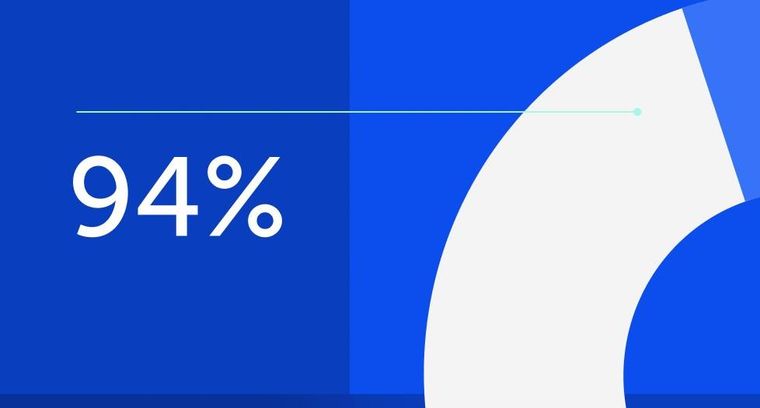
94% of researchers rate our articles as excellent or good
Learn more about the work of our research integrity team to safeguard the quality of each article we publish.
Find out more
ORIGINAL RESEARCH article
Front. Clim., 12 January 2024
Sec. Predictions and Projections
Volume 5 - 2023 | https://doi.org/10.3389/fclim.2023.1330388
This article is part of the Research TopicCoastal Climate Monitoring and Downscaling for Adaptation Planning in the Adriatic SeaView all 12 articles
Increasing sea levels and water temperatures have been detected at several coastal locations worldwide with severe consequences on the communities and ecosystems. Coastal lagoons are particularly vulnerable to such changes due to their low land elevation and limited connections with the open sea. Here the recent and future climatic changes in the Lagoon of Venice (Italy) are investigated using in-situ observations and high-resolution hydrodynamic modeling. Trend analysis was applied to observed time series of meteorological and oceanographic climate essential variables to identify significant long-term changes in mean and extreme values. The mean relative sea level rose at a rate of 4.9 mm per year in Venice due to the combined action of eustacy and subsidence while air and sea temperatures increased on average by 1.8 and 1.1°C in 30 years, respectively. These rates, as well as climate projections, were used following a pseudo-global-warming approach to investigate the near future (up to 2050) evolution of the lagoon's dynamics focusing on sea level and temperature extremes. The lagoon will amplify the temperature changes expected for the Adriatic Sea, especially in the shallow tidal flats where the intensity of the marine heat waves will be more than four times larger than that in the open sea. Moreover, the model allowed us to perform “what-if” scenarios to explore to which extent the flood protection MoSE barriers will modify the lagoon's dynamics. According to the simulations, the number of floods and therefore of the MoSE closure strongly increases with sea level rise. In the most severe scenario, MoSE will have to close for more than 20% of the time in October, November, and December resulting in the reduction of water exchange with the open sea and exacerbation of marine cold spells. Some considerations on the implications of the expected changes on the lagoon's ecology are proposed.
The Mediterranean basin is a region of high cultural and landscape richness that has been heavily impacted by human activity and represents a focal area for climate change studies (MedECC, 2020; Chiggiato et al., 2023; Lionello et al., 2023). In this region, the long-term evolution of the physical processes is only partially explained by global trends (Sannino et al., 2022) and the intensity and trends of multiple climate-related hazards are amplified with respect to the global averages (Vautard et al., 2014; Dosio and Fischer, 2018; Lionello and Scarascia, 2018). The frequency of extreme events, including high precipitation, heat waves, and cold spells, is likely to be altered due to global warming, which might severely threaten the coastal environment and economies (Tuel and Eltahir, 2020; Ali et al., 2022).
This is most certainly true for many coastal environments, like lagoons, which have been identified as hotspots of climate change and vulnerability to environmental and anthropogenic pressures (Ferrarin et al., 2014; Newton et al., 2014). On the other hand, coastal and transitional systems are a key hub for economic productivity in the region, as well as a strategic ground in the endeavor to meet sustainable development objectives and climate change mitigation goals set at the International level. A typical example in this direction is given by the multiple ecosystem services provided by coastal wetlands and seagrass beds, which can combine ecological conservation and carbon sequestration functions with recreational implications and coastal protection instances in a nature-based solution perspective. Gattuso et al. (2018) suggest that sea level rise threatens the existence of a large fraction of the global coastal wetlands, with more than 50% loss by the end of the present century in response to a relatively optimistic 50-cm rise. More generally, it has been pointed out that extreme thermal stressors such as marine heat waves can have notably strong effects on shallow environments, particularly intertidal shores or tidal flats, where organisms and communities typically live close to their tolerance limits (Domínguez et al., 2021). The relatively small scale of the processes that characterize coastal and transitional systems, in particular in the occurrence of extreme events (Schlegel et al., 2017), alongside with the responsiveness to local forcing (Ozer et al., 2022), and the relevance of these environments call for a high-resolution characterization of climate-change impacts over a multi-decadal time scale.
The understanding of the dynamics leading to extreme sea levels and temperatures and of the future evolution of their intensity and frequency is thus of paramount importance for a realistic assessment of present and future risks. Moreover, the description and comprehension of the interconnections between sea level and sea temperature extremes represent a major concern in coastal environments. Their role in the medium-term reshaping of the local habitats features in transitional waters and consequently the extension of available ecological niches is still little studied, although it deserves extreme attention, especially in the presence of the undoubted climate drift underway. This study focuses on the Lagoon of Venice, which according to Ferrarin et al. (2014), is—as well as other coastal environments in the Mediterranean Sea—highly responsive to climate change and can act as a sentinel system for the observation of climate change. Due to its unique historical and cultural value, a flood protection plan (called MoSE, from the Italian acronym for Experimental Electromechanical Module) is operating to protect the City of Venice from flooding. The MoSE is formed by a series of mobile barriers located at the inlets which rise up to emerge and block the flow of the incoming sea level signal in the lagoon during high-tide events.
Even if climate change, sea level rise and coastal flooding in Venice have been deeply investigated by several authors (see the review papers of Lionello et al., 2021; Zanchettin et al., 2021), the changes in the dynamics of the lagoon in the future—when the exchange with the open sea will increasingly be regulated by the MoSE barriers—are still not investigated in detail. Nonetheless, it has already been pointed out that the operation of the MoSE system in a rising sea scenario will bring along a number of implications for the efficiency of marine traffic (Umgiesser and Matticchio, 2006), the morphodynamic processes of shoals, and saltmarshes (Tognin et al., 2022), and more broadly the long-term physical and social-ecological evolution of Venice, its lagoon and the neighboring coastal plain (Tagliapietra and Umgiesser, 2023). The aims of the research reported here are (i) characterizing the recent (1991–2020) climate in the Northern Adriatic Sea and the Lagoon of Venice from observations (Section 3), (ii) investigating future (up to 2050) climatic changes in the Lagoon of Venice and their effects particularly on extreme sea levels and water temperatures (Section 4), (iii) exploring to which extent the MoSE barriers will modify the lagoon's hydrodynamics and thermohaline regime (Section 5). Some considerations on the impact of climate change and MoSE on the ecological dynamics in the lagoon are finally provided (Section 6).
As shown in Figure 1, Venice is located at the center of a coastal shallow lagoon connected to the Northern Adriatic Sea via three inlets (Lido, Malamocco, and Chioggia) which ensure an active exchange of water and substances with the open sea (Umgiesser et al., 2004). The Lagoon of Venice is characterized by a complex network of tidal channels (maximum depth around 15 m), shallow water tidal flats with an average depth on the order of 1 m and salt marshes (Madricardo et al., 2017).
Figure 1. Unstructured model grid and bathymetry of the Lagoon of Venice with the dots marking the monitoring stations (in green the ARPAV meteorological stations). The pink triangle marks the analysis point in the shallow tidal flat (STF). The magenta bars indicate the MoSE barriers at the inlets.
The hydrodynamics in the Venice Lagoon is mainly driven by the tide (about 1 m excursion during spring tides) and the wind. The tide propagates into the lagoon along the deep, narrow channels onto the tidal flats and tidal marshes (Umgiesser et al., 2004). The sea level oscillations in the Northern Adriatic propagate into the lagoon through the three inlets and then follow the main tidal channels. These oscillations, once they have entered the lagoon, propagate nearly without damping to the City of Venice, where the sea levels are comparable to the ones close to the inlets, with a typical 1-h delay (Figure 2). Other more remote areas of the lagoon show a higher phase shift of up to 3 h with respect to the inlets and an attenuation of the sea level (Ferrarin et al., 2015). Due to the high tidal energy and relatively shallow water, the water masses in the lagoon are generally vertically well-mixed (Pivato et al., 2018). Stratification of water masses occurs only in the inner lagoon where the tidal energy is attenuated.
Figure 2. Observed sea level outside (AAOT) and inside (PDS) the lagoon during the first decade of December 2020. The horizontal green line identifies the threshold level for the activation of the MoSE system.
Winter months, particularly November, are more frequently characterized by meteo-marine conditions favorable to the onset of severe storm surges in the Northern Adriatic Sea, mostly driven by southeasterly winds (Scirocco), determining to flood in Venice (Lionello et al., 2021). The frequency of flooding events in Venice increased constantly over the last century (Ferrarin et al., 2022) and the flood risk is expected to rise in future due to the combined action of sea level rise and subsidence (Schlumberger et al., 2022). Indeed, since October 2020, the MoSE (from the Italian acronym for Experimental Electromechanical Module) mobile barriers at the three inlets have started to be in a pre-operational phase closing the lagoon during severe events to protect Venice from flooding. An example of the effect of the MoSE barriers in blocking the incoming tide and limiting the sea level in Venice is presented in Figure 2 for the high tide events of 2, 4, 5, 6, and 9 December of the same year. On December 8th, due to an erroneous interpretation of the forecast, the MoSE was not activated, resulting in flooding in the City of Venice.
Among the different observed parameters, we decided to focus our investigation on a few Essential Climate Variable (ECV, defined as the variables that critically contribute to the characterization of the climate; https://gcos.wmo.int/en/essential-climate-variables) for which long-term timeseries are available in the area of interest. We selected three variables characterizing the surface ocean physics (sea level, sea temperature, and salinity) and three variables describing the surface atmosphere (air temperature, precipitation, and wind speed).
The Northern Adriatic Sea and the Lagoon of Venice are well-monitored by several meteorological and marine stations (Ferrarin et al., 2020; Pérez Gómez et al., 2022). We considered the 1991–2020 period which represents an observational record of 30 years, generally recognized as adequate to assess climatic changes and trends (Mudelsee, 2019). Among the different observational sites, we considered those for which long-term hourly or daily time series were available:
• The Acqua Alta oceanographic tower—AAOT in the shelf sea (15 km Offshore the coast on a 15 m depth) monitoring several meteorological (air temperature, wind speed and direction, precipitation, relative humidity and solar radiation) and oceanographic (sea level, sea temperature, salinity, current velocity) variables.
• Five meteorological stations along the Venetian littoral belonging to the Regional Agency for Environmental Protection and Prevention of the Veneto (ARPAV) measuring air temperature and precipitation (Cavallino Treporti, Mira, Chioggia Sant'Anna, Codevigo, Eraclea).
• The meteo-marine station of Palazzo Cavalli in Venice (CAV) monitoring air and sea temperatures from 2000 to 2020.
• The tide gauge of Punta della Salute in Venice (PDS). All sea level values reported in this manuscript refer to the Punta della Salute official datum (called ZMPS; corresponding to the mean sea level of the 1885–1909 period).
• The marine station of Trieste (TRI), located about 110 km from Venice. Even if this station is quite far from the study site, it has a long record of sea level and sea temperature (Raicich and Colucci, 2019) which can be used as a benchmark for describing the Northern Adriatic Sea conditions. Indeed, Trieste is not affected by significant vertical land motions and therefore it has been widely used as a reference for estimating subsidence in Venice (Zanchettin et al., 2021).
The monitoring stations considered in this study are indicated with dots in Figure 1.
Among the ocean variables monitored by satellite, we considered:
• Sea level anomaly (SLA): 1993–2020 daily mean estimates of sea level anomaly based on satellite altimetry measurements and delivered at 0.125° by the Copernicus Climate Change Service (C3S, 2018). Sea level anomaly is defined as the height of water over the mean sea surface in a given time and region computed with respect to a 20-year mean reference period (1993–2012);
• Sea surface temperature (SST): 1993–2020 daily (nighttime) optimally interpolated 0.05° grid resolution SST maps over the Mediterranean Sea (Pisano et al., 2020) and delivered by the Copernicus Marine Service (https://doi.org/10.48670/moi-00269);
• Sea surface salinity (SSS): 2010–2020 daily dataset at 0.0625° grid resolution covering the entire Mediterranean Sea (Sammartino et al., 2022).
The time series data have been extracted from the gridded datasets in proximity to the Acqua Alta oceanographic station (as the 3 × 3 pixel box average), which is close to the open sea boundary of the numerical simulations.
Coastal climate downscaling is performed here using the pseudo-global-warming (PGW) approach (Brogli et al., 2023) which consists in imposing changes in the climate system on a control climate simulation (usually representing current conditions) by modifying the atmospheric forcing and initial and boundary conditions. This approach has been preferred with respect to the classical dynamical downscaling technique—where outputs from a large-scale climate model are directly used to force limited-area models (Drenkard et al., 2021)—because no detailed regional climate simulations representing correctly the past distribution of the main meteorological and ocean variables are actually available for the Northern Adriatic Sea.
The pseudo-global-warming approach, by applying climate change deltas to the simulation forcings, assumes the preservation of the historical statistical distribution of the specific variable with the advantage of representing correctly both the mean and extreme values (which is often misrepresented in global and regional climate models, Mishra et al., 2023) and the disadvantage that potential changes in the intra-annual, interannual, and future variability might be missed (Brogli et al., 2023). Moreover, PWG has the advantage of performing short numerical simulations, thus limiting the computational and storage costs. The PWG approach has been used by Ferrarin et al. (2014) for simulating climate changes in several Mediterranean coastal environments.
The numerical experiments consisted of simulating the circulation in the Lagoon of Venice using the open-source System of HydrodYnamic Finite Element Modules (SHYFEM, Arpaia et al., 2023) (https://github.com/SHYFEM-model/shyfem). In a 3D approach, the model solves the shallow water equations in their formulations with levels and transports using a finite element numerical method and a semi-implicit time stepping. The model has already been applied in the Mediterranean Sea and several coastal environments (Umgiesser et al., 2022, and references therein). The hydrodynamic numerical computation is performed on a spatial domain that represents the Venice Lagoon and its adjacent shore (Figure 1). The use of elements of variable sizes, typical of finite element methods, is fully exploited to suit the complicated geometry of the basin, the sharp spatial gradients of the topographic features, and the complex bathymetry. The water column is discretised into 17 vertical levels with progressively increasing thickness, varying from 1 m for the topmost 10 to 7 m for the deepest layer of the outer shelf. The model bathymetry was obtained from the data collected in 2002 by Magistrato alle Acque di Venezia—merged with later surveys—and the high-resolution bathymetry acquired in the main channels of the lagoon in 2014 (Madricardo et al., 2017).
Climate anomalies are computed as differences with respect to a control situation. The year 2020 is considered for the control simulation using the observations (hourly sea level, current velocity, temperature, salinity, wind speed, wind direction, air temperature, mean sea level pressure, solar radiation, relative humidity, and cloud cover) acquired at the Acqua Alta oceanographic tower as boundary and forcing conditions. This period has been chosen because the year 2020 is at the end of the 30-year climate period considered for assessing the recent climate evolution, and also because in October 2020 the MoSE barrier system started operating for the protection of the historical city of Venice from flooding (https://www.MoSEvenezia.eu/MoSE/). The numerical simulation of the MoSE closure was modeled by increasing bottom shear stress and viscosity in the inlet areas.
The future reference time horizon is 2050, thus considering a 30-year projection of the actual situation. The climate change deltas used to perturb the control situation were both extrapolated from the observed past trends over the 1991–2020 period (where statistically significant) and derived from climate projections according to three Representative Concentration Pathway scenarios (RCPs 2.6, 4.5, and 8.5). Future climate change deltas for the three RCPs scenarios were computed as the multi-model ensemble mean of EURO-CORDEX (https://www.euro-cordex.net/) and Med-CORDEX (https://www.medcordex.eu/) model experiments for the meteorological and oceanographic climate variables, respectively. The EURO-CORDEX (taken here as the mean of an ensemble of the HadGEM2-ES_RACMO22E, MPI-ESM-LR_REMO2009, EC-EARTH_CCLM4-8-17, EC-EARTH_RACMO22E, and EC-EARTH_RCA4 models) and Med-CORDEX (CNMR-CM5_CNRM-RCSM4) model experiments were selected according to the availability of data and scenarios for the study area and following the study of expected climate change in the nearby Friuli Venezia Giulia region, where Trieste is located (ARPA-FVG, 2018). Future projections of the mean sea level for the three RCPs scenarios were taken from the IPCC 6th Assessment Report (Fox-Kemper et al., 2021) and made available via the NASA Sea Level Projection Tool (https://sealevel.nasa.gov/ipcc-ar6-sea-level-projection-tool). The climate perturbations were applied to air temperature, wind speed, sea level, salinity, and sea temperature.
To summarize, for analyzing the climate change effects on lagoon hydrodynamics, we performed five pairs of 13-month numerical experiments with SHYFEM applied over the Lagoon of Venice with different initial and forcing conditions as summarized below:
• CTRL-2020: control simulation for 2020 using observations as forcings;
• TREND-2050: climate change simulation extrapolating the past observed 30-year perturbations to 2050;
• RCP2.6-2050: climate change simulation using RCP2.6 scenario perturbations for 2050;
• RCP4.5-2050: climate change simulation using RCP4.5 scenario perturbations for 2050;
• RCP8.5-2050: climate change simulation using RCP8.5 scenario perturbations for 2050.
All above-mentioned simulations were performed also in “what-if” scenarios considering the closure of the MoSE barriers. The SHYFEM numerical model allows the fluxes at the inlets to be reduced for simulating the closing and subsequent opening of the mobile barriers (Ferrarin et al., 2013; Umgiesser, 2020). According to the tide gauge observations registered since October 2020, the MoSE is raised when a sea level is forecasted to surpass the 1.1 m flooding threshold. However, to save Venice from flooding, the MoSE barriers have to be closed in advance, generally when the sea level in Venice is between 60 and 80 cm (depending on the meteorological situation) and opened in the subsequent descending tide (Figure 2). In the case of consecutive flooding events, the MoSE is kept closed continuously for several hours or even days. The closing of the barriers takes 30 min, and in all three inlets the barriers are generally closed at the same moment. These managing procedures were considered in projecting the future operation of MoSE in the 2050 climate change scenarios. A similar MoSE operation procedure was adopted in the modeling study by Umgiesser (2020).
The initial condition in the numerical simulations is always the calm state. This is certainly no problem for the current velocity and the water level since these quantities approach a dynamic state very fast (less than a day) in such a dynamic tidal and wind-driven environment. The time to reach a steady state distribution is larger for the water temperature and salinity. Therefore, salinity and water temperature values measured in the lagoon in December 2019 (and modified by the climate change deltas in the climatic simulations) have been chosen as initial conditions and we considered a spin-up time of 1 month (December 2019). Such a period is larger than the average residence time of the lagoon (Ferrarin et al., 2013) and therefore is enough for the variables to approach a dynamic steady state.
The application of the SHYFEM model to the Lagoon of Venice has been validated in previous work reproducing correctly tidal propagation, storm surge, water flows at the lagoons' inlets, and water temperature, and salinity variability (Ferrarin et al., 2021, and references therein). The control experiment (CTRL-2020) has been validated by comparing the model results with sea level (SL) and sea temperature (ST) observations acquired at PDS. The model results compare reasonably well with the measurements: the root mean square error is 7 cm for SL and 1.0°C for ST; the correlation coefficient is about 0.98 for both variables; the difference between the averages of simulated and observed values (BIAS) is −2 cm for SL and 0.7°C for ST.
Monthly averaged time series data were processed with the Python pyMannKendall analysis tool (https://pypi.org/project/pymannkendall/, Hussain and Mahmud, 2019) for detecting consistently increasing or decreasing linear trends (monotonic trends). The Mann-Kendall Trend (MKT) test is a non-parametric test, which means it works for all distributions (i.e., data doesn't have to meet the assumption of normality), but data should have no serial correlation (Mann, 1945). In this study, the seasonal MKT was used to remove the effect of seasonality in the data. The trend is here considered statistically significant when the p-value of the MKT is less than 0.05.
Sea level extremes are commonly defined in Venice as the high tide event (locally called acqua alta) exceeding the flooding threshold of 1.1 m above ZMPS (Lionello et al., 2021). Such a flooding threshold is also used in the sea level forecasts for the activation of the MoSE system.
Marine heat waves (MHW) occur when the sea temperatures are abnormally warm for the time of the year relative to historical temperatures, with that extreme warmth persisting for a prolonged period (five consecutive days according to Hobday et al., 2016). A single day of sea temperature above the threshold value is defined as a marine heat spike (MHS). The day-of-the-year threshold is computed as being the daily 90th percentile of the sea temperature distribution over a long (ideally 30-year) historical baseline period (Hobday et al., 2016). In the MHW analysis, we used the marineHeatWaves python module (https://github.com/ecjoliver/marineHeatWaves) which implements the MHW definition of Hobday et al. (2016). The daily climatological mean and threshold time series are smoothed using a 30-day moving window.
Contrary to MHW, Marine Cold Spells (MCS; Schlegel et al., 2021), happen when the sea temperatures are unusually low for the time of year compared to climatological values for a prolonged time (5 days). As a threshold for the identification of MCSs, we used the 10th percentile of the daily climatological sea temperature distribution.
In this study, we analyzed in-situ and satellite observations acquired in the period 1991–2020 to assess recent climatic changes in the Northern Adriatic Sea and the Lagoon of Venice. The characterization of the recent climate and its changes in time are presented and discussed separately for meteorological and ocean variables, with special attention on the second group. Linear climatic trends for all considered variables are reported in Table 1.
Table 1. Linear climatic trends from observations for the 1991–2020 period (if not differently specified) at the 95% significant level.
The analysis of the air temperature datasets revealed an increasing trend of 0.58°C/10y over the Veneto Coast with values up to 0.9°C/10y in the City of Venice (calculated over the 2003–2020 period). This means that in the study area, the air temperature rose by at least 1.8°C in the last three decades, therefore already overpassing the global limit of 1.5°C above pre-industrial levels defined by the Paris Agreement (https://unfccc.int/process-and-meetings/the-paris-agreement). Temperature-derived indicators reveal an increasing trend in heat waves of 3 days per decade (not statistically significant), tropical nights (days when the temperature does not fall under 20°C during the night time) of about 4 days per decade (statistically significant) and frost-free days (days with a minimum daily temperature above 0°C) of 8 days per decade (statistically significant) (Figure 3). These results confirm previous climatic studies highlighting the Northern Adriatic Sea, and generally the Mediterranean, as a hotspot for climate change (MedECC, 2020).
Figure 3. Mean decadal values of heat waves, tropical nights, and frost-free days in the Veneto coast.
The long-term wind speed timeseries recorded at AAOT show a slight decreasing trend of 0.01 m s−1, in accordance with the detected decrease of storm waves in the Adriatic Sea (Pomaro et al., 2018). Decreasing trends in wind intensity and wave height over the Adriatic Sea have also been found in several climate studies (e.g., Bonaldo et al., 2017; Benetazzo et al., 2022).
The precipitation dataset used in this study does not reveal any significant linear trend (Table 1), in partial contrast with the findings by Philandras et al. (2011), which identified the Adriatic region as one of the areas in which the long-term trends in precipitation decrease have been the most intense in the last century. Nevertheless, it is worth noting that the region enclosed between the Northern Adriatic Sea and the Alpine Ridge exhibits strong spatial gradients in terms of precipitation rates, recent past trends (Christidis and Stott, 2022), and future projections (Zittis et al., 2021), suggesting that global and regional-scale modeling and coarse-resolution gridded observational products could fail in matching the measured precipitation trend in the Veneto coastal region. End-of-century RCP8.5 regional projections by Rajczak et al. (2013) show for the Adriatic Sea and its hydrographic basin decreasing precipitation frequency throughout the whole year (except in the north in winter), wetter autumn/winters (in the north) and dryer springs/summers, increasing intensity particularly in autumn and winter.
According to Zanchettin et al. (2021), the relative mean sea level (RMSL) in Venice has risen by more than 30 cm since 1870 with an average linear trend of 2.5 mm y−1. A higher rate of sea-level rise has been observed in more recent years (1991–2020) with values reaching 4.9 mm y−1 (Table 1 and black line in Figure 4). A similar trend value has been found for the AAOT monitoring station (4.8 mm y−1). However, it is well-known these two locations are strongly influenced by subsidence, whose rate is estimated by the comparison with the sea level trend registered in Trieste (3.4 mm y−1) which is considered unaffected by vertical land movements (Zanchettin et al., 2021, and references therein). The comparative assessment of the two tide gauges (PDS and TRI) estimates the mean subsidence in Venice of 1.5 mm y−1 over the last three decades. The analysis of the satellite data recorded in the shelf sea reported an increasingly significant trend of 3.0 mm y−1, a value similar to what was observed in Trieste and within the range estimated by Mohamed et al. (2019) (3.0 ± 0.5 mm y−1 over the 1993–2017 period) and Meli et al. (2023) (2.6 ± 0.8 mm y−1 over the 1993–2019 period) for the entire Adriatic Sea.
Figure 4. Number of events per year exceeding the 1.1 m flooding threshold. The blue bar indicates the number of MoSE closures in 2020. The time evolution of the relative mean sea level is shown as a black dashed line.
As reported by Lionello et al. (2021) and Baldan et al. (2023), the frequency of floods in Venice has increased over the decades (Figure 4). Ferrarin et al. (2022) found that this is mostly due to the rise of the RMSL and partially to changes in the long-term forcings associated with planetary atmospheric waves and seasonal to inter-annual oscillations. It has to be noted that in the Lagoon of Venice, Caruso et al. (2010) found a statistically significant difference between yearly mean and maximum sea level trends and suggested a possible explanation for the different contributions of the lagoon morphology to energy dissipation.
The analysis of the sea temperature revealed an increasing long-term trend in all monitoring stations with values ranging from 0.44 to 0.48°C per decade (Table 1). The rise of the mean sea temperature determined a general increase (not statistically significant) in the number of marine heatwaves and a decrease in marine cold spells (Figure 5). The catalogs of MHW and MCS events in Venice are proposed in Supplementary Tables 1, 2, respectively. We detected a total of 49 MHW events; the strongest occurred in February/March 2007 and lasted for 35 days with a cumulative intensity (computed as the integral of the intensity over the duration of the event) of 36°C·day (Figure 5). The year 2007 was characterized by anomalously high temperatures during the first half of the year (with six marine heatwaves) and unusually low sea temperatures from September to December (with four marine cold spells). The year 2020, considered here as a control period, shows a sea temperature variability in line with the long-term climatology with only a short MHW event in December and no MCSs.
Figure 5. Daily sea temperature time series recorded in the Venice Lagoon at PDS (black line). The dashed red, the dot-dashed green and the dashed blue lines indicate the daily 90th percentile threshold defining MHW, the mean daily climatology and the daily 10th percentile threshold defining MCS, respectively. Light red and light blue shadings display the detected MHW and MCS events, respectively.
Within the Mediterranean basin, the Northern Adriatic Sea has been identified as a hotspot for marine heatwave intensity (Dayan et al., 2023), particularly in spring and summer, with the number of total yearly MHW days increased from a few units to around one hundred per year (Juza et al., 2022; Martínez et al., 2023). Schlegel et al. (2017) have shown that the occurrence of marine heatwaves and cold spells in coastal environments can only partially be explained by mesoscale ocean conditions while being strongly controlled by local and sub-mesoscale processes.
Salinity recorded at AAOT in the period 2003–2020 does not show any significant trend. On the contrary, the sea surface salinities recorded by satellite manifested an increasing trend of 0.08 pss from 2010 to 2020, highlighting the Northern Adriatic Sea salinification in the last decade (Sammartino et al., 2022). Camatti et al. (2023) detected an increasing trend in salinity in the inner part of the Venice Lagoon and not near the inlet. This is explained by the fact that the relative sea level rise (RSLR) produces an increase in the lagoon volume that, even considering no significant reduction in the freshwater runoff, induces the long-term salinization of the lagoon.
Four scenario simulations were performed to assess the future changes in the lagoon characteristics. The climate change deltas used in the numerical experiments are reported in Table 2. The table reports also the deltas uncertainties as derived from the analysis of the observed climatic trends and from the standard deviation of the multi-model ensembles. The results of the climate experiments were used to investigate anomalies with respect to the 2020 control simulation.
Table 2. Climate change deltas used in the different climate simulations to perturb the 2020 boundary and forcing conditions.
Even if we are aware that changes in the climate do not follow a linear evolution, the reported values allow the comparison of the observed past climate changes with future projections. In this context, the observed-based scenario—which replicates the actual trend of the site-specific climatic conditions—provides a benchmark for the global and regional model projections. From a first assessment, we can observe that the rate of change of the relative sea level in the recent past (last 30 years) is slightly lower than what is expected for the future (next 30 years) by all climate scenarios. On the other side, a much more intense climate warming was recently experienced in the study site (1.8 and 1.4°C for the air and sea temperatures, respectively) than foreseen by the climate models in the different RCPs (1.1 and 1.0°C for the air and sea temperatures, respectively, in the most extreme scenario).
The number of extreme sea levels and consequent flooding of Venice will increase with sea level rise (Table 3). With a rise of the mean sea level of 0.22 m—as predicted in the RCP8.5 scenario for 2050—the number of acqua alta events will triplicate (54 in total) with 9 exceptional floods (considered when the peak sea level reaches 1.4 m and causing the flooding of about 60% of the city of Venice). The flooding events will remain concentrated in the winter period even if some events will occur in spring and summer. It has to be noted that our simulations do not consider changes in the storm surge component of the sea level boundary conditions and therefore the evolution of the extreme sea levels within the lagoon is mainly due to the rise of the RMSL. Climate studies generally agree in predicting a slight weakening of severe weather conditions in the Adriatic Sea (Lionello et al., 2017; Bonaldo et al., 2020; Benetazzo et al., 2022) with the consequent attenuation of the storm surge (Makris et al., 2023). However, our simulations consider changes in the tide and storm propagation within the lagoon due to future sea level rise—as a consequence of modifications in the dissipative processes—which could impact the extreme sea levels more than the expected variation in storminess (Ferrarin et al., 2015; Lionello et al., 2021).
Table 3. Number of acqua alta events and hours (also in % of the year) of MoSE closure in the different numerical experiments.
The maximum sea levels simulated in the whole domain were extracted for all considered scenarios. As shown in Figure 6, a clear sea-to-land positive gradient is evident in the lagoon which reflects the influence of the two main wind regimes: Bora from the North-East and Sirocco from the South-East. In 2020 (Figure 6A), the sea level in the shelf sea reached a peak value of almost 1.4 m and increased to 1.6 m in the Northern lagoon (under the action of the Sirocco wind) and to 1.8 m in the southern part (pushed by the Bora wind). In the TREND-2050 (Figure 6B) and RCP8.5 (Figure 6C) scenarios (and similarly also in the other 2050 climate simulations), the maximum sea level pattern will remain similar to the 2020 situation but shifted by the climate delta imposed at the boundary condition.
Figure 6. Maximum sea level distribution as simulated in the CTRL-2020 (A), TREND-2050 (B), and TREND-2050 with MoSE (C) experiments.
Since the numerical simulations consider a spatial domain comprising the Lagoon of Venice and part of the shelf sea, MHWs and MCSs were computed considering the climatologies (90th and 10th percentiles for MHW and MCS, respectively) of the sea temperatures registered at the Acqua Alta oceanographic tower. In this way, changes can be discussed with respect to the anomalies imposed at the sea boundary, thus highlighting how the shallow environment will respond to the surface forcing and boundary conditions. Modeling results are presented in Figures 7, 8 in terms of the cumulative MHW (MCS) intensity over the whole year and corresponding to the sum of the intensity of all detected MHW (MCS) events.
Figure 7. Cumulative MHW intensity as simulated in the CTRL-2020 (A), TREND-2050 (B), and RCP8.5-2050 (C) experiments.
Figure 8. Cumulative MCS intensity as simulated in the CTRL-2020 (A), TREND-2050 (B), and RCP8.5-2050 (C) experiments.
The lagoon's water temperature, especially in the inner shallow tidal flats, has higher daily and seasonal variability than in the open sea. The lagoon is usually warmer in summer and colder in winter than the open sea because the shallow water environment quickly responds to the heat fluxes through the air-sea interface (Pivato et al., 2018). Such a spatial variability results in a marked land-to-sea gradient of MHW (Figure 7) and MCS (Figure 8). The sea temperature in the year 2020 (control simulation) was in line with the climatological values and no significant MHW was detected (Figure 7A). Due to the active tidal exchange, a similar situation is found in the inlets and main channels. The yearly cumulative MHW intensity increases toward the lagoon margins reaching peak values of about 100°C·day. As shown in Figures 7A, B, climate change will exacerbate the occurrence and intensity of marine heat waves with values higher than 250°C·day in the inner lagoon. The numerical results indicate that climate change will increase the sea temperature in the lagoon more than what is expected in the open sea demonstrating that the mesoscale sea temperature forcing is not the main driver of extreme coastal events (Schlegel et al., 2017). As a consequence, in 2050 and under the TREND-2050 scenario, the yearly cumulative MHW intensity in the lagoon is expected to increase by more than 10 times with respect to the present situation (Figure 9).
Figure 9. Cumulative MHW and MCS intensity as computed in three locations: in the open sea (AAOT), in Venice (PDS) and in the inner lagoon over shallow tidal flats (STF).
The lagoon experienced much colder water with respect to the sea, resulting in a high intensity of marine cold spells (Figure 8A), confirming,—as for MHW—that the surface heat fluxes are the main driver of extreme coastal events (Juza et al., 2022). As also shown in Figure 9, the open sea-nearshore-lagoon MCS gradient is even stronger than the one detected for MHW. The general rise in water temperature induced by climate change will strongly reduce the occurrence and intensity of MCS events (Figures 8B, C). Such a change is stronger in the inner part of the lagoons (170°C·day at STF from 2020 to 2050; Figure 9) than in the deep channels that are more influenced by the water exchanges through the inlets (128°C·day at PDS from 2020 to 2050; Figure 9).
In this section, we investigate and discuss the effect of the MoSE flood barrier closures on the lagoon hydrodynamics and thermal variability. As shown in Figure 10A, the MoSE is effective in protecting the City of Venice from severe flooding during storm surge events and limits the maximum sea water level to values lower than 1.2 m even in climate change scenarios. However, the southern part of the lagoon depicts peak water level values close to 1.8 m, indicating that the along-lagoon sea level gradient—due to the wind setup—is magnified when the lagoon is isolated from the sea (Mel et al., 2023).
Figure 10. (A) Maximum sea levels in the RCP8.5-2050 simulation considering the operation of the MoSE barriers and (B) mean monthly water discharge through the inlets in the CTRL-2020 and RCP8.5-2050 scenarios with and without the MoSE.
The tidal action ensures an active water exchange through the inlets that may reach values up to 20,000 m3 s−1 during spring tides (Umgiesser, 2020). The monthly water discharge through the inlets computed from the CTRL-2020 results fluctuates around 10,000 m3 s−1 with higher values in the last part of the year due to the combined action of tide and stormy winds (red continuous line in Figure 10B). Such a water exchange guarantees a constant renewal of the lagoon waters with an average flushing time (computed as the lagoon volume by the water flux at the inlets) estimated in 1.5 days (Umgiesser et al., 2014). In 2020, the MoSE effect was detected only during October and December, when most of the acqua alta events occurred, with a maximum decrease of the water fluxes of 18% (light-red dashed line in Figure 10B).
Sea level rise will determine an increase in the lagoon volume and inlets cross sections with the resultant enhancement of the lagoon-sea water exchange by almost 10% in the RCP8.5-2050 scenario (blue continuous line in Figure 10B). However, future RSLR will increase the number of hours the MoSE will need to be closed by up to four times (Table 3). Consequently, the lagoon will be more isolated resulting in a decrease in the mean water flux through the inlets reaching 40% in December (light-blue dotted line in Figure 10B). Contrary to the 2020 situation, under the RCP8.5-2050 scenario, the MoSE will affect the lagoon hydrodynamics during the spring and summer months too (as occurred in August 2023). The reduction of the sea-lagoon fluxes determines a decrease in the lagoon renewal capacity (Ferrarin et al., 2013).
The results of the “what-if” numerical experiments indicate that the MoSE will significantly impact the lagoon water temperature from September to December by decreasing the mean daily values during the closure. This occurs because the barriers interrupt the input of sea waters which are generally warmer than the lagoon waters in winter. As a consequence, the MoSE closure determined a slightly higher water temperature variability in the lagoon. The performed simulations show that the MoSE does not affect the occurrence and intensity of marine heat waves in the lagoon (Figure 11A). On the other side, the closure of the MoSE barriers will exacerbate the intensity and duration of marine cold spells, especially in the shallow water areas between the inlets (Figure 11B).
Figure 11. MHW (A) and MCS (B) cumulative intensity differences between the experiments with and without the MoSE in the RCP8.5-2050 scenario.
In summary, in future, the closure of the MoSE barrier will be operating more often with a substantial decrease in the sea-lagoon fluxes that are essential for maintaining an active renovation of the lagoon waters. Our simulation results indicate that the lagoon will experience more stagnant and colder waters during the winter months when the storm events concentrate. It is however expected that with higher rates of RLSR the MoSE barriers will have likewise to close in summer determining a further increase in the lagoon water temperature and MHW events.
Many interrelated and overlapping aspects make it difficult to predict the ecosystem's response to the combined effect of climate change and human interventions. In discussing the possible consequences, we must keep in mind on the one hand the time scale and the synchronization of physical and biological events, and on the other hand, we must consider the richness and complexity of the composition of biological communities.
The two most plain climate change-induced processes the lagoon is facing—as well as many other coastal locations worldwide—are the rise of the mean sea level and water temperature. The first, in addition to determining an increase in flood frequency, induces the long-term salinization of the lagoon. Indeed, Camatti et al. (2023) detected a significant increase of salinity in the 25-year in the inner part of the lagoons, while no clear drift is observed near the inlets. Ferrarin et al. (2013) also underlined that the sea level rise increases both the salinity and the renewal time in the lagoon. This implies a lower connection of the lagoon with the sea and potentially an increase in saprobicity (Tagliapietra et al., 2012a) strictly related to habitat typology, community composition and distribution (Guelorget and Perthuisot, 1989).
The MHW represents a thermal stress for the actual environment, but at the same time, is the process which forces the temperature drift toward the future temperature regime. The MHWs seem to occur mainly in the first 6 months of the year while verified MHWs are also distributed over long periods in summer (Garrabou et al., 2022). The vulnerability of the marine ecosystem depends not only on the magnitude and duration of MHW but also on the season and frequency of occurrence, in addition to the sensitivity and the short-term adaptive capacity of the ecosystem to these events (Frölicher and Laufkötter, 2018).
Even events on a short time scale can lead to an irreversible collapse of some components of the ecosystem (the most sensitive, the least tolerant of those that have no escape routes). In turn, this can lead to the collapse of food webs, even if they are well-developed. In this respect, MHWs represent a thermal stress capable of exceeding the tolerance level of organisms in a short time. The increase in frequency and intensity of MHW has physiological and morphological effects in several organisms in addition to bringing a change in the distribution of their optimal habitat. Mobile species can move toward thermal refugia, modifying their spatial distribution and the balance of the food web. If the ecosystem is structurally robust, i.e., if it presents suitable functional richness at the different levels, recovery is rapid and usually favored, in these dynamic ecosystems, by the co-presence of very well-connected patchy and scattered habitats which act as sources for the recolonization of sites.
As reported here and also in Ferrarin et al. (2013), sea level rise and temperature drift reduce the resilience of the system, favoring a compression of spatial gradients. This causes a reduction in both the surface area and the diversity of the more brackish habitats, which are therefore more confined along the lagoon margins and more fragmented and less connected, leading to a lower recovery capacity of the entire ecosystem after a crisis. This is confirmed by our results: the most affected areas to MHW are the inner part of the lagoon and shallow water areas, the wetland and salt marshes areas. This means that the most exposed species are the ones living in this part of the lagoon and having no possibility to escape or to find refuge, as terrestrial and marine flora and sessile organisms.
To evaluate the potential impact we can learn from the past by examining the consequences on the ecosystems of the exceptional summer MHW in the northwestern Mediterranean Sea that occurred in the year 2003. This event began in early June 2003 and lasted until mid-August. The increase in water temperature was estimated at 3–5°C above the 1982–2016 reference period (Frölicher and Laufkötter, 2018; Garrabou et al., 2022). Garrabou et al. (2009) reported extensive mass mortality in late summer 2003 in rocky benthic macroinvertebrate species along several thousand kilometers of coastline in the Northwestern Mediterranean. Munari (2011) investigated considerable changes in the benthic community structure and relative composition, persisting until the year 2005, in Comacchio Lagoon (Northern Adriatic Sea, Italy). In a close area, Ponti et al. (2017) report the decline of the available stock of Manila clam in a coastal lagoon due to overfishing and heat exposure. From this short and non-exhaustive list, we can assume that the main biological impacts verified in previous MHW in the world are the geographical species shift, the widespread changes in species composition and the mass mortalities of particular species. The literature has studied MHW-related impacts when striking events (die-offs, anoxias) are developed. Little is known about how MHWs can instead restructure the community simply by altering the timing and fitness of species.
The imbalance of the climatological waveform of the average temperatures, particularly in the first half of the year, reasonably induces a modulation of the community assembly processes consolidated over time. The MHW influences the relative weight of structuring processes such as “habitat filtering” (i.e., the effect of local physical-chemical variables on populations), “dispersal assembly” (i.e., the possibility of stochastic arrival of new species in a given site) and “limiting similarity” (i.e., the limitation on the coexistence between species due, e.g., to competition). Cutting-edge coexistence theory believes that both stochastic and niche-based processes act simultaneously to shape communities (Weiher et al., 2011) and modeling the relative importance of different assembly processes is a promising approach (van der Plas et al., 2015). However, understanding how these structuring forces reach a new equilibrium in each lagoon site currently appears to be a very complex question, even taking into account that there are certainly trade-off mechanisms between processes and underlying causes that are difficult to identify a priori and quantify experimentally.
The loss in timing synchronization of the functional groups can bring a tipping point in the architecture of the ecosystem, as well as heat waves and cold spells can disrupt the larval settlement and recruitment compromising the juvenile and adult population recovery (Ghezzo et al., 2018; Smith et al., 2023). Non-indigenous species will certainly play a very important role, especially the more tolerant ones and particularly if they can synchronize the times of their life cycle better than the resident species with the temperature waveform drift (in terms of advances and durations above the threshold).
Marine cold spells play a similar role as MHW but toward the opposite edge of the tolerance range. Cold spells can have short-term impacts ranging from mass mortality, population decrease, and changes in species distribution and phenology. As in MHW, the combination of different factors (due to the characteristics of the MSC and the ecosystem tolerance) defines the severity of the ecological impacts (Schlegel et al., 2017). The mortality due to MHW and MSC is higher in the shallow environment because, as in our results, the water column has lower thermal inertia and offers no deep water as a refuge. The occurrence of MSC can slow the spreading of non-native or invasive species (Schlegel et al., 2017), even if proceeding with the expected global warming would probably reduce this effect.
In the Venice Lagoon, the tidal exchange and the river input are the main factors driving the gradient of nutrients, salinity, suspended sediment, as well as dissolved oxygen and pollutants. The reduction of the sea exchange due to the MoSE protection and the reduction of the water renewal capability of the lagoon has an impact on the distribution of nutrients, oxygen and organic matter in the lagoon, and on the local eutrophication and saprobicity. This means that the variation in water renewal transforms the biological communities and biodiversity. The MoSE effect is to reduce the lagoon-sea exchanges during the winter season but also during the summer following the stronger climate change scenario. Reduced flushing combined with increasing temperature, overall during the summer season, increases the water stratification with the risk of anoxia or hypoxia mainly in deep channels overall if it occurs within calm weather (Tagliapietra et al., 2012b). The anticipation in biological activity due to the increase in temperature enlarges the risk of anoxia also to the winter period. The closure of the lagoon has an effect also on pollutant persistence and larval connectivity between the lagoon and the sea. This aspect is particularly relevant considering the role of the lagoon as a spawning area, nursery for marine species, and feeding ground for migrant species from rivers (anadromous and catadromous). The increasing closure time exacerbates the separation of the lagoon to the sea not only in winter but also in summer increasing the risk to interfere with the biological life cycle and consequently with the conservation of the populations.
In this study, the recent past (1991–2020) and near future (2021–2050) dynamics of the Lagoon of Venice were investigated through the use of long-term observations and numerical modeling. Furthermore, the model allows us to simulate how the system would react to “what if” scenarios of a lagoon regulated by the flood protection MoSE barriers. Our analysis concentrates on the evolution of sea level and temperature extremes. The major conclusions of this work are summarized in the following points.
• The relative mean sea level rose at a rate of 4.9 mm per year determining a significant increase in the frequency and duration of flooding events in Venice.
• The Northern Adriatic region underwent a warming of about 1.8°C in 30 years which determined an increase of more than 1°C of the mean sea surface temperature with a rise of intensity and frequency of marine heat waves. Such air and sea temperature anomalies are much higher than what is predicted by climate models for the near future.
• Due to its shallow morphology, the lagoon will amplify the temperature changes predicted for the Northern Adriatic Sea, especially in the shallow tidal flats where the cumulative intensity of the marine heat waves will be more than four times that in the open sea.
• The continuous rise of the sea level will lead to a lagoon more and more regulated by the MoSE barriers. Saving Venice from frequent floods will determine a reduction of the water exchange through the inlets by more than 40 % in the winter months. MoSE may determine a further increase in MHW events in summer and MCS events in winter.
• The above-mentioned compound effects, where climatic changes combine with the anthropogenic management of the environment, can lead to significant ecological implications such as changes in the phenology of the organisms, and in the distribution of species and habitats. Modification in the ecosystem equilibrium can move the ecosystem trajectories toward a collapse or toward a new structure where non-indigenous species could be more adapted.
The future of Venice from a climate change perspective is moving toward a closure and warm lagoon. Tagliapietra and Umgiesser (2023) depict a rational framework, considering that the actual trend will be the normal state in a not so far future and that the lagoon will be transformed into a coastal pond when the sea level rises. In the Lagoon of Venice, intertidal ecosystems will most likely not be able to counteract the rapid expected relative sea level rise and therefore such valuable habitats are going to be gradually lost. Theoretically, at the moment to help the survival of these morphologies which are the result of a delicate long-term dynamic balance between sedimentation and auto-compaction (Zoccarato et al., 2022), it would be necessary to keep the average water level low enough to allow net accretion rates in the order of RSLR. On the other hand, sedimentation is ensured only under suitable sediment supply to these systems which in the absence of significant riverine inputs mainly occurs during major sea storms (Tognin et al., 2022). The question could therefore be: Is it possible to rethink the MoSE now or in the near future not only as a defense against high waters (i.e., to cut off the extremes that are too harmful for the city) but as a dynamic regulator of the average internal level to counteract its increase? Could MoSE also be used to regulate the heat balance to reduce the effects of MHW and MCS events?
The raw data supporting the conclusions of this article will be made available by the authors, without undue reservation.
CF: Conceptualization, Data curation, Formal analysis, Funding acquisition, Methodology, Software, Visualization, Writing—original draft, Writing—review & editing. DB: Conceptualization, Writing—original draft, Writing—review & editing. AB: Writing—review & editing. MG: Data curation, Software, Writing—original draft, Writing—review & editing.
The author(s) declare financial support was received for the research, authorship, and/or publication of this article. This work was partially supported by the Interreg project AdriaClim (Climate change information, monitoring and management tools for adaptation strategies in Adriatic coastal areas; Project ID 10252001) funded by the European Union under the V A Interreg Italy-Croatia CBC programme.
The authors wish to thank the Italian Institute for Environmental Protection and Research (ISPRA), the Tide Forecast and Early Warning Center of the city of Venice (CPSM), the Regional Agencies for Environmental Protection and Prevention of the Veneto (ARPAV), and Friuli Venezia-Giulia (ARPA FVG) for providing meteorological and oceanographic data. We thank Dr. Michela Sammartino for providing SSS data over the Northern Adriatic Sea. We thank Dr. Georg Umgiesser for critically reviewing the manuscript.
The authors declare that the research was conducted in the absence of any commercial or financial relationships that could be construed as a potential conflict of interest.
The author(s) declared that they were an editorial board member of Frontiers, at the time of submission. This had no impact on the peer review process and the final decision.
All claims expressed in this article are solely those of the authors and do not necessarily represent those of their affiliated organizations, or those of the publisher, the editors and the reviewers. Any product that may be evaluated in this article, or claim that may be made by its manufacturer, is not guaranteed or endorsed by the publisher.
The Supplementary Material for this article can be found online at: https://www.frontiersin.org/articles/10.3389/fclim.2023.1330388/full#supplementary-material
Ali, E., Cramer, W., Carnicer, J., Georgopoulou, E., Hilmi, N., Cozannet, G. L., et al. (2022). “CCP4–Mediterranean region,” in Climate Change 2022? Impacts, Adaptation and Vulnerability, eds H.-O. Pörtner, D. C. Roberts, M. Tignor, E. S. Poloczanska, K. Mintenbeck, A. Alegría, M. Craig, S. Langsdorf, S. Löschke, V. Möller, A. Okem, B. Rama (Cambridge, UK; New York, NY: Cambridge University Press), 2233–2272.
ARPA-FVG (2018). Studio conoscitivo dei cambiamenti climatici e di alcuni loro impatti in Friuli Venezia Giulia. Tech. Rep. I, Palmanova.
Arpaia, L., Ferrarin, C., Bajo, M., and Umgiesser, G. (2023). A flexible z-layers approach for the accurate representation of free surface flows in a coastal ocean model (SHYFEM v. 7_5_71). Geosci. Model Dev. 16, 6899–6919. doi: 10.5194/gmd-16-6899-2023
Baldan, D., Coraci, E., Crosato, F., Cornello, M., Ferla, M., Morucci, S., et al. (2023). Return periods of extreme sea levels: from magnitude to frequency, duration and seasonality. Implications in a regulated coastal lagoon. Sci. Tot. Environ. 866, 161326. doi: 10.1016/j.scitotenv.2022.161326
Benetazzo, A., Davison, S., Barbariol, F., Mercogliano, P., Favaretto, C., and Sclavo, M. (2022). Correction of ERA5 wind for regional climate projections of sea waves. Water 14, 1590. doi: 10.3390/w14101590
Bonaldo, D., Bucchignani, E., Pomaro, A., Ricchi, A., Sclavo, M., and Carniel, S. (2020). Wind waves in the Adriatic Sea under a severe climate change scenario and implications for the coasts. Int. J. Climatol. 40, 5389–5406. doi: 10.1002/joc.6524
Bonaldo, D., Bucchignani, E., Ricchi, A., and Carniel, S. (2017). Wind storminess in the Adriatic Sea in a climate change scenario. Acta Adriat. 58, 195–208. doi: 10.32582/aa.58.2.1
Brogli, R., Heim, C., Mensch, J., Sørland, S. L., and Schär, C. (2023). The pseudo-global-warming (PGW) approach: methodology, software package PGW4ERA5 v1.1, validation, and sensitivity analyses. Geosci. Model Dev. 16, 907–926. doi: 10.5194/gmd-16-907-2023
C3S (2018). Sea Level Daily Gridded Data from Satellite Observations for the Mediterranean Sea from 1993 to 2020. Copernicus Climate Change Service (C3S) Climate Data Store (CDS). doi: 10.24381/cds.012e84d8
Camatti, E., Acri, F., De Lazzari, A., Nurra, N., Pansera, M., Schroeder, A., et al. (2023). Natural or anthropogenic variability? A long-term pattern of the zooplankton communities in an ever-changing transitional ecosystem. Front. Mar. Sci. 10, 1176829. doi: 10.3389/fmars.2023.1176829
Caruso, G., Leonardi, M., Monticelli, L., Decembrini, F., Azzaro, F., Crisafi, E., et al. (2010). Assessment of the ecological status of transitional waters in Sicily (Italy): First characterisation and classification according to a multiparametric approach. Mar. Pollut. Bull. 60, 1682–1690. doi: 10.1016/j.marpolbul.2010.06.047
Chiggiato, J., Schroeder, K., Mourre, B., Miramontes, E., Lionello, P., Marcos, M., et al. (2023). “Chapter 1: Introduction,” in Oceanography of the Mediterranean Sea, eds K. Schroeder and J. Chiggiato (Amsterdam: Elsevier), 1–11. doi: 10.1016/B978-0-12-823692-5.00002-9
Christidis, N., and Stott, P. A. (2022). Human influence on seasonal precipitation in Europe. J. Climate 35, 5215–5231. doi: 10.1175/JCLI-D-21-0637.1
Dayan, H., McAdam, R., Juza, M., Masina, S., and Speich, S. (2023). Marine heat waves in the Mediterranean Sea: an assessment from the surface to the subsurface to meet national needs. Front. Mar. Sci. 10, 1045138. doi: 10.3389/fmars.2023.1045138
Domínguez, R., Olabarria, C., Woodin, S. A., Wethey, D. S., Peteiro, L. G., Macho, G., et al. (2021). Contrasting responsiveness of four ecologically and economically important bivalves to simulated heat waves. Mar. Environ. Res. 164, 105229. doi: 10.1016/j.marenvres.2020.105229
Dosio, A., and Fischer, E. M. (2018). Will half a degree make a difference? Robust projections of indices of mean and extreme climate in Europe under 1.5 C, 2 C, and 3 C global warming. Geophys. Res. Lett. 45, 935–944. doi: 10.1002/2017GL076222
Drenkard, E. J., Stock, C., Ross, A. C., Dixon, K. W., Adcroft, A., Alexander, M., et al. (2021). Next-generation regional ocean projections for living marine resource management in a changing climate. ICES J. Mar. Sci. 78, 1969–1987. doi: 10.1093/icesjms/fsab100
Ferrarin, C., Bajo, M., Bellafiore, D., Cucco, A., De Pascalis, F., Ghezzo, M., et al. (2014). Toward homogenization of Mediterranean lagoons and their loss of hydrodiversity. Geophys. Res. Lett. 41, 5935–5941. doi: 10.1002/2014GL060843
Ferrarin, C., Bajo, M., and Umgiesser, G. (2021). Model-driven optimization of coastal sea observatories through data assimilation in a finite element hydrodynamic model (SHYFEM v.7_5_65). Geosci. Model Dev. 14, 645–659. doi: 10.5194/gmd-14-645-2021
Ferrarin, C., Ghezzo, M., Umgiesser, G., Tagliapietra, D., Camatti, E., Zaggia, L., et al. (2013). Assessing hydrological effects of human interventions on coastal systems: numerical applications to the Venice Lagoon. Hydrol. Earth Sys. Sci. 17, 1733–1748. doi: 10.5194/hess-17-1733-2013
Ferrarin, C., Lionello, P., Orlić, M., Raicich, F., and Salvadori, G. (2022). Venice as a paradigm of coastal flooding under multiple compound drivers. Sci. Rep. 12, 5754. doi: 10.1038/s41598-022-09652-5
Ferrarin, C., Tomasin, A., Bajo, M., Petrizzo, A., and Umgiesser, G. (2015). Tidal changes in a heavily modified coastal wetland. Cont. Shelf Res. 101, 22–33. doi: 10.1016/j.csr.2015.04.002
Ferrarin, C., Valentini, A., Vodopivec, M., Klaric, D., Massaro, G., Bajo, M., et al. (2020). Integrated sea storm management strategy: the 29 October 2018 event in the Adriatic Sea. Nat. Hazards Earth Syst. Sci. 20, 73–93. doi: 10.5194/nhess-20-73-2020
Fox-Kemper, B., Hewitt, H., Xiao, C., Aoalgeirsdóttir, G., Drijfhout, S., Edwards, T., et al. (2021). Ocean, Cryosphere and Sea Level Change. Cambridge, UK; New York, NY: Cambridge University Press.
Frölicher, T. L., and Laufkötter, C. (2018). Emerging risks from marine heat waves. Nat. Commun. 9, 650. doi: 10.1038/s41467-018-03163-6
Garrabou, J., Coma, R., Bensoussan, N., Bally, M., Chevaldonné, P., Cigliano, M., et al. (2009). Mass mortality in Northwestern Mediterranean rocky benthic communities: effects of the 2003 heat wave. Glob. Chang. Biol. 15, 1090–1103. doi: 10.1111/j.1365-2486.2008.01823.x
Garrabou, J., Gómez-Gras, D., Medrano, A., Cerrano, C., Ponti, M., Schlegel, R., et al. (2022). Marine heatwaves drive recurrent mass mortalities in the Mediterranean Sea. Glob. Chang. Biol. 28, 5708–5725. doi: 10.1111/gcb.16301
Gattuso, J.-P., Magnan, A. K., Bopp, L., Cheung, W. W. L., Duarte, C. M., Hinkel, J., et al. (2018). Ocean solutions to address climate change and its effects on marine ecosystems. Front. Mar. Sci. 5, 337. doi: 10.3389/fmars.2018.00337
Ghezzo, M., Pellizzato, M., De Pascalis, F., Silvestri, S., and Umgiesser, G. (2018). Natural resources and climate change: a study of the potential impact on Manila clam in the Venice lagoon. Sci. Tot Environ. 645, 419–430. doi: 10.1016/j.scitotenv.2018.07.060
Guelorget, O., and Perthuisot, J. P. (1989). The Paralic Realm, Geological, Biological and Economic Expression of Confinement. Tech. Rep. FAO-FI-RAB/83/016, FAO, Rome.
Hobday, A. J., Alexander, L. V., Perkins, S. E., Smale, D. A., Straub, S. C., Oliver, E. C. J., et al. (2016). A hierarchical approach to defining marine heatwaves. Prog. Oceanogr. 141, 227–238. doi: 10.1016/j.pocean.2015.12.014
Hussain, M. M., and Mahmud, I. (2019). pyMannKendall: a Python package for non parametric Mann Kendall family of trend tests. J. Open Source Softw. 4, 1556. doi: 10.21105/joss.01556
Juza, M., Fernández-Mora, A., and Tintoré, J. (2022). Sub-regional marine heat waves in the Mediterranean Sea from observations: long-term surface changes, sub-surface and coastal responses. Front. Mar. Sci. 9, 785771. doi: 10.3389/fmars.2022.785771
Lionello, P., Barriopedro, P., Ferrarin, C., Nicholls, R., Orlić, M., Raicich, F., et al. (2021). Extreme floods of Venice: characteristics, dynamics, past and future evolution (review article). Nat. Hazards Earth Syst. Sci. 21, 2705–2731. doi: 10.5194/nhess-21-2705-2021
Lionello, P., Conte, D., Marzo, L., and Scarascia, L. (2017). The contrasting effect of increasing mean sea level and decreasing storminess on the maximum water level during storms along the coast of the Mediterranean Sea in the mid 21st century. Glob. Planet. Change 151, 80–91. doi: 10.1016/j.gloplacha.2016.06.012
Lionello, P., Giorgi, F., Rohling, E., and Seager, R. (2023). “Mediterranean climate: past, present and future,” in Oceanography of the Mediterranean Sea, eds K. Schroeder and J. Chiggiato (Amsterdam: Elsevier), 41–91. doi: 10.1016/B978-0-12-823692-5.00011-X
Lionello, P., and Scarascia, L. (2018). The relation between climate change in the Mediterranean region and global warming. Reg. Environ. Change 18, 1481–1493. doi: 10.1007/s10113-018-1290-1
Madricardo, F., Foglini, F., Kruss, A., Ferrarin, C., Pizzeghello, N. M., Murri, C., et al. (2017). High-resolution multibeam and hydrodynamic datasets of tidal channels and inlets of the Lagoon of Venice. Sci. Data 4, 170121. doi: 10.1038/sdata.2017.121
Makris, C. V., Tolika, K., Baltikas, V. N., Velikou, K., and Krestenitis, Y. N. (2023). The impact of climate change on the storm surges of the Mediterranean Sea: coastal sea level responses to deep depression atmospheric systems. Ocean Model. 181, 102149. doi: 10.1016/j.ocemod.2022.102149
Mann, H. B. (1945). Nonparametric tests against trend. Econometrica 13, 245–259. doi: 10.2307/1907187
Martínez, J., Leonelli, F. E., García-Ladona, E., Garrabou, J., Kersting, D. K., Bensoussan, N., et al. (2023). Evolution of marine heatwaves in warming seas: the Mediterranean Sea case study. Front. Mar. Sci. 10, 1193164. doi: 10.3389/fmars.2023.1193164
MedECC (2020). Climate and Environmental Change in the Mediterranean Basin - Current Situation and Risks for the Future. First Mediterranean Assessment Report. Tech. rep., Marseille. doi: 10.5281/zenodo.4768833
Mel, R. A., Coraci, E., Morucci, S., Crosato, F., Cornello, M., Casaioli, M., et al. (2023). Insights on the extreme storm surge event of the 22 November 2022 in the Venice Lagoon. J. Mar. Sci. Eng. 11, 1750. doi: 10.3390/jmse11091750
Meli, M., Camargo, C. M. L., Olivieri, M., Slangen, A. B. A., and Romagnoli, C. (2023). Sea-level trend variability in the Mediterranean during the 1993-2019 period. Front. Mar. Sci. 10, 1150488. doi: 10.3389/fmars.2023.1150488
Mishra, A. K., Jangir, B., and Strobach, E. (2023). Does increasing climate model horizontal resolution be beneficial for the Mediterranean region?: Multimodel evaluation framework for high-Resolution model intercomparison project. J. Geophys. Res. Atmos. 128, e2022JD037812. doi: 10.1029/2022JD037812
Mohamed, B., Abdallah, A. M., Alam El-Din, K., Nagy, H., and Shaltout, M. (2019). Inter-annual variability and trends of sea level and sea surface temperature in the Mediterranean Sea over the last 25 years. Pure Appl. Geophys. 176, 3787–3810. doi: 10.1007/s00024-019-02156-w
Mudelsee, M. (2019). Trend analysis of climate time series: a review of methods. Earth Sci. Rev. 190, 310–322. doi: 10.1016/j.earscirev.2018.12.005
Munari, C. (2011). Effects of the 2003 European heatwave on the benthic community of a severe transitional ecosystem (Comacchio Saltworks, Italy). Mar. Pollut. Bull. 62, 2761–2770. doi: 10.1016/j.marpolbul.2011.09.011
Newton, A., Icely, J., Cristina, S., Brito, A., Cardoso, A. C., Colijn, F., et al. (2014). An overview of ecological status, vulnerability and future perspectives of european large shallow, semi-enclosed coastal systems, lagoons and transitional waters. Estuar. Coast. Shelf Sci. 140, 95–122. doi: 10.1016/j.ecss.2013.05.023
Ozer, T., Gertman, I., Gildor, H., and Herut, B. (2022). Thermohaline temporal variability of the SE Mediterranean coastal waters (Israel) - long-term trends, seasonality, and connectivity. Front. Mar. Sci. 8, 799457. doi: 10.3389/fmars.2021.799457
Pérez Gómez, B., Vilibić, I., Šepić, J., Medugorac, I., Ličer, M., Testut, L., et al. (2022). Coastal sea level monitoring in the Mediterranean and Black seas. Ocean Sci. 18, 997–1053. doi: 10.5194/os-18-997-2022
Philandras, C. M., Nastos, P. T., Kapsomenakis, J., Douvis, K. C., Tselioudis, G., and Zerefos, C. S. (2011). Long term precipitation trends and variability within the Mediterranean region. Nat. Hazards Earth Syst. Sci. 11, 3235–3250. doi: 10.5194/nhess-11-3235-2011
Pisano, A., Marullo, S., Artale, V., Falcini, F., Yang, C., Leonelli, F. E., et al. (2020). New evidence of Mediterranean climate change and variability from sea surface temperature observations. Remote Sens. 12, 132. doi: 10.3390/rs12010132
Pivato, M., Carniello, L., Gardner, J., Silvestri, S., and Marani, M. (2018). Water and sediment temperature dynamics in shallow tidal environments: the role of the heat flux at the sediment-water interface. Adv. Water Resour. 113, 126–140. doi: 10.1016/j.advwatres.2018.01.009
Pomaro, A., Cavaleri, L., Papa, A., and Lionello, P. (2018). 39 years of directional wave recorded data and relative problems, climatological implications and use. Sci. Data 5, 180139. doi: 10.1038/sdata.2018.139
Ponti, M., Castellini, A., Ragazzoni, A., Gamba, E., Ceccherelli, V., and Abbiati, M. (2017). Decline of the Manila clams stock in the northern Adriatic lagoons: a survey on ecological and socio-economic aspects. Acta Adriat. 58, 89–104. doi: 10.32582/aa.58.1.7
Raicich, F., and Colucci, R. R. (2019). A near-surface sea temperature time series from Trieste, northern Adriatic Sea (1899–2015). Earth Sys. Sci. Data 11, 761–768. doi: 10.5194/essd-11-761-2019
Rajczak, J., Pall, P., and Schár, C. (2013). Projections of extreme precipitation events in regional climate simulations for Europe and the Alpine Region. J. Geophys. Res. Atmos. 118, 3610–3626. doi: 10.1002/jgrd.50297
Sammartino, M., Aronica, S., Santoleri, R., and Buongiorno Nardelli, B. (2022). Retrieving Mediterranean sea surface salinity distribution and interannual trends from multi-sensor satellite and in situ data. Remote Sens. 14, 2502. doi: 10.3390/rs14102502
Sannino, G., Carillo, A., Iacono, R., Napolitano, E., Palma, M., Pisacane, G., et al. (2022). Modelling present and future climate in the Mediterranean Sea: a focus on sea-level change. Clim. Dynam. 59, 357–391. doi: 10.1007/s00382-021-06132-w
Schlegel, R., Darmaraki, S., Benthuysen, J., Filbee-Dexter, K., and Oliver, E. (2021). Marine cold-spells. Prog. Oceanogr. 198, 102684. doi: 10.1016/j.pocean.2021.102684
Schlegel, R. W., Oliver, E. C., Wernberg, T., and Smit, A. J. (2017). Nearshore and offshore co-occurrence of marine heatwaves and cold-spells. Prog. Oceanogr. 151, 189–205. doi: 10.1016/j.pocean.2017.01.00
Schlumberger, J., Ferrarin, C., Jonkman, S. N., Diaz Loaiza, A., Antonini, A., and Fatorić, S. (2022). Developing a framework for the assessment of current and future flood risk in Venice, Italy. Nat. Hazards Earth Syst. Sci. 22, 2381–2400. doi: 10.5194/nhess-22-2381-2022
Smith, K. E., Burrows, M. T., Hobday, A. J., King, N. G., Moore, P. J., Sen Gupta, A., et al. (2023). Biological impacts of marine heatwaves. Annu. Rev. Mar. Sci. 15, 119–145. doi: 10.1146/annurev-marine-032122-121437
Tagliapietra, D., Aloui-Bejaoui, N., Bellafiore, D., Wit, R. D., Ferrarin, C., Gamito, S., et al. (2012a). The Ecological Implications of Climate Change on the Lagoon of Venice. Tech. rep., UNESCO Venice Office, Venice.
Tagliapietra, D., Sigovini, M., and Magni, P. (2012b). Saprobity: a unified view of benthic succession models for coastal lagoons. Hydrobiologia 686, 15–28. doi: 10.1007/s10750-012-1001-8
Tagliapietra, D., and Umgiesser, G. (2023). Venice and its lagoon fin de siecle. Reg. Environ. Change 23, 125. doi: 10.1007/s10113-023-02120-4
Tognin, D., Finotello, A., D'Alpaos, A., Viero, D. P., Pivato, M., Mel, R. A., et al. (2022). Loss of geomorphic diversity in shallow tidal embayments promoted by storm-surge barriers. Sci. Adv. 8, eabm8446. doi: 10.1126/sciadv.abm8446
Tuel, A., and Eltahir, E. A. B. (2020). Why is the Mediterranean a climate change hot spot? J. Clim. 33, 5829–5843. doi: 10.1175/JCLI-D-19-0910.1
Umgiesser, G. (2020). The impact of operating the mobile barriers in Venice (MOSE) under climate change. J. Nat. Conserv. 54, 125783. doi: 10.1016/j.jnc.2019.125783
Umgiesser, G., Ferrarin, C., Bajo, M., Bellafiore, D., Cucco, A., De Pascalis, F., et al. (2022). Hydrodynamic modelling in marginal and coastal seas - The case of the Adriatic Sea as a permanent laboratory for numerical approach. Ocean Model. 179, 102123. doi: 10.1016/j.ocemod.2022.102123
Umgiesser, G., Ferrarin, C., Cucco, A., De Pascalis, F., Bellafiore, D., Ghezzo, M., et al. (2014). Comparative hydrodynamics of 10 Mediterranean lagoons by means of numerical modeling. J. Geophys. Res. Oceans 119, 2212–2226. doi: 10.1002/2013JC009512
Umgiesser, G., and Matticchio, B. (2006). Simulating the mobile barrier (MOSE) operation in the Venice Lagoon, Italy: global sea level rise and its implication for navigation. Ocean Dyn. 56, 320–332. doi: 10.1007/s10236-006-0071-4
Umgiesser, G., Melaku Canu, D., Cucco, A., and Solidoro, C. (2004). A finite element model for the Venice Lagoon. Development, set up, calibration and validation. J. Mar. Syst. 51, 123–145. doi: 10.1016/j.jmarsys.2004.05.009
van der Plas, F., Janzen, T., Ordonez, A., Fokkema, W., Reinders, J., Etienne, R. S., et al. (2015). A new modeling approach estimates the relative importance of different community assembly processes. Ecology 96, 1502–1515. doi: 10.1890/14-0454.1
Vautard, R., Gobiet, A., Sobolowski, S., Kjellström, E., Stegehuis, A., Watkiss, P., et al. (2014). The European climate under a 2 C global warming. Environ. Res. Lett. 9, 034006. doi: 10.1088/1748-9326/9/3/034006
Weiher, E., Freund, D., Bunton, T., Stefanski, A., Lee, T., and Bentivenga, S. (2011). Advances, challenges and a developing synthesis of ecological community assembly theory. Philos. Trans. R. Soc. B 366, 2403–2413. doi: 10.1098/rstb.2011.0056
Zanchettin, D., Bruni, S., Raicich, F., Lionello, P., Adloff, F., Androsov, A., et al. (2021). Sea-level rise in Venice: historic and future trends (review article). Nat. Hazards Earth Syst. Sci. 21, 2643–2678. doi: 10.5194/nhess-21-2643-2021
Zittis, G., Bruggeman, A., and Lelieveld, J. (2021). Revisiting future extreme precipitation trends in the Mediterranean. Weather. Clim. Extremes 34, 100380. doi: 10.1016/j.wace.2021.100380
Keywords: climatic trends, relative sea level rise, extreme sea levels, marine heat waves, Venice
Citation: Ferrarin C, Bonaldo D, Bergamasco A and Ghezzo M (2024) Sea level and temperature extremes in a regulated Lagoon of Venice. Front. Clim. 5:1330388. doi: 10.3389/fclim.2023.1330388
Received: 30 October 2023; Accepted: 28 December 2023;
Published: 12 January 2024.
Edited by:
Jing Yang, Beijing Normal University, ChinaReviewed by:
Xin Qi, Ocean University of China, ChinaCopyright © 2024 Ferrarin, Bonaldo, Bergamasco and Ghezzo. This is an open-access article distributed under the terms of the Creative Commons Attribution License (CC BY). The use, distribution or reproduction in other forums is permitted, provided the original author(s) and the copyright owner(s) are credited and that the original publication in this journal is cited, in accordance with accepted academic practice. No use, distribution or reproduction is permitted which does not comply with these terms.
*Correspondence: Christian Ferrarin, Y2hyaXN0aWFuLmZlcnJhcmluQGNuci5pdA==
Disclaimer: All claims expressed in this article are solely those of the authors and do not necessarily represent those of their affiliated organizations, or those of the publisher, the editors and the reviewers. Any product that may be evaluated in this article or claim that may be made by its manufacturer is not guaranteed or endorsed by the publisher.
Research integrity at Frontiers
Learn more about the work of our research integrity team to safeguard the quality of each article we publish.