Sebos, I. (2022). Fossil fraction of CO2 emissions of biofuels. Carbon Manage. 13, 154–163. doi: 10.1080/17583004.2022.2046173
Sebos, I., Nydrioti, I., Katsiardi, P., and Assimacopoulos, D. (2023). Stakeholder perceptions on climate change impacts and adaptation actions in Greece. Euro-Mediterr. J. Environ. Integr. 8, 777–793. doi: 10.1007/s41207-023-00396-w
Sebos, I., Progiou, A., and Kallinikos, L. (2020). Methodological framework for the quantification of GHG emission reductions from climate change mitigation actions. SPEE. 39, 3–4. doi: 10.13052/spee1048-4236.391411
Shiqi, L., and Liming, J. (2020). Review on sustainable development of forest-based biodiesel. J. Nanjing For. Univ. 44, 216–224. doi: 10.3969/j.issn.1000-2006.201811023
Smith, T. W. P., Raucci, C., Sabio, N., and Argyros, D. (2014). Global Marine Fuel Trends 2030. London, UK: Lloyd’s Register Marine /UCL Energy Institute.
Statista (2024a). Global pulp for paper production 2022, by country. New York, USA: Statista Research Department.
Statista (2024b). Employment in the biofuels industry in the European Union 2013–2022. New York, USA: Statista Research Department.
Tavera-Ruiz, C., Martí-Herrero, J., Mendieta, O., Jaimes-Estévez, J., Gauthier-Maradei, P., Azimov, U., et al. (2023). Current understanding and perspectives on anaerobic digestion in developing countries: Colombia case study. Renew. Sust. Energ. Rev. 173:113097. doi: 10.1016/j.rser.2022.113097
Tyrovola, T., Dodos, G., Kalligeros, S., and Zannikos, F. (2017). The introduction of biofuels in marine sector. J. Environ. Sci. Eng. A 6, 415–421. doi: 10.17265/2162-5298/2017.08.006
UNEP (2009) Towards sustainable production and use of resources: assessing biofuels. Available at: https://www.resourcepanel.org/file/560/download?token=04PkF6fe
US EPA (2010) Renewable fuel standard program (RFS2) regulatory impact analysis (Accessed June 09, 2024).
Von Blottnitz, H., and Curran, M. A. (2007). A review of assessments conducted on bio-ethanol as a transportation fuel from a net energy, greenhouse gas, and environmental life cycle perspective. J. Clean. Prod. 15, 607–619. doi: 10.1016/j.jclepro.2006.03.002
Westeson, J. (2022). ‘“Det var vi urfolk som uppfann den gröna omställningen!”’ [“It was us indigenous people who invented the green transition!”]. Sweden: Amnesty Sápmi.
Xiao, Y., and Watson, M. (2019). Guidance on conducting a systematic literature review. J. Plan. Educ. Res. 39, 93–112. doi: 10.1177/0739456X17723971
Keywords: Forest-based biofuel, socio-economic impacts, Arctic sustainability, marine shipping, stakeholders engagement, local indigenous communities, economic benefits, challenges and research gaps
Citation: Yacout DMM, Tysklind M and Upadhyayula VKK (2024) Socio-economic implications of forest-based biofuels for marine transportation in the Arctic: Sweden as a case study. Front. Clim. 6:1414813. doi: 10.3389/fclim.2024.1414813
Edited by:
Anjal Prakash, Indian School of Business, IndiaReviewed by:
Mark Mba Wright, Iowa State University, United StatesIoannis Sebos, National Technical University of Athens, Greece
Abhishek Joshi, Mohanlal Sukhadia University, India
Copyright © 2024 Yacout, Tysklind and Upadhyayula. This is an open-access article distributed under the terms of the Creative Commons Attribution License (CC BY). The use, distribution or reproduction in other forums is permitted, provided the original author(s) and the copyright owner(s) are credited and that the original publication in this journal is cited, in accordance with accepted academic practice. No use, distribution or reproduction is permitted which does not comply with these terms.
*Correspondence: Dalia M. M. Yacout, Dalia.abdelfattah@slu.se