- 1Departamento de Oceanografía Física, CICESE, Ensenada, Baja California, Mexico
- 2Universidad de Buenos Aires-Consejo Nacional de Investigaciones Científicas y Técnicas, Departamento de Ciencias de la Atmósfera y los Océanos, Buenos Aires, Argentina
- 3Instituto Franco-Argentino de Estudios sobre el Clima y sus Impactos (IFAECI - IRL 3351), CNRS-CONICET-IRD-UBA, Buenos Aires, Argentina
- 4Department of Geography and Geology, The University of the West Indies, Mona, Jamaica
- 5University of California, Riverside, CA, United States
- 6Department of Geomatics Engineering and Land Management, Faculty of Engineering, The University of the West Indies, St. Augustine, Trinidad and Tobago
- 7Grupo de Ingeniería y Gestión Ambiental (GIGA), Escuela Ambiental, Facultad de Ingeniería, Universidad de Antioquia, Medellín, Colombia
- 8Instituto Argentino de Nivología, Glaciología y Ciencias Ambientales (IANIGLA), Centro Científico Tecnológico CONICET Mendoza, Mendoza, Argentina
- 9Instituto de Recursos Naturais, Universidade Federal de Itajubá, Itajubá, Minas Gerais, Brazil
- 10Universidad de Buenos Aires, Facultad de Ciencias Exactas y Naturales, Departamento de Ciencias de la Atmósfera y los Océanos (DCAO), Buenos Aires, Argentina
- 11CONICET – Universidad de Buenos Aires, Centro de Investigaciones del Mar y la Atmósfera (CIMA), Buenos Aires, Argentina
- 12Escuela de Física, Centro de Investigaciones Geofísicas y Centro de Investigación en Matemática Pura y Aplicada, Universidad de Costa Rica, San José, Costa Rica
- 13Escuela de Física, Centro de Investigaciones Geofísicas y Centro de Investigación en Ciencias del Mar y Limnología, Universidad de Costa Rica, San José, Costa Rica
- 14Department of Physics, The University of the West Indies, Mona, Jamaica
- 15Facultad de Ciencias Exactas y Naturales, Universidad de Buenos Aires, Buenos Aires, Argentina
- 16Instituto de Ingeniería, Universidad Nacional Autónoma de México, Sisal, Yucatán, Mexico
- 17Interamerican Institute for Global Change Research (IAI), Montevideo, Uruguay
- 18Centro de Estudios Ambientales y de Biodiversidad, Universidad del Valle de Guatemala, Guatemala City, Guatemala
- 19Comisión Económica para América Latina y el Caribe (CEPAL / ECLAC), Ciudad de México, Mexico
- 20The Caribbean Institute for Meteorology and Hydrology, Bridgetown, Barbados
The limited success of international efforts to reduce global warming at levels established in the Paris Agreement, and the increasing frequency and strength of climate impacts, highlight the urgent need of adaptation, particularly in developing countries. Unfortunately, current levels of adaptation initiatives are not enough to counteract the observed impacts and projected risks from climate change in Latin America and the Caribbean (LAC). In this paper, we review and highlight relevant issues that have limited the capacity to transform climate knowledge and parties’ ambitions into action in the region. Current vulnerabilities and climatic impact-drivers in LAC are diverse, complex, and region-specific and their effects are expected to be exacerbated by climate change. However, the advancement of regional and domestic climate agendas has been hindered by scientific gaps, political support, institutional capacity, and financial, technical, human, and economic limitations that are common to many LAC countries. Transforming climate data into multidimensional metrics with useful thresholds for different sectors and understanding their contribution for feasible adaptation strategies are delayed by regional and local conundrums such as lack of inclusive governance, data availability, equity, justice, and transboundary issues. We discuss ways to move forward to develop local and regional climate resilient development actions and a more sustainable future in LAC. The climate science community in LAC needs to strengthen its local, national, and international connections and with decision/policymakers and society to establish a three-way engagement by proposing suitable adaptation actions and international negotiations to reduce the risks and vulnerability associated with climate extremes, climate variability and climate change in the region. The discussions and insights presented in this work could be extrapolated to other countries in the Global South.
1 Introduction
Human-induced climate change has already emerged as a millennium challenge for most countries, particularly those in the Global South, including Latin America and the Caribbean (LAC) region. The significant increase of global surface air temperature and ocean warming pose a major threat to life on our planet, as recent extreme weather and climate events and changes in large-scale atmospheric circulation patterns have multiplied regional and local climatic risks. Most ecological systems require substantial time to adapt, often spanning decades or longer. However, some systems have already reached their limits for adaptation, while many others will be in danger at 1.5°C of global warming (Travis et al., 2018; IPCC, 2023). Societies are not far from this threshold as evidence shows that the mean global surface temperature has increased 1.1°C in 2011–2020 relative to the 1850–1900 preindustrial period (IPCC, 2021). Moreover, the global mean temperature in 2023 was the highest on record, 1.48°C above the pre-industrial average (Copernicus, 2024); and ocean heat content and sea surface temperature (SST) anomalies also reached unprecedented high records (Cheng et al., 2024). The same year, tropical regions across South America experienced the longest number of days with temperatures that were strongly attributable to climate change, while Jamaica and Guatemala experienced temperatures that were more than four times more likely produced by climate change (Wong, 2023). Observed temperature trends in LAC in the last few decades are widespread; in many areas the warming ranges between 0.2°C and 0.3°C/decade (Hidalgo et al., 2013, 2017, 2019; Jones et al., 2016; Rao et al., 2016; Alfaro-Córdoba et al., 2020; Cavazos et al., 2020; Dereczynski et al., 2020; Almazroui et al., 2021; Gutiérrez et al., 2021; Reboita et al., 2022; Stephenson et al., 2022; Avila-Diaz et al., 2023; Gu and Adler, 2023), but with differentiated impacts in subregions, sectors, and ecosystems (Galindo et al., 2015; de Lacerda et al., 2019; Muelbert et al., 2021; Castellanos et al., 2022; Reboita et al., 2022). Without urgent mitigation action, global temperature may continue to rise to more than 2°C above the preindustrial period by the middle of this century or earlier (IPCC, 2021, 2023). Thus, any solution requires immediate actions on both mitigation and adaptation strategies (Galindo et al., 2015; IPCC, 2021) to avoid soft and hard losses and damages (Hagen et al., 2022; Ley et al., 2023; UNEP, 2023a) which not only include economic losses, but also people’s values and belief systems.
The diverse geography and ethnic, social, and economic inequalities in LAC combined with the large exposure to weather and climate-related hazards enhance differentiated vulnerabilities in the region. Between 1998 and 2017, 53% of global economic losses from climate-related disasters occurred in LAC, suggesting that regional efforts to adapt still have a long way to go. As an example, climatic risk prevention should be incorporated into the planning of public and private investments (e.g., UNDRR, 2021) as soon as possible to increase equitable socio-ecological resilience.
The aim of this paper is to review and discuss major challenges for adaptation to climate change in the LAC region, which have delayed the proposal and implementation of suitable actions and regional solutions for long-term adaptation. Section 2 briefly describes some of the main climatic impact-drivers (CIDs) in LAC and their regional impacts under current and future climate change conditions. Section 3 presents scientific gaps and commonalities in observed and future climatic information that have blocked regional advances in monitoring and preventive actions; it also emphasizes some inadvertent biases that have diminished the recognition of local and regional climate science and scientists. Section 4 discusses a series of adaptation conundrums driven by the diversity of population, ecosystems, governance, migration, and environmental and social finance barriers in the region. We conclude by discussing possible actions to move forward in LAC to have a more climate resilient society and environment.
2 Climatic impact-drivers (CIDs) in LAC
Changes in CIDs can have detrimental repercussions but may also provide potential opportunities depending on the anomalous conditions of a weather or climate phenomenon and its interaction with regional factors; therefore, CIDs are important elements for climate change adaptation strategies (IPCC, 2021; Ranasinghe et al., 2021; Ruane et al., 2022).
Some regions in LAC have experienced sea level rise and increased frequency of droughts, heat waves and heavy precipitation, and other CIDs such as floods, landslides, and wildfires, affecting livelihoods, infrastructure, and access to services (Herrera and Ault, 2017; Arias et al., 2021; Ranasinghe et al., 2021; Seneviratne et al., 2021; Spinoni et al., 2021; Trotman et al., 2021; Adler et al., 2022; Castellanos et al., 2022; Mycoo et al., 2022). Heat stress also poses a major human health challenge and danger in LAC (e.g., Vargas and Magaña, 2020; Di Napoli et al., 2022; Vanos et al., 2023), and even mortality risk in major urban centers (Bell et al., 2008). Humid heat is more intense than previously reported and increasingly severe, particularly in some coastal cities of LAC (Raymond et al., 2020). In addition to these, endemic and emerging climate-related infectious diseases are projected to increase with global warming (Castellanos et al., 2022).
At the same time, it is important to consider non-climatic drivers that create shortcomings and barriers to climate change adaptation (Gotlieb and García-Girón, 2020). Increased risks to extreme CIDs occur particularly in vulnerable and exposed human and natural systems. This is the case of the Caribbean Islands (Lincoln, 2017; IPCC, 2022a), where approximately 22 million people live at 6 m or less above sea level (Cashman and Nagdee, 2017), and in densely populated urban centers (Estrada et al., 2023), where 76% of the population live in informal settlements (UNDRR, 2021; WMO, 2023). Latin America is one of the most urbanized regions of the world; thus, it has a large population at risk of urban heat island exposure (Vargas and Magaña, 2020; Tuholske et al., 2021). Other communities at risk are those located on hillsides and canyons prone to landslides and floods (Sepúlveda and Petley, 2015), or impoverished communities that face limited access to basic services and infrastructure (IPCC, 2022a), such as clean water and sanitation (Romano et al., 2021) and depend on climate sensitive land use activities, such as agriculture (Ocampo-Melgar et al., 2023) and forest ecosystem services (Gotlieb and García-Girón, 2020; Arenas-Wong et al., 2023). Floods and droughts in some South American equatorial and tropical river basins have already intensified, particularly in the Amazon (home of the largest rainforest in the world), where agriculture, cattle-raising and mining compete for finite natural resources and have aggravated deforestation and forest fires. These activities, in particular deforestation, contributed to a peak in GHG emission levels in 2020 (dos Santos et al., 2024). These changes have affected biodiversity and the stability and survival of this key ecosystem and have threatened the livelihoods of local and Indigenous Peoples (Lapola et al., 2020). Moreover, droughts are a continuous hazard in regions with relatively high climatological aridity, such as large parts of Mexico and Central America (Méndez and Magaña, 2010; Andrade-Gómez and Cavazos, 2024), the Central American Dry Corridor (Hidalgo et al., 2019; Gotlieb and García-Girón, 2020; Anderson et al., 2023), Brazil (Freitas et al., 2021, 2023), the Orinoco River basin (Paredes-Trejo et al., 2023), Chile (Garreaud et al., 2019), and the Central Andes (Morales et al., 2023).
With climate change, some existing CIDs in LAC are evolving to create compounding risks and cascading impacts for which local communities, Indigenous population, and urban centers are not yet prepared. Impacts can threaten the water-food-energy security (Ridder et al., 2022), as well as the social, economic, and political stability in the region, mainly due to systemic vulnerabilities, including poverty, marginalization, and poor or lack of governance and finance (Ryan and Bustos, 2019; IPCC, 2022a). For example, intense hydrological and socioeconomic droughts at the peak of the summer season have exerted significant pressure on water, agriculture, and energy resources in large cities. These droughts (linked to compound multidimensional and multiscale feedback processes) are governed by the combination of natural rainfall variability and teleconnections (mainly El Niño-Southern Oscillation (ENSO) events), climate change, human decision activities, increased urban population, and altered microclimate conditions due to changes in land use, and water management (AghaKouchak et al., 2021). Moreover, recent drought events in Brazil (Silva et al., 2015; Van Loon et al., 2016), Central America (Anderson et al., 2023), and Mexico (Magaña et al., 2021) are largely attributed to a suite of human activities, such as deforestation, urbanization, lack of integrative planning and basin management, and monitoring and evaluation (e.g., Downs et al., 2022); these factors have created surface water and groundwater exploitation, water scarcity, sectoral competition over water, and conflicts (Battistello Espíndola and Costa Ribeiro, 2020). With global warming, droughts may even emerge in wet regions such as northern South America and the South American monsoon region (Spinoni et al., 2020; Pascale et al., 2021; Seneviratne et al., 2021), exacerbating current and future risks (Hidalgo and Alfaro, 2012; Garro-Quesada et al., 2023; WMO, 2023).
On the other hand, convective rainfall, and tropical cyclones (TC) and hurricanes are expected to intensify with warming of the oceans (Emanuel, 2021) in response to natural variability and human greenhouse gas (GHG) emissions (Sobel et al., 2016). Currently, there is a record high of the heat ocean content of the upper 2000 m of the oceans (Cheng et al., 2024). Particularly worrying for our region is the increase of 1.24 GJ/m2 in the ocean heat content in 2023 relative to 1981–2010 in the Gulf of Mexico and the tropical North Atlantic in the main TC development region of this basin. Weak TCs have already intensified in all oceans during 1991–2020 (Wang et al., 2022). Observed warming in the North Atlantic basin and the Gulf of Mexico have resulted in a slower decay of TCs (Li and Chakraborty, 2020) and increased duration of hurricanes producing heavy rainfall and strong winds in the southern United States, the Caribbean Islands, Central America, and Mexico. In October 2023, Acapulco, in the southern Pacific coast of Mexico, was severely affected by hurricane Otis, the first Pacific TC to make landfall as Category 5, surpassing Hurricane Patricia in October 2015, as the strongest landfalling Pacific hurricane on record. The rapid intensification of Otis from a tropical storm into category 5 in just 12 h took forecasters and society by surprise, thus there was no early warning (Appendini, 2024). Hurricane Otis is currently the top climate and weather-related disaster in Mexico ($15.1 billion USD in damages) after Hurricane Wilma in 2005 (Sánchez Rodríguez and Cavazos, 2015; Gallagher, 2024). Additional to the rapid intensification of the TCs (Bhatia et al., 2019) is the large uncertainty of the possible changes of the trajectories and landing positions, as most impacts are not necessarily associated with strong hurricanes (e.g., Hidalgo et al., 2020, 2022).
Warming of the Pacific and Atlantic oceans in the last three decades (Bhatia et al., 2022; Li Z. et al., 2023) in combination with natural variations, such as ENSO, the positive phase of the Atlantic Multidecadal Oscillation (AMO), and other climate teleconnections have favored regional changes in TCs (e.g., Wang et al., 2008) and in physical processes conducive to convective systems and extreme precipitation events, such as atmospheric rivers and low-level jets (e.g., Gimeno et al., 2016; Montini et al., 2019) and tropical-extratropical interactions (e.g., Cavalcanti, 2012). Interannual climate variability in LAC is strongly associated with ENSO in different ways, due to thermally direct changes and atmospheric teleconnections (e.g., Cai et al., 2020; Durán-Quesada et al., 2020; Luna-Niño et al., 2020; Ley et al., 2023). In Central America, the recent 2019–2023 La Niña events brought extremely wet summer conditions as expected, while the 2023 El Niño condition was forecast to produce drier than normal conditions, but instead the unusually warm Caribbean and Atlantic oceans contributed to atypical wet conditions in some parts of the region. In contrast, the three consecutive La Niña events of 2019–2023 (Li X. et al., 2023) have been linked to drought in central South America (Argentina, Brazil, Uruguay, Paraguay, and Bolivia; Geirinhas et al., 2023; Arias et al., 2024). Moreover, a recent study by the World Weather Attribution team concluded that the severity of the 2023 droughts in the Amazon basin, which affects several countries, was entirely related to increased global temperatures and not to the 2023 El Niño event. Together, the droughts in Argentina and Brazil are considered as the third global natural-related disaster in 2023 (with $16.4 billion USD in economic losses) followed by Hurricane Otis in Mexico in the fourth position (Gallagher, 2024). At the same time, in the last 3–4 years, most of the LAC region has been affected by severe droughts, as it can be seen in the maps of the Global Drought Monitor, which in some regions were exacerbated by the occurrence of heat waves induced by anthropogenic climate change (Kew et al., 2023; Rivera et al., 2023; Arias et al., 2024). This shows the necessity of disentangling the interplay between human-induced climate change and natural climate variability not only to understand the physical processes, but also to adequately project the impacts of regional climate change in LAC and to propose suitable adaptation actions.
In this section we highlighted some examples of severe negative impacts of climate variability and climate change in LAC, but many CIDs and their changes may also produce benefits, particularly when using early warning systems (e.g., Adams et al., 2003) and seasonal forecasts, such as in agriculture and the water sector. Also, rainfall from the tails of TCs, atmospheric rivers, and other convective systems serve to fill dams and relieve temporarily drought conditions in many regions (e.g., Boyd and Ibarrarán, 2009; Martinez et al., 2015).
Regional capacities produce weather, seasonal and subseasonal forecasts, historical climate information and climate change scenarios, but there is still the need for more integrative programs and co-production of knowledge to reduce risks and vulnerability, prevent disasters, and develop adaptation responses to increase our opportunities for a better future (Figure 1). For this, it is necessary to act in a synergistic way as countries or regions; as a first step, and from the CIDs perspective, it is very relevant to increase the financial support for weather and climate services to enhance weather and climate monitoring, improve and increase rapid response plans, early warning systems, communication to society, and drought and flood contingency plans. Other relevant actions are to augment the availability and transparency of historical climate data and operational information platforms, similar to the North American Drought Monitor and the Global Drought Monitor, and to translate historical and future climate information into useful metrics for different sectors to avoid or to reduce risks and unintended consequences.
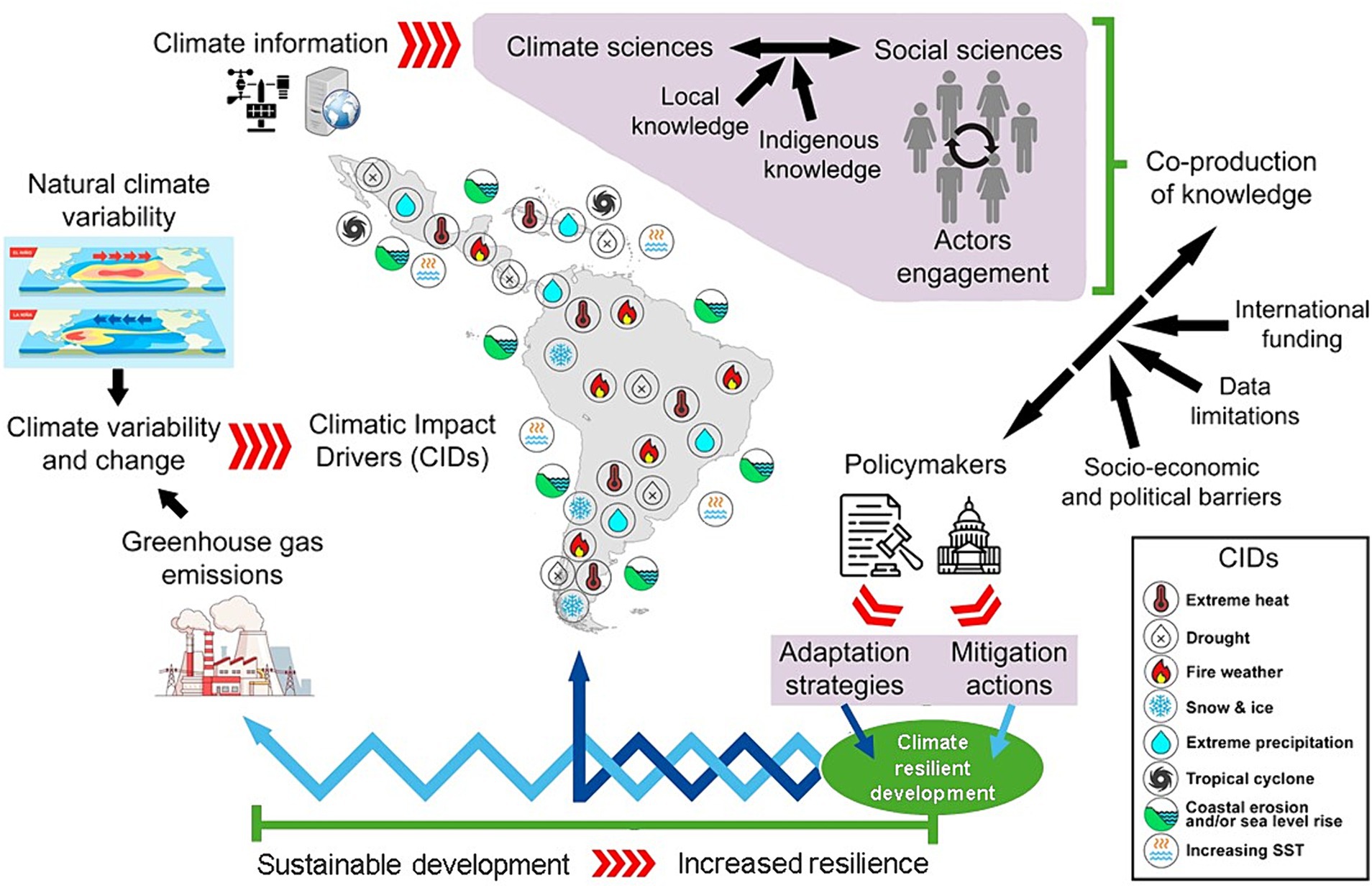
Figure 1. Schematic diagram of different aspects related to the interplay of climate adaptation and mitigation actions for a climate resilient development. The simplified depiction of some current CIDs in the LAC region is based on different studies (e.g., SOCC, 2020; Hagen et al., 2022; IPCC, 2022a; Avila-Diaz et al., 2023; Ley et al., 2023).
3 Gaps and commonalities
There are several factors that are common to most LAC countries that have hindered the advancement of the climate science agenda in the region. Moreover, the recognition and visibility of what is achieved and can be achieved by local climate scientists is affected by regional, national, and global socioeconomic and geopolitical processes. In this section we underscore some gaps faced and commonalities experienced by research communities and academic sectors in LAC countries, when analyzing the physical processes related to CIDs and their changes, and when assessing the regional impacts that may affect and shape adaptation actions.
3.1 Observational and data constraints
The lack of sufficient observational climatic and weather data is a common issue across the LAC countries mainly due to weak monitoring network infrastructures and resources. Some regions are characterized by low density, or in some cases, a lack of in situ observations (Mahon et al., 2019; Quesada-Montano et al., 2019; Condom et al., 2020; Balmaceda-Huarte et al., 2021; Sáenz et al., 2023) even for the most common climatic variables such as precipitation and air temperature. This situation is even worse for other relevant variables, such as aerological measurements (radiosonde observations), coastal sea level, SST, surface soil moisture, and solar radiation. Data at a fine temporal scale (for instance, sub-daily data from automatic stations) is scarce and intermittent. Low density of in situ observational data records are partially linked to the demographic distribution of the regions. This is the case of low population density areas, such as the high Andes, the Orinoco basin, the Brazilian Pantanal, deserts, and mountain and forest regions of Mexico and Central America. However, even in densely populated areas, such as large cities, some key observations for addressing the impacts of different CIDs (e.g., air pollution, extreme weather conditions, fires, droughts) are still lacking. Sometimes the data exists but it is not easily accessible due to different constraints: many LAC national weather services do not have open data policies; the data is not quality controlled, or it is not completely digitized; in other cases, measurement and collection of data are done by academic institutions or private companies (for example, cereal, coffee, banana or sugar cane consortiums, and mining companies) that do not necessarily follow the protocols and the standards of the World Meteorological Organization (WMO).
Some efforts to increase observational data and knowledge have been carried out in different field campaigns and international programs related to specific phenomena of regional interest, such as RELAMPAGO (Remote sensing of Electrification, Lightning, And Meso-scale/micro-scale Processes with Adaptive Ground Observations); CACTI (Clouds, Aerosols, and Complex Terrain Interactions); NAME (North American Monsoon Experiment) and MESA (Monsoon Experiment South America) (Vera et al., 2006); CLARIS LPB (Europe-South America Network for Climate Change Assessment and Impact Studies in La Plata Basin); OTREC (Organization of Tropical East Pacific Convection; Fuchs-Stone et al., 2020; Huaman et al., 2022); and the EUREC4A (Elucidating the role of clouds-circulation coupling in climate; Bony et al., 2017). These projects are mainly linked to international initiatives fostering networking and collaborations which are key to gathering and organizing the available data for a specific period and phenomenon. However, there are significant obstacles to sustaining the instrumentation from these efforts after the conclusion of such projects.
As in situ observations are not always spatially and temporally available and sufficient for climate variability analyses, other state-of-the-art products from multiple sources (radar, satellite data, satellite merged with station data, and reanalysis) are commonly employed in climate analyses and regional model intercomparisons and model evaluations (e.g., Cerezo-Mota et al., 2016; de Lima and Alcântara, 2019; Centella et al., 2020; Bettolli et al., 2021; Colorado-Ruiz and Cavazos, 2021; Stewart et al., 2022; Avila-Diaz et al., 2023). However, it is important to understand their advantages and limitations. For instance, satellite estimates involve uncertainties associated with instrument calibration and sensor limitations, cloud cover, data processing, and algorithms used (Funk et al., 2015; Beck et al., 2017; Dorigo et al., 2017). Reanalysis data are affected by similar inhomogeneities identified in situ observations (in spatial patterns and temporal evolutions), which may be related to different parameterizations, spatial resolution, processes involved in data assimilation, as well as to model uncertainty, particularly in the Southern Hemisphere (Hegerl et al., 2015; Parker, 2016; Schröder et al., 2019). Reanalyzes are meant to complement observations, not to substitute near-surface (hydro) meteorological station data and radiosonde observations, but network density has been significantly reduced in recent decades in some LAC countries (Quesada-Montano et al., 2019; Sáenz et al., 2023). Nevertheless, the spatial and temporal continuity and temporal resolution of these alternative datasets are very important for comparison purposes and evaluation of dynamic variables of regional and global climate model simulations. The availability of continuous and dense local observational datasets in LAC are still very relevant to improve and to evaluate reanalyzes, weather forecasts, and to assess climate change projections.
3.2 Socioeconomic data
Climate adaptation is context specific and relies heavily on accurate, localized, and timely data. In many parts of the LAC region, there is a scarcity of reliable climate and socioeconomic data at national and subnational levels, impeding the development of adequate and localized vulnerability assessments and adaptation strategies (Eakin et al., 2014; UNEP, 2022). Expanding and improving knowledge on climatic impacts in different sectors and for vulnerability and adaptation actions requires a better understanding of sociodemographic, economic, and biophysical conditions at the local level. Although there are differences in sociodemographic and economic data collected through national censuses, a common element is that the national census is the most comprehensive and reliable source available for this kind of information. Unfortunately, a census is designed based on twenty century needs and neglects 21st century problems like climate change, because it only includes a limited number of variables relevant in the analysis of climate vulnerability and impacts. The national census also has limitations to provide data at the local level needed in the analysis of vulnerability and adaptation. Data collection through individual projects (e.g., atlas of vulnerability, weather, and climate hazard risk atlases) has been done in several countries of the region on an ad hoc basis, but there is no coordinated effort to create cumulative and open data repositories that could support future more ambitious research at regional and local (municipal/provincial) scales.
3.3 Climate assessments
There are national and international efforts to report the state of climate in the region such as the recent series of the State of the Climate in Latin America and the Caribbean of the WMO (2022, 2023) and the yearly State of the Climate report published in the Bulletin of the American Meteorological Society since 2011, which includes the participation of local scientists and National Weather Services (known in Spanish as Servicio Meteorológico Nacional, SMN). Many SMN have also produced climate reports and bulletins; for example, the Mexican SMN has monthly and annual climate reports (Reporte del Clima de México) since 2011; Argentina has annual reports since 2021 (El Clima en Argentina) and a climate atlas (Atlas Climático de Argentina); and Brazil has monthly agroclimatological bulletins (Boletim Agroclimatológico Mensal); Costa Rica has monthly and/or seasonal bulletins continuously since 1977 (available online since 2005), and the Caribbean has the State of the Caribbean Climate Report (SOCC, 2020). These reports highlight the most important CIDs and their regional impacts and risks, which are very relevant when doing research on historical hazards, vulnerability, and adaptation. Some IPCC focal country points have also produced national climate assessments in response to the decisions of the Conference of the Parts (COPs) of the United Nations Framework Convention of Climate Change (UNFCCC); since these documents are written in national languages (e.g., Sietsma et al., 2023), they are not necessarily taken into account in some reports, such as those of the IPCC, particularly if local scientists are not authors or contributors of the reports. The IPCC focal points have also coordinated the National Communications that are reported to the UNFCCC; in Mexico the focal point has coordinated State Climate Change Action Programs (Programa Estatal de Acción Ante el Cambio Climático, PEACC) and National Climate Strategies. Most of these are focused on mitigation and climate change scenarios, while some also include adaptation actions (e.g., PEACC-BC, 2012). Present and future atlases of hazards, danger or risks, and vulnerability (e.g., Maynard-Ford et al., 2007; Monterroso Rivas et al., 2014; Sepúlveda and Petley, 2015; Herrera and Ault, 2017; SOCC, 2020; Coppola et al., 2021; Di Napoli et al., 2022; Hagen et al., 2022; Avila-Diaz et al., 2023; Estrada et al., 2023) are very important during the integrative process for finding solutions to adaptation actions, but they are needed at the state and municipal/regional level, and need to be dynamic, as vulnerability changes with time. Two good examples are (1) the AdaptaBrasil MCTI platform that contains climatic risks, vulnerability, and exposure information for strategic sectors at municipal level (Ometto et al., 2021); and (2) MEGADAPT-Mexico City, a dynamic multi-scalar adaptation framework for transforming the socio-hydrological risk of this megalopolis (Bojórquez-Tapia et al., 2021); it has a decision-support tool that allows decisionmakers to test different risk outcomes under climate scenarios.
The Assessments Reports (AR) of the Intergovernmental Panel on Climate Change (IPCC) are a primary source for understanding regional climate changes in the global context. The last report, the AR6 (IPCC, 2021) defined a set of broad regions (Iturbide et al., 2020) with the purpose of summarizing global aggregated ensembles of climate change scenarios with a fair climatic consistency. However, these broad areas often cover several climate types in a single IPCC region. In this context, some observed trends and attribution of specific CIDs, such as those shown in the AR6 and its Atlas (Gutiérrez et al., 2021), may show a low confidence due to the combination of contrasting climatic regions, which are not necessarily representative at finer scales. Central America, the Caribbean Islands, and locations near mountain regions are examples of contrasting climate regimes in leeward and windward slopes, and land-sea influences. Some of the spatial results shown in the Atlas are an initial effort to include regional (downscaled) climate information at finer scale resolution with information derived from CORDEX (Coordinated Regional Downscaling Experiment), but their summaries are still averaged over the large IPCC regions. To overcome this kind of biases and to make climate information available in maps and at grid point scale, the Copernicus Interactive Climate Atlas was launched in February, 2024; it includes a large number of atmospheric variables from global observations, reanalyzes, regional projections from CORDEX and global projections from the Climate Model Intercomparison Project (CMIP) phases 5 and 6 (Diez-Sierra et al., 2022). Thus, it is up to the LAC climate and social scientists to produce timely and comprehensive regional climate risk assessments and publish articles useful for adaptation strategies using this and other information available for the region.
3.4 Regional climate information from statistical and dynamical downscaling models
IPCC Working Group I of the AR6 addressed methodologies to construct regional climatic information in its Chapter 10, stressing that a regional focus is necessary to properly inform risk assessment and adaptation actions (Doblas-Reyes et al., 2021). Tailored climate information needed for impact assessment is closely linked to regional climate modeling, either using dynamical regional climate models (RCM) or empirical statistical models (ESD) to downscale global climate model simulations. The CORDEX of the World Climate Research Programme (WCRP) is the first global effort to tackles this problem by coordinating the science and application of regional climate downscaling through global partnerships using a common experimental framework (Giorgi and Gutowski, 2015). The research communities greatly benefited from the historical and future climate change projections developed in these coordinated experiments. However, most of these RCM simulations were conducted by research centers in the Global North and only a few were conducted in LAC institutes by LAC researchers, particularly in Brazil and Argentina. The large computational resources required to run and store the model simulations and the lack of sufficient funding and skilled and dedicated experts in numerical modeling are some of the obstacles. In this regard, the activities led by the International Centre for Theoretical Physics (ICTP) and CORDEX in collaboration with LAC CORDEX scientists have been key for building capacity for advancing regional climate modeling studies (e.g., support for students and young scientists visits to the ICTP and co-organization of regional online or hybrid workshops in different countries of LAC). These activities reinforced the co-production of knowledge among LAC climate modeling groups. Another example is the activities of the South American and Mesoamerican Affinity Groups led by the National Center for Atmospheric Research (NCAR), where research groups of the United States and LAC are brought together to co-design high-resolution climate experiments over different regions of LAC. High spatial resolution climate experiments using convection permitting modeling (e.g., Prein et al., 2015; Bettolli et al., 2021; Dominguez et al., 2024) are needed to better understand the impact of weather and climate events at local scale (at few kilometers or less), particularly extreme events.
3.5 Publication biases and funding barriers
The lack of sufficient funding for research and computing capabilities is a common constraint across the LAC countries, which is partially tied to the low budget assigned to science and development (S&D) by the governments (58% of the funding comes from the government and performed primarily by academic institutions, UNESCO, 2021). According to UNESCO, most LAC countries dedicate less than 0.7% of their Gross Domestic Product to S&D, which also affects the possibilities for publishing scientific research and local knowledge in high impact and open access journals (e.g., Carbon Brief, 2021; Castro-Torres and Alburez-Gutierrez, 2022; Ahmed et al., 2023). The exorbitant cost of the article processing charges (APC) of the open data system benefits mainly the Global North, where there is a much larger access to a variety of research funds than in the Global South. Even in the Global North there have been complaints and protests for the excessive APCs (Sanderson, 2023).
The language barrier emerges as an additional limitation for the regional scientific literature to be used as evidence in the IPCC assessments; for example, many local and regional studies on climate change with focus on LAC are written in Spanish or Portuguese, although most of them are in peer reviewed journals. Some of the scientific literature related to CIDs is written in local languages partly to communicate results and actions to decision and policymakers. In fact, a recent analysis of the 13,500 citations assessed by the IPCC Working Group I (WG I) of the AR6 shows that 99.95% of the cited references are written in English and 75% of all the literature cited featured at least one author based in either the United States or the United Kingdom (Carbon Brief, 2023). This problem is directly linked to the weak representation of LAC scientists and the lack of regional diversity of the authors selected to assess the IPCC reports, who if selected could bring in peer-reviewed literature related to more LAC countries and some in local languages (Gay-Antaki, 2021; Liverman et al., 2022). The selection process relies on the nomination of possible authors by each country focal point to the IPCC, but this process is generally weak in the Global South countries, such as in LAC, where the top-down information does not flow properly. Regional climate scientists should be informed by other scientists participating in the IPCC reports to avoid dependency and biases from the focal points. The formation of regional networks that can work with national focal points would help to keep the information flow during the IPCC selection process (for example, from which contributing authors can be drawn) is another approach that may offer value and more diverse authorship representation across LAC countries.
A strategic plan with regional focus and engaging regional communities is essential to enhance knowledge, understanding, and dissemination of climate change information, impacts and adaptation in LAC.
4 Adaptation conundrums
The LAC region is facing profound challenges in terms of climate change adaptation actions due to the complex and interactive effects of weather and climate variations (CIDs) on society, the economy, and ecosystems (Guillén Bolaños et al., 2022; Vignola et al., 2022) and the high levels of vulnerability observed in the region primarily arising from poverty and inequality issues (Castellanos et al., 2022). The IPCC (2022a) considers that even under low-emission scenarios, the region can expect more severe climate risks before the end of this century, but evidence mentioned in section 2 suggests these risks are occurring much sooner. International efforts have failed to reduce GHG emissions up to now, and there is no clear indication the Paris Agreement goal to limit global warming to 1.5°C or 2°C by 2,100 will be met (UNEP, 2023b). Current trends indicate extreme events will become more frequent and intense in coming decades (IPCC, 2023) strengthening the need to reduce emissions and vulnerability and speed up and expand adaptation response. While some countries across the region have made progress in adaptation plans (Castellanos et al., 2022; Mycoo et al., 2022; dos Santos et al., 2024), with clear priority areas (agriculture and food security, water, health, and ecosystems) (WMO, 2023), operational actions at local, regional, and national scales are urgently needed to keep pace with escalating climate risks and impacts (e.g., WCRP, 2024). Simply put, adaptation progress across the region is “too little and too slow” (UNEP, 2022; Sietsma et al., 2023). IPCC AR6 identifies that actions on adaptation have been uneven, slow and with a growing divergence between the adaptation actions taken and what is needed, particularly among lower income populations (IPCC, 2022a). The report identifies additional shortcomings in adaptation: first, a persistent gap between planned adaptations and actual implementation efforts; and second, the observed adaptations are fragmented (e.g., Ryan and Bustos, 2019), operating at a small scale, in sector specific, and designed to respond to short-term impacts but neglecting long-term risks. IPCC also highlights that adaptation options diminish and their cost increases with any increase in global warming and that the window of opportunity to secure a safe planet is small and closing rapidly. Additional challenges identified are that actions related to mitigation and adaptation are addressed separately rather than interdependently, thus ignoring the synergies and trade-offs between them and the systemic nature and transdisciplinary connections that are required to integrate the co-production of knowledge for climate action responses (Figure 1).
How can the region achieve effective adaptation at the scale and pace required to deal with the climate crisis? Apart from the needs and obstacles already mentioned in sections 2 and 3, here we highlight other adaptation challenges facing the LAC region and present ideas around five themes to stimulate future research and actions: (1) strengthening adaptation governance processes and priorities, (2) improving access to adaptation finance, (3) assessing adaptation limits, loss, and damage, (4) promoting transboundary adaptation and understanding migration, and (5) measuring adaptation progress.
4.1 Adaptation governance
Fragmented governance, insufficient institutional capacity, a lack of actor participation, especially the most vulnerable and marginalized, and weak transdisciplinary collaboration obstruct comprehensive adaptation initiatives across the LAC region (Ryan and Bustos, 2019; Castellanos et al., 2022; Cisneros et al., 2024). Effective adaptation governance requires a clear understanding of local needs, vulnerabilities, and the synergies between national and sub-national adaptation actors and policies. Integrated governance frameworks, which promote coordination across sectors and scales, can better address the multidimensional and interconnected challenges of climate change (Biesbroek et al., 2013). Multiple policy endeavors coexist, but the synergy between them often lacks robustness, leading to fragmented and competing actions and outcomes (Nagy et al., 2019). For instance, policies formulated at the national level frequently lack alignment with regional or local strategies and human, economic and financial resources, thereby creating implementation bottlenecks. At the same time, local vulnerabilities and adaptation needs are often not recognized in national adaptation policies and strategies. Improving multidimensional governance in Latin American countries requires structural changes in institutional culture and operation, and political structures. This is a chronic problem in many developed and developing countries that is difficult to manage (e.g., Fila et al., 2024), but climate change illustrates the urgent need to address it.
Historically, the LAC region has grappled with a variety of socio-political challenges resulting in widespread poverty and inequality that play a role in shaping existing governance conditions. The inherently diverse geographical expanse, coupled with socio-economic disparities, requires a response approach to governance that is both integrative and nuanced (Magrin et al., 2014). Diverse actors ranging from government entities, non-governmental organizations (NGOs), business organizations, academic institutions, indigenous communities, and local communities have different priorities and perspectives. However, the absence of a centralized, collaborative platform can result in misaligned actions, thereby diluting the efficacy of adaptation efforts (Vicuña et al., 2018). The LAC region is home to many Indigenous Peoples and offers a repository of ecological knowledge (Ladio, 2017). However, governance frameworks often overlook this Indigenous wisdom and local knowledge, thereby missing out on invaluable insights and perspectives that could shape, refine, and improve the effectiveness of adaptation strategies and which could be lost (Brondízio et al., 2016; Iwama et al., 2021). Addressing these governance challenges involves fostering cross-sectoral collaborations, promoting actor inclusivity, institutional capacity building, and integrating both contemporary and Indigenous knowledge systems.
In some countries, challenges in adapting to climate change are related to low climate literacy among decision-makers, lack of meaningful community engagement, and limited access to funding for policy and project implementation. Shortcomings in institutional capacity in local governments and communities are a barrier assessing vulnerability and designing and implementing effective and efficient adaptation actions (e.g., Ryan and Bustos, 2019). Institutional strengthening is essential to avoid fragmented and silo approaches to adaptation, reducing the risk of maladaptation.
The lack of strong governance in urban planning across many cities in LAC has contributed to increased urban sprawl and informal settlements, which are pushing these areas to their adaptation limits (Mycoo, 2018a,b). Nevertheless, private property owners and developers have significant control over the selection and implementation of urban adaptation measures (Petzold et al., 2023).
4.2 Adaptation finance
Most governments and climate experts in LAC have identified inadequate financing as a significant barrier to adaptation in their Nationally Determined Contributions (NDCs) and National Adaptation Plans (NAPs) (Castellanos et al., 2022; Mycoo et al., 2022; Mohan, 2023). Effective utilization of financial resources is pivotal for implementing adaptation actions. However, the disparity between required funds and available resources is a pressing concern. Despite international commitments to support developing countries, the financial flows for adaptation in the LAC region are suboptimal. UNEP (2022) identified that the share of adaptation in total climate finance to developing countries was 34% in 2020, far behind mitigation finance. It estimates that annual adaptation needs are in the range of $160–340 billion USD by 2030 and $315–565 billion USD by 2050. It also considers the adaptation finance gap in developing countries is likely five to 10 times greater than current international adaptation finance flows and continues to widen. International organizations such as UNEP (2022) and IFRC (Swithern, 2020) and several authors (Weiler et al., 2018; Kissinger et al., 2019; Peterson and Skovgaard, 2019; Binet et al., 2021; Eriksen et al., 2021; Sánchez Rodríguez and Fernández Carril, 2024) have addressed barriers in climate financing to reach the most vulnerable countries and communities caused by misalignment between financing institutions and donor’s interest and the needs in developing countries. The misalignment is due to several reasons: political and trade agreements, pressure to favor low-risk investments, political incentives, pressure to show quick results leading to invest finite funds where they can make the most apparent results, weak institutions and lack of transparency managing funds in recipient countries. Although some countries in Latin America have received climate financing for adaptation during the last two decades from multilateral and bilateral funds, they are far for meeting the needs in the region; they tend to concentrate in some countries, and even only in some parts within these countries; they focus on certain sectors, and do not always reach the most vulnerable communities (Milhorance et al., 2022). Similar problems in adaptation financing have been reported in the literature in developing countries in Africa and Asia (Weiler et al., 2018; Swithern, 2020; Binet et al., 2021; UNEP, 2022).
It is also important to consider regional differences in financial needs in LAC. For example, the Caribbean is the most exposed region to climate disasters and requires $100 billion USD in adaptation investment (Galindo et al., 2022). However, accessing funds for adaptation is complex (Mohan, 2022), and Caribbean countries have been only approved $800 million USD from climate funds (Guerson et al., 2023). The current climate finance system needs to be improved with a stronger focus on adaptation to meet the urgent needs of the Small Island Developing States (SIDS) and other developing countries in the LAC region.
Governance frameworks overseeing the allocation, utilization, and monitoring of such funds lack the required transparency and accountability, thereby impacting the overall governance efficacy (Engels et al., 2019). Bridging the finance-implementation gap is possible by leveraging private sector involvement, promoting innovative financial instruments, and building local financial capacities (Clark et al., 2018). While public financing instruments like grants and concessional loans play a significant role, there is a palpable vacuum in private sector engagement in adaptation financing. Given the vast potential of private investments, the lack of streamlined mechanisms to tap into private funds limits the region’s financial agility in addressing climate challenges (Surminski and Oramas-Dorta, 2014).
Due to the peculiar vulnerabilities of ecosystems and communities in the region, there are limits to what adaptation can achieve by itself. For instance, the Amazon Forest is a crucial biome for global mitigation efforts and climate investment. To address deforestation’s negative impact on biodiversity, on Indigenous communities, and on feedback processes related to climate change, implementation of mitigation measures can provide multiple adaptation benefits when proper social safeguards are observed. Understanding and illuminating the synergies between adaptation and mitigation has the potential to unlock new revenue streams and future investment, but up to now co-benefits between mitigation and adaptation remain underexplored across LAC, in part due to siloed approaches.
The narrative on adaptation finance has been narrowly focused on access, with very little attention paid to how efficiently funds are utilized by national and local governments. Effective implementation of adaptation plans, policies, and programs is a major challenge for the LAC region with limitations arising from low technical capacities and corruption. Financial allocations, even when adequate, might not translate into desired outcomes if adaptation initiatives lack context specificity, actor inclusivity, and monitoring and evaluation mechanisms.
4.3 Adaptation limits, loss and damage
The discourse on adaptation limits presents a stark reality - there are thresholds beyond which incremental adaptation responses might become ineffective. Losses and damages encapsulate the impacts of climate change beyond adaptation limits in which we can face irreversible damage (Dow et al., 2013). There is a differentiation between soft and hard limits; however, the window of opportunity in which to act to avoid reaching these limits is narrow. Beyond this window, adaptation options lose effectiveness or might not be effective at all, therefore, as Working Group II of the IPCC AR6 indicates, we need to take urgent action while there is still an opportunity (IPCC, 2022a). In the LAC region, certain ecosystems like coral reefs and glacial systems are approaching their adaptation limits. This results in irreversible damage, impacting ecosystems, biodiversity, and the services they provide, such as water, fisheries, and livelihood sources (Warner and van der Geest, 2013). In certain cases, the residual risk associated with adaptation measures may be intolerable, which would decrease the viability of the strategy. Understanding the full extent of losses and damages necessitates comprehensive assessments that integrate scientific, socio-economic, local, and Indigenous knowledge perspectives. While tangible losses, such as infrastructural damages, can be quantified, intangible losses, like health and well-being, and cultural and physical displacements, require nuanced methodologies for assessment and redressal (Thomas and Benjamin, 2019). Some losses and damages cannot be quantified as they are attached to non-monetary values, therefore, there is also a need to consider other value systems in our responses (e.g., IPBES, 2022). Addressing these challenges requires transformative strategies and policies, which might encompass ecosystemic restorations, community relocations, socio-economic diversifications, and cultural preservation initiatives.
4.4 Transboundary adaptation and migration
Through shared ecosystems and natural resources, trade flows and social networks and transboundary climate risks (TCRs) connect multiple neighboring countries (Anisimov and Magnan, 2023). However, adaptation planning processes across LAC, as in many other regions, are isolated and fragmented. For example, some river basins and forest regions are shared by two or more countries; coral reef losses and the relatively recent sargassum surges in the Caribbean affect most Caribbean countries and Mexico, and adaptation priorities and actions in either country have implications for the other. While some transboundary fisheries cooperation exists, adaptation planning processes are independent. Additionally, the Caribbean is one of the most import-dependent regions in the world and, as such, is highly exposed to TCRs and supply chain disruptions. The LAC region is also highly dependent on cereal imports from the United States (Anisimov and Magnan, 2023), which are critical to livestock production and food security and thus vulnerable to transboundary climate impacts (Adams et al., 2021). Countries in the region have limited capacity to influence international markets. However, through regional cooperation and integration, governments can establish regional adaptation frameworks around economic and trade policy to unlock resilient pathways to address multiple TCRs (Anisimov and Magnan, 2023). The implementation of regional adaptation governance mechanisms to identify and manage TCRs is an important step toward addressing adaptation isolation driven by socioeconomic and development priorities. At the subnational level, adaptation actions in one community can create potential cascading risks for other communities. Despite these environmental and socioeconomic connections, TCRs and transboundary adaptation are poorly integrated in the NAPs of the LAC countries.
Central to transboundary issues associated with climate change is international migration within and from the region. Migration is often considered an adaptation response to climate variability and climate change impacts and, despite the substantial body of literature on climate and migration nexus, its underlying mechanisms remain poorly understood (Hoffmann et al., 2020). The relationship between climate and migration is not deterministic and unique across countries over time (Ibiden). There is also inadequate based research as well as distortions in political debates about migration (Thiede et al., 2016). Climatic conditions alone are rarely the only driver for migration and other interconnected compounding drivers should be considered as well (violence, poverty, inequality, loss of livelihoods, poor access to services, weak governance, expectation for a better life) (Kaenzig and Piguet, 2014; Simon and Riosmena, 2022). Migration is one of several potential responses to climatic stress and it should be analyzed considering other adaptation strategies (Silva Rodríguez de San Miguel et al., 2021). Ley et al. (2023) indicate that climate variability and changes in extreme events such as floods, hurricanes and droughts and their associated impacts (i.e., loss and damage of livelihoods, impacts on annual crop systems of subsistence farmers augmenting food insecurity, ecosystems degradation) can trigger displacement and migration (Vega-García, 2004; Kaenzig and Piguet, 2014; Balsari et al., 2020; Kaczan and Orgill-Meyer, 2020).
Authors studying the nexus between migration and climate change in Latin America highlight the unbalance in studies in the region. Simon and Riosmena (2022) suggest that compared to the body of scholarship on climate migration in Mexico, there is far less research on Central America in large part due to much lower availability of data on migration. Kaenzig and Piguet (2014) point out that, although climate change could lead to environmental disruptions without historical precedents, treating migration as an inevitable consequence of climate change, as well as trying to quantify the number of future migrants based on a simple count of the population living in the threatened areas, is a misleading simplification. Simon and Riosmena (2022) suggest that although climate and environmental shocks will likely continue to influence migration patterns in and from Latin America with often complex effects, it is uncertain that increasing climate variability will lead to more displacement, particularly international. Other authors consider resource constraints aggravated by climate change impacts, which may limit the ability to migrate (Benveniste et al., 2022). We believe the nexus of climate change and migration in LAC remains poorly understood and there is a need to better study and conceptualize migration as an adaptive response to climate change, as well as to define what constitutes successful migration and adaptation and for whom. The extensive social, economic, and political implications of this nexus require large attention and research.
4.5 Measuring adaptation progress
In contrast to mitigation initiatives that can be tracked by quantifiable metrics, adaptation is a more complex (and subjective) process that requires a long-term vision that transcends the usual temporality of short-term government programs (Cavazos, 2012). The definition of successful adaptation, developed by Doria et al. (2009) and based on any adjustment that reduces the risks associated with climate change, has recently been challenged among LAC experts (Guillén Bolaños et al., 2022), highlighting the need for improved indicators of dynamic evaluation of adaptation measures. To avoid additional burden on countries, the United Nations Foundation (2023) has already compiled a set of illustrative indicators and targets for the Global Goal Adaptation based on different instruments and reports. The assessment of the feasibility of adaptation measures is another useful tool to identify possible opportunities and barriers for implementation (Singh et al., 2020). The multidimensional feasibility assessment (MFA) was developed in the IPCC AR6 starting in the Special Report on Global warming at 1.5°C and continuing to the Assessments of WG II (IPCC, 2022a) (across sectors and regions) and WG III with the goal of assessing the feasibility of adaptation and mitigation responses across multiple dimensions, and assessing synergies and trade-offs between mitigation, adaptation, and the Sustainable Development Goals (IPCC, 2022b). Essential for measuring adaptation progress is implementing efficient monitoring and evaluation processes for each project or initiative. Monitoring and evaluation must be integrated into the design of adaptation actions from the beginning, and should clearly include actors, responsibilities, actions, and financial resources.
5 Building new pathways to just, inclusive, efficient, and sustainable climate agendas
The United Nations continuous warnings of the climate crisis and the urgent need to speed up adaptation in developing countries have not resonated in LAC countries. We have emphasized several shortcomings and problems in climate responses including isolated and independent sectoral adaptation options, and this last section focuses on steps that countries in the region could use for creating new pathways for building climate agendas focused on adaptation that consider co-benefits with mitigation actions as well as sustainable development, for example, through the Sustainable Development Goals.
We begin by addressing what we regard as fundamental issues to create efficient, just, equitable, ethical, and inclusive adaptation to climate change. Except for the Caribbean countries, most policymakers in Latin America have not placed climate change at the center of policies and debates. Climate change is considered an environmental problem and not a development challenge despite the development setbacks amongst growing evidence of extreme hydroclimate events, their cascading impacts and national and subnational consequences across the economy, social wellbeing, and ecosystem health. Government’s political support is essential to create, expand, improve, and accelerate actions to reduce vulnerability and to create adaptation actions; despite diverse positions among countries regarding climate change, up to now it is very difficult to secure short-term and long-term climate change commitments.
We recognize that almost all countries in Latin America and the Caribbean have National Climate Change Plans addressing adaptation, either as National Adaptation Plans or as part of National Climate Strategies that include mitigation and adaptation. Most of these plans have been created during the last decade and some of the older ones have been updated in recent years. They have been created through the support of international cooperation (Segura et al., 2022) and national and state governmental support. Unfortunately, there is little information on how many of these national plans and strategies have been implemented and even less information if they have been evaluated and what outcomes they have had (Berrang-Ford et al., 2021). Also, a point of concern is the lack of information on adaptation plans and strategies at the subnational level. Limited financial, technical, and human resources in most subnational governments are a major obstacle for building inclusive and efficient climate agendas (Ryan and Ramírez Cuesta, 2016; Ryan and Bustos, 2019). Moreover, mitigation and adaptation plans seem to be implemented and assessed independently of each other. Adaptation is so far limited to short-term; it is sector and place specific based on projects developed mostly through international financial support (UNEP, 2022; IPCC, 2022a). However, adaptation planning must not be sectoral and must also look at potential synergies and trade-offs, and potential for maladaptation (which has also caused significant setbacks in the region). This also means that when designing climate change adaptation policies, countries need to consider the root causes of vulnerability (IPCC, 2018, 2022a). Knowledge and information on local vulnerability and adaptation options is up to now limited in the region. Future research on national and subnational adaptation could provide useful lessons and best practices to increase efficient actions, set the basis to strengthen and expand regional cooperation, and create a framework to address transboundary adaptation.
LAC countries, as many others in the world, are confronted by climatic impacts mentioned in section 2, gaps in data availability and quality to support the design research and the design of policies and strategies mentioned in section 3, and challenges in adaptation governance and finance addressed in section 4. We recognize it is not easy to build new pathways for climate adaptation agendas in this context, particularly when governments in the region show little political will to strengthen adaptation at the national and subnational level.
We also recognize that current shortcomings in climate finance for adaptation are an obstacle to creating more efficient and inclusive climate agendas in LAC countries. It is not clear if and how climate finance will meet current and future requirements in the near and long term. A significant increase in climate finance is critical to expand adaptation actions and responses based on gray, green and blue infrastructures. However, we believe there are efficient low-cost actions LAC countries could implement to develop a more ambitious and efficient, just, inclusive, and sustainable climate agendas in the region.
We find it important to strengthen and expand climate literacy among national and subnational governments but also within society at large (Moser, 2010; Lee et al., 2015; Ryan and Bustos, 2019; CEPAL, 2022). Although general information on climate change has grown in recent years in the region, it is still considered an environmental problem with impacts on the future. There is little awareness of current extreme events and their increasing frequency and intensity in the short and middle terms (beyond the impacts immediately following an extreme weather event), as well as their impacts on social wellbeing, the economy, ecosystems and biodiversity, political stability, and aggravating development obstacles (McBean and Rodgers, 2010; IPCC, 2022a). An informed society is essential to develop adaptation public policies and strategies and that build transformational changes in adaptation strategies. It is also essential to create accountability and transparency in adaptation processes. Climate science scholars can play an important role by disseminating climate change knowledge among society (Dilling and Lemos, 2011). Improving communication between climate scientists and local communities will not only improve adaptation, but also empower communities to make informed decisions (Fernández-Llamazares et al., 2015).
Linked to raising climate change awareness in governments and society are efforts to improve and expand capacity development among decision-makers across different levels. Lack of adaptation public policies is often due to limited knowledge and information on vulnerability, impacts, risks, and opportunities to create adaptation, and lack of monitoring and evaluation (Hinkel, 2011; Ryan and Bustos, 2019). Capacity development is also essential for strengthening institutions, a basic step to expand efficient climate change agendas (Moser and Erkstrom, 2010). Climate science scholars can also play a major role in capacity development efforts among national and subnational governments, and in climate services. Some countries have implemented pilot efforts in this direction, but they have not been sustained after a few years. New knowledge and information on climate impacts, vulnerability and adaptation options could bring a new dimension to capacity development. Regional cooperation (south–south and north–south) can also play a major role in these efforts, as the examples given in section 3.4.
Other potential actions include diversifying knowledge, values and worldviews used in adaptation strategies. Particularly relevant in the case of LAC countries is the use of local and Indigenous Knowledge, often sidelined in mainstream data repositories. Its inclusion can provide depth, context, and richness to adaptation strategies (Fernández-Llamazares et al., 2015; IPCC, 2022a; Ley et al., 2023), especially in the case of nature-based solutions (e.g., Aide and Grau, 2004; Marsters et al., 2021). LAC countries could also invest in preventive actions, such as continuous hydroclimate monitoring and operational weather and climate forecasts, early warning systems and response plans. Improving socio-demographic data is essential for the analysis of vulnerability and investing in innovative structural (physical) resilience in critical sectors is an important mitigation and adaptation response. These actions can also benefit from regional cooperation and learning through practice.
As can be inferred throughout this article, climate change adaptation responses depend on the increase in mean temperature and GHG emissions, therefore, it is very relevant to consider the intertwined response of adaptation and mitigation actions (Figure 1). Climate Resilient Development (CRD) effectively brings together emissions reduction, vulnerability reduction, protection of biodiversity and achievement of sustainable development (and specifically the Sustainable Development Goals until 2030) (IPCC, 2022b). CRD brings together different worldviews and values, different knowledge systems (including Indigenous Knowledge), and includes all actors, especially the most vulnerable and marginalized. CRD is enabled by several elements, amongst them the multidimensional feasibility assessment that helps look beyond the usual technical and economic dimensions by including institutional, socio-cultural, environmental, and geophysical dimensions and brings together equity and inclusion indicators. CRD also considers system approaches that break sectoral silos and create linkages between sectors such as the interdependence between forests, agriculture, and water. Singh et al. (2020) exemplifies how water-based adaptation options can be used to link system transitions, highlighting how energy, urban, terrestrial, marine, and oceanic ecosystems are brought together, and more importantly, how adaptation options taken in one sector can have profound impacts, both positive and negative, on another system. This may help identify and maximize synergies and reduce trade-offs within systemic approaches (or transitions). However, it is necessary to transform CRD from an attractive concept to an operational approach by overcoming the barriers and challenges mentioned in this article and other works (e.g., Sánchez Rodríguez and Fernández Carril, 2024).
Time is of the essence and LAC governments need to include the whole of society to jointly develop feasible actions that are just, equitable and inclusive. Adaptation options are closing rapidly, and their cost will grow with every increase in global warming until limits are reached and adaptation feasibility and effectiveness decreases or options are no longer feasible or effective because of irreversible changes in human and natural systems. Further delays in adapting to climate change can have severe consequences for present and future generations.
Author contributions
TC: Conceptualization, Funding acquisition, Supervision, Writing – original draft, Writing – review & editing, Investigation, Formal analysis, Methodology, Visualization, Validation. MB: Conceptualization, Writing – original draft, Writing – review & editing, Investigation, Methodology, Supervision. DC: Investigation, Writing – original draft, Writing – review & editing, Methodology, Supervision. RS: Investigation, Writing – original draft, Writing – review & editing, Methodology, Supervision. MM: Investigation, Writing – review & editing, Writing – original draft. PA: Conceptualization, Investigation, Writing – review & editing, Writing – original draft. JR: Conceptualization, Investigation, Writing – review & editing, Visualization, Writing – original draft. MR: Conceptualization, Investigation, Writing – review & editing, Writing – original draft. CG: Conceptualization, Investigation, Writing – review & editing, Writing – original draft. HH: Conceptualization, Investigation, Writing – review & editing, Writing – original draft. EA: Conceptualization, Investigation, Writing – review & editing, Writing – original draft. TS: Writing – review & editing, Conceptualization, Investigation, Writing – original draft. AS: Investigation, Writing – review & editing, Writing – original draft. RC-M: Investigation, Writing – review & editing, Writing – original draft. EC: Investigation, Writing – review & editing, Writing – original draft. DL: Investigation, Visualization, Writing – original draft, Writing – review & editing. RM: Conceptualization, Writing – review & editing, Writing – original draft.
Funding
We thank the WCRP for supporting the publication of this article (Article Publication Charges). TC was supported by an Internal Project of the Department of Physical Oceanography, CICESE. HH and EA wish to acknowledge partial funding for this study through the following Vicerrectoría de Investigación, Universidad de Costa Rica grants: B9454 (supported by Fondo de Grupos), C2103, C3991 (UCREA), A4906 (PESCTMA) and B0-810. DL, EA, HH, and EC were partially supported by a grant awarded by the International Development Research Centre (IDRC), Ottawa, Canada, and the Central American University Council (CSUCA-SICA) to the Red Centroamericana de Ciencias sobre Cambio Climático (RC4) project (CR-66, SIA 0054-2, the opinions expressed here do not necessarily represent those of IDRC, CSUCA, or the Board of Governors).
Acknowledgments
We appreciate the positive and useful comments from two reviewers. We acknowledge the support and initial discussions with Detlef Stammer and Helen Cleugh, co-chairs of the Scientific Committee of the WCRP Open Science Conference 2023 in Kigali, who encouraged the realization of this and other Concept Papers on the Future of Climate Research. This paper is also part of the immediate climate action of the Kigali Declaration (WCRP, 2024). We also thank Beatriz Balino for her support on our online discussion conferences and for bridging us with WCRP. RS thanks the Department of Physical Oceanography, CICESE, México, for his sabbatical visit during the writing of this article.
Conflict of interest
The authors declare that the research was conducted in the absence of any commercial or financial relationships that could be construed as a potential conflict of interest.
The author(s) declared that they were an editorial board member of Frontiers, at the time of submission. This had no impact on the peer review process and the final decision.
Publisher’s note
All claims expressed in this article are solely those of the authors and do not necessarily represent those of their affiliated organizations, or those of the publisher, the editors and the reviewers. Any product that may be evaluated in this article, or claim that may be made by its manufacturer, is not guaranteed or endorsed by the publisher.
References
Adams, K.M., Benzie, M., Croft, S., and Sadowski, S. (2021). Climate change, trade, and global food security: a global assessment of transboundary climate risks in agricultural commodity flows. SEI report. Stockholm: Stockholm Environment Institute.
Adams, R. M., Houston, L. L., McCarl, B. A., Tiscareño, M., Matus, J., and Weiher, R. F. (2003). The benefits to Mexican agriculture of an El Niño-southern oscillation (ENSO) early warning system. Agric. For. Meteorol. 115, 183–194. doi: 10.1016/S0168-1923(02)00201-0
Adler, C., Wester, P., Bhatt, I., Huggel, C., Insarov, G. E., Morecroft, M. D., et al. (2022). ““Cross-chapter paper 5: mountains,” in climate change 2022: impacts, adaptation and vulnerability” in Contribution of working group II to the sixth assessment report of the intergovernmental panel on climate change. eds. H.-O. Pörtner, D. C. Roberts, M. Tignor, E. S. Poloczanska, K. Mintenbeck, and A. Alegría, et al. (Cambridge, UK and New York, NY, USA: Cambridge University Press), 2273–2318.
AghaKouchak, A., Mirchi, A., Madani, K., Di Baldassarre, G., Nazemi, A., Alborzi, A., et al. (2021). Anthropogenic drought: definition, challenges, and opportunities. Rev. Geophys. 59:e2019RG000683. doi: 10.1029/2019RG000683
Ahmed, A., Al-Khatib, A., Boum, Y., Debat, H., Gurmendi Dunkelberg, A., Janicke Hinchliffe, L., et al. (2023). The future of academic publishing. Nat. Hum. Behav. 7, 1021–1026. doi: 10.1038/s41562-023-01637-2
Aide, T. M., and Grau, H. R. (2004). Ecology, globalization, migration, and Latin American ecosystems. Science 305, 1915–1916. doi: 10.1126/science.1103179
Alfaro-Córdoba, M., Hidalgo, H. G., and Alfaro, E. J. (2020). Aridity trends in Central America: a spatial correlation analysis. Atmos 11:427. doi: 10.3390/atmos11040427
Almazroui, M., Ashfaq, M., Islam, M. Z., Rashid, I. U., Kamil, S., Abid, M. A., et al. (2021). Assessment of CMIP6 performance and projected temperature and precipitation changes over South America. Earth Syst. Environ. 5, 155–183. doi: 10.1007/s41748-021-00233-6
Anderson, T. G., McKinnon, K. A., Pons, D., and Anchukaitis, K. J. (2023). How exceptional was the 2015–2019 central American drought? Geophys. Res. Lett. 50:e2023GL105391. doi: 10.1029/2023GL105391
Andrade-Gómez, L., and Cavazos, T. (2024). Historical meteorological droughts over the CORDEX-CAM (Central America, Caribbean, and Mexico) domain: evaluating the simulation of dry hot spots with RegCM4. Int. J. Climatol. doi: 10.1002/joc.8374
Anisimov, A., and Magnan, A.K. (2023). The global transboundary climate risk report. The Institute for Sustainable Development and International Relations and Adaptation Without Borders. Available at: https://adaptationwithoutborders.org/knowledge-base/adaptation-without-borders/the-global-transboundary-climate-risk-report (Accessed December 23, 2023).
Appendini, C. M. (2024). Developing rapid response protocols for rapidly intensifying tropical cyclones. Bull. Am. Meteorol. Soc. 105, E518–E520. doi: 10.1175/BAMS-D-23-0327.1
Arenas-Wong, R. A., Robles-Morúa, A., Bojórquez, A., Martínez-Yrízar, A., Yépez, E. A., and Alvarez-Yépiz, J. C. (2023). Climate-induced changes to provisioning ecosystem services in rural socioecosystems in Mexico. Weather Clim Extremes 41:100583. doi: 10.1016/j.wace.2023.100583
Arias, P. A., Bellouin, N., Coppola, E., Jones, R. G., Krinner, G., Marotzke, J., et al. (2021). “Technical summary” in Climate change 2021: the physical science basis. Contribution of working group I to the sixth assessment report of the intergovernmental panel on climate change. eds. V. Masson-Delmotte, P. Zhai, A. Pirani, S. L. Connors, C. Péan, and S. Berger, et al. (United Kingdom and New York, NY, USA: Cambridge University Press, Cambridge), 33–144.
Arias, P. A., Rivera, J. A., Sörensson, A. A., Zachariah, M., Barnes, C., Philip, S., et al. (2024). Interplay between climate change and climate variability: the 2022 drought in Central South America. Clim. Chang. 177:6. doi: 10.1007/s10584-023-03664-4
Avila-Diaz, A., Torres, R. R., Zuluaga, C. F., Cerón, W. L., Oliveira, L., Benezoli, V., et al. (2023). Current and future climate extremes over Latin America and Caribbean: assessing earth system models from high resolution model intercomparison project (HighResMIP). Earth Syst. Environ. 7, 99–130. doi: 10.1007/s41748-022-00337-7
Balmaceda-Huarte, R., Olmo, M. E., Bettolli, M. L., and Poggi, M. M. (2021). Evaluation of multiple reanalyses in reproducing the spatio-temporal variability of temperature and precipitation indices over southern South America. Int. J. Climatol. 41, 5572–5595. doi: 10.1002/joc.7142
Balsari, S., Dresser, C., and Leaning, J. (2020). Climate change, migration, and civil strife. Curr. Environ. Heal. Rep. 7, 404–414. doi: 10.1007/s40572-020-00291-4
Battistello Espíndola, I., and Costa Ribeiro, W. (2020). Transboundary waters, conflicts and international cooperation - examples of the La Plata basin. Water Int. 45, 329–346. doi: 10.1080/02508060.2020.1734756
Beck, H. E., Vergopolan, N., Pan, M., Levizzani, V., van Dijk, A. I. J. M., Weedon, G. P., et al. (2017). Global-scale evaluation of 22 precipitation datasets using gauge observations and hydrological modeling. Hydrol. Earth Syst. Sci. 21, 6201–6217. doi: 10.5194/hess-21-6201-2017
Bell, M. L., O’Neill, M. S., Ranjit, N., Borja-Aburto, V. H., Cifuentes, L. A., and Gouveia, N. C. (2008). Vulnerability to heat-related mortality in Latin America: a case-crossover study in São Paulo, Brazil, Santiago, Chile and Mexico City, Mexico. Int. J. Epidemiol. 37, 796–804. doi: 10.1093/ije/dyn094
Benveniste, H., Oppenheimer, M., and Fleurbaey, M. (2022). Climate change increases resource-constrained international immobility. Nat. Clim. Chang. 12, 634–641. doi: 10.1038/s41558-022-01401-w
Berrang-Ford, L., Siders, A., Lesnikowski, A., Fisher, A., Callaghan, M., Haddaway, N., et al. (2021). A systematic global stocktake of evidence on human adaptation to climate change. Nat. Clim. Chang. 2021, 989–1000. doi: 10.1038/s41558-021-01170-y
Bettolli, M. L., Solman, S. A., da Rocha, R. P., Llopart, M., Gutierrez, J. M., Fernández, J., et al. (2021). The CORDEX flagship pilot study in Southeastern South America: a comparative study of statistical and dynamical downscaling models in simulating daily extreme precipitation events. Clim. Dyn. 56, 1589–1608. doi: 10.1007/s00382-020-05549-z
Bhatia, K., Baker, A., Yang, W., Vecchi, G., Knutson, T., Murakami, H., et al. (2022). A potential explanation for the global increase in tropical cyclone rapid intensification. Nat. Commun. 13:6626. doi: 10.1038/s41467-022-34321-6
Bhatia, K. T., Vecchi, G. A., Knutson, T. R., Murakami, H., Kossin, J., Dixon, K. W., et al. (2019). Recent increases in tropical cyclone intensification rates. Nat. Commun. 10:635. doi: 10.1038/s41467-019-08471-z
Biesbroek, G. R., Klostermann, J. E., Termeer, C. J., and Kabat, P. (2013). On the nature of barriers to climate change adaptation. Reg. Environ. Chang. 13, 1119–1129. doi: 10.1007/s10113-013-0421-y
Binet, S., De Bruijn, M., Horikoshi, D., Kim, R., Lee, B., Markrich, M., et al. (2021). Independent evaluation of the adaptation portfolio and approach of the green climate fund. Evaluation Report No. 9, February 2021. Independent Evaluation Unit, Green Climate Fund. Songdo, South Korea.
Bojórquez-Tapia, L. A., Eakin, H., Hernández-Aguilar, B., and Shelton, R. (2021). Addressing complex, political and intransient sustainability challenges of transdisciplinarity: the case of the MEGADAPT project in Mexico City. Environ. Dev. 38:100604. doi: 10.1016/j.envdev.2020.100604
Bony, S., Stevens, B., Ament, F., Bigorre, S., Chazette, P., Crewell, S., et al. (2017). EUREC4A: a field campaign to elucidate the couplings between clouds, convection and circulation. Surv. Geophys. 38, 1529–1568. doi: 10.1007/s10712-017-9428-0
Boyd, R., and Ibarrarán, M. E. (2009). Extreme climate events and adaptation: an exploratory analysis of drought in Mexico. Environ. Dev. Econ. 14:371. doi: 10.1017/S1355770X08004956
Brondízio, E. S., de Lima, A. C. B., Schramski, S., and Adams, C. (2016). Social and health dimensions of climate change in the Amazon. Ann. Hum. Biol. 43, 405–414. doi: 10.1080/03014460.2016.1193222
Cai, W., McPhaden, M. J., Grimm, A. M., Rodrigues, R. R., Taschetto, A. S., Garreaud, R. D., et al. (2020). Climate impacts of the El Niño–Southern Oscillation on South America. Nat Rev Earth Environ 1, 215–231. doi: 10.1038/s43017-020-0040-3
Carbon Brief (2021). Many researchers from the global south face obstacles towards conducting and publishing high-quality climate research. Available at: https://www.carbonbrief.org/researchers-the-barriers-to-climate-science-in-the-global-south/) (Accessed February 10, 2024).
Carbon Brief. (2023). What 13,500 citations reveal about the IPCC's climate science report. Posted by Sarah Connors, IPCC WGI. 16 March 2023. Available at: https://www.carbonbrief.org/guest-post-what-13500-citations-reveal-about-the-ipccs-climate-science-report/ (Accessed March 10, 2024).
Cashman, A., and Nagdee, M. (2017). Impacts of climate change on settlements and infrastructure in the coastal and marine environments of Caribbean Small Island developing states (SIDS). Caribb. Mar. Clim. Chang. Rep. Card: Sci. Rev. 11, 155–173. Available at: https://assets.publishing.service.gov.uk/media/5a82c330ed915d74e623781c/11._Settlements_and_Infrastructure_combined.docx.pdf [Revised on 9 April 2024].
Castellanos, E., Lemos, M. F., Astigarraga, L., Chacón, N., Cuvi, N., Huggel, C., et al. (2022). “Central and South America. In: climate change 2022: impacts, adaptation and vulnerability” in Contribution of working group II to the sixth assessment report of the intergovernmental panel on climate change. eds. H.-O. Pörtner, D. C. Roberts, M. Tignor, E. S. Poloczanska, K. Mintenbeck, and A. Alegría, et al. (Cambridge, UK and New York, NY, USA: Cambridge University Press), 1689–1816.
Castro-Torres, A. F., and Alburez-Gutierrez, D. (2022). North and south: naming practices and the hidden dimension of global disparities in knowledge production. Proc. Natl. Acad. Sci. 119:e2119373119, 1–7. doi: 10.1073/pnas.2119373119
Cavalcanti, I. F. A. (2012). Large scale and synoptic features associated with extreme precipitation over South America: a review and case studies for the first decade of the 21st century. Atmos. Res. 118, 27–40. doi: 10.1016/j.atmosres.2012.06.012
Cavazos, T. (2012). “Challenges of Mexico to face climate change” in Experimental and theoretical advances in fluid dynamics. Environmental science and engineering. eds. J. Klapp, A. Cros, O. Velasco Fuentes, C. Stern, and M. Rodriguez Meza (Berlin, Heidelberg: Springer)
Cavazos, T., Luna-Niño, R., Cerezo-Mota, R., Fuentes-Franco, R., Mendez, M., Pineda Martinez, L. F., et al. (2020). Climatic trends and regional climate models intercomparison over the CORDEX-CAM (Central America, Caribbean and Mexico) domain. Int. J. Climatol. 40, 1396–1420. doi: 10.1002/joc.6276
Centella, A., Bezanilla-Morlot, A., Taylor, M. A., Herrera, D. A., Martinez-Castro, D., Gouirand, I., et al. (2020). Evaluation of sixteen gridded precipitation datasets over the Caribbean region using gauge observations. Atmos 11:12. doi: 10.3390/atmos11121334
CEPAL (2022). Panorama social de América Latina y el Caribe 2022: la transformación de la educación como base para el desarrollo sostenible. Comisión Económica para América Latina y el Caribe. Santiago de Chile. Available at: https://repositorio.cepal.org/bitstream/handle/11362/48518/1/S2200947_es.pdf/.
Cerezo-Mota, R., Cavazos, T., Arritt, R., Torres-Alavez, A., Sieck, K., Nikulin, G., et al. (2016). CORDEX-NA: factors inducing dry/wet years on the North American monsoon region. Int. J. Climatol. 36, 824–836. doi: 10.1002/joc.4385
Cheng, L., Abraham, J., Trenberth, K. E., Boyer, T., Mann, M. E., Zhu, J., et al. (2024, 2024). New Record Ocean temperatures and related climate indicators in 2023. Adv. Atmos. Sci. doi: 10.1007/s00376-024-3378-5
Cisneros, P., Solorio, I., and Trimble, M. (2024). Editorial: thinking climate action from Latin America: a perspective from the local. NPJ Clim. Action 3:5. doi: 10.1038/s44168-023-00081-2
Clark, R., Reed, J., and Sunderland, T. (2018). Bridging funding gaps for climate and sustainable development: pitfalls, progress and potential of private finance. Land Use Policy 71, 335–346. doi: 10.1016/j.landusepol.2017.12.013
Colorado-Ruiz, G., and Cavazos, T. (2021). Trends of daily extreme and non-extreme rainfall indices and intercomparison with different gridded datasets over Mexico and the southern United States. Int. J. Climatol. 41, 5406–5430. doi: 10.1002/joc.7225
Condom, T., Martínez, R., Pabón, J. D., Costa, F., Pineda, L., Nieto, J. J., et al. (2020). Climatological and hydrological observations for the south American Andes: in situ stations, satellite, and reanalysis data sets. Front. Earth Sci. 8:92. doi: 10.3389/feart.2020.00092
Copernicus (2024). Global climate highlights 2023. Available at: https://climate.copernicus.eu/global-climate-highlights-2023 (Accessed January 10, 2024).
Coppola, E., Raffaele, F., Giorgi, F., Giuliani, G., Xuejie, G., Ciarlo, J. M., et al. (2021). Climate dynamics 57, 1293–1383. doi: 10.1007/s00382-021-05640-z,
de Lacerda, L. D., Borges, R., and Ferreira, A. C. (2019). Neotropical mangroves: conservation and sustainable use in a scenario of global climate change. Aquatic Conserv Marine Freshw. Ecosyst. 29, 1347–1364. doi: 10.1002/aqc.3119
de Lima, J., and Alcântara, C. (2019). Comparison between ERA interim/ECMWF, CFSR, NCEP/NCAR reanalysis, and observational datasets over the eastern part of the Brazilian Northeast region. Theor. Appl. Climatol. 138, 2021–2041. doi: 10.1007/s00704-019-02921-w
Dereczynski, C., Chou, S. C., Lyra, A., Sondermann, M., Regoto, P., Tavares, P., et al. (2020). Downscaling of climate extremes over South America—part I: model evaluation in the reference climate. Weather Clim. Extremes 29:100273. doi: 10.1016/j.wace.2020.100273
Di Napoli, C., Allen, T., Méndez-Lázaro, P. A., and Pappenberger, F. (2022). Heat stress in the Caribbean: climatology, drivers, and trends of human biometeorology indices. Int. J. Climatol. 43, 405–425. doi: 10.1002/joc.7774
Diez-Sierra, J., Iturbide, M., Gutiérrez, J. M., Fernández, J., Milovac, J., Cofiño, A. S., et al. (2022). The worldwide C3S CORDEX grand ensemble: a major contribution to assess regional climate change in the IPCC AR6 atlas. Bull. Am. Meteorol. Soc. 103, E2804–E2826. doi: 10.1175/BAMS-D-22-0111.1
Dilling, L., and Lemos, M. C. (2011). Creating usable science: opportunities and constraints for climate knowledge use and their implications for science policy. Glob. Environ. Chang. 21, 680–689. doi: 10.1016/j.gloenvcha.2010.11.006
Doblas-Reyes, F. J., Sörensson, A. A., Almazroui, M., Dosio, A., Gutowski, W. J., Haarsma, R., et al. (2021). “Linking global to regional climate change” in Climate change 2021: the physical science basis. Contribution of working group I to the sixth assessment report of the intergovernmental panel on climate change. eds. V. Masson-Delmotte, P. Zhai, A. Pirani, S. L. Connors, C. Péan, and S. Berger, et al. (Cambridge, United Kingdom and New York, NY, USA: Cambridge University Press), 1363–1512.
Dominguez, F., Rasmussen, R., Liu, C., Ikeda, K., Prein, A., Varble, A., et al. (2024). Advancing south American water and climate science through multidecadal convection-permitting Modeling. Bull. Am. Meteorol. Soc. 105, E32–E44. doi: 10.1175/BAMS-D-22-0226.1
Doria, M., Boyd, E., Tompkins, E. L., and Adger, W. N. (2009). Using expert elicitation to define successful adaptation to climate change. Environ. Sci. Pol. 12, 810–819. doi: 10.1016/j.envsci.2009.04.001
Dorigo, W., Wagner, W., Albergel, C., Albrecht, F., Balsamo, G., Brocca, L., et al. (2017). ESA CCI soil moisture for improved earth system understanding: state-of-the art and future directions, remote Sens. Environment 203, 185–215. doi: 10.1016/j.rse.2017.07.001
dos Santos, R. B., de Brito Duval, I., Menezes, J. A., de Lima Barata, M. M., and Confalonieri, U. E. C. (2024). “Building scenarios of social and health vulnerability to climate change: a study for municipalities in the Mato Grosso do Sul, Brazil” in Akhtar, R. (eds) Climate change and human health scenarios: Global Perspectives on Health Geography. Akhtar, R. Ed. (Springer Nature Switzerland: Cham), 435–449. doi: 10.1007/978-3-031-38878-1_27
Dow, K., Berkhout, F., and Preston, B. L. (2013). Limits to adaptation to climate change: a risk approach. Curr. Opin. Environ. Sustain. 5, 384–391. doi: 10.1016/j.cosust.2013.07.005
Downs, T. J., Ruelle, M., Brissett, N., Hanumantha, R., Mazari-Hiriart, M., Krueger, R., et al. (2022). An integrative collaborative project approach to climate-change resilience and urban/regional sustainability for the Mexico-Lerma-Cutzamala hydrological region. Open J. Civil Eng. 12, 101–138. doi: 10.4236/ojce.2022.121008
Durán-Quesada, A. M., Sorí, R., Ordoñez, P., and Gimeno, L. (2020). Climate perspectives in the intra–Americas seas. Atmos 11:959. doi: 10.3390/atmos11090959
Eakin, H. C., Lemos, M. C., and Nelson, D. R. (2014). Differentiating capacities as a means to sustainable climate change adaptation. Glob. Environ. Chang. 27, 1–8. doi: 10.1016/j.gloenvcha.2014.04.013
Emanuel, K. (2021). Response of global tropical cyclone activity to increasing CO2: results from downscaling CMIP6 models. J. Clim. 34, 57–70. doi: 10.1175/JCLI-D-20-0367.1
Engels, F., Wentland, A., and Pfotenhauer, S. M. (2019). Testing future societies? Developing a framework for test beds and living labs as instruments of innovation governance. Res. Policy 48:103826. doi: 10.1016/j.respol.2019.103826
Eriksen, S., Schipper, E. L. F., Scoville-Simonds, M., Vincent, K., Adam, H. N., Brooks, N., et al. (2021). Adaptation interventions and their effect on vulnerability in developing countries: help, hindrance or irrelevance? World Dev. 141:105383. doi: 10.1016/j.worlddev.2020.105383
Estrada, F., Perron, P., and Yamamoto, Y. (2023). Anthropogenic influence on extremes and risk hotspots. Sci. Rep. 13:35. doi: 10.1038/s41598-022-27220-9
Fernández-Llamazares, A., Méndez-López, M. E., Díaz-Reviriego, I., McBride, M. F., Pyhälä, A., Rosell-Melé, A., et al. (2015, 2015). Links between media communication and local perceptions of climate change in an indigenous society. Clim. Chang. 131, 307–320. doi: 10.1007/s10584-015-1381-7
Fila, D., Fünfgeld, H., and Dahlmann, H. (2024). Climate change adaptation with limited resources: adaptive capacity and action in small-and medium-sized municipalities. Environ. Dev. Sustain. 26, 5607–5627. doi: 10.1007/s10668-023-02999-3
Freitas, A. A., Drumond, A., Carvalho, V. S., Reboita, M. S., Silva, B. C., and Uvo, C. B. (2021). Drought assessment in São Francisco river basin, Brazil: characterization through SPI and associated anomalous climate patterns. Atmos 13:41. doi: 10.3390/atmos13010041
Freitas, A. A., Reboita, M. S., Carvalho, V. S. B., Drumond, A., Ferraz, S. E. T., Silva, B. C., et al. (2023). Atmospheric and oceanic patterns associated with extreme drought events over the Paraná hydrographic region, Brazil. Climate 11:12. doi: 10.3390/cli11010012
Fuchs-Stone, Ž., Raymond, D. J., and Sentić, S. (2020). OTREC2019: convection over the East Pacific and Southwest Caribbean. Geophys. Res. Lett. 47:e2020GL087564. doi: 10.1029/2020GL087564
Funk, C., Peterson, P., Landsfeld, M., Pedreros, D., Verdin, J., Shukla, S., et al. (2015). The climate hazards infrared precipitation with stations--a new environmental record for monitoring extremes. Sci Data 2:150066. doi: 10.1038/sdata.2015.66
Galindo, L.M., Hoffmann, B., and Vogt-Schilb, A. (2022). How much will it cost to achieve the climate goals in Latin America and the Caribbean? IDB working paper series no. IDB-WP-01310. Inter-American Development Bank, climate change and sustainable development sector and Office of Research and Chief Economist. Available at: https://publications.iadb.org/en/how-much-will-it-cost-achieve-climate-goals-latin-america-and-caribbean
Galindo, L. M., Samaniego, J., Alatorre, J. E., Ferrer, J., Gómez, J. J., Lennox, J., et al. (2015). The economics of climate change in Latin America and the Caribbean paradoxes and challenges of sustainable development. Santiago de Chile UN and the Economic Commission for Latin America and the Caribbean (Santiago de Chile: ECLAC).
Gallagher (2024). Natural catastrophe and climate report 2023. Available at: https://www.ajg.com/gallagherre/-/media/files/gallagher/gallagherre/news-and-insights/2024/january/natural_catastrophe_and_climate_report_2023.pdf (Accessed January 18, 2024).
Garreaud, R. D., Boisier, J. P., Rondanelli, R., Montecinos, A., Sepúlveda, H. H., and Veloso-Aguila, D. (2019). The Central Chile mega drought (2010–2018): a climate dynamics perspective. Int. J. Climatol. 2019, 1–19. doi: 10.1002/joc.6219
Garro-Quesada, M. M., Vargas-Leiva, M., Girot, P. O., and Quesada-Román, A. (2023). Climate risk analysis using a high-resolution spatial model in Costa Rica. Climate 11:127. doi: 10.3390/cli11060127
Gay-Antaki, M. (2021). Stories from the IPCC: an essay on climate science in fourteen questions. Glob. Environ. Chang. 71:102384. doi: 10.1016/j.gloenvcha.2021.102384
Geirinhas, J. L., Russo, A. C., Libonati, R., Miralles, D. G., Ramos, A. M., Gimeno, L., et al. (2023). Combined large-scale tropical and subtropical forcing on the severe 2019–2022 drought in South America. NPJ Clim. Atmos. Sci. 6:185. doi: 10.1038/s41612-023-00510-3
Gimeno, L., Dominguez, F., Nieto, R., Trigo, R., Drumond, A., Reason, C.J.C., et al. (2016). Major mechanisms of atmospheric moisture transport and their role in extreme precipitation events. Annu. Rev. Environ. Resour. 41, 117–141. doi: 10.1146/annurev-environ-110615-085558
Giorgi, F., and Gutowski, W. J. (2015). Regional dynamical downscaling and the CORDEX initiative. Annu. Rev. Environ. Resour. 40, 467–490. doi: 10.1146/annurev-environ-102014-021217
Gotlieb, Y., and García-Girón, J. D. (2020). The role of land use conversion in shaping the land cover of the central American dry corridor. Land Use Policy 94:104351. doi: 10.1016/j.landusepol.2019.104351
Gu, G., and Adler, R. F. (2023). Observed variability and trends in global precipitation during 1979–2020. Clim. Dyn. 61, 131–150. doi: 10.1007/s00382-022-06567-9
Guerson, A., Morsink, J., and Muñoz, S. (2023). Caribbean climate crisis demands urgent action by governments and investors. IMF Blog. Available at: https://www.imf.org/en/Blogs/Articles/2023/06/27/caribbean-climate-crisis-demands-urgent-action-by-governments-and-investors (Accessed February 11, 2024).
Guillén Bolaños, T., Scheffran, J., and Máñez Costa, M. (2022). Climate adaptation and successful adaptation definitions: Latin American perspectives using the Delphi method. Sustain. For. 14:5350. doi: 10.3390/su14095350
Gutiérrez, J. M., Jones, R. G., Narisma, G. T., Alves, L. M., Amjad, M., Gorodetskaya, I. V., et al. (2021). “Atlas” in Climate change 2021: the physical science basis. Contribution of working group I to the sixth assessment report of the intergovernmental panel on climate change. eds. V. Masson-Delmotte, P. Zhai, A. Pirani, S. L. Connors, C. Péan, and S. Berger, et al. (United Kingdom and New York, NY, USA: Cambridge University Press, Cambridge), 1927–2058.
Hagen, I., Huggel, C., Ramajo, L., Chacón, N., Ometto, J. P., Postigo, J. C., et al. (2022). Climate change-related risks and adaptation potential in central and South America during the 21st century. Environ. Res. Lett. 17:033002. doi: 10.1088/1748-9326/ac5271
Hegerl, G. C., Black, E., Allan, R. P., Ingram, W. J., Polson, D., Trenberth, K. E., et al. (2015). Challenges in quantifying changes in the global water cycle. Bull. Am. Meteorol. Soc. 96, 1097–1115. doi: 10.1175/BAMS-D-13-00212.1
Herrera, D., and Ault, T. (2017). Insights from a New high-resolution drought atlas for the Caribbean spanning 1950–2016. J. Clim. 30, 7801–7825. doi: 10.1175/JCLI-D-16-0838.1
Hidalgo, H., and Alfaro, E. (2012). Some physical and socio-economical aspects of climate change in Central America. Prog. Phys. Geogr. 36, 380–399. doi: 10.1177/0309133312438906
Hidalgo, H. G., Alfaro, E. J., Amador, J. A., and Bastidas, A. (2019). Precursors of quasi-decadal dry-spells in the Central America dry corridor. Clim. Dyn. 53, 1307–1322. doi: 10.1007/s00382-019-04638-y
Hidalgo, H. G., Alfaro, E. J., Hernández-Castro, F., and Pérez-Briceño, P. M. (2020). Identification of tropical cyclones’ critical positions associated with extreme precipitation events in Central America. Atmos. 11:1123. doi: 10.3390/atmos11101123
Hidalgo, H. G., Alfaro, E. J., and Quesada-Montano, B. (2017). Observed (1970–1999) climate variability in Central America using a high-resolution meteorological dataset with implication to climate change studies. Clim. Chang. 141, 13–28. doi: 10.1007/s10584-016-1786-y
Hidalgo, H. G., Alfaro, E. J., Valverde, K. T., and Bazo, J. (2022). Probability of induced extreme precipitation events in Central America due to tropical cyclone positions in the surrounding oceans. Nat. Hazards 116, 2917–2933. doi: 10.1007/s11069-022-05790-1
Hidalgo, H. G., Amador, J. A., Alfaro, E. J., and Quesada, B. (2013). Hydrological climate change projections for Central America. J. Hydrol. 495, 94–112. doi: 10.1016/j.jhydrol.2013.05.004
Hinkel, J. (2011). Indicators of vulnerability and adaptive capacity: towards a clarification of the science-policy interface. Glob. Environ. Chang. 21, 198–206. doi: 10.1016/j.gloenvcha.2010.08.002
Hoffmann, R., Dimitrova, A., Muttarak, R., Crespo Cuaresma, J., and Peisker, J. (2020). A meta-analysis of country-level studies on environmental change and migration. Nat. Clim. Chang. 10, 904–912. doi: 10.1038/s41558-020-0898-6
Huaman, L., Schumacher, C., and Sobel, A. H. (2022). Assessing the vertical velocity of the East Pacific ITCZ. Geophys. Res. Lett. 49:e2021GL096192. doi: 10.1029/2021gl096192
IPBES (2022). “Summary for policymakers of the methodological assessment report on the diverse values and valuation of nature of the intergovernmental science-policy platform on biodiversity and ecosystem services” in IPBES secretariat. eds. U. Pascual, P. Balvanera, M. Christie, B. Baptiste, D. González-Jiménez, and C. B. Anderson, et al. (Germany: Bonn)
IPCC (2018). “Summary for policymakers” in Global Warming of 1.5°C. An IPCC Special Report on the impacts of global warming of 1.5°C above pre-industrial levels and related global greenhouse gas emission pathways, in the context of strengthening the global response to the threat of climate change, sustainable development, and efforts to eradicate poverty. eds. V. Masson-Delmotte, P. Zhai, H. O. Pörtner, D. Roberts, J. Skea, and P. R. Shukla, et al. (Cambridge, UK and New York, NY, USA: Cambridge University Press), pp. 3–24.
IPCC (2021). “Summary for policymakers” in Climate change 2021: the physical science basis. Contribution of working group I to the sixth assessment report of the intergovernmental panel on climate change. eds. V. Masson-Delmotte, P. Zhai, A. Pirani, S. L. Connors, C. Péan, and S. Berger, et al. (United Kingdom and New York, NY, USA: Cambridge University Press, Cambridge), 3–32.
IPCC (2022a). “Summary for policymakers” in Climate change 2022: Impacts, adaptation and vulnerability. Contribution of working group II to the sixth assessment report of the intergovernmental panel on climate change. eds. H.-O. Pörtner, D. C. Roberts, E. S. Poloczanska, K. Mintenbeck, M. Tignor, and A. Alegría, et al. (Cambridge, UK and New York, NY, USA: Cambridge University Press), 3–33.
IPCC (2022b). “Summary for policymakers” in Climate change 2022: mitigation of climate change. Contribution of working group III to the sixth assessment report of the intergovernmental panel on climate change. eds. P. R. Shukla, J. Skea, A. Reisinger, R. Slade, R. Fradera, and M. Pathak, et al. (Cambridge, UK and New York, NY, USA: Cambridge University Press)
IPCC (2023). “Summary for policymakers” in Climate change 2023: synthesis report. Contribution of working groups I, II and III to the sixth assessment report of the intergovernmental panel on climate change. eds. H. Lee and J. Romero (Geneva, Switzerland: IPCC), 1–34.
Iturbide, M., Gutiérrez, J. M., Alves, L. M., Bedia, J., Cerezo-Mota, R., Cimadevilla, E., et al. (2020). An update of IPCC climate reference regions for subcontinental analysis of climate model data: definition and aggregated datasets. Earth Syst. Sci. Data 12, 2959–2970. doi: 10.5194/essd-12-2959-2020
Iwama, A. Y., Araos, F., Anbleyth-Evans, J., Marchezini, V., Ruiz-Luna, A., Ther-Ríos, F., et al. (2021). Multiple knowledge systems and participatory actions in slow-onset effects of climate change: insights and perspectives in Latin America and the Caribbean. Curr. Opin. Environ. Sustain. 50, 31–42. doi: 10.1016/j.cosust.2021.01.010
Jones, P. D., Harpham, C., Harris, I., Goddess, C. M., Burton, A., Centella-Artola, A., et al. (2016). Long-term trends in precipitation and temperature across the Caribbean. Int. J. Climatol. 36, 3314–3333. doi: 10.1002/joc.4557
Kaczan, D. J., and Orgill-Meyer, J. (2020). The impact of climate change on migration: a synthesis of recent empirical insights. Clim. Chang. 158, 281–300. doi: 10.1007/s10584-019-02560-0
Kaenzig, R., and Piguet, E. (2014). “Migration and climate change in Latin America and the Caribbean” in People on the move in a changing climate. Global migration issues. eds. E. Piguet and F. Laczko, vol. 2 (Cham: Springer, Dordrecht)
Kew, S., Pinto, I., Alves, L., Santos, D., Libonati, R., Liberato, M. L. R., et al. (2023). Strong influence of climate change in uncharacteristic early spring heat in South America. London, UK: World Weather Attribution.
Kissinger, G., Gupta, A., Mulder, I., and Unterstell, N. (2019). Climate financing needs in the land sector under the Paris agreement: an assessment of developing country perspectives. Land Use Policy 83, 256–269. doi: 10.1016/j.landusepol.2019.02.007
Ladio, A. H. (2017). Ethnobiology and research on global environmental change: what distinctive contribution can we make? Ethnobiol. Conserv. 6, 1–8. doi: 10.15451/ec2017-07-6.7-1-8
Lapola, D. M., Silva, J. M. C., Braga, D. R., Carpigiani, L., Ogawa, F., Torres, R. R., et al. (2020). A climate-change vulnerability and adaptation assessment for Brazil's protected areas. Conserv. Biol. 34, 427–437. doi: 10.1111/cobi.13405
Lee, T. M., Markowitz, E., Howe, P., Ko, C., and Leiserowitz, A. (2015). Predictors of public change awareness and risk perception around the world. Nat. Clim. Chang. 5, 1014–1020. doi: 10.1038/nclimate2728
Ley, D., Guillen Bolaños, T., Castaneda, A., Hidalgo, H. G., Pignot, P. O. G., Fernández, R., et al. (2023). Central America urgently needs to reduce the growing adaptation gap to climate change 2023. Front. Clim. 5:1215062. doi: 10.3389/fclim.2023.1215062
Li, L., and Chakraborty, P. (2020). Slower decay of landfalling hurricanes in a warming world. Nature 587, 230–234. doi: 10.1038/s41586-020-2867-7
Li, Z., England, M. H., and Groeskamp, S. (2023). Recent acceleration in global ocean heat accumulation by mode and intermediate waters. Nat. Commun. 14:6888. doi: 10.1038/s41467-023-42468-z
Li, X., Hu, Z.-Z., McPhaden, M. J., Zhu, C., and Liu, Y. (2023). Triple-dip La Niñas in 1998–2001 and 2020–2023: impact of mean state changes. J. Geophys. Res. Atmos. 128:e2023JD038843. doi: 10.1029/2023JD038843
Lincoln, S. (2017). Impacts of climate change on Society in the Coastal and Marine Environments of Caribbean Small Island developing states (SIDS). Caribbean Marine Clim. Change Rep. Card Sci. Rev. 9, 115–123.
Liverman, D., vonHedemann, N., Nying'uro, P., Rummukainen, M., Stendahl, K., Gay-Antaki, M., et al. (2022). Survey of gender bias in the IPCC. Nature 602, 30–32. doi: 10.1038/d41586-022-00208-1
Luna-Niño, R., Cavazos, T., Torres-Alavez, J., Giorgi, F., and Coppola, E. (2020). Interannual variability of the boreal winter subtropical jet stream and teleconnections over the CORDEX-CAM domain during 1980–2010. Clim. Dyn. 57, 1571–1594. doi: 10.1007/s00382-020-05509-7
Magaña, V., Herrera, E., Ábrego-Góngora, C. J., and Ávalos, J. A. (2021). Socioeconomic drought in a mexican semi-arid city: Monterrey metropolitan area, a case study. Front. Water 3:579564. doi: 10.3389/frwa.2021.579564
Magrin, G, Marengo, J, Boulanger, JP, Silveira Buckeridge, M, Castellano, E, Poveda, Germán, et al. (2014). Central and South America. Climate change 2014: impacts, adaptation, and vulnerability. Working group II contribution to the IPCC fifth assessment report. Vol. 2. Regional Aspects. Cambridge: Cambridge University Press, pp. 1499–1566.
Mahon, R., Greene, C., Cox, S.-A., Guido, Z., Gerlak, A. K., Petrie, J.-A., et al. (2019). Fit for purpose? Transforming National Meteorological and hydrological services into National Climate Service Centers. Clim. Ser. 13, 14–23. doi: 10.1016/j.cliser.2019.01.002
Marsters, L., Morales, G., Ozment, S., Silva, M., Watson, G., Netto, M., et al. (2021). Nature-based solutions in Latin America and the Caribbean: Financing mechanisms for regional replication. Washington, DC: Inter-American Development Bank and World Resources Institute.
Martinez, S., Kralisch, S., Escolero, O., and Perevochtchikova, M. (2015). Vulnerability of Mexico City’s water supply sources in the context of climate change. J. Water Clim. Change 6, 518–533. doi: 10.2166/wcc.2015.083
Maynard-Ford, M.C., Phillips, E. C., and Chirico, P. G. (2007). Mapping vulnerability to disasters in Latin American and the Caribbean, 1900-2007. USGS and Office of the Foreign Disaster Assistance. Open-File Report 2008-1294. Available at: https://pubs.usgs.gov/of/2008/1294/ofr2008-1294.pdf (Accessed January 25, 2024).
McBean, G., and Rodgers, C. (2010). Climate hazards and disasters: the need for capacity building. WIREs Clim. Change 1, 871–884. doi: 10.1002/wcc.77
Méndez, M., and Magaña, V. (2010). Regional aspects of prolonged meteorological droughts over Mexico and Central America. J. Clim. 23, 1175–1188. doi: 10.1175/2009JCLI3080.1
Milhorance, C., Howland, F., Sabourin, E., and Le Coq, J.-F. (2022). Tacking the implementation gap of climate adaptation strategies: understanding policy translation in Brazil and Colombia. Clim. Pol. 22, 1113–1129. doi: 10.1080/14693062.2022.2085650
Mohan, P. S. (2022). Implementing nationally determined contributions under the Paris agreement: an assessment of climate finance in Caribbean small island developing states. Clim. Pol. 22, 1281–1289. doi: 10.1080/14693062.2022.2101978
Mohan, P. S. (2023). Financing climate change mitigation and adaptation in Caribbean SIDS. PLoS Clim. 2:e0000167. doi: 10.1371/journal.pclm.0000167
Monterroso Rivas, A, Fernandez, EA, Trejo, VRI, Conde, AAC, Escandón, CJ, and Villers, RL. (2014). Vulnerabilidad y adaptación al cambio climático en México. PINCC, UNAM. Available at: http://atlasclimatico.unam.mx/VyA (Accessed February 4, 2024).
Montini, T. L., Jones, C., and Carvalho, L. M. V. (2019). The south American low-level jet: a new climatology, variability, and changes. J. Geophys. Res. Atmos. 124, 1200–1218. doi: 10.1029/2018JD029634
Morales, M. S., Crispín-DelaCruz, D. B., Álvarez, C., Christie, D. A., Ferrero, M. E., Andreu-Hayles, L., et al. (2023). Drought increase since the mid-20th century in the northern South American altiplano revealed by a 389-year precipitation record. Clim. Past 19, 457–476. doi: 10.5194/cp-19-457-2023
Moser, S. (2010). Communicating climate change: history, challenges, process and future directions. WIREs Clim. Change 1, 31–53. doi: 10.1002/wcc.11
Moser, S., and Erkstrom, J. (2010). A framework to diagnose barriers to climate change adaptation. PNAS 107, 2202–22031. doi: 10.1073/pnas.100788710
Muelbert, M. M. C., Copertino, M., Cotrim da Cunha, L., Lewis, M. N., Polejack, A., Peña-Puch, A. C., et al. (2021). The ocean and cryosphere in a changing climate in Latin America: knowledge gaps and the urgency to translate science into action. Front. Clim. 3:748344. doi: 10.3389/fclim.2021.748344
Mycoo, M. (2018a). Beyond 1.5C: vulnerabilities and adaptation strategies for Caribbean Small Island developing states. Reg. Environ. Chang. 18, 2341–2353. doi: 10.1007/s10113-017-1248-8
Mycoo, M. (2018b). Urban sustainability in Caribbean Small Island developing states: a conceptual framework for urban planning using a case study of Trinidad. Int. Dev. Plan. Rev. 40, 143–174. doi: 10.3828/idpr.2018.8
Mycoo, M, Wairiu, M, Campbell, D, Duvat, V, Golbuu, Y, Maharaj, S, et al. (2022). Small Islands. In: Climate change 2022: impacts, adaptation and vulnerability. Contribution of working group II to the sixth assessment report of the intergovernmental panel on climate change [Pörtner, H.-O., and Roberts, D.C., M. Tignor, E.S. Poloczanska, K. Mintenbeck, and A. Alegría, et al. (eds.)]. Cambridge University Press, Cambridge, UK and New York, NY, USA, pp. 2043–2121.
Nagy, G. J., Gutiérrez, O., Brugnoli, E., Verocai, J. E., Gómez-Erache, M., Villamizar, A., et al. (2019). Climate vulnerability, impacts and adaptation in central and South America coastal areas. Reg. Stud. Mar. Sci. 29:100683. doi: 10.1016/j.rsma.2019.100683
Ocampo-Melgar, A., Lutz-Ley, A., Zúñiga, A., Cerda, C., and Goirán, S. (2023). Latin American drylands: challenges and opportunities for sustainable development. Metode Sci. Stud. J. 13, 33–39. doi: 10.7203/metode.13.21458
Ometto, J., Toledo, P., Arcoverde, G., Alves, L., and Andrade, P. (2021). AdaptaBrasil MCTI innovative platform for monitoring climate change impacts in Brazil. In AGU fall meeting 2021, New Orleans.
Paredes-Trejo, F., Olivares, B. O., Movil-Fuentes, Y., Arevalo-Groening, J., and Gil, A. (2023). Assessing the spatiotemporal patterns and impacts of droughts in the Orinoco River basin using earth observations data and surface observations. Hydrology 10:195. doi: 10.3390/hydrology10100195
Parker, W. S. (2016). Reanalyses and observations: What’s the difference? Bull. Amer. Meteor. Soc. 97, 1565–1572. doi: 10.1175/BAMS-D-14-00226.1
Pascale, S., Kapnick, S. B., Delworth, T. L., Hidalgo, H. G., and Cooke, W. F. (2021). Natural variability vs forced signal in the 2015–2019 central American drought. Clim. Chang. 168:16. doi: 10.1007/s10584-021-03228-4
PEACC-BC. (2012). Programa Estatal de acción ante el cambio climático de Baja California (PEACC-BC). Available at https://biblioteca.semarnat.gob.mx/janium/Documentos/Ciga/Libros2013/CD001769.pdf (Accessed February 4, 2024).
Peterson, L., and Skovgaard, J. (2019). Bureaucratic politics and the allocation of climate finance. World Dev. 117, 72–97. doi: 10.1016/j.worlddev.2018.12.011
Petzold, J., Joe, E. T., Kelman, I., Magnan, A. K., Mirbach, C., Nagle Alverio, G., et al. (2023). Between tinkering and transformation: a contemporary appraisal of climate change adaptation research on the world's islands. Front. Clim. 4:1072231. doi: 10.3389/fclim.2022.1072231
Prein, A. F., Langhans, W., Fosser, G., Ferrone, A., Ban, N., Goergen, K., et al. (2015). A review on regional convection-permitting climate modeling: demonstrations, prospects, and challenges. Rev. Geophys. 53, 323–361. doi: 10.1002/2014RG000475
Quesada-Montano, B., Wetterhall, F., Westerberg, I., Hidalgo, H., and Halldin, S. (2019). Characterising droughts in Central America with uncertain hydro-meteorological data. Theor. Appl. Climatol. 137:14. doi: 10.1007/s00704-018-2730-z
Ranasinghe, R., Ruane, A.C., Vautard, R., Arnell, N., Coppola, E., Cruz, F.A., et al. (2021). Climate change information for regional impact and for risk assessment. In climate change 2021: the physical science basis. Contribution of working group I to the sixth assessment report of the intergovernmental panel on climate change [V Masson-Delmotte., P. Zhai, A. Pirani, S.L. Connors, C. Péan, and S. Berger, et al. (eds.)]. Cambridge University Press, Cambridge, United Kingdom and New York, NY, USA, pp. 1767–1926.
Rao, V. B., Franchito, S. H., Santo, C. M., and Gan, M. A. (2016). An update on the rainfall characteristics of Brazil: seasonal variations and trends in 1979–2011. Int. J. Climatol. 36, 291–302. doi: 10.1002/joc.4345
Raymond, C., Matthews, T., and Horton, R. M. (2020). The emergence of heat and humidity too severe for human tolerance. Sci. Adv. 6:eaaw1838. doi: 10.1126/sciadv.aaw1838
Reboita, M. S., Kuki, C. A. C., Marrafon, V. H., de Souza, C. A., Ferreira, G. W. S., Teodoro, T., et al. (2022). South America climate change revealed through climate indices projected by GCMs and eta-RCM ensembles. Clim. Dyn. 58, 459–485. doi: 10.1007/s00382-021-05918-2
Ridder, N. N., Ukkola, A. M., Pitman, A. J., and Perkins-Kirkpatrick, S. E. (2022). Increased occurrence of high impact compound events under climate change. NPJ Clim Atmos Sci 5:3. doi: 10.1038/s41612-021-00224-4
Rivera, J. A., Arias, P. A., Sörensson, A. A., Zachariah, M., Barnes, C., Philip, S., et al. (2023). 2022 early-summer heatwave in southern South America: 60 times more likely due to climate change. Clim. Chang. 176:102. doi: 10.1007/s10584-023-03576-3
Romano, S. T., Nelson-Nuñez, J., and LaVanchy, G. T. (2021). Rural water provision at the state-society interface in Latin America. Water Int. 46, 802–820. doi: 10.1080/02508060.2021.1928973
Ruane, A. C., Vautard, R., Ranasinghe, R., Sillmann, J., Coppola, E., Arnell, N., et al. (2022). The climatic impact-driver framework for assessment of risk-relevant climate information. Earth’s Future 10:e2022EF002803. doi: 10.1029/2022EF002803
Ryan, D., and Bustos, E. (2019). Knowledge gaps and climate adaptation policy: a comparative analysis of six Latin American countries. Clim. Pol. 19, 1297–1309. doi: 10.1080/14693062.2019.1661819
Ryan, D., and Ramírez Cuesta, A. (2016). ¿Qué sabemos sobre cambio climático y toma de decisiones en América Latina? Una revisión sistemática de publicaciones en revistas con referato. Toma de decisiones y cambio climático: acercando la ciencia y la política en América Latina y el Caribe. Montevideo, Uruguay: UNESCO, 18–33.
Sáenz, F., Hidalgo, H. G., Muñoz, Á. G., Alfaro, E. J., Amador, J. A., and Vázquez-Aguirre, J. L. (2023). Atmospheric circulation types controlling rainfall in the central American isthmus. Int. J. Climatol. 43, 197–218. doi: 10.1002/joc.7745
Sánchez Rodríguez, R., and Cavazos, T. (2015). Capítulo 1: Amenazas naturales, sociedad y desastres. En T. Cavazos (Ed.), Conviviendo con la Naturaleza: El problema de los desastres asociados a fenómenos hidrometeorológicos y climáticos en México. Mexico, Ediciones ILCSA, pp. 1–45.
Sánchez Rodríguez, R. A., and Fernández Carril, L. R. (2024). Climate-resilient development in developing countries. Curr. Opin. Environ. Sustain. 2024:101391. doi: 10.1016/j.cosust.2023.101391
Sanderson, K. (2023). Who should pay for open-access publishing? APC alternatives emerge. Nature 623, 472–473. doi: 10.1038/d41586-023-03506-4
Schröder, M., Lockhoff, M., Shi, L., August, T., Bennartz, R., Brogniez, H., et al. (2019). The GEWEX water vapor assessment: overview and introduction to results and recommendations. Remote Sens. 11:251. doi: 10.3390/rs11030251
Segura, L. D., van Zeijl-Rozema, A., and Martens, P. (2022). Climate change adaptation in Central America: a review of the national policy efforts. Latin Am. Policy 13, 276–327. doi: 10.1111/lamp.12277
Seneviratne, S. I., Zhang, X., Adnan, M., Badi, W., Dereczynski, C., Di Luca, A., et al. (2021). “Weather and climate extreme events in a changing climate” in Climate change 2021: the physical science basis. Contribution of working group I to the sixth assessment report of the intergovernmental panel on climate change. eds. V. Masson-Delmotte, P. Zhai, A. Pirani, S. L. Connors, C. Péan, and S. Berger, et al. (United Kingdom and New York, NY, USA: Cambridge University Press, Cambridge), 1513–1766.
Sepúlveda, S. A., and Petley, D. N. (2015). Regional trends and controlling factors of fatal landslides in LatinAmerica and the Caribbean. Nat. Hazards Earth Syst. Sci. 15, 1821–1833. doi: 10.5194/nhess-15-1821-2015
Sietsma, A. J., Groenendijk, R. W., and Biesbroek, R. (2023). Progress on climate action: a multilingual machine learning analysis of the global stocktake. Clim. Chang. 176:173. doi: 10.1007/s10584-023-03649-3
Silva, A. C. S., Galvão, C. O., and Silva, G. N. S. (2015). Droughts and governance impacts on water scarcity: an analysis in the Brazilian semi-arid. Proc. Int. Assoc. Hydrol. Sci. 369, 129–134. doi: 10.5194/piahs-369-129-2015
Silva Rodríguez de San Miguel, J. A., Martínez Díaz, E., and Monroy Becerril, D. M. (2021). The relationship between climate change and internal migration in the Americas. Manag. Environ. Qual. 32, 822–839. doi: 10.1108/MEQ-01-2021-0020
Simon, D. H., and Riosmena, F. (2022). “Environmental migration in Latin America” in International handbook of population and environment. International handbooks of population. eds. L. M. Hunter, C. Gray, and J. Véron, vol. 10 (Cham: Springer)
Singh, C., Ford, J., Ley, D., Bazaz, A., and Revi, A. (2020). Assessing the feasibility of adaptation options: methodological advancements and directions for climate adaptation research and practice. Clim. Chang. 162, 255–277. doi: 10.1007/s10584-020-02762-x
Sobel, A. H., Camargo, S. J., Hall, T. M., Lee, C.-Y., and Tippett, M. K. (2016). Human influence on tropical cyclone intensity. Science 35, 242–246. doi: 10.1126/science.aaf6574
SOCC (2020). The state of the Caribbean climate. Climate Studies Group Mona (eds.) Jamaica, West Indies: Produced for the Caribbean Development Bank.
Spinoni, J., Barbosa, P., Bucchignani, E., Cassano, J., Cavazos, T., Cescatti, A., et al. (2021). Global exposure of population and land-use to meteorological droughts under different warming levels and SSPs: a CORDEX-based study. Int. J. Climatol. 41, 6825–6853. doi: 10.1002/joc.7302
Spinoni, J., Barbosa, P., Bucchignani, E., Cassano, J., Cavazos, T., Christensen, J. H., et al. (2020). Future global meteorological drought hot spots: a study based on CORDEX data. J. Clim. 33, 3635–3661. doi: 10.1175/JCLI-D-19-0084.1
Stephenson, T. S., Taylor, M. A., Trotman, A. R., Charlton, C., Allen, T., Clarke, L. A., et al. (2022). Caribbean [in “state of the climate in 2021”]. Bull. Amer. Meteor. Soc. 103, S359–S361. doi: 10.1175/2022BAMSStateoftheClimate.1
Stewart, I., Maurer, E., Stahl, K., and Joseph, K. (2022). Recent evidence for warmer and drier growing seasons in climate sensitive regions of Central America from multiple global datasets. Int. J. Climatol. 42:19. doi: 10.1002/joc.7310
Surminski, S., and Oramas-Dorta, D. (2014). Flood insurance schemes and climate adaptation in developing countries. Int. J. Disast. Risk Reduct. 7, 154–164. doi: 10.1016/j.ijdrr.2013.10.005
Swithern, S. (2020). Extreme natural events mitigation: an analysis of the National Disaster Funds in Latin America. Front. Clim. 2:603176. doi: 10.3389/fclim.2020.603176
Thiede, B., Gray, C., and Mueller, V. (2016). Climate variability and inter-provincial migration in South America, 1970–2011. Glob. Environ. Chang. 41, 228–240. doi: 10.1016/j.gloenvcha.2016.10.005
Thomas, A., and Benjamin, L. (2019). Non-economic loss and damage: lessons from displacement in the Caribbean. Clim. Pol. 20, 715–728. doi: 10.1080/14693062.2019.1640105
Travis, W. R., Smith, J. B., and Yohe, G. W. (2018). Moving toward 1.5°C of warming: implications for climate adaptation strategies. Curr. Opin. Environ. Sustain. 31, 146–152. doi: 10.1016/j.cosust.2018.03.003
Trotman, A., Mahon, R., Van Meerbeeck, C., and Riley, E. (2021). Advancing drought risk management in the Caribbean: a multi-sectoral perspective. Background paper for the UNDRR global assessment report (GAR) 2021. Available at: https://www.preventionweb.net/publications/view/78462 (Accessed December 23, 2023).
Tuholske, C., Caylor, K., Funk, C., Verdin, A., Sweeney, S., Grace, K., et al. (2021). Global urban population exposure to extreme heat. Proc. Natl. Acad. Sci. U. S. A. 118:e2024792118. doi: 10.1073/pnas.2024792118
UNDRR (2021). Regional assessment report on disaster risk in Latin America and the Caribbean, United Nations Office for disaster risk reduction (UNDRR). Available at: https://www.undrr.org/media/48868/download?startDownload=true (Accessed December 23, 2023).
UNEP (2022). The adaptation gap report. Too little, too slow: climate adaptation failure puts world at risk. United Nations Environmental Programme: Belgium.
UNEP. (2023a). Adaptation gap report 2023: Underfinanced. Underprepared. Nairobi: Inadequate Investment And Planning On Climate Adaptation Leaves World Exposed.
UNEP (2023b). Emissions gap report 2023. Broken record: temperatures hit new highs, yet world fails to cut emissions (again). Belgium: United Nations Environmental Programme.
UNESCO (2021) in UNESCO science report: the race against time for smarter development. eds. S. Schneegans, T. Straza, and J. Lewis (Paris: UNESCO Publishing)
United Nations Foundation (2023). Compilation of illustrative targets and indicators for the Global Goal on Adaptation. United Nations Foundation: Washington, D.C.
Van Loon, A. F., Gleeson, T., Clark, J., Van Dijk, A. I. J. M., Stahl, K., Hannaford, J., et al. (2016). Drought in the anthropocene. Nat. Geosci. 9, 89–91. doi: 10.1038/ngeo2646
Vanos, J., Guzman-Echevarria, G., Baldwin, J. W., Bongers, C., Ebi, K. L., and Jay, O. (2023). A physiological approach for assessing human survivability and liveability to heat in a changing climate. Nat. Commun. 14:7653. doi: 10.1038/s41467-023-43121-5
Vargas, N., and Magaña, V. (2020). Warm spells and climate risk to human health in the Mexico City metropolitan area. Weather Clim. Soc. 12, 351–365. doi: 10.1175/WCAS-D-19-0096.1
Vega-García, H. (2004). Migración ambiental inducida por variabilidad climática y su tratamiento en las políticas públicas regionales: el corredor centroamericano de la sequía. Universidad Nacional. Available at: https://repositorio.una.ac.cr/handle/11056/21789 (Accessed December 23, 2023)
Vera, C., Higgins, W., Amador, J., Ambrizzi, T., Garreaud, R., Gochis, D., et al. (2006). Toward a unified view of the American monsoon systems. J. Clim. 19, 4977–5000. doi: 10.1175/JCLI3896.1
Vicuña, S., Gil, M., Melo, O., Donoso, G., and Merino, P. (2018). Water option contracts for climate change adaptation in Santiago, Chile. Water Int. 43, 237–256. doi: 10.1080/02508060.2017.1416444
Vignola, R., Esquivel, M. J., Harvey, C., Rapidel, B., Bautista-Solis, P., Alpizar, F., et al. (2022). Ecosystem-based practices for smallholders’ adaptation to climate extremes: evidence of benefits and knowledge gaps in Latin America. Agronomy 12:2535. doi: 10.3390/agronomy12102535
Wang, C., Lee, S.-K., and Enfield, D. B. (2008). Atlantic warm Pool acting as a link between Atlantic multidecadal oscillation and Atlantic tropical cyclone activity. Geochem. Geophys. Geosyst. 9:Q05V03. doi: 10.1029/2007GC001809
Wang, G., Wu, L., Mei, W., and Xie, S. P. (2022). Ocean currents show global intensification of weak tropical cyclones. Nature 611, 496–500. doi: 10.1038/s41586-022-05326-4
Warner, K., and van der Geest, K. (2013). Loss and damage from climate change: local-level evidence from nine vulnerable countries. Int. J. Global Warm. 5, 367–386. doi: 10.1504/IJGW.2013.057289
WCRP (2024). Kigali declaration: climate science for a sustainable future for all. 01/2024. WCRP Open Science Conference, 23–27 October 2023. Kigali, Rwanda.
Weiler, F., Klöck, C., and Dornan, M. (2018). Vulnerability, good governance, or donor interests? The allocation of aid for climate change adaptation. World Dev. 104, 65–77. doi: 10.1016/j.worlddev.2017.11.001
WMO (2022). State of the climate in Latin America and the Caribbean 2021. WMO-No. 1295. Geneva. Available at: https://library.wmo.int/idurl/4/58014 (Accessed December 23, 2023).
WMO (2023). State of the climate in Latin America and the Caribbean 2022. WMO-No. 1322. Available at: https://library.wmo.int/idurl/4/66252 (Accessed December 23, 2023).
Keywords: adaptation challenges, Latin America and the Caribbean, climate change, climatic impact-drivers, gaps and commonalities, risks, climate resilience
Citation: Cavazos T, Bettolli ML, Campbell D, Sánchez Rodríguez RA, Mycoo M, Arias PA, Rivera J, Reboita MS, Gulizia C, Hidalgo HG, Alfaro EJ, Stephenson TS, Sörensson AA, Cerezo-Mota R, Castellanos E, Ley D and Mahon R (2024) Challenges for climate change adaptation in Latin America and the Caribbean region. Front. Clim. 6:1392033. doi: 10.3389/fclim.2024.1392033
Edited by:
Sirkku Juhola, University of Helsinki, FinlandReviewed by:
Daniel Andres Rodriguez, Federal University of Rio de Janeiro, BrazilSimone Lucatello, Mora Institute, Mexico
Copyright © 2024 Cavazos, Bettolli, Campbell, Sánchez Rodríguez, Mycoo, Arias, Rivera, Reboita, Gulizia, Hidalgo, Alfaro, Stephenson, Sörensson, Cerezo-Mota, Castellanos, Ley and Mahon. This is an open-access article distributed under the terms of the Creative Commons Attribution License (CC BY). The use, distribution or reproduction in other forums is permitted, provided the original author(s) and the copyright owner(s) are credited and that the original publication in this journal is cited, in accordance with accepted academic practice. No use, distribution or reproduction is permitted which does not comply with these terms.
*Correspondence: Tereza Cavazos, tcavazos@cicese.mx
†These authors have contributed equally to this work