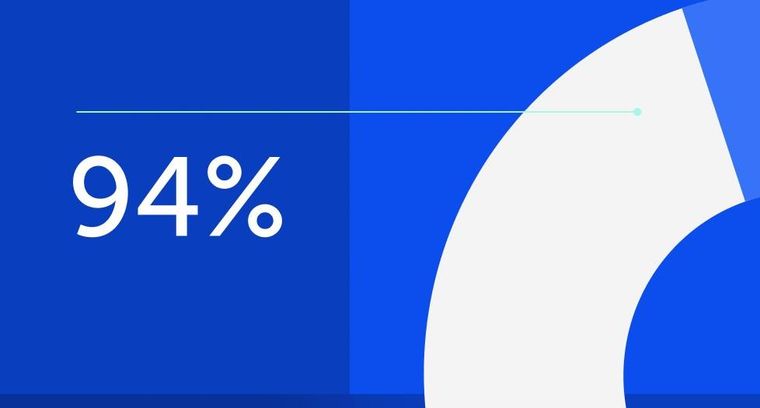
94% of researchers rate our articles as excellent or good
Learn more about the work of our research integrity team to safeguard the quality of each article we publish.
Find out more
ORIGINAL RESEARCH article
Front. Clim., 29 April 2024
Sec. Predictions and Projections
Volume 6 - 2024 | https://doi.org/10.3389/fclim.2024.1368413
This article is part of the Research TopicCoastal Climate Monitoring and Downscaling for Adaptation Planning in the Adriatic SeaView all 12 articles
The Adriatic Sea, characterized by unique local features in comparison to the broader Mediterranean Sea, stands out as a highly susceptible region to climate change. In this context, our study involves a focused climate downscaling approach, concentrating on the Adriatic water cycle. This encompasses integrated modeling at the mesoscale, covering the atmosphere, hydrology, and marine general circulation. The study period spans from 1992 to 2050, considering the high emission scenario RCP8.5. We aim at evaluating how the river release projection affects the local density stratification and the sea level rise. Indeed, the river release is found to decrease by approximately 35% in the mid-term future and condition the stratification of the water column with differences between the Northern and Southern sub-basins. The projected runoff decrease has a major impact on the Northern sub-basin, where the stratification is haline-dominated and the foreseen salinization prevails on the heating through the whole water column. Conversely, the runoff decrease has a lower impact on the Southern sub-basin, where the future changes of other mechanisms may play a major role, e.g., the changing properties of the Mediterranean water entering the Otranto Strait and the foreseen heating prevails on the salinization from the intermediate to deep water column. The study provides the first evidence of how the decreasing river discharge locally reduces the density stratification, increases the dense water, and mitigates the sea level rise in the Northern Adriatic Sea, thus acting in the opposite direction to the global warming. To minimize uncertainty in coastal ocean projections around the world, it is essential that the climate downscaling integrates high-resolution hydrology and hydrodynamics models to correctly reproduce the link between surface buoyancy and stratification and the resulting dynamics.
The state-of-the-art Global Climate Models (GCMs), provide the primary source of information to understand and investigate the climate change at the global scale. However, they cannot provide a reliable representation of the global coastal ocean and of the local climate impacts due to the coarseness of their time and space resolution, lacking in the representation of physical processes and simplified or neglected links among the Earth system components (Holt et al., 2017).
The dynamical and the statistical downscaling offer a strategy to refine the coastal ocean response to climate change and infer small scale features from large-scale information of GCMs. Downscaling with physical models, used within ensemble and coupling approaches, is largely used to provide future projections supporting climate impact assessment and definition of adaptation strategies at a very local scale. In addition, machine learning algorithms are among the newest and most promising tools for statistically downscaling data at different scales.
However limits to the climate downscaling with physical models persist, e.g. the computational costs, the resolution jumps between driving and nested climate models, the model uncertainty treatment and the deficiency of coastal monitoring, among the others (Drenkard et al., 2021).
The density stratification in the global coastal ocean is a critical and highly vulnerable characteristic that influences dynamics and biogeochemistry at local scales. Accurate representation of seasonal variations in density stratification is essential for comprehending the changing conditions of the coastal ocean and marine ecosystems across different climate change scenarios. Existing research on regional climate downscaling underscores a prevailing trend of increased seasonal stratification in response to global warming. Holt et al. (2022) provided a review of 45 published future climate downscaling studies in regional seas around the world, and most of them reporting a consistent increase in future stratification mainly triggered by the projected increase in sea temperature and the freshening of Artic and Atlantic water.
Nevertheless, none of them considers the Mediterranean region and its sub-basins. Parras-Berrocal et al. (2022) analyzed the effect of climate change on the stratification in the North Western Mediterranean using a Regional Climate Model (RCM) and demonstrated a strong increase in the future stratification caused by changes in temperature and salinity properties of Modified Atlantic Water and Levantine Intermediate Water with a consequent collapse of dense water formation by 2040–2050 under RCP 8.5. Denamiel et al. (2020), Denamiel and Vilibić (2023), and Tojčić et al. (2024) proposed a limited area climate downscaling over the Adriatic Sea using a coupled atmosphere-ocean-wave modeling system at kilometer-scale and with a nested ocean-wave model at sub-kilometer scale. Their high resolution projections contrast with the previously published literature and suggest that there are understudied regions of the Adriatic coast which might be more vulnerable to sea level rise and increasing storm surges than well-researched areas, such as the Venice Lagoon. Despite these studies provide the most comprehensive climate projections over the Adriatic basin, the limited area downscaling is performed using the pseudo-global-warming (PGW) approach (Brogli et al., 2023) instead of the classical dynamical downscaling technique. Thus, the computational costs reduced but future variability and changes at local scales might be missed. Moreover, the future river discharge is not prognostically solved but it is based on historical data which have been climatologically changed on a monthly basis under RCP scenarios (following the method proposed by Macias et al. (2018).
It is well known that the river runoff makes the Adriatic Sea a dilution basin (Cushman-Roisin et al., 2008). Previous sensitivity studies have demonstrated that rivers play a key role on the Adriatic thermohaline circulation and dynamics. Among them, Vilibićet al. (2016) investigated how the overestimated runoff climatologies may unreasonably reduce or prevent the Adriatic local dense waters; Verri et al. (2018) demonstrated that a high runoff can make the surface buoyancy flux at the basin scale positive, reduce the local dense water in the Southern Adriatic sea, and reverse the regional overturning circulation; similarly Vodopivec et al. (2022) show that the salinity of the Adriatic surface water has a strong influence on dense water formation and circulation down to the bottom of the deepest part of the basin. In addition, they demonstrate that saltier freshwater balance produces larger peaks in dense water volume. Nevertheless, to the best of our knowledge, no studies still provide a faithful representation of the river release in the climate modeling of the Adriatic Sea dynamics, thus with no way to take into account how the decreasing river release combines with the other forcing mechanisms affected by the climate changes. We believe that this lack in modeling of the coastal water cycle of the Adriatic basin may prevent to advance the knowledge of the Adriatic present and future climate and evaluate the potential impacts of decreasing runoff and saltier freshwater balance on the local stratification and dynamics under climate change.
To fill this gap, based on physical models, a limited area climate downscaling has been designed and implemented over the Adriatic Sea within the AdriaClim project (https://www.italy-croatia.eu/web/adriaclim) with an integrated and high-resolution modeling of the local water cycle.
In this study, we use our modeling results to draw some straightforward conclusions on the potential effects of the river release projections with respect to the future stratification and dynamics of the Adriatic Sea.
The study area and the downscaling methodology are described in Section 2. The relevant numerical findings are analyzed in Section 3. Concluding remarks and insights for the new-generation climate downscaling are shown in Section 4.
The Adriatic Sea is a semi-enclosed elongated basin, approximately 800 km long and 150 km wide, located in the northern part of the Mediterranean Sea. It is characterized by a highly variable bathymetry which is shallow, approximately 30 m, in the northern Adriatic (NAd) with a very weak bathymetric gradient and gradually increases toward the 280 m deep Pomo (or Jabuka) Pit in the central part and up to approximately 1,270 m deep Southern Adriatic Pit. The Palagruža Sill connects the Middle Adriatic (MAd) and South Adriatic (SAd) Pits, whereas the Otranto Strait connects the Adriatic with the Northern Ionian (NI) Sea. On the western side, the coastal morphology is regular and characterized mainly by sandy beaches, while the eastern coastline is irregular and characterized by rocky coastlines with a considerable amount of islands (Artegiani et al., 1997a). The main forcings of the basin circulation are the surface buoyancy flux, the wind stress and the energy and mass exchanges through the Strait of Otranto. The latter includes the salty and warm waters of Levantine origins entering the Strait at intermediate depths (Modified Levantine Intermediate Waters, MLIW) and playing a key role in the local dense water formation (Cushman-Roisin et al., 2008).
Three surface cyclonic gyres dominate the northern, middle, and southern basin circulations, respectively, with seasonal variability (Artegiani et al., 1997b; Poulain, 2001). The Po river release in the NAd drives the outward Western Adriatic Coastal Current (WACC) along the western coast. On the other hand, the inward Eastern Southern Adriatic Current (ESAC) flows along the eastern side of the Adriatic Sea reaching the MAd: and it transports the water masses entering the Otranto strait from the Mediterranean Sea and partially collects the outflow of large rivers located in northern Albania and southern Croatia.
The three-dimensional thermohaline circulation consists of a main antiestuarine overturning cell located between the SAd and the NI Sea and driven by the SAd sink of dense water. Dense water formation sites are located in all the three sub-basins with the SAd being one of the three main formation sites of the whole Mediterranean Sea in terms of volume rate. A secondary estuarine cell is located at the sea surface between the NAd and the MAd and driven by the local buoyancy gain.
The Adriatic Sea receives more than 30% of the Mediterranean Sea runoff, mainly coming from the Po river providing almost one-third of the total runoff of the Adriatic Sea. Other important rivers are located in the Northern part of the Italian coastline and the eastern side in northern Albania and southern Croatia. The riverine freshwater input makes the Adriatic Sea a “dilution basin,” i.e., its freshwater budget, defined as evaporation minus precipitation and runoff, and has a negative annual mean of approximately −1m/year (Artegiani et al., 1997b; Verri et al., 2018). The Adriatic surface buoyancy flux, which combines by definition the net heat flux and the net freshwater gain (Cessi et al., 2014), is slightly negative on an annual basis but it may become zero or positive when large river runoff occurs (Pinardi et al., 2006), with the consequence of weakening and eventually reversing the basin overturning circulation cell (Verri et al., 2018).
A limited area climate downscaling is proposed over the Adriatic Sea using an integrated modeling system solving the meso-scale with uncoupled atmosphere, land, hydrology, hydrodynamics, waves, and biochemistry earth system components.
The driving regional climate model RCM considered is provided by the Med-CORDEX coordinated initiative (Ruti et al., 2016), i.e., the named LMDz-NEMOMED system (L'Hévéder et al., 2013). It consists of the atmospheric model LMDz4-regional (Li et al., 2012) with approximately 30 km horizontal resolution, a land surface submodel ORCHIDEE with the same resolution as LMDz4, and the ocean model NEMOMED8 (Beuvier et al., 2010) with approximately 10km horizontal resolution. The limited area multi-model LAM we nested within LMDz-NEMOMED system is designed to solve the mesoscale processes over the Adriatic Sea and consists of six modeling components: the atmosphere (WRF, Skamarock et al., 2008), the land surface (NOAH, Niu et al., 2011), and the hydrology (WRF-Hydro, Gochis et al., 2020) components share the same computational domain over the Central Mediterranean Sea with 6 km as horizontal resolution till 600 m along the river networks (Figure 1 left); the marine hydrodynamics (NEMO, Madec and team, 2017; Aumont et al., 2018), waves (WW3), and biochemistry (BFM, Vichi et al., 2007, 2020) components cover a smaller area over the Adriatic Sea, with an open boundary south of the Otranto Channel and approximately 2 km horizontal resolution (Figure 1 right). This grid spacing (i) ensures a proper resolution ratio to perform the subregional climate downscaling and (ii) makes the LAM of the AdriaClim project capable to represent the mesoscale processes and air-sea-land interactions occurring at these scales. Moreover, the Adriatic LAM is intended to provide climate simulations with higher time frequency than the driving RCM: the atmospheric fields have 6 h frequency, the hydrology fields have 1 h, and the hydrodynamics fields have 3 h at the sea surface and daily over the water column.
Figure 1. The computational domains of the limited area climate downscaling. The WRF, NOAH, and WRF-Hydro models share the domain on the left side. The NEMO, BFM, and WW3 models share the domain on the right side.
Figure 2 shows the workflow of the multi-model system used to perform the limited area climate downscaling.
The LAM components have demonstrated good skills in reproducing the present climate in the Adriatic region (see Supplementary material on the calibration and validation tests of the modeling components).
The driving RCM and the nested LAM scenarios are in the high-emission pathway RCP8.5. The switch of the climate experiments from “historical” to “scenario” mode occurs in 2006. This means that the GHG concentration dataset (released within CMIP5) that enters the model parameterization of the long wave radiation consists of climatological values till 2005 while projected values are based on RCP 8.5 starting from 2006.
The approach for climate downscaling employed in this study is relatively novel. It aims to refine the simulated field during the historical period to closely align with observations. This correction process is extended to projections as well. To achieve this, the Adriatic LAM components are interconnected in a one-way mode, allowing hydrology and marine circulation to utilize the most accurate estimates of atmospheric fields.
Showing all the details on the model sensitivity and the evaluation of the preprocessing, running, and postprocessing phases of the climate downscaling is out of the scope of this study and can be retrieved from the AdriaClim Project database (see the Data Availability Statement section).
Two time slice simulations have been carried out with all the LAM components and are discussed here. The WRF model runs as first and has been bias-corrected. The climate simulations cover the range 1990–2050 with reinitialization in 2020, and the starting years of the two time slices 1990–2020 and 2020–2050 are considered as spin-up periods. Doing this, the time slice experiments with the hydrological and the ocean components are initialized 1 year and 2 years later, respectively. The reinitialization strategy allows to reduce the computational costs by running the past and future climate experiments simultaneously and minimizes the potential climate drifts when the modeling components are integrated for several decades.
An asset of the proposed LAM over the Adriatic Sea is that it offers for the first time a prognostic and fully distributed modeling of approximately 145 catchments ending into the Adriatic Sea, i.e., the ones with a basin area major than 70km2. This is made possible by the lateral water routing systems offered by WRF-Hydro (a 2-dimensional overland waterflow and a 1-dimensional channel streamflow are solved with diffusive shallow-water equations) and the very high resolution adopted for hydrological modeling, i.e., 600 m.
A subset of 71 rivers has been selected within the 145 rivers solved by WRF-Hydro as representative of the Adriatic riverine sources and used to force the marine components NEMO and BFM in a reasonable and trustable way. This subset follows some constraints: a lower threshold has been introduced and only rivers with catchment areas greater than 500km2 are considered as they have been either calibrated or their behavior was compared with historical observed or reconstructed (EFAS) data. The Sile, Rijecina, Dubracina, Jadro, Uso, and Rubicone rivers make exceptions to this rule, and they are maintained in the subset because (i) their runoff is>10m3s−1 or (ii) they enter the Adriatic basin close to each other with a total non-negligible runoff in the adjacent shelf sea. Figure 3 shows the WRF-Hydro active catchments ending into the Adriatic Sea and embedded in the final subsetting.
Figure 3. Sketch of the 71 catchments, among the 145 ones prognostically solved by WRF-Hydro, which provide river release to NEMO model.
It is well known that rivers affect the so-called “barrier layer” of the global ocean (Sprintall and Tomczak, 1992). There have been various studies highlighting that the non-seasonal river discharge influences the physics and biochemistry of the regional seas (Skliris et al., 2007; Fournier et al., 2016; Piecuch et al., 2018a; Dandapat et al., 2020) to the global ocean (Chandanpurkar et al., 2022), with measurable impacts on sea surface salinity and sea surface height on multiple timescales, from daily basis to decadal range.
However, the way river release may affect the long term projections of the coastal ocean is still ignored or represented in a highly simplified way. Indeed, some regional climate models include a 1D river-routing modeling component, which converts the surface runoff of the land surface model into river discharge values at river mouths but with a very coarse resolution, i.e., 0.5 deg at most (Anav et al., 2024), and this often requires local adjustment to prevent unreasonable freshwater inputs (Sevault et al., 2014). Other studies applied climate change deltas to historical runoff values in order to statistically infer the future river release (Denamiel et al., 2020; Denamiel and Vilibić, 2023; Tojčić et al., 2024).
The AdriaClim project has provided a first attempt (i) to predict the water budget of all the major catchments surrounding the Adriatic sea with a fully distributed modeling of land surface and subsurface waters and (ii) to provide the net release at river mouths ending into the Adriatic Sea, as detailed in the section above.
River runoff values provided by the WRF-Hydro model along the river networks have been extracted at the closest points to the river mouths, and they enter the NEMO model surface boundary conditions for the vertical velocity and the diffusive salt flux. A constant value of salinity is prescribed at river mouths while no river temperature information is provided to NEMO; this is a fair assumption as the Mediterranean river plumes are driven by the salinity gradient. Salinity values have been chosen equal to 15 psu for all rivers, except for the Po river where 17 psu is considered. These values are based on the analysis of salinity profiles measured at river mouths (Simoncelli et al., 2011) and the center of the basin (Oddo et al., 2005). We plan to further refine this methodology in the future by providing reasonable values of temperature and salinity at the river mouths by means of an intermediate box modeling approach which solves the estuarine water exchange (Verri et al., 2020, 2021; Maglietta et al., 2024 under review).1
A seasonal comparison between the downscaled LAM and the driving RCM is shown in Figure 4 in terms of Sea Surface Temperature SST, Salinity SSS, Total Sea level TSL, and Mixed Layer Depth MLD, having the Copernicus Mediterranean Forecasting System reanalises as a benchmark. There is a qualitative agreement between the two models in the representation of the seasonal cycle of the SST and TSL over the historical slice 1992–2011 (Figure 4, first and third panels). Nevertheless, the downscaled SSS (Figure 4, second panel, blue line) is found to be lower than the driving RCM through all seasons, and the diagnosed MLD is thinner (Figure 4, fourth panel, blue line). Both SSS and MLD are found to be more realistically represented by the downscaled LAM using reanalysis as a benchmark with RMSE index equal to 0.1 psu and 4.7 m, respectively, while the RMSE values of the driving RCM are 0.5 psu and 25.5 m.
Figure 4. Comparison of driving MedCordex RCM (black), downscaled AdriaClim LAM (blue), and CMEMS Reanalises (red). Average day-of-year over the historical time slice 1992–2011 for: sea surface temperature, sea surface salinity, total sea level, and mixed layer depth.
The lower salinity content and the higher stratification of the top of the water column are quite expected results as the downscaled LAM has a higher time and space resolution (both in the horizontal and the vertical direction) than the driving RCM but also a more accurate representation of the freshwater buoyancy inputs since a highly detailed reconstruction of the Adriatic river release is considered.
These results hint that the limited-area climate downscaling here proposed may enhance the understanding of the Adriatic Sea climate.
Two 20-year long climate time slices, 1992–2011 and 2031–2050, have been extracted from the AdriaClim climate simulations for comparing the mid-term projection with respect to the past climate.
In the historical slice, we retained a few years (2006–2011) of projected GHG emission data to extend the time slice to 20 years without altering the average behavior of the main variables analyzed, as evident in the computation of the climate change indicators by Fedele et al. (2024) and Santos da Costa et al. (2024) (under review).2 Moreover, we took out the first years of the projection experiment to exclude any “step-like” unreasonable effect. In fact, the modeling system was reinitialized in 2020.
The analyses of the results proposed here focuses on the seasonal and inter-seasonal changes of relevant variables with the seasons defined for the Adriatic Sea as follows: winter is January–February–March, spring is April–May–June, summer is July–August–September, and autumn is October-November-December.
We focus on the near surface atmospheric fields to identify kilometer-scale atmospheric trends that may affect the Adriatic climate. We found that the seasonal trends of the near surface atmosphere have a strong land-sea difference.
There is a clear positive trend of the 2m air Temperature (Figure 5A) and negative trend of 2m Relative Humidity (Figure 5B) through all seasons over the sea. A lower but still positive trend of 2m air Temperature is identified over the land but with seasonal variability over the Balkans and the North Africa. In the same land areas, the 2m Relative Humidity shows a strong positive trend in all seasons. The seasonal change in the rainfall patterns (Figure 5C) indicate an overall decrease in the cumulated rainfall on the Italian peninsula, especially over the Pianura Padana while a positive trend is observed over the Balkans through all seasons. By focusing on the Adriatic Sea and the hydrological catchments surrounding the basin, a general decrease in precipitation is observed with the only exception of the western side in autumn.
Figure 5. Seasonal change between projection (2031–2050) and historical (1992–2011) ranges for (A) the 2m air temperature (degC), (B) the relative humidity (%), (C) the total rainfall (mm).
The seasonal change in the latent heat flux in the Adriatic Sea (negative defined in the downward direction, see Figure 6) shows an increasing heat loss by evaporation in the mid term which is coherent with the near surface air temperature and relative humidity trends (while no relation with the seasonal changes in the near surface wind provided as Supplementary material), but its seasonal change is less homogeneous and reduces or even reverses sign in the areas of freshwater influence, where the density stratification is expected to reduce (see the next section results), and it may occur that the local seasonal trend of the sea surface temperature is less pronunced than the ones of air temperature and relative humidity.
Figure 6. Seasonal change between projection (2031–2050) and historical (1992–2011) ranges for the Latent Downward Heat flux (Wm−2).
The future changes in the riverine release may play a key role in modulating the response of a coastal basin to the changing climate. The top panel of Figure 7 depicts the average-day-of-year for the total river discharge throughout the historical and projected timeframes. It indicates that this freshwater input is expected to decrease by 2050 of approximately 37% on an annual basis, with the most substantial reduction occurring during winter and spring. Specifically, the Po River, contributing nearly one-third of the total discharge, is expected to reduce its flow by approximately 35% (bottom panel of Figure 7) (all rivers ending into the Adriatic basin show negative trends, and the rivers on the eastern side have a slightly lower one. The related dataset is available on the AdriaClim public repository on ERDDAP server).
Figure 7. River release (m3/s) into the Adriatic Sea. (Top) Total river release, (Bottom) Po river release. Black (blue) line is the average day-of-year over the historical (projection) time slice.
Seasonal changes in sea surface temperature and salinity in the mid term future (Figure 8) show positive trends through all seasons. The sea surface temperature changes are quite homogeneous over the entire basin and resemble the ones of the near surface atmospheric fields with no relevant differences among the sub-basins (Figure 8A), while the variations of the sea surface salinity are heterogeneous with the highest increase, more than 2 psu, in the regions of freshwater influence as a result of the reducing river discharge (Figure 8B).
Figure 8. Seasonal change between projection (2031–2050) and historical (1992–2011) ranges for (A) the sea surface temperature (degC), (B) the sea surface salinity (psu), (C) the maximum brunt vaisala frequency over the top 100 m depth (s−2).
The seasonal variations of the maximum Brunt Vaisala Frequency in the top 100 m are shown in Figure 8C. The future changes in the Adriatic stratification resemble the ones on SSS with local differences and the strongest effect in the Northern sub-basin. The loss of stratification affects the entire basin in summer and autumn, while in winter and spring, it is relevant on the shelf areas influenced by the main river discharge.
Looking at the regions of freshwater influence, a strong connection can be noticed between lowering stratification at the top of the water column (i.e., the high negative N2) and growing salinity, particularly in winter and autumn. Here, river influence plays a key role, making salinization to prevail on heating under lowering runoff projection.
Conversely, the inner basin in the Middle and Southern regions exhibits a less prominent decline in stratification during summer and autumn. It even shows a slight local increase in stratification during winter and spring, coinciding with the period of the most substantial evaporation rise (as indicated by the dark blue patch in Figure 6). This implies that heating outweighs salinization in the upper 100 meters of the water column. One contributing factor is the lower sensitivity of the inner basin of the Southern Adriatic to runoff changes. Additionally, alternative stratification mechanisms may take precedence. Thus, we extend the stratification analysis to the entire water column by looking also at the effect on dense water formation, and we investigate the future changes in the properties of the MLIW entering into the eastern side of the Otranto Strait.
The profiles of the mean N2 in the NAd (panels of Figure 9) confirm the high loss of stratification through all seasons and the whole column.
Figure 9. Seasonal stratification of the Northern Adriatic water column. Historical (black) and projection (blue) profiles of the mean Brunt Vaisala Frequency (s−2).
Similarly, the panels of Figure 10 display the seasonal profiles in the SAd: here, the spatially average N2 profile hints a lowering stratification through all seasons up to 100 m depth. Looking at the intermediate to deep water column, the stratification of the SAd is expected to increase especially in winter, meaning the heating effect prevails on salinization.
Figure 10. Seasonal stratification of the Southern Adriatic water column. Historical (black) and projection (blue) profiles of the mean Brunt Vaisala Frequency (s−2).
We analyzed dense water formation by computing the volume of water in each sub-basin with the potential density anomaly, PDA, equal to or larger than a threshold value, i.e., 29.0kgm−3. The top panels of Figures 11, 12 indicate that the volume rate of dense water formation is expected to increase in the NAd by 2050 and decrease in the SAd. Percentages of +150% and −24% are found respectively. If we consider a more restrictive PDA threshold for the NAd dense water volume, 29.2kgm−3 based on previous studies (Artegiani et al., 1997b; Mantziafou and Lascaratos, 2004; Vilibićet al., 2016), the percentage increase is even higher, i.e., +200%.
Figure 11. Dense water formation in the Northern Adriatic sub-basin. (Top) Volume rate (km3/day), (Middle) Temperature, and (Bottom) Salinity. Black (Blue) line is the average day-of-year over the historical (projection) time slice 1992–2011 (2031–2050).
Figure 12. Dense water formation in the Southern Adriatic sub-basin. (Top) Volume rate (km3/day), (Middle) Temperature, and (Bottom) Salinity. Black (Blue) line is the average day-of-year over the historical (projection) time slice 1992–2011 (2031–2050).
It is interesting to notice that both NAd and SAd deep waters are expected to be saltier (Figures 11 bottom, 12 bottom) and warmer (Figures 11 middle, 12 middle).
To the best of our knowledge, this is the first study showing an increase in the Northern Adriatic dense water in the mid-term future. Previous studies are conditioned by a number of limitations preventing a faithful representation of the Adriatic thermohaline properties, especially in the Northern sub-basin, e.g., the coarse spatial resolution of the ocean model and its driving atmospheric forcings (Dunić et al., 2019, 2022) and the misrepresentation of the future riverine release which is based on a statistical approach or derived by a simplified and coarse resolution dynamical approach (Tojčić et al., 2024).
We tried to explain why the future changes on N2 and dense water formation differ between the NAd and SAd and derive some straightforward conclusion on the future thermohaline circulations of the Adriatic sub-basins. In the NAd sub-basin, where most of the Adriatic river release is located, the foreseen runoff decrease and a slight increase in the surface heat loss by evaporation (Figure 6) are found to reduce stratification of the whole water column, especially in the areas of freshwater influence, enhancing water sinking and local dense water volume rate. The NAd Dense Water is found to become saltier but also warmer in the mid term, supporting the conclusion that the river release plays a major role in determining the local thermohaline circulation and the future changes in the NAd stratification (Figure 9) are haline-driven.
Vice-versa in the SAd sub-basin, the runoff decrease and the evaporation increase (particularly high in the SAd in winter time, see Figure 6 left) are found to reduce stratification in the mid-term future till 200 m depth. Moreover, the intensifying Southern Adriatic gyre (see seasonal maps of surface circulation in Supplementary material) enlarges the potential surface where the increasing heat losses may trigger vertical convection. In contrast, a stratification increase is observed in the deeper water column (Figure 10), especially in winter time.
Thus, we speculate that the future lowering of water sinking and dense water formation in the SAd are mainly driven by the projected change in the salt MLIW entering into the Eastern flank of the Otranto strait during summer and autumn.
Model results indicate that the water inflowing through the Otranto strait at intermediate depth will be confined at approximately 100 m depth, warmer (see Supplementary material) and less salty (Figure 13).
Figure 13. Seasonal change between projection (2031–2050) and historical (1992–2011) ranges for the Salinity at the Otranto channel (i.e., 40°N).
Overall, the projected decrease in river release affects the SAd stratification only at the top of the water column (Figure 10). However, the changes in the deep water stratification and dense water formation are thermal-driven and conditioned by the changing properties of the Mediterranean waters entering at the Otranto channel.
The haline contraction and the thermal expansion of the Adriatic water column, which are driven by the sea surface salinization and heating, respectively, act in the opposite way on the resulting density stratification and, consequently, the Total Sea Level (TSL) changes.
In details, the TSL is defined as the sum of an incompressible or mass component, i.e., the sea surface height SSH solved by the ocean models under the incompressible assumption, and the compressible or steric component that accounts for the thermosteric (i.e., the thermal expansion due to temperature variations) and halosteric (i.e., the haline contraction due to salinity variations) contributions (Pinardi et al., 2014).
An additional contribution to the TSL is the vertical land movement, i.e., the glacial isostatic adjustment and the land subsidence, which can be relevant at local scales (Galassi and Spada, 2014; Piecuch et al., 2018b; Zanchettin et al., 2021), but they are neglected within this study.
We found that the magnitude of the Adriatic sea level rise by 2050 is driven by the steric components with differences among sub-basins.
Our results hint that the mass component (SSH) is expected to decrease in the mid term through all seasons (Figure 14B), and we found this is the result of the surface loss of freshwater volume flux owing to the slightly lowering precipitation, the highly lowering runoff, and the increasing evaporation rate. Indeed, the highest reduction of the SSH is up to 10cm in the Northern Adriatic Sea. Moreover, a local minimum is found in the core of the Southern Adriatic cyclonic gyre, and it is explained with the intensifying SAd cyclonic gyre which favors the local upwelling and divergence (Figure 14B).
Figure 14. Seasonal change between projection (2031–2050) and historical (1992–2011) ranges for (A) the Total Sea Level (cm) and (B) the Sea Surface Height (cm).
Looking at the water exchange at the Otranto strait, the net outflow over the historical range is consistent with the literature, i.e., −3*10−3Sv (Mantziafou and Lascaratos, 2004; Verri et al., 2018), while it reduces over the projection range, −0.7*10−3Sv, meaning more water will enter the Adriatic basin through the Otranto Strait but not enough to balance the freshwater volume losses at the sea surface.
We diagnosed the TSL by adding the steric components. Our results on the seasonal changes suggest that the TSL will rise at basin scale through all seasons, with a mean annual rate of approximately +3mm/year by 2050 (Figure 14A) and local rate up to +5mm/year in the SAd where the thermal expansion prevails.
A mitigated TSL rise is found in the NAd, i.e., −0.7mm/year, as it is mostly influenced by the lowering freshwater release which reduces the incompressible component and increases the haline contraction making it prevailing of the thermal expansion. As stated above, the TSL results presented here are limited by the lack of land subsidence, which has not been considered but may play a key role at local scale (Piña-Valdés et al., 2022), e.g., by contributing to the local Sea Level Rise (SLR) in the Venice lagoon of approximately 50% (Zanchettin et al., 2021). Moreover, we argue that the contribution of the Terrestrial Ice Melting is underestimated as it mainly enters as remote effect through the barotropic transport at the ocean model open boundary located at south of the Otranto Strait, and thus, it depends on the quality of the regional climate forcing.
The main result of our study is the first depiction of the spatial variability of the Adriatic SLR, owing to a prognostic representation of the riverine release into the Adriatic Sea till 2050 (more than 70 rivers with hourly frequency are considered), which allows that concluding the SL incompressible component reduces through all basin with the highest effect on the Northern Adriatic Sea, and the haline contraction prevails on the thermal expansion in the Northern Adriatic and vice-versa in the Southern one.
The kilometer-scale and integrated climate downscaling we proposed over the Adriatic Sea is one of the first attempts to provide a comprehensive and high resolution climate modeling of the coastal water cycle which prognostically solves all the major catchments ending into the Adriatic basin.
This study demonstrates that the decrease of river runoff, along with the enhancement of evaporation, is a major mechanism for the projected salinity increase in the Adriatic basin till 2050.
The mid-term scenario provides evidence of the competition between the runoff decrease and the sea surface temperature increase for determining a haline-driven or a thermal-driven stratification: a haline-driven stratification is found to prevail in the NAd where the decreasing runoff is found to weaken the local stratification and enhance the local dense water formation, vice-versa the future changes in the properties of water entering the Otranto Strait are found to strongly contribute to increase the stratification of the intermediate to deep water column in the SAd, thus reducing the local water sinking and the dense water formation.
Moreover, the projection of the riverine release into the Adriatic Sea till 2050 allowed us to capture the spatial variability of the Adriatic SLR and conclude that the haline contraction prevails on the thermal expansion in the Northern Adriatic Sea, with a resulting modulating effect on the total SLR. In the Southern Adriatic Sea, the steric contribution to the total SLR is driven by the thermal expansion.
These detailed projections on the physics of the Adriatic Sea can be employed to properly support the projection of the biogeochemical dynamics, Mentaschi et al. (2024) (submitted), and hyper-resolution coastal vulnerability studies, e.g., the shoreline evolution (Mannarini et al., 2024) (submitted) and the salinization of inland water (Verri et al., 2024) (submitted).
The main lessons of the climate downscaling exercise proposed in this study can be summarized as follows:
• The future changes in the near-surface atmosphere and the thermohaline properties of the Adriatic Sea show different behaviors under global warming. The former is quite homogeneous, and the latter is more heterogeneous and mainly affected by the reducing river release in the Northern sub-basin and the changing properties of water flows through the Otranto Strait in the Southern sub-basin.
• A decrease of the river release in coastal and marginal seas acts in the opposite direction to the global heating by weakening the density stratification, increasing the dense water formation, and reducing the total sea level rise.
Hence, the overall insight is that the new generation climate downscaling benefits from high space and time resolutions and a more comprehensive representation of the local water cycle.
Finally, our climate LAM has been nested within a single Med-CORDEX RCM, and a single climate scenario has been carried out thus with no possibility to quantify the climate uncertainty. To get more robust information on future changes under a climate scenario, an ensemble of downscaled systems is necessary. While the building of a reliable Med-CORDEX ensemble with the last generation CMIP6 projections is ongoing, most datasets produced within Med-CORDEX and other regional climate downscaling initiatives have been only partially stored, thus they are not suitable for further local downscaling. The AdriaClim project aims at contributing to shed light on the importance of defining best practices and shared rules for the new generation regional-to-local climate downscaling to provide trustable scenarios with very high time and space details.
The datasets presented in this study can be found in online repositories. The names of the repository/repositories and accession number(s) can be found in the article/Supplementary material.
GV: Conceptualization, Data curation, Formal analysis, Funding acquisition, Investigation, Methodology, Project administration, Resources, Software, Supervision, Validation, Visualization, Writing –original draft, Writing –review & editing. LF: Data curation, Investigation, Software, Visualization, Writing –review & editing. MG: Data curation, Investigation, Software, Writing –review & editing. AS: Formal analysis, Investigation, Software, Writing –review & editing. VS: Data curation, Formal analysis, Investigation, Writing –review & editing. AD: Data curation, Validation, Visualization, Writing –review & editing. GF: Data curation, Investigation, Writing –review & editing. IM: Data curation, Investigation, Writing –review & editing. LM: Formal analysis, Writing –review & editing. EC: Formal analysis, Writing –review & editing. GC: Funding acquisition, Supervision, Writing –review & editing. PM: Methodology, Supervision, Writing –review & editing. GM: Supervision, Writing – review & editing. NP: Funding acquisition, Methodology, Supervision, Writing –review & editing.
The author(s) declare that financial support was received for the research, authorship, and/or publication of this article. This work has received funding from the AdriaClim project, Italy (Climate change information, monitoring and management tools for adaptation strategies in Adriatic coastal areas; project ID 10252001).
The authors are grateful to the AdriaClim project Coordinator Dr. Andrea Valentini and the leading Partner Agency for Prevention, Environment, and Energy of Emilia-Romagna (Arpae). The authors would like to thanks Rita Lecci and Alessandro De Donno from OPA Division of CMCC Foundation for the setting up and the maintenance of the AdriaClim Project repository on ERDDAP Server. Finally, the authors would like to thank Samuel Somot from Meteo-France/CNRS and Laurent Li from IPSL for sharing the CNRS-IPSL MedCordex RCM dataset used to drive the AdriaClim modeling system.
The authors declare that the research was conducted in the absence of any commercial or financial relationships that could be construed as a potential conflict of interest.
All claims expressed in this article are solely those of the authors and do not necessarily represent those of their affiliated organizations, or those of the publisher, the editors and the reviewers. Any product that may be evaluated in this article, or claim that may be made by its manufacturer, is not guaranteed or endorsed by the publisher.
The Supplementary Material for this article can be found online at: https://www.frontiersin.org/articles/10.3389/fclim.2024.1368413/full#supplementary-material
GCM, Global Climate Model; RCM, Regional Climate Model; LAM, Limited Area Model; RCP, Representative Concentration Pathway; GHG, Greenhouse Gases; NAd, Northern Adriatic; MAd, Middle Adriatic; SAd, Southern Adriatic; MLIW, Modified Levantine Intermediate Water; SST, Sea Surface Temperature; SSS, Sea Surface Salinity; MLD, Mixed Layer Depth; RMSE, Root Mean Square Error; PDA, Potential Density Anomaly; SLR, Sea Level Rise; TSL, Total Sea Level.
1. ^Maglietta, R., Verri, G., Saccotelli, L., De Lorenzis, A., Cherubini, C., Caccioppoli, R., et al. (2024). Advancing estuarine box modeling: a novel hybrid machine learning and physics-based approach. Environ. Model. Softw. (Under review).
2. ^Santos da Costa, V., Alessandri, J., Verri, G., Mentaschi, L., Guerra, R., and Pinardi, N. (2024). Perspectives on climate change in the Adriatic Sea through sea state indicators. Front. Clim. (Under Review).
Anav, A., Antonelli, M., Calmanti, S., Carillo, A., Catalano, F., Dell Aquila, A., et al. (2024). Dynamical downscaling of CMIP6 scenarios with ENEA-REG: an impact-oriented application for the Med-CORDEX region. Clim. Dyn. 2024, 1–27. doi: 10.1007/s00382-023-07064-3
Artegiani, A., Paschini, E., Russo, A., Bregant, D., Raicich, F., and Pinardi, N. (1997a). The adriatic sea general circulation. Part I: Air sea interactions and water mass structure. J. Phys. Oceanogr. 27:1492–1514. doi: 10.1175/1520-0485(1997)027<1492:TASGCP>2.0.CO;2
Artegiani, A., Paschini, E., Russo, A., Bregant, D., Raicich, F., and Pinardi, N. (1997b). The adriatic sea general circulation. Part II: baroclinic circulation structure. J. Phys. Oceanogr. 27:1515–1532. doi: 10.1175/1520-0485(1997)027<1515:TASGCP>2.0.CO;2
Aumont, O., Ethe, C., Lovato, T., Mouchet, A., Nurser, G., Palmieri, J., et al. (2018). Tracers In Ocean Paradigm (TOP) the NEMO passive tracers engine. Available online at: https://zenodo.org/records/1471700 (accessed January, 2023).
Beuvier, J., Sevault, F., Herrmann, M., Kontoyiannis, H., Ludwig, W., Rixen, M., et al. (2010). Modeling the Mediterranean sea interannual variability during 1961–2000: focus on the Eastern Mediterranean Transient. J. Geophys. Res. 115:JC005950. doi: 10.1029/2009JC005950
Brogli, R., Heim, C., Mensch, J., Sørland, S. L., and Schär, C. (2023). The pseudo-global-warming (PGW) approach: methodology, software package PGW4ERA5 v1.1, validation, and sensitivity analyses. Geosci. Model Dev. 16, 907–926. doi: 10.5194/gmd-16-907-2023
Cessi, P., Pinardi, N., and Lyubartsev, V. (2014). Energetics of semienclosed basins with two-layer flows at the strait. J. Phys. Oceanogr. 44, 967–979. doi: 10.1175/JPO-D-13-0129.1
Chandanpurkar, H. A., Lee, T., Wang, X., Zhang, H., Fournier, S., Fenty, I., et al. (2022). Influence of nonseasonal river discharge on sea surface salinity and height. J. Adv. Model. Earth Syst. 14:e2021MS002715. doi: 10.1029/2021MS002715
Cushman-Roisin, Gacić, M., Poulain, P. M., and Artegiani, A. (2008). Physical oceanography of the Adriatic Sea. Cham: Springer. doi: 10.21236/ADA533698
Dandapat, S., Gnanaseelan, C., and Parekh, A. (2020). Impact of excess and deficit river runoff on bay of bengal upper ocean characteristics using an ocean general circulation model. Deep Sea Res. Part II. 172:104714. doi: 10.1016/j.dsr2.2019.104714
Denamiel, C., Pranić, P., Quentin, F., Mihanović, H., and Vilibić, I. (2020). Pseudo-global warming projections of extreme wave storms in complex coastal regions: the case of the Adriatic Sea. Clim. Dyn. 55, 1–27. doi: 10.1007/s00382-020-05397-x
Denamiel, C., and Vilibić, I. (2023). Next generation atmosphere-ocean climate modelling for storm surge hazard projections. EGUsphere 2023, 1–30. doi: 10.5194/egusphere-2023-913
Drenkard, E. J., Stock, C., Ross, A. C., Dixon, K. W., Adcroft, A., Alexander, M., et al. (2021). Next-generation regional ocean projections for living marine resource management in a changing climate. ICES J. Mar. Sci. 78, 1969–1987. doi: 10.1093/icesjms/fsab100
Dunić, N., Supić, N., Sevault, F., and Vilibić, I. (2022). The northern Adriatic circulation regimes in the future winter climate. Clim. Dyn.. 60, 3471–3484. doi: 10.1007/s00382-022-06516-6
Dunić, N., Vilibić, I., Šepić, J., Mihanović, H., Sevault, F., Somot, S., et al. (2019). Performance of multi-decadal ocean simulations in the Adriatic Sea. Ocean Model. 134, 84–109. doi: 10.1016/j.ocemod.2019.01.006
Fedele, G., Manco, I., Barbato, G., Verri, G., and Mercogliano, P. (2024). Evaluation of atmospheric indicators in the Adriatic coastal areas: a multi-hazards approach for a better awareness of the current and future climate. Front. Clim. 6:1330299. doi: 10.3389/fclim.2024.1330299
Fournier, S., Lee, T., and Gierach, M. M. (2016). Seasonal and interannual variations of sea surface salinity associated with the Mississippi river plume observed by SMOS and Aquarius. Rem. Sens. Environ. 180, 431–439. doi: 10.1016/j.rse.2016.02.050
Galassi, G., and Spada, G. (2014). Sea-level rise in the mediterranean sea by 2050: Roles of terrestrial ice melt, steric effects and glacial isostatic adjustment. Global Planet. Change 123, 55–66. doi: 10.1016/j.gloplacha.2014.10.007
Gochis, D., Barlage, M., Cabell, R., Casali, M., Dugger, A., FitzGerald, K., et al. (2020). The WRF-Hydro Modeling System Technical Description (Version 5.2.0). NCAR Technical Note, 108.
Holt, J., Harle, J., Wakelin, S., Jardine, J., and Hopkins, J. (2022). Why is seasonal density stratification in shelf seas expected to increase under future climate change? Geophys. Res. Lett. 49:e2022GL100448. doi: 10.1029/2022GL100448
Holt, J., Hyder, P., Ashworth, M., Harle, J., Hewitt, H. T., Liu, H., and Wood, R. (2017). Prospects for improving the representation of coastal and shelf seas in global ocean models. Geosc. Model Dev. 10, 499–523. doi: 10.5194/gmd-10-499-2017
L'Hévéder, B., Li, L., Sevault, F., and Somot, S. (2013). Interannual variability of deep convection in the Northwestern Mediterranean simulated with a coupled AORCM. Clim. Dyn. 41, 937–960. doi: 10.1007/s00382-012-1527-5
Li, L., Casado, A., Congedi, L., Dell-Aquila, A., Dubois, C., Elizalde, A., et al. (2012). “Modeling of the mediterranean climate system,” in The Climate of the Mediterranean Region. (Elsevier Inc.), 419–448. doi: 10.1016/B978-0-12-416042-2.00007-0
Macias, D., Stips, A., Garcia-Gorriz, E., and Dosio, A. (2018). Hydrological and biogeochemical response of the Mediterranean Sea to freshwater flow changes for the end of the 21st century. PLoS ONE 13, 907–926. doi: 10.1371/journal.pone.0192174
Madec, G., and Team, N. (2017). Nemo ocean engine. Available online at: https://zenodo.org/records/3248739 (accessed January, 2023).
Mannarini, G., Salinas, M. L., Verri, G., Costa, V. S., Barzaghi, R., and Carrion, D. (2024). Coastal vulnerability to sea level rise for long-term urban planning: the case of Lecce, Italy. ResearchGate. doi: 10.13140/RG.2.2.18379.57123
Mantziafou, A., and Lascaratos, A. (2004). An eddy resolving numerical study of the general circulation and deep-water formation in the adriatic sea. Oceanogr. Res. 51, 921–952. doi: 10.1016/j.dsr.2004.03.006
Mentaschi, L., Lovato, T., Butenschon, M., Alessandri, J., Aragao, L., Verri, G., et al. (2024). Projected oligotrophication of the Adriatic marine ecosystems. Front. Clim. 6:1338374 doi: 10.3389/fclim.2024.1338374
Niu, G.-Y., Yang, Z.-L., Mitchell, K., Chen, F., Ek, M., Barlage, M., et al. (2011). The community noah land surface model with multi parameterization options (Noah-MP): 1. Model description and evaluation with local-scale measurements. J. Geophys. Res. 116:15139. doi: 10.1029/2010JD015139
Oddo, P., Pinardi, N., and Zavatarelli, M. (2005). A numerical study of the interannual variability of the Adriatic Sea (2000-2002). Sci. Total Environ. 353, 39–56. doi: 10.1016/j.scitotenv.2005.09.061
Parras-Berrocal, I. M., Vázquez, R., Cabos, W., Sein, D. V., Álvarez, O., Bruno, M., et al. (2022). Surface and intermediate water changes triggering the future collapse of deep water formation in the north western mediterranean. Geophys. Res. Lett. 49:e2021GL095404. doi: 10.1029/2021GL095404
Piecuch, C. G., Bittermann, K., Kemp, A. C., Ponte, R. M., Little, C. M., Engelhart, S. E., et al. (2018a). River-discharge effects on United States Atlantic and Gulf coast sea-level changes. Proc. Nat. Acad. Sci. 30:115. doi: 10.1073/pnas.1805428115
Piecuch, C. G., Huybers, P., Hay, C. C., Kemp, A. C., Little, C. M., Mitrovica, J. X., et al. (2018b). Origin of spatial variation in US East Coast sea-level trends during 1900–2017. Nature 564, 400–404. doi: 10.1038/s41586-018-0787-6
Pinardi, N., Arneri, E., Crise, A., Ravaioli, M., and Zavatarelli, M. (2006). The physical, sedimentary and ecological structure and variability of shelf areas in the Mediterranean Sea. Sea 14, 1243–1330.
Pinardi, N., Bonaduce, A., Navarra, A., Dobricic, S., and Oddo, P. (2014). The mean sea level equation and its application to the mediterranean sea. J. Clim. 27, 442–447. doi: 10.1175/JCLI-D-13-00139.1
Piña-Valdés, J., Socquet, A., Beauval, C., Doin, M. P., D'Agostino, N., and Shen, Z. K. (2022). 3d gnss velocity field sheds light on the deformation mechanisms in europe: Effects of the vertical crustal motion on the distribution of seismicity. J. Geophys. Res. 127:e2021JB023451. doi: 10.1029/2021JB023451
Poulain, P. M. (2001). Adriatic sea surface circulation as derived from drifter data between 1990 and 1999. J. Marine Syst. 29, 3–32. doi: 10.1016/S0924-7963(01)00007-0
Ruti, P. M., Somot, S., Giorgi, F., Dubois, C., Flaounas, E., Obermann, A., et al. (2016). MED-CORDEX initiative for Mediterranean climate studies. Bull. Am. Meteorol. Soc. 97, 1187–1208. doi: 10.1175/BAMS-D-14-00176.1
Sevault, F., Somot, S., Alias, A., Dubois, C., Lebeaupin-Brossier, C., Nabat, P., et al. (2014). A fully coupled Mediterranean regional climate system model: design and evaluation of the ocean component for the 1980–2012 period. Tellus A. 66:23967. doi: 10.3402/tellusa.v66.23967
Simoncelli, S., Pinardi, N., Oddo, P., Mariano, A. J., Montanari, G., Rinaldi, A., et al. (2011). Coastal rapid environmental assessment in the Northern Adriatic Sea. Dyn. Atmosph. Oceans 52, 250–283. doi: 10.1016/j.dynatmoce.2011.04.004
Skamarock, W. C., Klemp, J. B., Dudhia, J., Gill, D., Barker, D., Duda, M., et al. (2008). A description of the Advanced Research WRF. Version 3, NCAR tech note. TN 475 STR, 125.
Skliris, N., Sofianos, S., and Lascaratos, A. (2007). Hydrological changes in the Mediterranean Sea in relation to changes in the freshwater budget: a numerical modelling study. J. Marine Syst. 65, 400–416. doi: 10.1016/j.jmarsys.2006.01.015
Sprintall, J., and Tomczak, M. (1992). Evidence of the barrier layer in the surface layer of the tropics. J. Geophy. Res. 97, 7305–7316. doi: 10.1029/92JC00407
Tojcić, I., Denamiel, C., and Vilibić, I. (2024). Kilometer-scale trends, variability, and extremes of the Adriatic far-future climate (RCP 8.5, 2070-2100). Front. Mar. Sci. 16, 907–926.
Verri, G., Furnari, L., Gunduz, M., Senatore, A., Costa, V. S, De Lorenzis, A., et al. (2024). Climate projections of the Adriatic Sea: the role of river release. Front. Clim. 6:1368413. doi: 10.3389/fclim.2024.1368413
Verri, G., Mahmoudi Kurdistani, S., Coppini, G., and Valentini, A. (2021). Recent advances of a box model to represent the estuarine dynamics: time”?variable estuary length and eddy diffusivity. J. Adv. Model. Earth Syst. 13:e2020MS002276. doi: 10.1029/2020MS002276
Verri, G., Pinardi, N., Bryan, F., Tseng, Y.-H., Coppini, G., and Clementi, E. (2020). A box model to represent estuarine dynamics in mesoscale resolution ocean models. Ocean Modeling 148:101587. doi: 10.1016/j.ocemod.2020.101587
Verri, G., Pinardi, N., Oddo, P., Ciliberti, S., and Coppini, G. (2018). River runoff influences on the central mediterranean overturning circulation. Clim. Dyn. 50, 1675–1703. doi: 10.1007/s00382-017-3715-9
Vichi, M., Lovato, T., Butenschön, M., Tedesco, L., Lazzari, P., Cossarini, G., et al. (2020). The Biogeochemical Flux Model (BFM): equation description and user manual. BFM version 5.2. BFM Report series N. 1, 89–109.
Vichi, M., Pinardi, N., and Masina, S. (2007). A generalized model of pelagic biogeochemistry for the global ocean ecosystem. part I: theory. J. Mar. Syst. 64, 89–109. doi: 10.1016/j.jmarsys.2006.03.006
Vilibić, I., Mihanović, H., Janeković, I., and Sepić, J. (2016). Modelling the formation of dense water in the northern adriatic: sensitivity studies. Ocean Modell. 101, 17–29. doi: 10.1016/j.ocemod.2016.03.001
Vodopivec, M., Zaimi, K., and Peliz, J. (2022). The freshwater balance of the Adriatic Sea: a sensitivity study. J. Geophy. Res. 127:e2022JC018870. doi: 10.1029/2022JC018870
Keywords: limited area climate modeling, physical downscaling, integrated modeling of local water cycle, Adriatic dilution basin, river release projection, river role on water stratification, river role on sea level rise, local dense water formation
Citation: Verri G, Furnari L, Gunduz M, Senatore A, Santos da Costa V, De Lorenzis A, Fedele G, Manco I, Mentaschi L, Clementi E, Coppini G, Mercogliano P, Mendicino G and Pinardi N (2024) Climate projections of the Adriatic Sea: role of river release. Front. Clim. 6:1368413. doi: 10.3389/fclim.2024.1368413
Received: 10 January 2024; Accepted: 02 April 2024;
Published: 29 April 2024.
Edited by:
Annalisa Cherchi, National Research Council (CNR), ItalyReviewed by:
Ivica Vilibic, Rudjer Boskovic Institute, CroatiaCopyright © 2024 Verri, Furnari, Gunduz, Senatore, Santos da Costa, De Lorenzis, Fedele, Manco, Mentaschi, Clementi, Coppini, Mercogliano, Mendicino and Pinardi. This is an open-access article distributed under the terms of the Creative Commons Attribution License (CC BY). The use, distribution or reproduction in other forums is permitted, provided the original author(s) and the copyright owner(s) are credited and that the original publication in this journal is cited, in accordance with accepted academic practice. No use, distribution or reproduction is permitted which does not comply with these terms.
*Correspondence: Giorgia Verri, Z2lvcmdpYS52ZXJyaUBjbWNjLml0
Disclaimer: All claims expressed in this article are solely those of the authors and do not necessarily represent those of their affiliated organizations, or those of the publisher, the editors and the reviewers. Any product that may be evaluated in this article or claim that may be made by its manufacturer is not guaranteed or endorsed by the publisher.
Research integrity at Frontiers
Learn more about the work of our research integrity team to safeguard the quality of each article we publish.