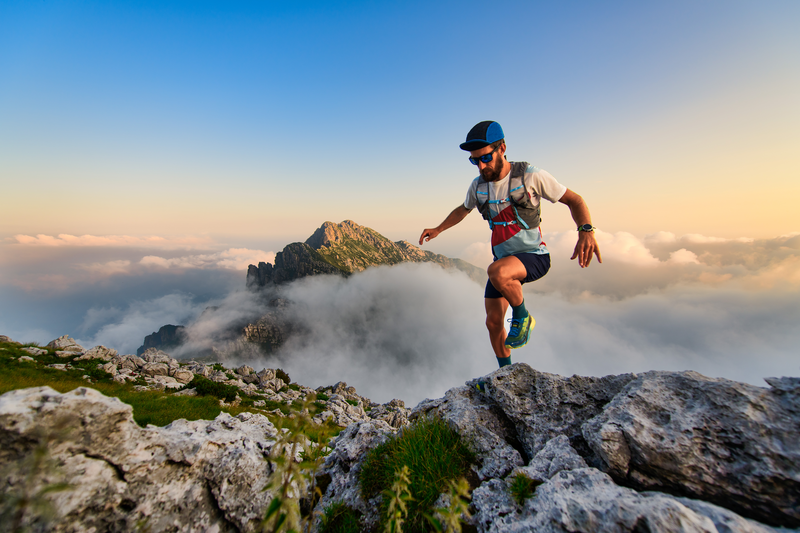
94% of researchers rate our articles as excellent or good
Learn more about the work of our research integrity team to safeguard the quality of each article we publish.
Find out more
REVIEW article
Front. Clim. , 06 July 2023
Sec. Carbon Dioxide Removal
Volume 5 - 2023 | https://doi.org/10.3389/fclim.2023.1169665
This article is part of the Research Topic Nature-Based Solutions, Climate Mitigation, Biodiversity Conservation View all 5 articles
The deep sea (below 200 m depth) is the largest carbon sink on Earth. It hosts abundant biodiversity that underpins the carbon cycle and provides provisioning, supporting, regulating and cultural ecosystem services. There is growing attention to climate-regulating ocean ecosystem services from the scientific, business and political sectors. In this essay we synthesize the unique biophysical, socioeconomic and governance characteristics of the deep sea to critically assess opportunities for deep-sea blue carbon to mitigate climate change. Deep-sea blue carbon consists of carbon fluxes and storage including carbon transferred from the atmosphere by the inorganic and organic carbon pumps to deep water, carbon sequestered in the skeletons and bodies of deep-sea organisms, carbon buried within sediments or captured in carbonate rock. However, mitigating climate change through deep-sea blue carbon enhancement suffers from lack of scientific knowledge and verification, technological limitations, potential environmental impacts, a lack of cooperation and collaboration, and underdeveloped governance. Together, these issues suggest that deep-sea climate change mitigation is limited. Thus, we suggest that a strong focus on blue carbon is too limited a framework for managing the deep sea to contribute to international goals, including the Sustainable Development Goals (SDGs), the Paris Agreement and the post-2020 Biodiversity Goals. Instead, the deep sea can be viewed as a more holistic nature-based solution, including many ecosystem services and biodiversity in addition to climate. Environmental impact assessments (EIAs), area-based management, pollution reduction, moratoria, carbon accounting and fisheries management are tools in international treaties that could help realize benefits from deep-sea, nature-based solutions.
Many international policy instruments, including the Sustainable Development Goals (SDGs), the Paris Agreement, the post-2020 Biodiversity framework, and the new high seas treaty on biodiversity and sustainable use (BBNJ Agreement) potentially have an important role to play in promoting a greater understanding, valuation, and conservation of deep-sea ecosystems and their services. The ocean contributes to international social, biodiversity and climate wellbeing, and the deep sea is frequently called out as a major carbon repository. Inversely, prioritizing the marine environment in the international community has increased awareness of actors and guided actions such as the UN Decade of Ocean Science for Sustainable Development (Levin, 2022).
A decade ago, the term “blue carbon” was coined to highlight the disproportionate and substantial contribution of coastal vegetated ecosystems to global carbon sequestration (Macreadie et al., 2019). The contribution of blue carbon in climate change mitigation and adaptation has gained international attention but also raised many questions about its potential as a solution in the form of human-induced interventions (Williamson and Gattuso, 2022). Blue carbon is defined here as all biologically-driven carbon fluxes and storage in marine systems that are amenable to management (Intergovernmental Panel on Climate Change, 2019; Lovelock and Duarte, 2019). There is current debate regarding the application of the Blue Carbon concept to other coastal and non-coastal processes and ecosystems, including the open ocean (Intergovernmental Panel on Climate Change, 2019). Coastal blue carbon has been widely adopted in international frameworks, most prominently in the UN Framework Convention on Climate Change (UNFCCC), to mitigate climate change (Herr and Landis, 2016; Hilmi et al., 2021). Recent discussions highlight the possibility of conserving and enhancing carbon sequestration in the open ocean and deep sea (Hilmi et al., 2021; Oostdijk et al., 2022; Levin et al., 2023).
The ocean covers almost 71% of the earth surface with an average depth of 3,688 m. It has sequestered more than 25% of excess carbon dioxide emitted into the atmosphere since the mid-1990s (Gruber et al., 2019; Watson et al., 2020), significantly buffering climate change. The deep sea is defined as marine areas of more than 200 m of depth. At this depth, there is little to no photosynthesis, creating unique conditions (Kaartvedt et al., 2019). In the context of this article, the deep sea includes waters, ocean floor and subsurface sediments, rocks, and biota. The deep-sea environment comprises over 95% of the habitable volume on the planet (IUCN, 2022).
Our knowledge of the deep sea is still limited. Nevertheless, it is known that the biophysical processes and biodiversity found in the deep sea support significant ecosystem services for humanity and life on Earth (Armstrong et al., 2012; Thurber et al., 2014). The biological carbon pump refers to organic carbon captured in the bodies of marine life (Sarmiento and Gruber, 2013). Marine life also actively transports carbon to deeper ocean layers, thus contributing to its sequestration in deep water and within the seafloor (Sarmiento and Gruber, 2013). While sinking occurs, active transport is conducted primarily by species inhabiting the mesopelagic zone (200–1,000 m) which can migrate vertically hundreds of meters each day (Boyd et al., 2019). The mesopelagic zone has been estimated to be the most biomass-rich ecosystem on our planet (1.8–16 Gt; Proud et al., 2019) and to contain approximately one million undescribed species (Robison, 2009).
Socioeconomic specificities of the deep ocean impact whether and how the deep sea is regulated, controlled and therefore managed. Based on the UN Convention on the Law of the Sea (UNCLOS), the deep ocean is split in two different legal regimes: two different legal regimes: areas within national jurisdiction such as Exclusive Economic Zones (EEZs) (up to 200 nautical miles from the coastline) and the continental shelf (Food Agriculture Organization, 2020) and where control and management of resources and conservation is under international conventions rather than national legislation of coastal states. Some nations have entitlements to an outer continental shelf which gives them access to additional resources on the seafloor beyond 200 nm. Furthermore, deep-sea ecosystems are not well characterized and there are great uncertainties regarding the amount and nature of actual or potential ecosystem services. While international rules have been agreed by most states under UNCLOS and are applicable to all different maritime zones, these rules outline minimum requirements. States that have signed and ratified UNCLOS can thus decide to develop stricter or more specific frameworks if they conform to the minimum international standards. Consequently, the different legal regimes and related rules applicable to the deep sea have an important impact on the management and control over its ecosystems, and thus on their socio-economic specificities.
In addition, there are biophysical, economic and governance factors to consider in assessing whether the management of deep-sea blue carbon could effectively contribute to the mitigation of climate change. One key factor is whether processes are amenable to management. Mitigation as defined above is reduction or prevention of emissions. This means that to be accounted for as mitigation action blue carbon must remove emissions from the atmosphere in the long-term and beyond natural sequestration rates. A guidance on such carbon removal has been developed for coastal wetlands (IPCC, 2013). Recent synthesis of protected areas worldwide demonstrate that they enhance carbon sequestration and thus can be considered to contribute to mitigation (Jacquemont et al., 2022).
This paper first discusses the biophysical foundations of deep-sea carbon and its changing dynamics. Then it considers the socioeconomic role the deep sea plays at the moment, as well as likely changes in the future. It expands on ecosystem services that play a significant socioeconomic role and values the ecosystem services provided by the deep sea. Third, it discusses the scientific, economic and governance factors limiting potential for deep-sea blue carbon to mitigate climate change. Together, these issues suggest that deep-sea climate change mitigation is limited. Thus, we suggest that a strong focus on blue carbon has limited value for achieving international climate goals. Instead, and in addition to its climate mitigation contributions, the deep sea can be viewed as contributory to more holistic nature-based solutions, including many ecosystem services and biodiversity in addition to climate. Hereupon, we discuss possible holistic international governance using common management instruments to realize benefits from deep-sea nature-based solutions.
The deep ocean consists of a heterogeneous set of ecosystems with different geomorphologies, physical and chemical attributes, and distinct animal and microbial communities (Ramirez-Llodra et al., 2011). On the sea floor, abyssal nodule provinces, canyons, seamounts, trenches, ridges, coral and sponge reefs and oxygen minimum zones are as varied as the environments on land. But the ocean encompasses a much larger third dimension that includes many different water column environments and the subseafloor.
Deep-sea ecosystems interface with climate change and the carbon emissions responsible for climate change in many ways. In the non-living (abiotic) realm, density stratification, thermohaline circulation and chemical interactions drive exchanges of heat, energy and chemical ions between surface and deep waters with consequences for atmospheric warming, sea level rise, ocean oxygen content and ocean acidification.
Living organisms from small plankton to fish in deep waters play roles in the uptake and fixation, transport, storage and sequestration of carbon. This is termed the biological pump and is crucial in transporting anthropogenic carbon from the atmosphere into the deep sea. Natural sinking of macroalgae (seaweed), marine mammal, shark and fish carcasses, as well as wood falls also contribute to the seafloor carbon stock (Krause-Jensen and Duarte, 2016; Chami et al., 2019). Once organic carbon reaches the deep ocean it may be remineralized by microbes and animals or buried. Even as remineralized CO2, it may remain sequestered for hundreds to thousands of years depending on the water depth and ocean basin (Siegel et al., 2021). The ubiquitous presence of relatively fresh, photosynthetically active diatom cells in the deep sea indicates that fast-sinking mechanisms such as the formation of aggregates and fecal pellets inject fresh organic carbon into deep oligotrophic regions globally (Agusti et al., 2015). Microbes and animals can also sequester carbon by precipitating carbonate. Animals oxidize methane (e.g., at vents and seeps) and store carbon in their tissues, carbonate skeletons and shells, and bury carbon through bioturbation (Le et al., 2022). The vast seafloor stores much of the ocean carbon (Figure 1) (Atwood et al., 2020). There are 2,239–2,391 Pg C in the top 1 m of ocean sediments, with 79% at abyssal depths and just under half the total carbon within EEZs (Atwood et al., 2021). Ocean sediments hold more than twice the carbon found in terrestrial soils.
Figure 1. Carbon sequestration rates in the ocean sediments (source: Atwood et al., 2020).
The deep sea is simultaneously under threat from climate change, contaminants including plastics and from resource extraction activities (Ramirez-Llodra et al., 2011; Mengerink et al., 2014). These anthropogenic challenges yield a host of physical, chemical and mechanical stressors that act on deep-sea ecosystems. Climate-induced changes in the environment and ocean circulation patterns can affect many deep-sea organisms at the individual, population, community and ecosystem level (Levin and Le Bris, 2015; Sweetman et al., 2017; Bindoff et al., 2019; Cheung et al., 2022), as revealed by both paleo and modern studies across natural gradients (Sperling et al., 2016; Bindoff et al., 2019; Yasuhara et al., 2020; Cooley et al., 2022; Yasuhara and Deutsch, 2022). Massive heat uptake by the ocean has led to warming that alters species distributions, changes phenology, raises metabolic demand, lowers the solubility of oxygen, increases stratification—affecting nutrient upwelling and oxygen mixing—all with effects on productivity, food supply to the seafloor, biomass production, biodiversity, body size and more (Sweetman et al., 2017; Bindoff et al., 2019). Although relatively few direct observations of change over time in the deep sea exist outside the fossil record, earth system model projections provide indication of extent of change expected (Sweetman et al., 2017; Kwiatkowski et al., 2020), time of emergence from natural variability and velocity of change (Brito-Morales et al., 2020). Altered ocean circulation change may also occur, affecting the distribution of heat, oxygen, CO2 and transport of organisms including propagules/larvae. CO2 uptake leads to ocean acidification with effects on metabolism, behavior, calcification, reproduction, and development (Sweetman et al., 2017). Taken together, there are modeled changes in global biodiversity (Cheung et al., 2022), population connectivity (Levin et al., 2020) and habitat suitability (Morato et al., 2020) that could result from excess carbon emissions. But no one has really examined how deep-sea systems might respond to climate stressors if they are simultaneously subject to anthropogenic stressors (e.g., fisheries, deep-sea mining, oil, and gas exploitation) that result in disruption or loss of suitable substrate, smothering from sediment plumes, toxicity from metal contaminant released from sediments or from hydrocarbons released in an oil spill. Loss of fish biomass by wild harvest removes carbon from the natural marine food chain and acts to reduce carbon storage in the deep ocean.
Recent IPCC reports indicate a need for active carbon removal from the atmosphere to achieve the goals of the Paris Agreement (IPCC, 2018, 2023). Increasingly, ocean-based climate interventions are being proposed or investigated that seek to enhance removal of CO2 from the atmosphere and use the deep ocean as a carbon repository, based on long residence times (NASEM, 2022). Many of these also have the potential to threaten the deep ocean's functions that transport, store or sequester carbon (Levin et al., 2023). Ocean warming is expanding low oxygen zones at bathyal depths and ocean fertilization [enhancing phytoplankton production through dissolved iron (Fe) and nutrient addition] and artificial upwelling are likely to exacerbate deoxygenation and enhance acidification. Some diel vertical migrators have migration depths set by oxygen tolerance, so this might reduce the daily vertical transport of carbon. Sinking of seaweed or crop waste into the deep ocean, proposed to sequester carbon, may also act to disrupt natural water column processes, smother benthic communities, create anoxic, low pH conditions and release additional greenhouse gasses (Boyd et al., 2022; Levin et al., 2023).
The question arises whether humans can co-opt and enhance the natural climate change mitigation services of the deep sea and how acceptable will this be to society? We know that the ocean capacity for heat and carbon dioxide storage is massive, that without the deep ocean, the planet would already be much hotter, but that mitigation capacity will diminish and negative feedbacks will occur over time as the ocean warms and becomes more acidic and deoxygenated (Laffoley et al., 2020). Additionally, the earlier discussion reveals that the existing sequestration functions we rely on may be compromised by stressors associated with climate change, resource exploitation and other anthropogenic activities in the deep ocean (e.g., Crain et al., 2008). Restoration of these services is notably missing from the industrial radar and in fact may not be possible in the deep sea given the slow growth and long generation times of many deep-sea organisms. Most proposals for ocean-based carbon dioxide removal or atmospheric albedo change will, if employed at the scale necessary to remove enough carbon to benefit climate, are almost certain to have negative environmental impacts on deep-sea pelagic or seafloor ecosystems, but the science is severely lacking (Levin et al., 2023). There is also minimal governance of these activities at present. While the London Convention and London Protocol regulate ocean dumping, among the CO2 removal technologies, only iron fertilization has been specifically addressed (in Annex 4 which has not yet gone into force). Addition of particles for ocean alkalinity enhancement, macroalgae, crop or wood waste sinking, or direct injection of CO2 are uncertain but could fall under the LC/LP, while artificial upwelling and downwelling, and OTEC are unregulated. State regulations for activities within EEZs are highly variable in terms of procedures and rigor. The new BBNJ Agreement could affect climate intervention activities beyond national jurisdiction (e.g., by identifying protected areas to avoid and requiring detailed EIAs), but states would still have control over their EIA process. Large-scale efforts to enhance carbon uptake and manipulate carbon in the ocean remain scientific, economic and legal frontiers.
The ecosystem services concept is a tool used to specify and quantify the link between human welfare and the environment (Böhnke-Henrichs et al., 2013). The deep sea, covering a vast area on the planet, provides a diversity of essential ecosystem regulating, provisioning and cultural services (Armstrong et al., 2012; Thurber et al., 2014; Ottaviani, 2020). Provisioning services are the products used by humans that are obtained directly from the ecosystem such as food, energy, and various chemicals. Regulating services are the benefits obtained through the natural regulation of ecosystem processes such as gas and climate regulation, and carbon sequestration. Cultural services are the non-material benefits people obtain from ecosystems through recreation, education, spirituality, aesthetic environment, “inspiration” and “awe”. Supporting services are those functions and processes that are necessary to produce all other ecosystem services, i.e., they feed into provisioning, regulating and cultural services thus feeding indirectly to human wellbeing. Their impacts on people are usually indirect, both physically and temporally (MA, 2005; Armstrong et al., 2010; Ottaviani, 2020). Consideration could also be given to other supporting services of the deep sea such as archeological sites and historical significance of the deep sea as trade routes and effects on civilizations, to name but some examples (Paine, 2015).
Direct uses or exploitation of deep-sea resources include oil and gas exploitation, fishing of various species, chemicals, minerals, and pharmaceuticals. Indirect uses of the deep sea underpin human existence on earth and include critical climate regulation. The oceans absorb about 90% of solar heat which it stores and distributes. The ocean holds about fifty times more CO2 than the atmosphere thus contributes extensively to temperature regulation. The deep sea is responsible for regeneration of nutrients from organic matter; as these return to surface waters they enable primary production that feeds life (including fish) in the ocean. It is difficult to estimate how much of these ecosystem services can be directly attributed to the deep sea alone. The deep sea provides habitat to many species which are of direct use to humans, including human wellbeing. Apart from these ecosystem services, the deep sea itself has value related to the advancement of knowledge and culture. Some parts of it are also recognized as world's cultural heritage sites (see further). There is a strong link between changes in ecosystem services and human wellbeing (MEA, 2005; Haines-Young and Potschin, 2011; Food Agriculture Organization, 2017). Because the deep sea is presently one of the least known and exploited areas of the planet, by learning from the past, pitfalls from land and shallow water can be avoided. Ongoing activities such as deep-water oil and gas extraction and bottom trawling, and emerging activities with direct impacts such as deep seabed mining, mesopelagic fisheries and offshore (deep) wind energy can benefit from accumulated knowledge and additional research.
Microbes underpin many of the deep-sea ecosystem services described above, in Table 1 and in more detail below, but these are rarely considered in management and conservation actions (Orcutt et al., 2020). Through production of biomass, metabolites, C fixation, oxidation and reduction processes, they can generate or remineralize organic matter releasing nutrients and sequestering carbon, transform greenhouse gases like CH4 and H2S, facilitate C sequestration through precipitation of carbonate, and detoxify compounds. They also provide food and cues that enable biodiversity, an array of genetic resources of industrial value, and have scientific and educational value (Orcutt et al., 2020).
The deep sea, as the world's largest biome (Ramirez-Llodra et al., 2011; Dawson, 2012), supplies us with a variety of ecosystem goods and services, some of which are tradeable and have a direct market value. These derive from living resources that support fisheries, or generate marine-genetic resources, and from non-living resources such as oil, gas, and potentially minerals.
Connections with surface waters and shallow coastal settings enable deep offshore environments to support the food web for a diversity of organisms and the societies that depend on them (St John et al., 2016). A diversity of fish (e.g., tuna, bill fish, and sharks), squid and shrimp, marine mammals, and marine reptiles feed on organisms in the deep sea, and also provide direct benefits to societies (Martin et al., 2020). Among these, tuna, bill fish, blue whiting, shrimp, and squid support economically important industries such as fisheries and/or tourism (St John et al., 2016; Hidalgo and Browman, 2019; Prellezo, 2019; Martin et al., 2020). Deep-sea fishing dates back to the late 1960s when factory trawlers were developed (Ramirez-Llodra et al., 2011). Larkin et al. (2015) proposed that almost 40% of the world's fishing beds are located in the deep sea. Ottaviani (2020) estimates catch volumes of 7.4 million tons with an economic value of 9,469 million USD per year. Alaska pollock, Atlantic cod, and Argentine shortfin squid account for 78% of the total catch volume. Deep sea commercial exploitation has traditionally been limited but recently received increasing attention in science and policy (Schadeberg et al., 2023). Fishing moratoria and research funding have been issued in different parts of the world (Brooks et al., 2022; NOAA, 2022).
Interest to feed the world's growing population, and a growing appetite for seafood and luxury fish products, has sparked interest in harvesting the mesopelagic zone for the development of fishmeal for aquaculture (Olsen et al., 2020; Dowd et al., 2022). The biomass of the mesopelagic zone is estimated to range between 1 and 16 GT, which makes it the most biomass-rich ecosystem on the planet (St John et al., 2016; Proud et al., 2019; Martin et al., 2020). It has been estimated to contain approximately one million undescribed species (Robison, 2009) and bristlemouths (Cyclothone), the most abundant vertebrate genus on Earth (Sutton et al., 2010; Sutton, 2013).
There is a high level of diversity recognized in the deep sea although many species remain undescribed (Kendall and Haedrich, 2006; Campbell et al., 2011; Ramirez-Llodra et al., 2011). This diversity in general contains numerous chemical compounds which have potential as pharmaceuticals (Mayer et al., 2010; Martins et al., 2014; Blasiak et al., 2021), neutraceuticals and industrial agents, or uses in biotechnology or biomimicry (Jobstvogt et al., 2014b; Prellezo, 2019; Blasiak et al., 2022). How many reside in deep-sea areas is uncertain. Of the thousands of existing compounds, only a few have currently been approved by the US FDA and/or the European EMAEA for pharmaceutical use. Deep-sea organisms offer the largest pool of genetic resources and biological components (Armstrong et al., 2012). Marine genetic resources contain two types of biochemical and genetic substances of marine organisms (Oldham et al., 2014). Metabolites produced by microorganisms or bottom-dwelling fauna, such as corals, sponges and tunicates, can have pharmaceutical properties (Oldham et al., 2014; Bibi et al., 2017). Today, various initiatives show a rapidly growing interest in using organisms found in the deep sea to generate new drugs for diseases such as cancer, Alzheimer's disease, asthma, viral infections and for bone grafting (McAllister, 1988; Witherell and Coon, 2001; Grehan et al., 2003), nutraceuticals, and industrial products (Larkin et al., 2015). Biotechnological applications include creating fiber optics, manufacturing glass along with civil engineering uses, and semiconductor production (Hogg et al., 2010). Some deep-water sponges and cold-water corals are even used in designing structures in an ecologically friendly way, reflecting those found in nature. They are also used as templates for molecular modeling (Ehrlich et al., 2006). Estimates showed a total annual value of USD 2,300 million for marine origin drugs in pharmaceuticals in 2014.
The exploration for and extraction of oil and gas is now common in deeper waters (Merrie et al., 2014; Jouffray et al., 2020). Experts believe that nearly 37% of proven oil reserves (the amount with a 90% or greater probability of profitable exploitation) are below the seafloor and one-third are in deep-ocean areas (OECD, 2016). While just 2% of the oil had been extracted from the deep sea beyond the continental shelf in 2001, it is estimated that by 2015, almost 7% of offshore oil—~2% of the total world oil production—had been extracted from the deep sea (OECD, 2016). Offshore oil production is now estimated at 30% of global oil production (Ottaviani, 2020). The US Energy Information Administration however recognizes that deep-water production requires more investment, enabling only a few states to venture to these depths, with currently Brazil, the United States, Angola and Norway leading the way. (https://www.eia.gov/todayinenergy/detail.php?id=28552). Considering the exploitation of 6.1 million barrels/day by these states in 2014 (EIA, 2016), the economic value of deep-water and ultra-deep-water crude oil production has been assessed to be nearly USD 217 000 million (Ottaviani, 2020). Increased demand and higher prices of oil could incite producers to consider exploration in deeper waters. But there is a growing movement to leave deep-sea oil and gas in place, spurred by the recognition that reducing fossil fuel reliance is key to addressing climate change. Experts at one time believed that the gas-hydrate deposits found in deep-ocean sediments could be a valuable source of natural gas for the future (NOAA, 2018) but as concern over methane contributions to global warming rises, interest in this potential energy source has waned. Accordingly, there is a growing demand for investing in offshore renewable energy opportunities such as floating wind and deep-ocean turbines and Ocean Thermal Energy Conversion (OTEC) (Larkin et al., 2015; Haugan et al., 2020).
Corals have for a long time been used for ornament in many ways. Ten species of precious corals have commercial use in making jewelry. Economic data for just two species are available—the red coral (Corallium rubrum) and the pink coral (Pleurocorallium elatius). In 2014, 55 tons of red coral and 19 tons of pink coral traded with estimated values of USD 83 million and USD 38 million, respectively. It is difficult to assess the total economic value of this industry, but Ottaviani (2020) estimates it to be above 120 million USD per year. The Japanese collect deep-sea hexactinellid sponges (Euplectella sp.) that host a paired male and female shrimp inside for life, as wedding gifts to portend good fortune (Saito et al., 2002).
The deep sea contains many valuable minerals found within polymetallic nodules, massive sulfides, and cobalt-rich ferromanganese crusts (Sharma, 2022), as well as nutrients and rare earth elements in phosphorites (Hein et al., 2016). Polymetallic nodule mining is being explored as a potential source of copper, nickel, cobalt, titanium, vanadium, and iron needed for vehicle electrification and other aspects of the green revolution (Hein et al., 2013; Le et al., 2017). Cobalt-rich ferromanganese crusts are high sources of manganese and iron, along with exploitable minerals such as cobalt, copper, platinum, thallium and tellurium (Hein et al., 2013). They can provide up to 20% of the global cobalt demand (Cochonat et al., 2007), though exploitation has not yet been undertaken or shown to be cost-effective (Levin et al., 2020). In addition, massive sulfide deposits have high contents of zinc, copper, lead, cadmium, gold and silver useful in tech industries (Baker and German, 2008; Le et al., 2017). Notably, vent deposits represent a very small fraction of land-based reserves for metals of interest whereas the cobalt in nodule and crust zones host over 300% of land-based reserves (Levin et al., 2020). These estimations are however, based on deposit estimates. Ottaviani (2020) estimated the value of deep-sea mining is more than USD 2,900 million according to 2014 prices. Phosphorite deposits are found in the deep sea on continental margins and are rich in calcium, flouride and phosphorous (Le et al., 2017) as well as rare earth elements and yttrium (Hein et al., 2016) and also hold commercial value for industry. But no definitive quantity nor bulk volumes of these deep-sea resources are currently known, making it difficult to develop of any supply model nor any return-on-investment assessment for deep-sea contractors.
Services that enable provisioning, regulating and cultural services are often considered supporting services. These sit at the heart of the value of biodiversity. The organisms that provide habitat (substrate), food, refuge, breeding grounds, nurseries or detoxification benefits for species that comprise fisheries, or hold spiritual or carbon sequestration value are often overlooked. These might include ecosystem engineers like cold-water corals, sponges, mussel and clam beds, tubeworm bushes or xenophyophores (large agglutinating protozoans).
The deep ocean plays an important role in transporting and storing heat and consequently in regulating our climate and weather patterns. For example, the Atlantic Meridional Overturning Circulation transports and exchanges water masses and heat throughout the world's oceans from surface to several kilometers depth (Kuhlbrodt et al., 2007; Thurber et al., 2014). It also plays an essential role in climate regulation by transporting carbon to the deep sea where it can be sequestered away from the atmosphere for decades or even centuries (Sarmiento and Gruber, 2006). Carbon is transported to the deep sea via gravitational flux/settling of particles and the active injection by living organisms in the ocean's biological carbon pump through respiration, fecal pellets and deadfalls (Boyd et al., 2019).
Deep midwater/mesopelagic communities represented over 90% of the biosphere (those occurring approximately 200–1,000 m below the surface (Robison, 2009). These mesopelagic communities connect surface and deep-water ecosystems and facilitate transport of carbon and nutrients between them (St John et al., 2016). Daily vertical migrations of mesopelagic organisms accelerate the injection of carbon to the deep sea; this injection ranges between 1 and 30 mgCm−2day−1, corresponding to 14–18% of the local passive sinking flux. It has been estimated that atmospheric carbon levels would be 200 ppm higher without the activity of the biological carbon pump and the sequestration of carbon to the deep sea via diel vertical migrations in the mesopelagic (Maier-Reimer et al., 1996). Using a more recent estimate of two to six billion metric tons of carbon sequestered by the daily migrations of mesopelagic organisms each year, and average values of social cost of carbon, Hoagland et al. (2019) estimated the value of daily vertical migration to be 300,000–900,000 million USD annually.
Deep-sea hydrothermal vents and seeps play important roles in biogeochemical cycling of carbon, sulfur, nitrogen, iron and arsenic, and provide nutrition that supports trophic interactions amongst benthic and planktonic organisms (Levin et al., 2016). Moreover, deep-sea microorganisms and animals associated with hydrothermal vents and methane seeps prevent gasses such as methane, CO2 and sulfide from entering the hydrosphere where they could exacerbate the effects of climate change (Jørgensen and Boetius, 2007; Le et al., 2022).
One of the main categories of ecosystem services is cultural services which are the non-material benefits obtained from ecosystems (Ottaviani, 2020). They include communication, recreation, research and education and benefits for physical and mental health, tourism, aesthetics, cultural heritage, inspiration for art and design, and spiritual benefits (M. E. A., 2005; TEEB, 2010; Armstrong et al., 2012; Böhnke-Henrichs et al., 2013; Food Agriculture Organization, 2017; Ottaviani, 2020). The value of recreation and leisure in the deep sea has been assessed at USD four million per year (Ottaviani, 2020). This estimate was obtained through summing up values obtained through literature searches on number of tourists enjoying recreation and cultural services related to deep-sea activities multiplied by prices paid to access these services (Ottaviani, 2020). Given the difficulties in obtaining reliable data and information, this should be considered to be an estimate open for revaluation. Aesthetic appreciation and art inspiration are the contribution of ecosystems to generate an emotional response to the subsurface landscape, and to inspire elements of culture, literature, film art, and/or design from environmental features, respectively. Finally, cultural heritage refers to the contribution of ecosystems in cultural traditions and folklore (Böhnke-Henrichs et al., 2013; United Nations Educational Scientific and Cultural Organization, 2022).
It might not come as a surprise that the possibilities to measure depths contributed much to exploration of all parts of the ocean. Bathymetry itself enabled the laying of submarine cables, linking the first successful executions of these two activities closely in time halfway into the nineteenth century (Rozwadowski, 2018). Deep-sea and undersea cables support global telecommunications, and these can be integrated with sensors that monitor the marine environment to monitor, e.g., climate and assess earthquake risk (Sladen et al., 2019). Over the decades, these submarine cables appear to have become an essential part of the global economy and, although unseen, one of the more important uses of the ocean (Ash, 2014; Burnett et al., 2014).
Considering the funds for scientific projects in the deep sea, Ottaviani (2020) calculates an estimated economic value of USD 5,800 million for research and education. Research and education are valuable for societies since they can lead to unexpected discoveries with medical, industrial, or nutritional applications (Levin et al., 2019). Deep-ocean literacy campaigns are emerging. For example, the Woods Hole Oceanographic Ocean Twilight Zone project has promoted ocean literacy by advancing several international public events, campaigns, and exhibitions that highlight exploration and discovery in deep-sea ecosystems, particularly the mesopelagic zone.1
Iconic species such as some marine turtles, marine mammals and fish that depend on the deep sea may also have cultural, spiritual or recreational value to many societies (Noble et al., 2016). The deep sea also has intrinsic value for future generations as its health is essential for climate stability and food security and for the other ecosystem services it provides.
Human discovery and increasing use of the ocean over time tightly connect with its perception and its role in spirituality. Depending on which ocean—the Indian, the Atlantic or the Pacific—and the era, marine imaginations encompass both coastal areas as well as the open ocean including the abyss. Evidently, the form and significance of this spirituality related to the ocean varies very much between different regions. For many indigenous people, the deep ocean features in origin myths, ancestral connections (where ancestors reside), and in resource custodianship (DOSI, 2021 https://www.dosi-project.org/resources/dosi-policy-briefs/). Some of the strongest connections occur in local and indigenous communities on islands, where the ocean is an intrinsic part of their culture. Whereas, these cultural services of the (deep) ocean date back millennia, if not to time immemorial, human's exploration and use of the deep ocean started at the earliest in the nineteenth century.
Regulating and climate-regulating services are likely to decrease with increasing ecosystem pressures. In the coming decades, provisioning services are expected to rise across most industries, including pharmaceuticals, mining, and fishing. Cultural services are likely to increase for education and research, yet spirituality around the deep sea is still underexplored.
There are several different ways in which we can estimate the economic value of deep-sea ecosystems and their services. As Ottaviani (2020) discusses in detail, the measurement of true economic values for the different services is not an easy task, especially when measuring services that are not sold in the market, such as many of the indirect services discussed above, most notably carbon sequestration.
One way to assess the economic value of deep-sea ecosystems is to value their benefits. Various studies have done this by looking at the “willingness to pay (WTP)” of people living in several states. For example, Glenn et al. (2010) estimated the willingness to pay yearly at £0–10 for cold-water coral protection in Ireland using choice experiments. Jobstvogt et al. (2014a) assessed a yearly WTP of £70–77 to create new marine protected areas (MPAs) in deep-sea locations in Scottish waters. Aanesen et al. (2015) determined a WTP of €235–287 per year to protect cold-water coral in Norway. O'Connor et al. (2020) concluded that each person would pay €34.69 annually to restore the Dohrn deep-sea canyon in the Bay of Naples so that the aggregate value would be approximately €127 million per year. Shen et al. (2015) found that Japanese respondents had highest WTP for carbon mitigation.
The valuation of deep-sea ecosystems is also complex due the lack of knowledge and understanding of these ecosystems. While the methodology of valuation of these unknown ecosystems is being discussed, it is reasonable to expect that it should imply valuing direct and indirect services provided by these ecosystems, including their role in the food chain and climate regulation. It should be acknowledged that this task is arduous, especially when it comes to the valuation of social (for example health), cultural and spiritual services. The social dimension is of particular importance due to the impact of environmental health on human health (physical and wellbeing), making the risk of social-environmental conflicts high for any human activities.
Furthermore, beyond the question of the social acceptability of the WTP, the remoteness of these ecosystems and the lack of “bonding” between citizens and the deep sea might hinder assessment of WTP both for continental shelves and Area ecosystems. Scientists overall recognize the crucial need to deploy at a larger scale marine scientific research to strengthen knowledge and understanding of these ecosystems, which are a prerequisite for valuing the services they provide to humans. Trust funds might be established for restoration and for covering residual damages not compensated by international law (Seabed Dispute Chamber, Advisory Opinion 2011). But these trust funds, not yet established for deep-sea ecosystems, are generally funded by the industry, not citizens. This is actually the case of the International Oil Pollution Compensation Funds, created following the Torrey Canyon incident as a way to address the shortcomings regarding liability and compensation mechanisms for victims of oil pollution. Restoration of deep-sea ecosystems requires first and foremost knowledge of ecosystem baselines. Acquisition of this knowledge and the restoration activities themselves may involve immense time scales due to slow growth and organism longevities of hundreds to thousands of years, slow precipitation of essential substrates like nodules and crusts, and exceedingly high cost and vast spatial scales of activities (Danovaro et al., 2021).
In a nutshell the evaluation of deep-sea ecosystem services is not an obvious and straightforward task. It faces different challenges, though it is deemed of high importance. To deal with the challenges, various studies have applied different approaches, and the next section presents some obtained findings.
The deep sea can be studied using a natural capital perspective (Baker et al., 2020). The terms “natural capital” and “ecosystem service” are sometimes interchangeably used. These two are linked, but the most economically distinguishable facet of them is that natural capital is viewed as a stock or an asset, while ecosystem services can be considered as flows that occur from natural capitals (Hoagland et al., 2020).
As cultural ecosystem services, deep-sea research and education develop a more profound understanding and lead to more productive usage and preservation of the deep sea's natural capital and are considered as the non-material benefits people obtain from ecosystems (Hoagland et al., 2020). According to a Bayesian decision framework, Jin et al. (2020) estimated the value of oceanographic research in reducing the uncertainty concerning the estimate of ocean carbon sequestration. The results show that the discounted economic benefit of a putative twenty-year scientific research program could be about $0.5 trillion (USD) (~$25,000 million annually).
Pascual et al. (2011) presented a framework that links ecosystem services to how humans can benefit from them. Accordingly, provisioning services involve direct uses and have the market. Thus, we can calculate their economic value. Regulating services involves indirect uses, and cultural services involve both direct and passive uses; therefore, evaluating their monetary values is more complicated (Hoagland et al., 2020). De Groot et al. (2012) estimated the economic value of the flow of ecosystem services in a range of 490 int$ per year in a hectare of open ocean to almost 350,000 int$/year for the potential services of an “average” hectare of coral reefs. The estimation results for the monetary value for the “open-oceans” biome by Kubiszewski et al. (2017)—~$800/ha in 2019 dollars—is close to the results of De Groot et al. (2012)—$600/ha ± $500/ha in 2019 US dollars.
In a recent report, Ottaviani (2020) has estimated the economic value of the different services provided by the deep sea. The following two tables summarize the main results, using market-based evaluations. Ottaviani (2020) estimated the total economic value of deep-sea ecosystems at USD 267,000 million per year under a scenario in which all analyzed ecosystem services are valued based on traded prices in 2014. This study stated that, from the total economic value of deep-sea ecosystems, 92% arises from abiotic resources (oil and minerals), 11% from seabed minerals, 5% from biotic resources (fish, corals, and pharmaceuticals of marine origin), 2% from cultural services (scientific research and tourism/recreation), and 1% from carbon sequestration. However, under another scenario, in which carbon sequestration was valued by considering the social costs of increased carbon emissions, the overall total economic value of the deep seas is estimated to be nearly double and reached USD 423,000 million per year (Ottaviani, 2020). These estimates are subject to various uncertainties, not least when looking into the future, as there are immature markets for many of those economic services. Therefore, financial risks are high which probably have deterred investments in harnessing many of these resources and services.
According to these estimates, as seen in Table 2, the total economic market value of direct services from the deep sea is almost 258,000 million USD per year. Hoagland et al. (2020) believe that if the fisheries were managed more appropriately, the natural capital of the world's commercial fisheries could be on the order of $1.7 trillion. Sala et al. (2018) estimated high-seas fishing profits ranged between $364 million losses and $1,400 million gains.
Table 2. Estimates for total economic direct services values of the world's deep-sea ecosystems in million USD/year.
These calculations are based on market values which can fluctuate over time.
Considering a “carbon price” of $30 per metric ton of CO2, Hoagland et al. (2020) estimated the economic value of ocean carbon sequestration in a range of $400,000–$1,300,000 million per year. These numbers are notable and undoubtedly increase as the carbon price grows over time. More importantly, for the indirect use values, the average market prices for traded EU ETS in 2014 are used to calculate the economic value of carbon sequestration. As Ottaviani (2020) points out the value of carbon sequestration can also be estimated using a mixed valuation approach where the social cost of increased carbon emissions is used to estimate the value; this increases the value of carbon sequestration by a factor of 49 resulting in 159 074 million USD per year. Using this different methodology places carbon sequestration in second place as the most important economic services of the deep sea, after oil exploitation. From an economic standpoint neither method is more correct than the other, one must just be sure how to interpret the different results. All these estimates are uncertain and are sensitive to changes in assumptions and prices.
How to account for the regulating effect of the deep sea on climate change is challenging. Comparing the valuation of carbon sequestration and other ecosystem services demonstrates that other ecosystem services, such as oil, minerals, seafood, genetic resources, and research and education, can be more valuable. However, the carbon sequestration value is highly dependent on the social cost of carbon used. Using a different valuation method, the carbon sequestration value comes second after oil. This illustrates that the numbers contain considerable uncertainty.
Not included in these assessments are the fact that some of the services described above act to release carbon sequestered in the deep ocean (e.g., oil and gas extraction, seabed mining, bottom trawling, and deep-sea fish harvest), and this release will exacerbate global warming in ways that diminish the regulating (and possibly provisioning) services of the deep ocean.
Irrespective of the methods used for estimating the economic value of the deep sea, it is clear that the services of the deep sea are economically important. Not only are they economically important for specific states, regions or industries, but also as a decisive driver in securing the Earth's capability of fostering life.
Many countries have put forth policies to decrease the dependency on fossil fuels in transport with an increased focus on electrical vehicles. As mineral demand for electrification of vehicles is currently a primary motivation for deep-seabed mining, it is discussed in some detail here. Exploration started on the continental shelf below and beyond 200 nautical miles in states such as Japan, Canada, Papua New Guinea, France, Norway, Russia and the United States. However, no states have so far reached the phase of exploitation, although some of them are close. Papua New Guinea was indeed planning to start exploitation of hydrothermal vent minerals (sulfides) under Project Solwara 1, but the company leading the project, Nautilus (based in Canada), faced important local opposition, legal challenges regarding the management of environmental risks and funding difficulties which lead to its bankruptcy in 2019.
Exploration of minerals in areas beyond national jurisdiction (ABNJ) has taken place since 2001 (International Seabed Authority, 2004). As of May 2022, there were 31 exploration contracts managed by the International Seabed Authority Secretariat (ISA) involving 22 contractors. Out of these 31 contracts, 19 contracts concern polymetallic nodules, seven concern polymetallic sulfides, and five concern cobalt-rich ferromanganese crusts (International Seabed Authority, 2022). Since the signing of these contracts, Brazil and Vanuatu terminated their sponsorships of exploration activities in the Area.2 The ISA, consisting of 167 member states, is now working on a draft Exploitation regulation to enable these future activities to take place. Recent triggering of the “2-year rule” by Nauru has accelerated ISA development of regulations with a July 2023 target date to complete the elaboration of the mineral exploitation regulations (Singh P., 2022). Despite current prospects estimating exploitation to take place in the near future, the exploitation code, and therefore the legal requirements and the thresholds for approving or denying an exploitation plan of work, are not yet set out clearly.
Important uncertainties also remain regarding the economic benefits surrounding the exploitation of these resources and how they will accrue as the common heritage of mankind, particularly to developing states (Jaeckel et al., 2016; Armas-Pfirter, 2023). Higher prices and technical advances in exploration and exploitation could certainly create huge economic potentials in these untapped resources. Notably internalization of environmental costs of exploitation of minerals in the area is only just starting to be studied.3
Furthermore, the payment mechanism applicable for the exploitation of resources beyond national jurisdiction is still being developed by States, either at the national level for exploitation on the continental shelf beyond 200 nautical miles off shore (or UNCLOS, Art. 82), or through the ISA for exploitation in the Area. The financial framework applicable to exploitation activities in the Area raises many thorny questions. Indeed, the Area being the common heritage of mankind, exploitation activities shall thus be carried out for the benefit of mankind as a whole, taking the needs of developing states into particular consideration (UNCLOS, Art 140, para 1). Furthermore, distribution of revenues will occur after coverage of the administrative expenses of the International Seabed Authority Secretatiat in the management of these activities (Feichtner, 2019). So far, the distribution revenue mechanism is still under consideration with important pending issues such as the implementation of the concept of equitable sharing of revenues, the profit-sharing mechanism and the methodology applicable to the calculation of royalty payments. Dingwall (2023) highlights that these questions are central since the nascent deep seabed industry requires financial conditions able to attract investment and the development of the market, while at the same time, achieve meaningful benefit sharing in the long-term due to the implementation of the common heritage of mankind concept.
A different dimension of deep-seabed mining is its relationship with sustainable development (Singh P., 2022), biodiversity, and more specifically blue economy. Mining is increasingly controversial. A 10-year moratorium has been proposed by a growing consortium of Pacific and European states, as it could ultimately be the most disruptive human activity to impact the deep-sea floor directly (Ramirez-Llodra et al., 2011; Amon et al., 2022; Smith et al., 2022). While the International Seabed Authority has engaged in recent years in the promotion of Singh P. A. (2022), especially with regards to gender equality and marine scientific research, important questions remain as to how the deep-seabed mining sector could or could not be considered as contributing to the objectives of Singh P. A. (2022). The difficulty of this assessment is in the interconnectedness of sustainable development goals. Therefore, implementation of some of these goals such as SDG13 (climate change) or SDG5 (Gender Equality) does not demonstrate the sustainability of the sector and its activities, the sustainability lying first and foremost in the global positive outcomes economically, socially and environmentally, across all sustainable development goals.
The oceans are appealing to promote climate change mitigation. However, mitigating climate change through deep-sea blue carbon enhancement suffers from a lack of fundamental knowledge, technological limitations, potential environmental impacts, and a lack of cooperation, collaboration, and verification. There are four major barriers that hamper their role in developing climate change action in the deep sea. This section discusses scientific, economic, governance and political limitations.
Sustainable blue economy, a term widely used nowadays, has been recently defined by the United Nations Environment Programme Finance Initiative (UNEP FI) as “one that provides social and economic benefits for current and future generations; restores, protects and maintain diverse, productive and resilient ecosystems, and is based on clean technologies, renewable energy and circular material flows” (United Nations Environment Programme Finance Initiative, 2022). Taking into consideration the lack of knowledge regarding the marine environment, the paucity of data related to the ecological relationships and impact associated with deep-seabed mining (Amon et al., 2022), the resulting high level of scientific uncertainty and increasing opposition, UNEP FI considers that “there is currently no foreseeable way in which investment into DSM activities can be viewed as consistent with the Sustainable Blue Economy Finance Principles” (United Nations Environment Programme Finance Initiative, 2022).
A key limitation in promoting nature-based solutions for the oceans is our lack of understanding of the interactions of different oceanic eco-subsystems. Interest has grown in exploitation of deep-sea and high-seas areas. For example, fishing in the mesopelagic zone (200–1,000 m) and deep-seabed mining are not yet established as industries but have attracted substantial interest. Fishing in the mesopelagic zone is proposed to add significantly to our global food resources by providing aquaculture feed, while minerals sourced from the deep sea are sought to address shortages that will arise from a quick transition to electrification of individual transportation and digitalization.
All three zones—the epipelagic, the mesopelagic zone and the deep ocean below 1,000 m—interact with each other in ways yet to be fully characterized. Current research estimates that roughly one third of global CO2 emissions since the start of industrialization have been absorbed by the oceans through the biological pump. However, we do not know exactly how anthropogenic activities that interfere with any of the oceanic zones will impact the capacity of this carbon sink. Extracting minerals from the seabed or trawling for fish on the sea floor for instance, could release significant amounts of CO2 that is currently bound in the sediments (Levin et al., 2020; Sala et al., 2021). Similarly, extensive fishing in the mesopelagic zone might limit the amount of carbon that reaches deeper areas of the ocean and remains sequestered from the atmosphere.
The upper ocean mixes on time scales of decades whereas deep-sea water masses are renewed on time scales 10–100 times longer, creating potential for significantly delayed recovery of environmental conditions, even under significant CO2 removal (Heinze et al., 2021). We do not have a full understanding of the factors that influence how much CO2 can be absorbed by the oceans without dramatically slowing the uptake of CO2 (Chikamoto et al., 2023) or even creating ecological tipping points associated with warming, acidification, deoxygenation and changes in circulation (Heinze et al., 2021). There is also a distinct lack of information about natural carbon process rates and the specific roles of different organisms (from microbes to megafauna) in setting these rates. Proposals to strengthen the ocean uptake and storage of carbon from the atmosphere via photosynthesis have involved enhancing phytoplankton production through iron fertilization; and expand open ocean macroalgal culture with algal sinking to the deep seafloor (NASEM, 2022). However, relatively little is known about the efficiency of these processes and about possible negative interactions with other ecological systems (Yoon et al., 2018; Boyd et al., 2022) including those in the deep sea (Levin et al., 2023). Concerns have been raised about unintended side effects on deep-sea ecosystems from changes in light, turbidity, oxygen, pH and physical smothering associated with various technologies (Levin et al., 2023). There is a need to consolidate evidence on climate intervention approaches and to conduct further research to assess ecological and economic efficiency as well as social acceptability and governance modalities, in particular heeding the call by Pörtner et al. (2023) to maximize synergies and minimize trade-offs between climate and biodiversity action.
Any blue carbon conservation effort needs to link the climate-regulating services provided by the oceans to an economic incentive system. As discussed above, the deep sea offers significant economic potential, but at the cost of further deterioration of its fragile ecosystems. A simple preservation of interacting deep-sea ecosystems as a functional unit, therefore, comes with significant opportunity costs, i.e., costs of maintaining and not exploiting its full economic potential. Similarly, to the extent that the capacity of the ocean to absorb CO2 can be enhanced, for instance through macro-algal culture or iron fertilization, this will require substantial investment, the development of new technologies, and extensive science for reporting and verification, all requiring additional funds. For the moment, however, the economic and ecological spheres are not fully integrated, which creates significant frictions in channeling funds to ecological mitigation, preservation and restoration.
One way of strengthening incentives to reduce carbon emission has been through different forms of “green taxes”, specifically carbon taxes that levy taxes on production and consumption depending on their carbon footprint. Such taxes are typically being levied by individual jurisdictions and the revenue thus generated are typically being channeled back into the macro economy, for instance through a reduction in labor taxes. As such, most of these green taxation programs are revenue-neutral and only meant to strengthen incentives for lowering CO2 emissions. These funds are rarely, if ever, being used to support the restoration and maintenance of ecosystems outside national borders and beyond national jurisdiction.
Instead of using taxes, several jurisdictions have started using carbon emission trading systems—so-called “cap-and-trade” systems—whereby a total amount of carbon emissions per year is fixed for a specific industry or a state as a whole and companies need to buy and trade emission rights via auctions. Such a system is deemed more flexible and can react to individual peak emissions through the trading system in order to adjust the true cost of carbon in a dynamic manner. But similar to a system based on taxes, the revenues generated by this system are entering general government budgets where they can be earmarked for efforts to reduce the national footprint.
One way by which governments have tried to achieve some form of global collaboration in order to promote blue carbon sinks is through debt-for-nature swaps. In this case, selected highly indebted states see their debt burden decline in exchange for commitments to restore or protect local ecosystems. Such a mechanism could be used, for instance, to support maritime nations in their effort to maintain healthy ocean conditions within their EEZs. Such a mechanism is, however, limited to states that have a large external debt burden with creditors that are willing to forfeit part of their principal in exchange for maintaining local ecosystem services. Often, this might not be possible as different creditors cannot be aligned or sovereign debt is owed to multilateral organizations that are not ready to engage in such a swap. For island states, the deep sea is typically a large part of their EEZ and protection of deep-sea ecosystem services may be possible through debt-for-nature swap, as was done by Seychelles (Silver and Campbell, 2018). However, such mechanisms are not available for deep international waters that are regulated by international treaty bodies (see below).
An alternative to such bilateral interventions and with more potential for scale is to use part of the funds levied by carbon taxes and credits and channel them into dedicated funds, such as the Social Climate Fund proposed by the European Commission.4 Properly resourced, such a fund can support various global and local initiatives to complement the national or supra-national efforts to reduce carbon emissions through specific interventions enhancing carbon sinks. However, even in the case where such funds dispose of a significantly enlarged budget, the policy goals between reduced carbon emissions and enhanced carbon sinks might not be aligned. In the case of protecting the oceans, for instance, rising costs for maritime transport among those states that levy carbon taxes might displace such transport to more lenient states, with adverse consequences not only for carbon emissions but also for carbon sinks. A coordinated effort is, therefore, necessary to prevent such evasion strategies and support a successful transition to net zero in which oceans play an important role.
A final challenge in setting up financial mechanisms to support carbon sinks, especially in the oceans, is the lack of a proper valuation mechanism. Several attempts have been made to expand national accounts to integrate social and environmental concerns (such as the system of environmental economic accounting) but so far, no agreed standard exists. Recently, Chami et al. (2019) have proposed a new framework to assess the financial value of ecological services around carbon sinks, using available carbon trading prices as benchmarks.5 These estimates yield significant value even for individual species let alone entire ecosystems. However, considering the difficulty of an ecological assessment of the deep sea and the incomplete science around this topic, the financial valuation thus established can be at best a lower estimate of the true value of the oceans.
Besides incomplete knowledge, competing interests concerning the ocean's value and lack of proper economic incentive mechanisms, it is also the fragmented institutional structure that prevents the regulation and implementation of deep-ocean carbon protection. The proliferation of different governance mechanisms, replying to different needs at the regional or sectoral levels, also results in different political and economic interests as discussed above, preventing consistency.
Monitoring climate interventions and climate abuses in the vast ocean is a challenge in itself. Today's technological advancements offer some additional ways of enhancing autonomous surveillance but would still require substantial effort and investment to carry it out at scale, as well as stronger states' cooperation to implement effectively existing rules. Alternative approaches consist in setting up regulation and monitoring at specific neuralgic points, for instance by installing buoys around port areas to prevent whale ship strikes.
Concerning the governance of the deep ocean, there is a distinction between the areas where coastal states enjoy sovereign rights over their natural resources and the part of the ocean beyond that where all states have rights. Yet the living natural resources in the high seas, including deep-sea fisheries, will become property of the person who catches it (Grotius, 1916). Particularly this characteristic makes the high seas potentially subject to the tragedy of commons that comes from open access (Hardin, 1968). Regarding fisheries, this translates into a lack of incentive for individual fishers to reduce their catch at a certain moment with the objective to catch more later on. Most fishers will not hesitate to take the risk that the fish stock might not recover when everyone lacks this incentive. Long-term objectives are thus often non-existent in the fisheries industry without governmental regulation (Hannesson, 2004).6 The international community has tried to increasingly curb the freedom of fishing on the high seas (Takei, 2013; Harrison, 2017).
Almost half a century ago, the International Court of Justice already remarked “that the former laissez-faire treatment of the living resources of the sea in the high seas has been replaced by a recognition of a duty to have due regard to the rights of other States and the needs of conservation for the benefit of all.”7 Duties to conserve marine living resources and to co-operate between not only flag states but also with coastal states emerged and found their ways into legally binding agreements.8 States that fish for identical stocks or different ones but in the same area are obliged to co-operate in regional or subregional fisheries organizations.9 Flag state duties incorporate, among other things, the establishment of a national vessel record and compliance with regulation of licenses and international guidelines for markings, monitoring, control and surveillance.10 The national rules of the flag states should of course comply with the measures of regional fisheries management organizations.11 Thus, cooperation in a regional fisheries management organization might be the best way to fulfill the duty of cooperation (Borg, 2012).
Contrary to fisheries management, the governance regime for the mining of minerals from the ocean floor beyond the continental shelf is much more centralized (Jaeckel, 2017). States that want to engage in the exploration or exploitation of these minerals have to apply for a contract from the International Seabed Authority.12 The Authority is also responsible for the compliance with the regulations for exploration and exploitation, as well as the protection of the marine environment.13
The UNFCCC itself has limited control over ABNJ. State climate mitigation and adaptation actions as reflected in their Nationally Determined Contributions (NDCs) and National Adaptation Plans focus on their EEZs. There is no NDC for the ocean beyond national jurisdiction. Although shipping emissions are regulated by the International Maritime Organization, climate impacts of other activities in ABNJ would go unregulated. They could be subsumed under a “cumulative” EIA, however. The latest draft of the agreement under the UNCLOS on the conservation and sustainable use of marine biological diversity of ABNJ (BBNJ Agreement) of March 4th, 2023 defines cumulative impacts as “combined and incremental impacts resulting from different activities, including known past and present and reasonably foreseeable activities, or from the repetition of similar activities over time, and the consequences of climate change, ocean acidification and related impacts” [Art. 1(8)]. Cumulative impacts should be considered throughout the process of conducting an EIA in ABNJ [Artt. 24(1)(a)(ii) & (2)(e), 30(1)(b) & (c) & 35(2)]. This is one of the few occasions where the draft explicitly mentions the climate. There is scope within the BBNJ Agreement for the designation of protected areas in ABNJ that target biodiversity and habitats that are vulnerable to climate change and ocean acidification, as well as those valued for their carbon sequestration services, although this latter point is not listed among indicative criteria for identification of areas in Annex I of the Agreement. Capacity development and technology transfer elements in the Agreement also could address climate-biodiversity connections.
Conflicting economic interests also clash with political differences; benefactors and victims of the deep-sea economy are likely not the same. Most high-income states promote the preservation of the ocean's capacity to absorb CO2 emissions. At the same time, they continue to pursue economic exploitation of the deep sea. The deep sea represents substantial economic opportunities and significant ecosystem services that could be lost. Small-island states whose livelihoods are often highly intertwined with the ocean may not have the financial capacity to exploit the deep sea, but would bear the consequences of lost ecosystem services. These states also face considerable consequences from climate change in the form of rising sea water levels, flooding, intensified cyclones, and redistribution of lucrative fisheries (e.g., tuna) to outside their EEZs.
Sometimes, cultural barriers might preclude certain forms of use of the oceans. For instance, coastal populations might rely on fishing as their traditional economic activity. Massive macroalgae cultivation, as discussed in the previous section, would involve a significant change in the economic and cultural practices of such populations, which might be deemed unacceptable.
Several high-income nations have expressed interest or are already exploiting deep-sea resources. There is also a danger that it is considered a setting of marginal importance, suitable for waste and CO2 disposal. Finding and pursuing deep-sea solutions that engage low-income and middle-income nations, incorporate traditional knowledge, and are just and equitable in their outcomes remains a considerable challenge.
Climate change is among many contemporary societal challenges. Nature-based solutions (NBS) have conceptually been defined in a variety of ways that include the utilization of nature, or the inspiration of nature, to address contemporary societal challenges from a sustainability perspective. For example, Cohen-Shacham et al. (2016) defines NBS as “actions to protect, sustainably manage, and restore natural or modified ecosystems, that address societal challenges effectively and adaptively, simultaneously providing human wellbeing and biodiversity benefits”. This definition emphasizes the positive exploitation of ecosystem services from well-managed or restored ecosystems as key to the solutions. The European Commission (2020) offers a slightly broader definition of NBS: “Solutions that are inspired and supported by nature, which are cost-effective, simultaneously provide environmental, social and economic benefits and help build resilience. Such solutions bring more, and more diverse, nature and natural features and processes into cities, landscapes and seascapes, through locally adapted, resource-efficient and systemic interventions”.
There are significant differences between blue carbon and NBS. First, NBS are broader than blue carbon in that they encompass non-climate benefits and biodiversity in addition to climate. Regardless of the differences in emphases in the definitions, NBS is generally understood to reference benefits to biodiversity while simultaneously delivering beneficial ecosystem services to support the achievement of societies' objectives and to tackle societal challenges. The baseline is that climate change mitigation is only one of the benefits that can be derived from the deep sea. Second, NBS have direct implications and benefits for stakeholders and direct resource users. In contrast, the benefits of blue carbon manifest at the global scale and the difference any single intervention or conservation project will make is necessarily marginal. This can constrain motivation and dilute economic incentives. Understanding, valuing and protecting the deep sea for more than its carbon sink and sequestration capacity will help realize opportunities from various benefits, manage trade-offs, and mitigate risks and unintended consequences. Consequently, deep-sea NBS governance will have a significantly different trajectory compared to deep-sea blue carbon governance.
Yet, NBS have significant relevance in the adaptation and mitigation to climate change (Kabisch et al., 2016; Seddon et al., 2021). This is because, as stated by Seddon et al. (2020), “There is growing awareness that nature-based solutions can help to protect us from climate change impacts while slowing further warming, supporting biodiversity and securing ecosystem services”. The use of NBS in climate change, in whole or in part as adaptation and mitigation strategies, has received much contemporary attention. Among other things, climate change-related NBS have been discussed in relation to urban areas (Kabisch et al., 2016; Frantzeskaki et al., 2019; Seddon et al., 2020; Bayulken et al., 2021), land management (Keesstra et al., 2018), coastal (Cohen-Shacham et al., 2016; Nguyen, 2018; Chausson et al., 2020) and marine areas (Gattuso et al., 2018; Fullam et al., 2021).
Climate change-related NBS research and reviews linking to blue carbon include those by Macreadie et al. (2021), Quevedo et al. (2021), and Wedding et al. (2021). Discussions have referred to, among other things, blue carbon sequestration via (i) ecosystems such as seagrass beds (Stankovic et al., 2021), mangroves (Friess et al., 2020), seaweed aquaculture (Duarte et al., 2017; Kuwae and Crooks, 2021; Yong et al., 2022) and kelp forests (Wernberg and Filbee-Dexter, 2018; Seddon et al., 2021); (ii) ocean fauna such as whales and fish etc. (Mariani et al., 2020); and (iii) bright spots where climate mitigation and fisheries opportunities overlap (Queirós et al., 2021).
Ocean zones such as the deep seabed and the high seas have also been the subject of climate change-related NBS strategies (Wernberg and Filbee-Dexter, 2018; Chen et al., 2022). However, knowledge of this environment, both within and outside national jurisdiction, is currently limited (Ramirez-Llodra et al., 2011; Danovaro et al., 2017, 2020) and therefore, its use in NBS requires more rigorous and more extensive scientific research. Furthermore, some researchers have arrived at the conclusion that NBS themselves have limits in efficacy and contributions to climate change (Kabisch et al., 2016; Seddon et al., 2020; Kumar et al., 2021; Williamson et al., 2021). Notably, in both shallow and deep waters, microbes and microbial processes have yet to emerge as a focus of blue carbon initiatives, despite the fact that they play critical roles in the carbon cycle (Orcutt et al., 2020).
Does existing deep-sea governance provide opportunities to manage climate mitigation in addition to ecosystem services and biodiversity? Several international treaties govern the deep sea in ABNJ. These include the UNCLOS Part XI, the United Nations Fish Stock Agreement of the UNCLOS, Convention on Biological Diversity (CBD), UNFCCC, Convention on International Trade in Endangered Species of Wild Fauna and Flora (CITES), the Convention on the Conservation of Migratory Species of Wild Animals (CMS), the International Whaling Convention (IWC) and the London Convention/London Protocol (LC/LP). Most recent is the newly agreed upon high seas treaty governing biodiversity conservation and sustainable use in ABNJ. Relatively few deep-sea species are protected by the species-specific treaties such as the CMS, IWC and CITES, although cold-water corals and deep-diving whales are notable exceptions. In addition, development and technological plans are advanced to protect the deep sea. While many of these treaties refer to climate change, they rarely focus on blue carbon and the possibility of mitigating climate change while protecting biodiversity (Elsler et al., 2022).
EIAs are required for various deep-sea activities (such as for mining, oil and gas exploration and exploitation, new deep-sea fisheries). Ratification of the high seas treaty may see additional activities subject to EIA including deep-sea cables, scientific sampling, and genetic resource extraction. The 2021 Draft Standards and Guidelines for EIA Process of the International Seabed Authority do not include climate change, but the newest draft for the regulations on exploitation deal with emissions (Heinrich et al., 2020). The agreed upon draft of the high seas treaty remedies some of the gaps by including a broad “cumulative impact” category.
While climate change and greenhouse gas emissions are included in certain EIAs, carbon cycle disturbance, carbon release or inhibition of carbon sequestration are rarely taken into consideration. Human activities disturbing the seafloor and its biota may interfere with carbon sequestration or release carbon (e.g., Luisetti et al., 2019). Removal of fish and whale standing stock through harvest also reduces carbon sequestration (Mariani et al., 2020). Bottom trawling, seabed mining, oil spills or drilling anchors and infrastructure, such as offshore platforms, can create physical and chemical disturbance or pollution that can affect biodiversity and alter carbon cycling. The impact of infrastructure, installations and artificial islands on the marine environment, for the conduct of seabed activities, is generally overlooked in EIAs and the legal frameworks applicable to them, both on the continental shelf and in the EEZ, the high seas and the Area, still poses important implementation issues, especially when it comes to the protection of the marine environment (Gautier and Tassin, 2013; Schneider, 2018). Bravo et al. (2023) have suggested inclusion of detection and monitoring of ecosystem services as needed for impact assessment and management in the deep ocean.
Marine spatial planning and the design of MPAs have the primary focus of protection and rebuilding of biomass and biodiversity, often with positive side effects for fisheries. MPAs can function as bank capital “from which we will earn interest every year in the form of a harvestable catch” (Greenberg, 2010; Wood, 2014). MPAs are starting to consider carbon consequences and carbon conservation, but this is rare in the open ocean or deep sea (Jacquemont et al., 2022). Introduction of climate change in the regulation of fisheries appears to be also in its early stages (Oostdijk et al., 2022); the IWC has recognized the role of whales for carbon sequestration. Although not primarily declared for that purpose, the establishment of marine protected areas—not in the least for the protection of ecosystems that are able to sequester carbon—remains an additional tool available through the High Seas Treaty to contribute to the mitigation of climate change (Jakobsen, 2021). The objective of the treaty to protect, preserve, restore and maintain biodiversity and ecosystems is, among other things, meant to strengthen resilience to stressors such as climate change [Art. 7 (h) and Art. 17(c)]. One of the general approaches of the agreement is to build “ecosystems resilience, including to adverse effects of climate change, ocean deoxygenation and ocean acidification”, and also to maintain and restore “ecosystem integrity, including the carbon cycling services that underpin the ocean's role in climate” [Art. 7(h)]. In addition to classic MPAs, areas of particular importance are designated by some UN bodies with jurisdiction over the deep ocean (see Box 1). Although not a focus at present, these could incorporate protection of carbon services. Examples include the Ecologically or Biologically Significant Marine Areas (EBSAs) designated through the CBD and no-mining Areas of Particular Environmental Importance designated by the International Seabed Authority. The same is true for World Heritage Sites (Box 1), some of which include the deep sea.
Box 1. Biodiversity governance, world heritage, and deep-sea vents.
The rarity and great biological fragility of deep-sea vents, often described as deep-sea oases, led them to be recognized as “vulnerable ecosystems”, which is a category enabling specific management protection measures due to environmental concerns. The most significant recognition came first with the United Nations General Assembly in 2004, which called states to manage the biodiversity risks of these vents and consider the prohibition of destructive fishing practices around them. States have also acknowledged their fragility through the Conference of the Parties to the Convention on Biological Diversity highlighting, also in 2004 (Conference of the Parties, 2004), the urgent need for international cooperation and action to improve the conservation and sustainable use of biodiversity in ABNJ, including through the establishment of marine protected areas around these vents (Conference of the Parties, 2004). A few years later, in 2008, the same Conference of Parties (Conference of the Parties, 2008) recognized that ecologically and biologically significant areas requiring enhanced conservation and management measures could be established for the protection of these vents. The Food and Agricultural Organization (FAO) also recognized hydrothermal vents as an example of potential vulnerable habitat when adopting, in 2009, the International Guidelines for the Management of deep-sea fisheries in the High Seas (Food Agriculture Organization, 2009). Considering the concerns of the international community toward these ecosystems, the International Seabed Authority recognized these ecosystems as vulnerable (International Seabed Authority, 2011). The International Seabed Authority is currently working, since 2018, on expanding regional management plans for hydrothermal vent systems in the Mid-Atlantic, including an area known as the “Lost City” (Johnson, 2019), and the Indian Ocean. Notably, Lost City falls within the Poland exploration contract issued by the International Seabed Authority for massive sulfides.
At the international level, cultural and natural heritage are identified and protected through the 1972 UNESCO World Heritage Convention, which is aiming to avoid “deterioration or disappearance of any item of the cultural or natural heritage [which] constitutes a harmful impoverishment of the heritage of all the nations of the world” (UNESCO World Heritage, Preamble). Since the first incorporation of marine sites into the UN World Heritage site in 1981, 49 marine sites around the world have been added to this list, allowing greater protection and marine scientific research to be deployed, including in deep-sea areas within national jurisdiction. But at the time of writing this article, none of these world heritage sites are covering deep-sea ecosystems beyond national jurisdiction.
UNCLOS does not explicitly deal with climate change nor does it make any reference to climate change in the original treaty since it was negotiated in the 1970s. Since the ocean plays nonetheless an important role in both mitigation of, as well as adaptation to, climate change, the High Seas Treaty has included direct references to climate change thereby integrating it into the UNCLOS. While waiting for the signing and entry into force of this new agreement, it is worth noting that the emission of greenhouse gases would qualify as pollution of the marine environment under UNCLOS. The LC/LP regulate ocean dumping and apply to some geoengineered solutions for enhancing carbon storage in the seabed, although iron fertilization has been addressed (in new Annex 4 of the 2013 LP amendment). Reading this together with the 2015 Paris Agreement emphasizes the aim of greenhouse gas emission reduction rather than enhanced uptake of carbon by the ocean (Boyle, 2021).
Carbon accounting frequently refers to collecting, summarizing, and measuring carbon emissions data to enable emissions comparisons and facilitate independent reviews for compliance and data accuracy (Tang and Luo, 2014). Carbon accounting under the UNFCCC has been enabled in wetlands through the “Supplement to the 2006 IPCC Guidelines for National Greenhouse Gas Inventories: Wetlands” (IPCC, 2013). Equivalent guidelines for the deep sea do not exist. Measurability and attribution are major technical and legal concerns regarding carbon accounting under the UNFCCC (Oostdijk, 2021). Carbon emission reductions through the enhancement of the biological carbon pump, for instance, are notoriously difficult to measure. Any efforts by a single state would be difficult to attribute due to currents and movement of marine life. In addition, carbon accounting has a limited scope on climate-regulating services and disregards biodiversity and other crucial ecosystem services.
Within the UNFCCC Subsidiary Body for Scientific and Technical Advice there is now mandated an annual Ocean and Climate Change Dialogue focused on building ocean action into UNFCCC mitigation and adaptation activities. State and non-party submission to the first these dialogues in 2020 highlighted changing ocean impacts, carbon sinks, blue carbon opportunities, and the need for ecosystem resilience and biodiversity management (Dobush et al., 2021). While the conceptual joining of climate and biodiversity is evident, there was limited discussion of the deep ocean in the Dialogues in 2020 and 2022. The UNFCCC Ocean Dialogues offers a novel opportunity for ocean stakeholders to come together to discuss how to incorporate the ocean into climate negotiations—via NDCs, National Adaptation Plans and to the Nairobi, Marrakesh and other relevant UNFCCC work programs. Within these, there are many means to highlight protection of deep-ocean ecosystem services to enhance climate resilience. While there were several deep-ocean focused pavilion and side events at COP 27, they had limited policy impact due to the sheer size and separation of negotiators from observers. Because the COP 27 text now mandates that the Dialogue is reported to the annual UNFCCC COP, the Ocean Dialogue could become a more effective tool for advancing the conservation of deep-ocean ecosystem services as a holistic nature-based solution.
Most regional fisheries management organizations have not instigated direct action concerning climate change mitigation (Rayfuse, 2019; Molenaar, 2021), although several FAO technical reports have considered the issue for both coastal (Food Agriculture Organization et al., 2018) and deep-sea fisheries (Food Agriculture Organization, 2019). Just recently the South Pacific Regional Fisheries Management Organization adopted a decision making climate change a permanent agenda item for the scientific committee, compliance and technical committee and Commission in order to include climate change in Commission decisions. It is possible to use climate model projections and life-history data to assess species-specific vulnerability to climate changes in the deep sea in conjunction with fishing vulnerability and to manage accordingly (Cheung et al., 2022). However, ecosystem-based approaches to manage fisheries could also encompass carbon sequestration (Krabbe et al., 2022). The ecosystem approach aims at the shift from management of particular species to management of the ecological system as a whole (Charles, 2013; Takei, 2013; Marauhn and Böhringer, 2014; De Lucia, 2019). Protection of vulnerable marine ecosystems (VMEs) by RFMOs based on the presence of indicator taxa such as cold-water corals is an example of ecosystem-based practice that simultaneously protects the carbon stored in these systems. The law on straddling and highly migratory fish stocks contains details on the protection of dependent species and species within the same ecological system.14 Humans, however, are undeniably part of the ecological system as well. Thus, any ecosystem approach merely tries to manage humans' impact on the ecological system (Murawski, 2007). In this regard, states have to consider that the same fish stocks live under both national jurisdiction and the ocean beyond. Compatibility of measures should be a goal.15 Orrego Vicuña (1999) suggests that the ecosystem approach was the main driver behind the rules on compatibility between the exclusive economic zones and the high seas. A holistic approach to the ecological system not only emphasizes the interactions between species but between the legal regimes for the ocean within and beyond national jurisdiction (Orrego Vicuña, 1999).16
Prohibition of subsidies or other support for the fishing industry from the government appears to be a prerequisite for a well-working fisheries management system (Stone, 1999; Sumaila et al., 2021). State support distorts free competition among participants in the fishery. Nonetheless, a subsidy can function as a fee for a service that the fisher delivers to society if the fisher is seen as the steward of the fish stock. Crucial is then that fishers lose their right to fish when they fail to fulfill this duty (Greenberg, 2010). This also works on the level of regional fisheries management organizations, as trustees of the fish stock—the trust (Rayfuse and Warner, 2008). Stewardship is then “the ethical companion to the scientific practice of restoration” (Rayfuse and Warner, 2008; Telesetsky et al., 2017; Barritt, 2019). A precautionary approach to fisheries entails that any uncertainty about the value of an ecosystem should not be an argument against the protection of it. In fisheries management, one system that tries to incorporate this is that of individual transferable quota (ITQ) (Nomura, 2014). Participants in the fishery can buy, sell and lease ITQs, which leads to the fact that they often come in the possession of the highest bidder. That, in turn, is likely the person that will make use of the ITQ in the most effective way. Moreover, this system might reduce overcapacity when more efficient ITQ holders buy out the less efficient ones (Hannesson, 2004). In sum, the governance of the deep sea provides opportunities to mitigate climate change, but the highly sectoral nature of international agreements has limited the embrace of climate in many conventions outside the UNFCCC (Elsler et al., 2022). A key to protection of deep ocean ecosystem services will be capacity development in the arenas of deep-sea scientific research and monitoring, representation of youth, the poor, indigenous voices and a voice for wildlife, widespread deep-ocean literacy, and more integrative governance of deep water across sectors.
Possible future governance opportunities of the deep sea might also be found in financial markets. Non-fungible tokens are burgeoning in nature conservation and are an instrument that might be applied to deep-sea blue carbon. Presumably, area-based tokens could occur only within continental shelves given the commons and common heritage aspects of international waters described above.
It is very difficult to appraise or give value to many of the environmental services that the deep sea provides to us. A part of the problem lies in the fact that many environmental resources, such as those provided by the deep sea, are non-fungible assets meaning that they are globally unique and therefore not easily exchangeable in the marketplace. This makes it very difficult to estimate their prices, let alone their real values. Technological innovations such as issuing blockchain- based tokens assigned to different deep-sea environmental services might provide solutions to some of the problems related to their non-fungibility. Such tokens, often referred to as NFTs, are already being issued to tackle environmental issues, mostly conservation but these also allow for trade in non-fungible services (Far et al., 2022).
Examples of NFTs encompass biodiversity conservation and climate-change mitigation projects (Valeri and Baggio, 2021). For instance, Rewilder (www.rwilder.xyz) is experimenting with issuing tokens that match donations for land purchases for conservation. Each donor receives a non-fungible token that matches their donation and the holder of that token receives regular updates concerning the land they helped to purchase. The fact that the tokens are non-fungible assures that donors can attribute their donation to a specific parcel of land and can easily verify the state and condition of this specific piece of land. In addition, OceanDrop (www.oceandrop.art) is a charitable NFT art auction, hosted by the Opean Earth Foundation (www.openearth.org) which raised funds for marine conservation. NFTs are used to certify the uniqueness and authencity of digital artworks that are auctioned to raise money for marine conservation. Finally, Orb (www.orb.green) issues verified carbon credits in the form of NFTs, allowing for the validation, measurement and verification of carbon afforestation projects. Orb's project provides transperancy for afforestation projects, allows companies to buy carbon credits and individuals to support afforestation while at the same time uses satellite imagery and other high-tech methods to facilitate planning and registration of afforestation projects, estimate carbon sequestration and enable verifiable registration and trading of carbon credits.
These examples indicate that non-fungible tokens and related technologies can potentially provide opportunities for strengthening deep-sea conservation. They have also been applied in blue carbon conservation. Transferring these examples to the deep sea will heavily depend on the verifiability and transparency of NFTs and related technologies.
The fulfillment of the SDGs, the Paris Agreement, and the post-2020 Biodiversity Goals rely in part on the deep sea. Blue carbon has emerged as a popular means to include the ocean in the climate negotiations (Dobush et al., 2021). Blue carbon has been a potent driver of protection and rebuilding efforts in coastal areas where it is considered a no-risk action (Gallo et al., 2017; Gattuso et al., 2018). The international community increasingly recognizes that open-ocean and deep-sea processes were highly significant in buffering climate change from anthropogenic emissions in the past decades. Yet, in the deep sea, a similar trajectory for blue carbon and effective mitigation of climate change hinges on lack of scientific knowledge and verification, negative feedbacks limiting carbon uptake (i.e., no long-term solution), financial limitations, a lack of cooperation and implementation. The deep sea should be viewed as a more holistic nature-based solution to achieve international policy objectives. A suite of management instruments is available that could enable governance alignment of ecosystem services and biodiversity in addition to climate mitigation.
Carbon fluxes and storage in the deep ocean are substantial, yet they are changing fast. In previous decades, these mechanisms played a key role in mitigating climate change caused by anthropogenic emissions. Ocean acidification is caused by increasing carbon absorption in the ocean and has complicated interactions with marine life, which can alter biophysical processes connected with carbon fluxes and storage. Other climate change and anthropogenic impacts are projected to reduce overall future deep-sea carbon fluxes and, with them, the potential to mitigate climate change. The long legacy of climate impacts and likely slow recovery of the deep sea under climate mitigation may compromise the significant ecosystem services and vast—yet unknown—biodiversity of the deep ocean. Regulating services such as biogeochemical cycling, heat and carbon storage will decrease as anthropogenic pressures increase. During the coming decades, provisioning services are predicted to grow with novel and expanding deep-sea industries, including from fisheries, pharmaceuticals, blue energy and potentially minerals. Cultural services for education and research are likely to grow, but spiritual connections to deep water remains underexplored.
The value citizens place on different ecosystem services is vital to navigating trade-offs in decision-making. The social acceptability might be low for methods used in the economic valuation of non-tradeable ecosystem services. The economic value varies with the chosen valuation approach and does not reflect public care and concern. A first valuation shows that the value of carbon sequestration is below that of other ecosystem services, such as oil, minerals, fisheries, and research and education. The value of carbon sequestration, on the other hand, is strongly reliant on the social cost of carbon consumed. Using a different valuation approach, carbon sequestration comes in second place behind oil. This demonstrates the variability and uncertainty of the figures. While for our purposes, valuation is sufficient, a promising alternative approach for the deep sea is assessing care and concern. Recent research on care found that people's care for the deep sea is linked to the emotions, moods, and meanings that this environment evokes in them. Deep-sea literacy underpins the extent of caring. People generally care little about the deep sea, but more than 80% were highly and very highly concerned about deep-sea mining (Kaikkonen and van Putten, 2021).
The deep sea can be viewed as a more holistic NBS, including many ecosystem services and biodiversity in addition to climate. The valuation highlighted the importance of a variety of ecosystem services beyond climate. Recent advances in international discussions on terrestrial ecosystems, exhibit similar thoughts. The “Not Just Carbon” report from the World Resources Institute highlights the need for holistic consideration of benefits from forests (Seymour et al., 2022). First, understanding, valuing, and protecting the deep sea for more than its carbon sink and sequestration capacity will help realize opportunities from various benefits, manage trade-offs, and mitigate risks and unintended consequences. Second, NBS highlight direct benefits to users and stakeholders of the deep sea in addition to global climate benefits. These benefits include public care and concern and strong industrial interests, which have been traditionally difficult to balance in international negotiations. Consequently, deep-sea NBS governance will have a significantly different trajectory compared to deep-sea blue carbon governance.
The governance of the deep sea provides opportunities to mitigate climate change but is much richer in its history to manage impacts such as mining and other important ecosystem services such as provisioning services. Climate-regulating services could theoretically be included in treaties under development or as amendments, but these developments are still in the future.
NH wrote the first structure, started the draft, and lead the paper with LL's help. MS helped to restructure the text and added his inputs all along the text. SF and GH drafted the sections about economics. EE drafted the section about NFTs. ED and AC wrote about law and governance. MW and LL wrote the sections about fisheries. LL wrote the sections about science. All authors contributed to the article and approved the submitted version.
This paper has been drafted after the workshop organized by the Centre Scientifique de Monaco with the funding of the Principality of Monaco and the Foundation Prince Albert II of Monaco. MW and LE were funded by the Horizon 2020 project MEESO Ecologically and Economically Sustainable Mesopelagic Fisheries (2019-2023), grant agreement No. 817669. LL's efforts were supported by NSF awards OCE 2048720 and the NSF AccelNet Program award #2114717 via University of Texas subaward 308056-0001A.
The authors thank the reviewers JG and RB for their thoughtful suggestions. The authors thank Virginie Tassin Campanella for her comments on legal aspects.
GH was employed by Intellecon. EE was employed by ILO. AC was employed by Direction de l'environnement.
The remaining authors declare that the research was conducted in the absence of any commercial or financial relationships that could be construed as a potential conflict of interest.
All claims expressed in this article are solely those of the authors and do not necessarily represent those of their affiliated organizations, or those of the publisher, the editors and the reviewers. Any product that may be evaluated in this article, or claim that may be made by its manufacturer, is not guaranteed or endorsed by the publisher.
1. ^Dancers From the Deep Sea Shine on the UN for Climate Week, New York Times, September 2021 https://www.nytimes.com/2021/09/14/arts/design/video-united-nations-climate-week.html.
2. ^Website of ISA: https://www.isa.org.jm/exploration-contracts (accessed May 18, 2022).
3. ^Website of ISA: https://www.isa.org.jm/news/call-proposals-consultancy-services-undertake-study-internalization-environmental-costs (accessed January 1, 2023).
4. ^https://ec.europa.eu/clima/eu-action/european-green-deal/delivering-european-green-deal/social-climate-fund_en
5. ^https://www.researchgate.net/publication/344251550_On_Valuing_Nature-Based_Solutions_to_Climate_Change_A_Framework_with_Application_to_Elephants_and_Whales_On_Valuing_Nature-Based_Solutions_to_Climate_Change_A_Framework_with_Application_to_Elephants_a
6. ^Compare this with Grotius (1916): “all that which has been constituted by nature [...] ought in perpetuity to remain in the same condition as when it was first created by nature.”
7. ^International Court of Justice, Fisheries Jurisdiction (United Kingdom of Great Britain and Northern Ireland v. Iceland), Merits, Judgment, 25 July 1974, ICJ Reports 1974, 3, para. 72.
8. ^United Nations Convention on the Law of the Sea 21 International Legal Materials 1261 (1982) Artt. 116–119.
9. ^United Nations Convention on the Law of the Sea 21 International Legal Materials 1261 (1982) Art. 118.
10. ^Agreement for the Implementation of the Provisions of the United Nations Convention on the Law of the Sea Relating to the Conservation and Management of Straddling Fish Stocks and Highly Migratory Fish Stocks 34 International Legal Materials 1542 (1995) Art. 18(3).
11. ^Agreement for the Implementation of the Provisions of the United Nations Convention on the Law of the Sea Relating to the Conservation and Management of Straddling Fish Stocks and Highly Migratory Fish Stocks 34 International Legal Materials 1542 (1995) Art. 18(1)/(4).
12. ^United Nations Convention on the Law of the Sea 21 International Legal Materials 1261 (1982) Art. 157.
13. ^United Nations Convention on the Law of the Sea 21 International Legal Materials 1261 (1982) Artt. 139(1), 153(4) & (1).
14. ^Agreement for the Implementation of the Provisions of the United Nations Convention on the Law of the Sea Relating to the Conservation and Management of Straddling Fish Stocks and Highly Migratory Fish Stocks 34 International Legal Materials 1542 (1995) Art. 5(d)-(g).
15. ^Agreement for the Implementation of the Provisions of the United Nations Convention on the Law of the Sea Relating to the Conservation and Management of Straddling Fish Stocks and Highly Migratory Fish Stocks 34 International Legal Materials 1542 (1995) Art. 7.
16. ^Orrego Vicuña (1999): What the Fish Stocks Agreement essentially seeks to achieve is '[o]ne area of biological unity and distribution of stocks, subject to different jurisdictional regimes conceived in a supplementary manner as to fisheries conservation and management'; ibid., 183.
Aanesen, M., Armstrong, C., Czajkowski, M., Falk-Petersen, J., Hanley, N., and Navrud, S. (2015). Willingness to pay for unfamiliar public goods: preserving cold-water coral in Norway. Ecol. Econ. 112, 53–67. doi: 10.1016/j.ecolecon.2015.02.007
Agusti, S., González-Gordillo, J., Vaqué, D., Strada, M., Cerezo, M. I., Salazar, G., et al. (2015). Ubiquitous healthy diatoms in the deep sea confirm deep carbon injection by the biological pump. Nat. Commun. 6, 7608. doi: 10.1038/ncomms8608
Amon, D. J., Gollner, S., Morato, T., Smith, C. R., Chen, C., Christiansen, S., et al. (2022). Assessment of scientific gaps related to the effective environmental management of deep-seabed mining. Marine Policy. 138, 105006. doi: 10.1016/j.marpol.2022.105006
Armas-Pfirter, F. M. (2023). “Chapter 3 the “common heritage of mankind” principle and the equitable sharing of benefits,” in The United Nations Convention on the Law of the Sea, Part XI Regime and the International Seabed Authority: A Twenty-Five Year Journey, eds A. Ascencio-Herrera and M. H. Nordquist (Brill Nijhoff), 85–99.
Armstrong, C., Foley, N., Tinch, R., and van den Hove, S. (2010). Ecosystem Goods and Services of the Deep Sea. Deliverable D6.2: hotspot ecosystem research and man's impact on European seas. (HERMIONE), Universititet i Tromsø. Available online at: http://median-sustainability.com/IMG/pdf/ecosystem_goods_and_services.pdf
Armstrong, C. W., Foley, N. S., Tinch, R., and van den Hove, S. (2012). Services from the deep: Steps towards valuation of deep sea goods and services. Ecosyst. Serv. 2, 2–13. doi: 10.1016/j.ecoser.2012.07.001
Ash, S. (2014)., ‘The Development of Submarine Cables,” in Submarine Cables: The Handbook of Law and Policy, eds D. R., Burnett, R. C., and Beckman, and T. M., Davenport (Leiden), 19–39.
Atwood, T. B., Witt, A., Mayorga, J., Hammill, E., and Sala, E. (2020). Global patterns in marine sediment carbon stocks. Front. Marine Sci. 7, 165. doi: 10.3389/fmars.2020.00165
Atwood, T. B., Witt, A., Mayorga, J., Hammill, E., and Sala, E. (2021). Corrigendum: Global patterns in marine sediment carbon stocks. Front. Mar. Sci. 8, 673141. doi: 10.3389/fmars.2021.673141
Baker, M., and German, C. (2008). Going for Gold! Who will win the race to exploit ores from the deep? Ocean Chall. 16, 10–17.
Baker, M., Ramirez-Llodra, E., Tyler, P. A., and Tyler, P. (2020). Natural Capital and Exploitation of the Deep Ocean. Oxford University Press.
Barritt, E. (2019). “The story of stewardship and ecological restoration,” in Ecological Restoration Law: Concepts and Case Studies, eds. A. Akhtar-Khavari and B.J. Richardson (Milton Park), 72–92.
Bayulken, B., Huisingh, D., and Fisher, P. M. (2021). How are nature based solutions helping in the greening of cities in the context of crises such as climate change and pandemics? A comprehensive review. J. Cleaner Prod. 288, 125569. doi: 10.1016/j.jclepro.2020.125569
Bibi, F., Faheem, M., Azhar, E. I., Yasir, M., Alvi, S. A., Kamal, M. A., et al. (2017). Bacteria from marine sponges: a source of new drugs. Curr. Drug Metabol. 18, 11–15. doi: 10.2174/1389200217666161013090610
Bindoff, N. L., Cheung, W. W. L., Kairo, J. G., Arístegui, J., Guinder, V. A., Hallberg, R., et al. (2019). “Changing ocean, marine ecosystems, and dependent communities,” in IPCC Special Report on the Ocean and Cryosphere in a Changing Climate, eds H.-O. Pörtner, D. C. Roberts, V. Masson-Delmotte, P. Zhai, M. Tignor, E. Poloczanska, K. Mintenbeck, A. Alegría, M. Nicolai, A. Okem, J. Petzold, B. Rama, and N. M. Weyer (Cambridge; New York, NY: Cambridge University Press), 447–587. doi: 10.1017/9781009157964.007
Blasiak, R., Jouffray, J. B., Amon, D. J., Moberg, F., Claudet, J., Søgaard Jørgensen, P., et al. (2022). A forgotten element of the blue economy: marine biomimetics and inspiration from the deep sea. PNAS Nexus. 1, pgac196. doi: 10.1093/pnasnexus/pgac196
Blasiak, R., Kenchington, E., Arrieta, J. M., Bermúdez-Monsalve, J. R., Calumpong, H., Changwei, S., et al. (2021). Developments in the Exploration for and Use of Marine Genetic Resources. World Ocean Assessment II. Food and Agriculture Organization of the United Nations, AGRIS.
Böhnke-Henrichs, A., Baulcomb, C., Koss, R., Hussain, S. S., and de Groot, R. S. (2013). Typology and indicators of ecosystem services for marine spatial planning and management. J. Environ. Manag. 130, 135–145. doi: 10.1016/j.jenvman.2013.08.027
Borg, S. (2012). Conservation on the High Seas. Harmonizing Int. Reg. Sustain. Use Living Resour. 167, 649. doi: 10.4337/9780857935649
Boyd, P. W., Bach, L. T., Hurd, C. L., Paine, E., Raven, J. A., and Tamsitt, V. (2022). Potential negative effects of ocean afforestation on offshore ecosystems. Nat. Ecol. Evol. 6, 675–683. doi: 10.1038/s41559-022-01722-1
Boyd, P. W., Claustre, H., Levy, M., Siegel, D. A., and Weber, T. (2019). Multi-faceted particle pumps drive carbon sequestration in the ocean. Nature 568, 327–335 doi: 10.1038/s41586-019-1098-2
Boyle, A. (2021). “Protecting the marine environment from climate change: the LOSC part XII regime,” in The Law of the Sea and Climate Change: Solutions and Constraints, eds E. Johansen, S.V. Busch and I.U. Jakobsen (Cambridge: Cambridge University Press), 81–103, 87–96.
Bravo, M. E., Brandt, M. I., van der Grient, J. M. A., Dahlgren, T. G., Esquete, P., Gollner, S., et al. (2023). Insights from the management of offshore energy resources: toward an ecosystem-services based management approach for deep-ocean industries. Front. Mar. Sci. 9, 994632. doi: 10.3389/fmars.2022.994632
Brito-Morales, I., Schoeman, D. S., Molinos, J. G., Burrows, M. T., Klein, C. J., Arafeh-Dalmau, N., et al. (2020). Climate velocity reveals increasing exposure of deep-ocean biodiversity to future warming. Nat. Climate Change 10, 576–581. doi: 10.1038/s41558-020-0773-5
Brooks, C. M., Ainley, D. G., Jacquet, J., Chown, S. L., Pertierra, L. R., Francis, E., et al. (2022). Protect global values of the Southern Ocean ecosystem. Science. 378, 477–479. doi: 10.1126/science.add9480
Burnett, D., Davenport, T., and Beckman, R. (2014). “Why submarine cables?” in Submarine Cables: The Handbook of Law and Policy, eds D. R. Burnett, R. C. Beckman and T. M. Davenport (Leiden) 1–15.
Campbell, N., Neat, F., Burns, F., and Kunzlik, P. (2011). Species richness, taxonomic diversity, and taxonomic distinctness of the deep-water demersal fish community on the Northeast Atlantic continental slope (ICES Subdivision VIa). ICES J. Mar. Sci. 68, 365–376. doi: 10.1093/icesjms/fsq070
Chami, R., Cosimano, T., Fullenkamp, C., and Oztosun, S. (2019). Nature's Solution to Climate Change, Finance and Development. IMF. Available online at: https://www.imf.org/-/media/Files/Publications/Fandd/Article/2019/December/natures-solution-to-climate-change-chami.ashx
Charles, A. (2013). Fisheries management and governance: forces of change and inertia. 27 Ocean Yearbook 249–266, 253–255. doi: 10.1163/22116001-90000161
Chausson, A., Turner, B., Seddon, D., Chabaneix, N., Girardin, C. A., Kapos, V., et al. (2020). Mapping the effectiveness of nature-based solutions for climate change adaptation. Global Change Biol. 26, 6134–6155. doi: 10.1111/gcb.15310
Chen, W., Wallhead, P., Hynes, S., Groeneveld, R., O'Connor, E., Gambi, C., et al. (2022). Ecosystem service benefits and costs of deep-sea ecosystem restoration. J. Environ. Manag. 303, 114127. doi: 10.1016/j.jenvman.2021.114127
Cheung, W. W. L., Wei, C.-L., and Levin, L. A. (2022). Vulnerability of exploited deep-sea demersal species to ocean warming, deoxygenation, and acidification. Environ. Biol Fish. 105, 1301–1315. doi: 10.1007/s10641-022-01321-w
Chikamoto, M. O., DiNezio, P., and Lovenduski, N. (2023). Long-term slowdown of ocean carbon uptake by alkalinity dynamics. Geophys. Res. Lett. 50, e2022GL101954. doi: 10.1029/2022GL101954
Cochonat, P., Dürr, S., Gunn, V., Herzig, P., and Mevel, C. eds. (2007). The Deep-Sea Frontier: Science Challenges for a Sustainable Future. Brussels: Report published by the European Commission, Directorate-General for Research, Environment, Unit for Management of Natural Resources.
Cohen-Shacham, E., Walters, G., Janzen, C., and Maginnis, S., (eds.) (2016). Nature-Based Solutions to Address Global Societal Challenges. Gland: IUCN. xiii + 97.
Conference of the Parties (2004). Conference of the Parties to the Convention on Biological Diversity, Decision VII/5, Paragraph 30, Seventh Meeting. Kuala Lumpur: COP.
Conference of the Parties (2008). Conference of the Parties to the Convention on Biological Diversity, Decision IX/20, UNEP/CBD/COP/DEC/IX/20, Annex I. Bonn: COP.
Cooley, S., Schoeman, D., Bopp, L., Boyd, P., Donner, S., Ghebrehiwet, D. Y., et al. (2022). “Oceans and coastal ecosystems and their services,” in Climate Change 2022: Impacts, Adaptation and Vulnerability. Contribution of Working Group II to the Sixth Assessment Report of the Intergovernmental Panel on Climate Change, eds H.-O. Portner, D. C. Roberts, M. Tignor, E. S. Poloczanska, K. Mintenbeck, A. Alegra, M. Craig, S. Langsdorf, S. L. Schke, V. M.ller, A. Okem, and B. Rama (Cambridge; New York, NY: Cambridge University Press), 379–550. doi: 10.1017/9781009325844.005
Crain, C. M., Kroeker, K., and Halpern, B. S. (2008). Interactive and cumulative effects of multiple human stressors in marine systems. Ecol. Lett. 11, 1304–1315. doi: 10.1111/j.1461-0248.2008.01253.x
Danovaro, R., Aronson, J., Cimino, R., Gambi, C., Snelgrove, P. V. R., and Van Dover., C. (2021). Marine ecosystem restoration in a changing ocean. Restor. Ecol. 29, E13432. doi: 10.1111/rec.13432
Danovaro, R., Corinaldesi, C., Dell'Anno, A., and Snelgrove, P. V. (2017). The deep-sea under global change. Curr. Biol. 27, R461–R465. doi: 10.1016/j.cub.2017.02.046
Danovaro, R., Fanelli, E., Aguzzi, J., Billett, D., Carugati, L., Corinaldesi, C., et al. (2020). Ecological variables for developing a global deep-ocean monitoring and conservation strategy. Nat. Ecol. Evol. 4, 181–192. doi: 10.1038/s41559-019-1091-z
Dawson, M. N. (2012). Species richness, habitable volume, and species densities in freshwater, the sea, and on land. Front. Biogeogr. 4, 105–116. doi: 10.21425/F54312675
De Groot, R., Brander, L., Van Der Ploeg, S., Costanza, R., Bernard, F., Braat, L., et al. (2012). Global Estimates of the value of ecosystems and their services in monetary units. Ecosyst. Serv. 1, 50–61. doi: 10.1016/j.ecoser.2012.07.005
De Lucia, V. (2019). “The ‘ecosystem approach,”' in International Environmental Law: Genealogy and Biopolitics (London: Routledge). doi: 10.4324/9781315150772
Dingwall, J. (2023). “The Common heritage conundrum: devising a payment mechanism for exploitation activities in the deep seabed area,” in Virginie Tassin Campanella and Robert Makgill, eds. Seabed Mining and the Law of the Sea (Routledge) (in press).
Dobush, B. J., Gallo, N. D., Guerra, M., Guilloux, B., Holland, E., Seabrook, S., et al. (2021). A New Way Forward for Ocean-Climate Policy as Reflected in the UNFCCC Ocean and Climate Change Dialogue SubmissionsClimate Policy. Taylor & Francis Group.
DOSI (2021). Available online at: https://www.dosi-project.org/resources/dosi-policy-briefs/ (accessed February 15, 2023).
Dowd, S., Chapman, M., Koehn, L. E., and Hoagland, P. (2022). The economic tradeoffs and ecological impacts associated with a potential mesopelagic fishery in the California Current. Ecol. Applicat. 32, e2578. doi: 10.1002/eap.2578
Duarte, C. M., Wu, J., Xiao, X., Bruhn, A., and Krause-Jensen, D. (2017). Can seaweed farming play a role in climate change mitigation and adaptation? Front. Mar. Sci. 4, 100. doi: 10.3389/fmars.2017.00100
Ehrlich, H., Etnoyer, P., Litvinov, S. D., Olennikova, M. M., Domaschke, H., Hanke, T., et al. (2006). Biomaterial structure in deep-sea bamboo coral (Anthozoa: Gorgonacea: Isididae): perspectives for the development of bone implants and templates for tissue engineering. Materialwissenschaft Werkstofftechnik 37, 552–557. doi: 10.1002/mawe.200600036
EIA (2016). Offshore Oil Production in Deepwater and Ultra-Deepwater is Increasing. Independent Statistics and Analysis. U.S. Energy Information Administration. Available online at: http://www.eia.gov/todayinenergy/detail.php?id=28552# (accessed February 5, 2022).
Elsler, L. G., Oostdijk, M., Levin, L. A., Satterthwaite, E. V., Pinsky, M. L., Crespo, G. O., et al. (2022). Protecting ocean carbon through biodiversity and climate governance. Front. Marine Sci. 9, 880424. doi: 10.3389/fmars.2022.880424
Far, S. B., Bamakan, S. M. H., Qu, Q., and Jiang, Q. (2022). A review of non-fungible tokens applications in the real-world, and metaverse. Proced. Comput. Sci. 214, 755–762. doi: 10.1016/j.procs.2022.11.238
Feichtner, I. (2019). Sharing the riches of the sea: the redistributive and fiscal dimension of deep seabed exploitation. Eur. J. Intern. Law 30, 601–33. doi: 10.1093/ejil/chz022
Food and Agriculture Organization (2009). International Guidelines for the Management of Deep-Sea Fisheries in the High Seas, United Nations. Rome: FAO
Food Agriculture Organization (2017). Ecosystem Services and Biodiversity (ESB) – Background. Rome: FAO. Available online at: www.fao.org/ecosystem-services-biodiversity/background/en (accessed February 17, 2022).
Food Agriculture Organization (2019). “Deep-Ocean Climate Change Impacts on Habitat, Fish and Fisheries,” eds Lisa Levin, Maria Baker, and Anthony Thompson. FAO Fisheries and Aquaculture Technical Paper No. 638. Rome, FAO. 186. Available online at: http://www.fao.org/3/ca2528en/CA2528EN.pdf (accessed February 15, 2023).
Food and Agriculture Organization (2020). Report of the Areas Beyond National Jurisdiction Deep Sea Meeting 2019. Rome: FAO.
Food Agriculture Organization, Barange, M., Bahri, T., Beveridge, M. C. M., and Cochrane, K. L. (2018). Impacts of Climate Change on Fisheries and Aquaculture: Synthesis of Currrent Knowledge, Adaptation and Mitigation Options [2018] FAO Fisheries and Aquaculture Technical Paper (FAO) Eng No. 627.
Frantzeskaki, N., McPhearson, T., Collier, M. J., Kendal, D., Bulkeley, H., and Dumitru, A. (2019). Nature-based solutions for urban climate change adaptation: linking science policy, and practice communities for evidence-based decision-making. BioScience 69, 455–466. doi: 10.1093/biosci/biz042
Friess, D. A., Krauss, K. W., Taillardat, P., Adame, M. F., Yando, E. S., and Cameron, C. (2020). Mangrove Blue Carbon in the face of Deforestation, climate change, and restoration. Ann. Plant Rev. 3, 427–456. doi: 10.1002/9781119312994.apr0752
Fullam, C., Strong, A. L., Pouponneau, A., and Reiter, S. (2021). Sustainab. Climate Change 14, 366–376. doi: 10.1089/scc.2021.0078
Gallo, N. D., Victor, D. G., and Levin, L. A. (2017). Evaluating ocean commitments under the paris agreement. Nat. Clim. Chang. 7, 833–838. doi: 10.1038/NCLIMATE3422
Gattuso, J.-P., Magnan, A. K., Bopp, L., Cheung, W. W. L., Duarte, C. M., Hinkel, J., et al. (2018). Ocean solutions to address climate change and its effects on marine ecosystems. Front. Mar. Sci. 5, 337. doi: 10.3389/fmars.2018.00337
Gautier, P., and Tassin, V. J. (2013). Offshore platforms and international law. Annuaire Francais de Droit Int. (Online). 59, 185–220. doi: 10.3406/afdi.2013.4816
Glenn, H., Wattage, P., Mardle, S., Van Rensburg, T., Grehan, A., and Foley, N. (2010). Marine protected areas—substantiating their worth. Mar. Pol. 34, 421–430. doi: 10.1016/j.marpol.2009.09.007
Greenberg, P. (2010). Four Fish: The Future of the Last Wild Food (New York, NY: Penguin Books), 187.
Grehan, A., Long, R., Deegan, B., and O'Cinneide, M. (2003). The Irish coral task force and Atlantic coral ecosystem study report on two deep water coral conservation stakeholder workshops. Marine Environ. Health Series 11, 117.
Grotius, H. (1916). “Mare liberum sive de iure quod Batavis competit ad indicana commercia” (dissertatio translation by R. van Deman Magoffin) (New York), 29.
Gruber, N., Clement, D., Carter, B. R., Feely, R. A., Van Heuven, S., Hoppema, M., et al. (2019). The oceanic sink for anthropogenic CO2 from 1994 to 2007. Science. 363, 1193–1199. doi: 10.1126/science.aau5153
Haines-Young, R., and Potschin, M. (2011). “Common International Classification of Ecosystem Services (CICES)”. 2011 update. Paper prepared for the UN/World Bank/EEA Expert Meeting on Ecosystem Accounts, London.
Hardin, G. (1968). The tragedy of the commons. Science 162, 1243–1248. doi: 10.1126/science.162.3859.1243
Harrison, J. (2017). Saving the Oceans Through Law: The International Legal Framework for the Protection of the Marine Environment (Oxford: Oxford University Press), 172–194.
Haugan, P. M., Levin, L. A., Amon, D., Hemer, M., Lily, H., and Nielsen, F. G. (2020). What Role for Ocean-Based Renewable Energy and Deep Seabed Minerals in a Sustainable Future? Washington, DC: World Resources Institute. Available online at: http://www.oceanpanel.org/blue-papers/ocean-energy-and-mineral-sources (accessed February 15, 2023).
Hein, J. R., Koschinsky, A., Mikesell, M., Mizell, K., Glenn, C. R., and Wood, R. (2016). Marine phosphorites as potential resources for heavy rare earth elements and yttrium. Minerals 6, 88. doi: 10.3390/min6030088
Hein, J. R., Mizell, K., Koschinsky, A., and Conrad, T. A. (2013). Deep-ocean mineral deposits as a source of critical metals for high-and green-technology applications: comparison with land-based resources. Ore Geol. Rev. 51, 1–14. doi: 10.1016/j.oregeorev.2012.12.001
Heinrich, L., Koschinsky, A., Markus, T., and Singh, P. (2020). Quantifying the fuel consumption, greenhouse gas emissions and air pollution of a potential commercial manganese nodule mining operation. Marine Policy. 114, 103678. doi: 10.1016/j.marpol.2019.103678
Heinze, C., Blenckner, T., Martins, H., Rusiecka, D., Döscher, R., Gehlen, M., et al. (2021). The quiet crossing of ocean tipping points. PNAS. 118, 9e2008478118. doi: 10.1073/pnas.2008478118
Herr, D., and Landis, E. (2016). Coastal Blue Carbon Ecosystems. Opportunities for Nationally Determined Contributions. Policy Brief. Gland: IUCN; Washington, DC: TNC.
Hidalgo, M., and Browman, H. I. (2019). Developing the knowledge base needed to sustainably manage mesopelagic resources. ICES J. Marine Sci. 76, 609–615. doi: 10.1093/icesjms/fsz067
Hilmi, N., Chami, R., Sutherland, M. D., Hall-Spencer, J. M., Lebleu, L., Benitez, M. B., et al. (2021). The role of blue carbon in climate change mitigation and carbon stock conservation. Front. Clim. 3, 710546. doi: 10.3389/fclim.2021.710546
Hoagland, P., Jin, D., Holland, M., Kostel, K., Taylor, E., Renier, N., et al. (2019). Ecosystem Services of the Mesopelagic. Woods Hole Oceanographic Institution, 35 pp. Available online at: https://hdl.handle.net/1912/25013.1
Hoagland, P., Porter, H., Jin, D., and Beaulieu, S. (2020). “A primer on the economics of natural capital and its relevance to deep-sea exploitation and conservation,” in Natural Capital and Exploitation of the Deep Ocean. eds M. Baker, E. Ramirez-Llodra, P. Tyler. Available online at: https://global.oup.com/booksites/content/9780198841654/artwork/ (accessed February 15, 2023).
Hogg, M. M., Tendal, O. S., Conway, K. W., Pomponi, S. A., van Soest, R. W. M., Gutt, J., et al. (2010). Deep-sea Sponge Grounds: Reservoirs of Biodiversity. UNEP-WCMC Biodiversity Series No. 32. Cambridge: UNEP-WCMC.
Intergovernmental Panel on Climate Change (2019). “Annex I: glossary,” [Weyer, N.M. (ed.)]. in IPCC Special Report on the Ocean and Cryosphere in a Changing Climate, eds H.-O. Pörtner, D.C. Roberts, V. Masson-Delmotte, P. Zhai, M. Tignor, E. Poloczanska, K. Mintenbeck, A. Alegría, M. Nicolai, A. Okem, J. Petzold, B. Rama, N.M. Weyer. (Cambridge, New York, NY: Cambridge University Press),677–702.
International Seabed Authority (2004). “Polymetallic sulphides and cobalt rich ferromanganese crust deposits: establishment of environmental baselines and an associated monitoring programme during exploration,” in Proceedings of the International Seabed Authority's workshop, Kingston, Jamaica, 6–10.
International Seabed Authority (2011). Environmental Management Plan for the Clarion-Clipperton Zone, ISBA/17/LTC/7 2011. Paragraph 27. ISA. Available online at: https://www.isa.org.jm/minerals/environmental-management-plan-clarion-clipperton-zone (accessed February 15, 2023).
International Seabed Authority (2022). Exploration Contracts. ISA. Available online at: https://www.isa.org.jm/exploration-contracts (accessed May 18, 2022).
IPCC (2013). “Intergovernmental panel on climate change 2014,” in 2013 Revised Supplementary Methods and Good Practice Guidance Arising from the Kyoto Protocol, eds T. Hiraishi, T. Krug, K. Tanabe, N. Srivastava, J. Baasansuren, M. Fukuda, and T. G Troxler (IPCC).
IPCC (2018). “Global warming of 1.5°C,” in An IPCC Special Report on the Impacts of Global Warming of 1.5°C Above Pre-industrial Levels and Related Global Greenhouse Gas Emission Pathways, in the Context of Strengthening the Global Response to the Threat of Climate Change, Sustainable Development, and Efforts to Eradicate Poverty, eds V. Masson-Delmotte et al. (Cambridge; New York, NY: Cambridge University Press), 541–562. Available online at: https://report.ipcc.ch/ar6syr/pdf/IPCC_AR6_SYR_SPM.pdf
IPCC (2023). Synthesis Report of the IPCC Sixth Assessment Report (AR6) Summary for Policy Makers. IPCC
IUCN (2022). Governing Areas Beyond National Jurisdiction. International Union for Conservation of Nature, Issue Brief. Available online at: https://www.iucn.org/resources/issues-brief/governing-areas-beyond-national-jurisdiction#:~:text=Nearly%20two%2Dthirds%20of%20the%20world's%20ocean%20is%20beyond%20national,Earth's%20total%20habitat%20by%20volume (accessed February 15, 2023).
Jacquemont, J., Blasiak, R., Le Cam, C., Le Gouellec, M., and Claudet, J. (2022). Ocean conservation boosts climate change mitigation and adaptation. One Earth 5, 1126–1138. doi: 10.1016/j.oneear.2022.09.002
Jaeckel, A. (2017). The International Seabed Authority and the Precautionary Principle: Balancing Deep Seabed Mineral Mining and Marine Environmental Protection (Leiden: Brill Publishers), 88–189.
Jaeckel, A., Ardron, J. A., and Gjerde, K. M. (2016). Sharing benefits of the common heritage of mankind – _Is the deep seabed mining regime ready? Mar. Policy 70, 198–204. doi: 10.1016/j.marpol.2016.03.009
Jakobsen, I. U. (2021). “Marine protected areas and climate change,” in The Law of the Sea and Climate Change: Solutions and Constraints, E. Johansen, S. V. Busch and I. U. Jakobsen (Cambridge: Cambridge University Press), 234–262. doi: 10.1017/9781108907118
Jin, D., Hoagland, P., and Buesseler, K. (2020). The value of scientific research on the ocean's biological carbon pump. Sci. Total Environ. 749, 141357. doi: 10.1016/j.scitotenv.2020.141357
Jobstvogt, N., Hanley, N., Hynes, S., Kenter, J., and Witte, U. (2014a). Twenty thousand sterling under the sea: estimating the value of protecting deep-sea biodiversity. Ecol. Econ. 97, 10–19. doi: 10.1016/j.ecolecon.2013.10.019
Jobstvogt, N., Townsend, M., Witte, U., and Hanley, N. (2014b). How can we identify and communicate the ecological value of deep-sea ecosystem services? PLoS ONE 9, e100646. doi: 10.1371/journal.pone.0100646
Johnson, D. E. (2019). Protecting the lost city hydrothermal vent system: all is not lost, or is it? Mar. Policy. 107, 103593. doi: 10.1016/j.marpol.2019.103593
Jørgensen, B. B., and Boetius, A. (2007). Feast and famine—microbial life in the deep-sea bed. Nat. Rev. Microbiol. 5, 770–781. doi: 10.1038/nrmicro1745
Jouffray, J. B., Blasiak, R., Norström, A. V., Österblom, H., and Nyström, M. (2020). The blue acceleration: the trajectory of human expansion into the ocean. One Earth. 2, 43–54. doi: 10.1016/j.oneear.2019.12.016
Kaartvedt, S., Langbehn, T. J., and Aksnes, D. L. (2019). Enlightening the ocean's twilight zone. ICES J. Mar. Sci. 76, 803–812. doi: 10.1093/icesjms/fsz010
Kabisch, N. N., Frantzeskaki, S., Pauleit, S., Naumann, M., Davis, M., Artmann, D., et al. (2016). Nature-based solutions to climate change mitigation and adaptation in urban areas: perspectives on indicators, knowledge gaps, barriers, and opportunities for action. Ecol. Soc. 21, 39. doi: 10.5751/ES-08373-210239
Kaikkonen, L., and van Putten, I. (2021). We may not know much about the deep sea, but do we care about mining it? People Nat. 3, 843–860. doi: 10.1002/pan3.10224
Keesstra, S., Nunes, J., Novara, A., Finger, D., Avelar, D., Kalantari, Z., et al. (2018). The superior effect of nature based solutions in land management for enhancing ecosystem services. Sci. Total Environ. 610–611, 997–1009. doi: 10.1016/j.scitotenv.2017.08.077
Kendall, V. J., and Haedrich, R. L. (2006). Species richness in Atlantic deepsea fishes assessed in terms of the mid-domain effect and Rapoport's rule. Deep Sea Research Part I Oceanogr. Res. Papers 53, 506–515. doi: 10.1016/j.dsr.2005.12.005
Krabbe, N., Langlet, D., Belgrano, A., and Villasante, S. (2022). Reforming international fisheries law can increase blue carbon sequestration. Front. Mar. Sci. 9, e800972. doi: 10.3389/fmars.2022.800972
Krause-Jensen, D., and Duarte, C. M. (2016). Substantial role of macroalgae in marine carbon sequestration. Nat. Geosci. 9, 737–742. doi: 10.1038/ngeo2790
Kubiszewski, I., Costanza, R., Anderson, S., and Sutton, P. (2017). The future value of ecosystem services: global scenarios and national implications. Ecosyst. Serv. 26, 289–301. doi: 10.1016/j.ecoser.2017.05.004
Kuhlbrodt, T., Griesel, A., Montoya, M., Levermann, A., Hofmann, M., and Rahmstorf, S. (2007). On the driving processes of the Atlantic meridional overturning circulation. Rev. Geophys. 45, 166. doi: 10.1029/2004RG000166
Kumar, P., Debele, S. E., Sahani, J., Rawat, N., Marti-Cardona, B., and Alfieri, S. M. (2021). Nature-based solutions efficiency evaluation against natural hazards: modelling methods, advantages and limitations. Sci. Total Environ. 784, 147058. doi: 10.1016/j.scitotenv.2021.147058
Kuwae, T., and Crooks, S. (2021). Linking climate change mitigation and adaptation through coastal green–gray infrastructure: a perspective. Coastal Eng. J. 63, 188–199. doi: 10.1080/21664250.2021.1935581
Kwiatkowski, L., Torres, O., Bopp, L., Aumont, O., Chamberlain, M., Christian, J. R., et al. (2020). Twenty-first century ocean warming, acidification, deoxygenation, and upper-ocean nutrient and primary production decline from CMIP6 model projections. Biogeosciences 17, 3439–3470. doi: 10.5194/bg-17-3439-2020
Laffoley, D., Baxter, J. M., Amon, D. J., Claudet, J., Hall-Spencer, J. M., Grorud-Colvert, K., et al. (2020). Evolving the narrative for protecting a rapidly changing ocean, post-COVID-19. Aquatic Conserv. Mar. Freshw Ecosyst. 31, 1512–1534. doi: 10.1002/aqc.3512
Larkin, K. E., Donaldson, K., McDonough, N., and Rogers, A. (2015). Delving Deeper: How Can we Achieve Sustainable Management of Our Deep Sea Through Integrated Research? EMB Policy Brief No. 2. Ostend: European Marine Board.
Le, J. T., Girguis, P. R., and Levin, L. A. (2022). Using deep-sea images to examine ecosystem services associated with methane seeps. Mar. Environ. Res. 181, 105740. doi: 10.1016/j.marenvres.2022.105740
Le, J. T., Levin, L. A., and Carson, R. T. (2017). Incorporating ecosystem services into environmental management of deep-seabed mining. Deep Sea Research Part II Top. Stud. Oceanogr. 137, 486–503. doi: 10.1016/j.dsr2.2016.08.007
Levin, L. A. (2022). Ocean commitment and controversy. Nat. Geosci. 15, 754–755. doi: 10.1038/s41561-022-01042-w
Levin, L. A., Alfaro-Lucas, J. M., Colaço, A., Cordes, E. E., Craik, N., and Danovaro, R. (2023). Deep-sea impacts of climate interventions. Science 379, 978–981. doi: 10.1126/science.ade7521
Levin, L. A., Amon, D. J., and Lily, H. (2020). Challenges to the sustainability of deep-seabed mining. Nat. Sustain. 3, 784–794. doi: 10.1038/s41893-020-0558-x
Levin, L. A., Baco, A. R., Bowden, D. A., Colaco, A., Cordes, E. E., Cunha, M. R., et al. (2016). Hydrothermal vents and methane seeps: rethinking the sphere of influence. Front. Marine Sci. 3, 72. doi: 10.3389/fmars.2016.00072
Levin, L. A., Bett, B. J., Gates, A. R., Heimbach, P., Howe, B. M., Janssen, F., et al. (2019). Global observing needs in the deep ocean. Front. Mar. Sci. 6, 241. doi: 10.3389/fmars.2019.00241
Levin, L. A., and Le Bris, N. (2015). Deep oceans under climate change. Science 350, 766–768. doi: 10.1126/science.aad0126
Lovelock, C. E., and Duarte, C. M. (2019). Dimensions of blue carbon and emerging perspectives. Biol. Lett. 15, 20180781. doi: 10.1098/rsbl.2018.0781
Luisetti, T., Turner, R. K., Andrews, J. E., Jickells, T. D., Kröger, S., Diesing, M., et al. (2019). Quantifying and valuing carbon flows and stores in coastal and shelf ecosystems in the UK. Ecosyst. Serv. 35, 67–76. doi: 10.1016/j.ecoser.2018.10.013
MA (Millennium Ecosystem Assessment). (2005). Ecosystems and Human Well-Being: The Assessment Series (Four Volumes and Summary). Washington, DC: Island Press; World Resources Institute.
Macreadie, P. I., Anton, A., Raven, J. A., Beaumont, N., Connolly, R. M., Friess, D. A., et al. (2019). The future of Blue Carbon science. Nat. Commun. 10, 3998. doi: 10.1038/s41467-019-11693-w
Macreadie, P. I., Costa, M. D., Atwood, T. B., Friess, D. A., Kelleway, J. J., Kennedy, H., et al. (2021). Blue Carbon as a natural climate solution. Nat. Rev. Earth Environ. 2, 826–839. doi: 10.1038/s43017-021-00224-1
Maier-Reimer, E., Mikolajewicz, U., and Winguth, A. (1996). Future ocean uptake of CO2: interaction between ocean circulation and biology. Climate Dynamics 12, 711–722. doi: 10.1007/s003820050138
Marauhn, T., and Böhringer, A.-M. (2014). “An ecosystem approach to the transboundary protection of biodiversity,” in Transboundary Governance of Biodiversity, eds L. J. Kotzé and T. Marauhn (Leiden; Boston: Brill Publishers), 91–104.
Mariani, G. W., Cheung, W. L., Lyet, A., Sala, E., Mayorga, J., Velez, L., et al. (2020). Let more big fish sink: fisheries prevent Blue Carbon sequestration – half in unprofitable areas. Sci. Adv. 6, eabb4848. doi: 10.1126/sciadv.abb4848
Martin, A., Boyd, P., Buesseler, K., Cetinic, I., Claustre, H., Giering, S., et al. (2020). The oceans' twilight zone must be studied now, before it is too late. Nature 580, 26–28. doi: 10.1038/d41586-020-00915-7
Martins, A., Vieira, H., Gaspar, H., and Santos, S. (2014). Marketed marine natural products in the pharmaceutical and cosmeceutical industries: tips for success. Marine Drugs 12, 1066–1101. doi: 10.3390/md12021066
Mayer, A. M., Glaser, K. B., Cuevas, C., Jacobs, R. S., Kem, W., Little, R. D., et al. (2010). The odyssey of marine pharmaceuticals: a current pipeline perspective. Trends Pharmacol. Sci. 3, 255–265. doi: 10.1016/j.tips.2010.02.005
McAllister, D. E. (1988). Environmental, economic and social costs of coral reef destruction in the Philippines. Galaxea 7, 161–178.
MEA (2005). Ecosystems and Human Well-Being: Current State and Trends. Millennium Ecosystem Assessment, Vol. 1. Washington DC: Island Press.
M. E. A. (2005). A Report of the Millennium Ecosystem Assessment. Ecosystems and Human Well-Being. Washington, DC: Island Press.
Mengerink, K. J., Van Dover, C. L., Ardron, J., Baker, M., Escobar-Briones, E., Gjerde, K., et al. (2014). A call for deep-ocean stewardship. Science 344, 696–698. doi: 10.1126/science.1251458
Merrie, A., Dunn, D. C., Metian, M., Boustany, A. M., Takei, Y., Elferink, A. O., et al. (2014). An ocean of surprises–Trends in human use, unexpected dynamics and governance challenges in areas beyond national jurisdiction. Global Environ. Change 27, 19–31. doi: 10.1016/j.gloenvcha.2014.04.012
Molenaar, E. J. (2021). “Integrating climate change in international fisheries law,” in The Law of the Sea and Climate Change: Solutions and Constraints, eds E. Johansen, S.V. Busch and I.U. Jakobsen (Cambridge: Cambridge University Press).
Morato, T., González-Irusta, J.-M., Dominguez-Carrió, C., Wei, C. L., Davies, A., Sweetman, A. K., et al. (2020). Climate-induced changes in the suitable habitat of cold-water corals and commercially important deep-sea fishes in the North Atlantic. Glob. Chang. Biol. 26, 2181–2202. doi: 10.1111/gcb.14996
Murawski, S. A. (2007). Ten myths concerning ecosystem approaches to marine resources management. Mar. Policy 31, 681–690. doi: 10.1016/j.marpol.2007.03.011
NASEM (2022). A Research Strategy for Ocean-based Carbon Dioxide Removal and Sequestration. National Academies of Sciences, Engineering, and Medicine. Washington DC: The National Academies Press.
Nguyen, T. P. (2018). Melaleuca entrapping microsites as a nature based solution to coastal erosion: a pilot study in Kien Giang, Vietnam. Ocean Coastal Manag. 155, 98–103. doi: 10.1016/j.ocecoaman.2018.02.005
NOAA (2018). Gas Hydrates are Ice-Like Substances that Form in Deep-Sea Sediments. Ocean Exploration Facts. Available online at: http://oceanexplorer.noaa.gov/facts/hydrates.html (accessed February 19, 2022).
NOAA (2022). Port Restrictions under the Moratorium Protection Act. Available online at: https://www.fisheries.noaa.gov/content/port-restrictions-under-moratorium-protection-act (accessed February 15, 2023).
Noble, M., Duncan, P., Perry, D., Prosper, K., Rose, D., and Schnierer, S. (2016). Culturally significant fisheries: keystones for management of freshwater social-ecological systems. Ecol. Soc. 21, 222. doi: 10.5751/ES-08353-210222
Nomura, I. (2014). “Sustainable world fisheries: elements of success,” in Peaceful Order in the World's Oceans. Essays in Honor of Satya N. Nandan, eds M. W. Lodge and M. H. Nordquist (Leiden: Brill), 325–337,331, and 332. doi: 10.1163/9789004274976
O'Connor, E., Hynes, S., and Chen, W. (2020). Estimating the non-market benefit value of deep-sea ecosystem restoration: evidence from a contingent valuation study of the Dohrn Canyon in the Bay of Naples. J. Environ. Manag. 275, 111180. doi: 10.1016/j.jenvman.2020.111180
Oldham, P., Hall, S., Barnes, C., Oldham, C., Cutter, M., Burns, N., et al. (2014). Valuing the Deep: Marine Genetic Resources in Areas Beyond National Jurisdiction. DEFRA Contract MB0128. London: DEFRA.
Olsen, R. E., Strand, E., Melle, W., Nørstebø, J. T., Lall, S. P., Ringø, E., et al. (2020). Can mesopelagic mixed layers be used as feed sources for salmon aquaculture? Deep Sea Research Part II Top. Stud. Oceanogr. 180, 104722. doi: 10.1016/j.dsr2.2019.104722
Oostdijk, M. (2021). Fisheries Management under Individual Transferable Quota Outcomes for Ecology and Equity. University of Iceland, School of Engineering and Natural Sciences.
Oostdijk, M., Elsler, L., Ramirez-Monsalve, P., Orach, K., and Wisz, M. (2022). Governing open ocean and fish carbon: Perspectives and opportunities. Front. Marine Sci. 9, 764609. doi: 10.3389/fmars.2022.764609
Orcutt, B. N., Bradley, J. A., Brazelton, W. J., Estes, E. R., Goordial, J. M., Huber, J. A., et al. (2020). Impacts of deep-sea mining on microbial ecosystem services. Limnol. Oceanogr. 65, 1489–1510. doi: 10.1002/lno.11403
Orrego Vicuña, F. (1999). The Changing International Law of High Seas Fisheries. Cambridge: Cambridge University Press.
Ottaviani, D. (2020). Economic Value of Ecosystem Services From Deep Seas Beyond National Jurisdiction. FAO Fisheries and Aquaculture Circular No. 1210. Rome: FAO.
Paine, L. (2015). The Sea and Civilization: A Maritime History of the World. New York, NY: Vintage Books.
Pascual, U., Muradian, R., Brander, L., Gómez-Baggethun, E., Martín-López, B., Verma, M., et al. (2011). “The economics of valuing ecosystem services and biodiversity,” in The Economics of Ecosystems and Biodiversity: Ecological and Economic Foundations, eds P. Kumar (London: Routledge), 183–256.
Pörtner, H.-O., Scholes, R. J., Arneth, A., Barnes, D. K. A., Burrows, M. T., Diamond, S. E., et al. (2023). Overcoming the coupled climate and biodiversity crises and their societal impacts. Science 380, eabl4881. doi: 10.1126/science.abl4881
Prellezo, R. (2019). Exploring the economic viability of a mesopelagic fishery in the Bay of Biscay. ICES J. Mar. Sci. 76, 771–779. doi: 10.1093/icesjms/fsy001
Proud, R., Handegard, N. O., Kloser, R. J., Cox, M. J., and Brierley, A. S. (2019). From siphonophores to deep scattering layers: uncertainty ranges for the estimation of global mesopelagic fish biomass. ICES J. Marine Sci. 76, 718–733. doi: 10.1093/icesjms/fsy037
Queirós, A. M., Talbot, E., Beaumont, N. J., Somerfield, P. J., Kay, S., and Pascoe, C. (2021). Bright spots as climate-smart marine spatial planning tools for conservation and blue growth. Glob. Change Biol. 27, 5514–5531. doi: 10.1111/gcb.15827
Quevedo, J. M. D., Uchiyama, Y., and Kohsaka, R. (2021). Local perceptions of Blue Carbon ecosystem infrastructures in Panay Island, Philippines. Coastal Eng. J. 63, 227–247. doi: 10.1080/21664250.2021.1888558
Ramirez-Llodra, E., Brandt, A., Danovaro, R., De Mol, B., Escobar, E., German, C. R., et al. (2011). Deep, diverse and definitely different: unique attributes of the world's largest ecosystem. Biogeosciences 7, 2851–2899. doi: 10.5194/bg-7-2851-2010
Rayfuse, R. (2019). “Addressing climate change impacts in regional fisheries management organizations,” in Strengthening International Fisheries Law in an Era of Changing Oceans, eds R. Caddell and E. J. Molenaar (Oxford: Hart Publishing), 247–268.
Rayfuse, R., and Warner, R. (2008). Securing a sustainable future for the oceans beyond national jurisdiction: the legal basis for an integrated cross-sectoral regime for high seas governance for the 21st century. Int. J. Mar. Coastal Law 399–421, 419/411. doi: 10.1163/092735208X331845
Robison, B. H. (2009). Conservation of deep pelagic biodiversity. Conserv. Biol. 23, 847–858. doi: 10.1111/j.1523-1739.2009.01219.x
Saito, T., Uchida, I., and Takeda, M. (2002). Skeletal growth of the deep-sea hexactinellid sponge Euplectella oweni, and host selection by the symbiotic shrimp Spongicola japonica (Crustacea: Decapoda: Spongicolidae). J. Zool. 258, 521–529.
Sala, E., Mayorga, J., Bradley, D., Cabral, R. B., Atwood, T. B., Auber, A., et al. (2021). Protecting the global ocean for biodiversity, food and climate. Nature. 592, 397–402. doi: 10.1038/s41586-021-03371-z
Sala, E., Mayorga, J., Costello, C., Kroodsma, D., Palomares, M. L., Pauly, D., et al. (2018). The economics of fishing the high seas. Sci. Adv. 4, eaat2504. doi: 10.1126/sciadv.aat2504
Sarmiento, J. L, and Gruber, N. (2013). Ocean biogeochemical dynamics, ocean biogeochemical dynamics (Princeton University Press: Princeton). doi: 10.1515/9781400849079
Schadeberg, A., Kraan, M., Groeneveld, R., Trilling, D., and Bush, S. (2023). Science governs the future of the mesopelagic zone. NPJ Ocean Sustain. 2, 2. doi: 10.1038/s44183-023-00008-8
Schneider, C. L. (2018). “Marine refugia past, present, and future: Lessons from ancient geologic crises for modern marine ecosystem conservation,” in Marine Conservation Paleobiology, eds C. Tyler and C. Schneider (Cham: Springer), 163–208. doi: 10.1007/978-3-319-73795-9_8
Seddon, N., Chausson, A., Berry, P., Girardin, C. A. J., Smith, A., and Turner, B. (2020). Understanding the value and limits of nature-based solutions to climate change and other global challenges. Phil. Trans. R. Soc. B 375:20190120. doi: 10.1098/rstb.2019.0120
Seddon, N., Smith, A., Smith, P., Key, I., Chausson, A., Girardin, C., et al. (2021). Getting the message right on nature-based solutions to climate change. Glob. Change Biol 27, 1518–1546. doi: 10.1111/gcb.15513
Seymour, F., Wolosin, M., and Gray, E. (2022). Policies Underestimate Forests' Full Effect on the Climate. Washington, DC: World Resources Institute. Available online at: https://www.wri.org/insights/how-forests-affect-climate
Sharma, R. (2022). Perspectives on Deep-Sea Mining: Sustainability, Technology, Environmental Policy and Management. Switzerland: Springer.
Shen, Z., Wakita, K., Oishi, T., Yagi, N., Kurokura, H., Blasiak, R., et al. (2015). Willingness to pay for ecosystem services of open oceans by choice-based conjoint analysis: A case study of Japanese residents. Ocean Coast Manag. 103, 1–8. doi: 10.1016/j.ocecoaman.2014.10.016
Siegel, D. A., DeVries, T., Doney, S. C., and Bell, T. (2021). Assessing the sequestration time scales of some ocean-based carbon dioxide reduction strategies. Environ. Res. Lett. 16, 104003. doi: 10.1088/1748-9326/ac0be0
Silver, J. J., and Campbell, L. M. (2018). Conservation, development and the blue frontier: the Republic of Seychelles' Debt Restructuring for Marine Conservation and Climate Adaptation Program. Int. Soc. Sci. J. 68, 241–256. doi: 10.1111/issj.12156
Singh, P. (2022). What are the next steps for the international seabed authority after the invocation of the ‘two-year rule'? Int. J. Mar. Coastal Law 37, 1–14. doi: 10.1163/15718085-bja10078
Singh, P. A. (2022). “Deep seabed mining and sustainable development goal 14,” in Life Below Water. Encyclopedia of the UN Sustainable Development Goals, eds W. L. Filho, A. M. Azul, L. Brandli, L. Lange, A. Salvia, and T. Wall (Cham: Springer). doi: 10.1007/978-3-319-98536-7_135
Sladen, A., Rivet, D., Ampuero, J. P., De Barros, L., Hello, Y., Calbris, G., et al. (2019). Distributed sensing of earthquakes and ocean-solid Earth interactions on seafloor telecom cables. Nat. Commun. 10, 1–8. doi: 10.1038/s41467-019-13793-z
Smith, L. M., Cimoli, L., LaScala-Gruenewald, D., Pachiadaki, M., Phillips, B., Pillar, H., et al. (2022). The deep ocean observing strategy: addressing global challenges in the deep sea through collaboration. Mar. Technol. Soc. J. 50–66. doi: 10.4031/MTSJ.56.3.11
Sperling, E. A., Frieder, C. A., and Levin, L. A. (2016). Biodiversity response to natural gradients of multiple stressors on continental margins. Proc. R. Soc. B 283, 20160637. doi: 10.1098/rspb.2016.0637
St John, M. A., Borja, A., Chust, G., Heath, M., Grigorov, I., Mariani, P., et al. (2016). A dark hole in our understanding of marine ecosystems and their services: perspectives from the mesopelagic community. Front. Mar. Sci. 3, 31. doi: 10.3389/fmars.2016.00031
Stankovic, M., Ambo-Rappe, R., Carly, F., Dangan-Galon, F., Fortes, M. D., Hossain, M. S., et al. (2021). Quantification of Blue Carbon in seagrass ecosystems of Southeast Asia and their potential for climate change mitigation. Sci. Total Environ. 783, 146858. doi: 10.1016/j.scitotenv.2021.146858
Stone, C. D. (1999). Can the oceans be harboured? A four step plan for the 21st century. Rev. Eur. Commun. Int. Environ. Law 39, 37–47. doi: 10.1111/1467-9388.00176
Sumaila, U. R., Skerritt, D. J., Schuhbauer, A., Villasante, S., Cisneros-Montemayor, A. M., Sinan, H., et al. (2021). WTO must ban harmful fisheries subsidies. Science 374, 544–544. doi: 10.1126/science.abm1680
Sutton, T. T. (2013). Vertical ecology of the pelagic ocean: classical patterns and new perspectives. J. Fish Biol. 83, 1508–1527. doi: 10.1111/jfb.12263
Sutton, T. T., Wiebe, P. H., Madin, L., and Bucklin, A. (2010). Diversity and community structure of pelagic fishes to 5000 m depth in the Sargasso Sea. Deep Sea Res. Part II: Top. Stud. Oceanograph. 57, 2220–2233. doi: 10.1016/j.dsr2.2010.09.024
Sweetman, A. K., Thurber, A. R., Smith, C. R., Levin, L. A., Mora, C., Wei, C. L., et al. (2017). Major impacts of climate change on deep seafloor ecosystems. Elementa Sci. Anthr. doi: 10.1525/elementa.203
Takei, Y. (2013). Filling Regulatory Gaps in High Seas Fisheries. Discrete High Seas Fish Stocks, Deep-sea Fisheries and Vulnerable Marine Ecosystems (Leiden: Brill), 85–91, 33–104. doi: 10.1163/9789004248601
Tang, Q., and Luo, L. (2014). Carbon management systems and carbon mitigation. Austral. Account. Rev. 24, 84–98. Available online at: https://ssrn.com/abstract=3855192
TEEB (2010). Mainstreaming the Economics of Nature: A Synthesis of the Approach, Conclusions and Recommendations of TEEB.
Telesetsky, A., Cliquet, A., and Akhtar-Khavari, A. (2017). Ecological Restoration in International Environmental Law (London: Routledge), 86. doi: 10.4324/9781315757605
The European Commission (2020). European Commission Executive Agency for Small and Medium-sized Enterprises, Nature Based Solutions: Horizon 2020. NBS Research Projects Tackle the Climate and Biodiversity Crisis, Publications Office 2021. Available online at: https://data.europa.eu/doi/10.2826/59817 (accessed February 15, 2023).
Thurber, A. R., Sweetman, A. K., Narayanaswamy, B. E., Jones, D. O. B., Ingels, J., and Hansman, R. L. (2014). Ecosystem function and services provided by the deep sea. Biogeosci. Discuss. 11, 3941–3963. doi: 10.5194/bg-11-3941-2014
United Nations Educational Scientific Cultural Organization (2022). What is Intangible Cultural Heritage? UNESCO. Available online at: https://whc.unesco.org/en/news/2409 (accessed February 15, 2023).
United Nations Environment Programme Finance Initiative (2022). Harmful Marine Extractives: Understanding the Risks and Impacts of Financing Non-Renewable Extractive Industries. Geneva: United Nations Environment Programme Finance Initiative, 35.
Valeri, M., and Baggio, R. (2021). A critical reflection on the adoption of blockchain in tourism. Inf. Technol. Tourism. 23, 121–132. doi: 10.1007/s40558-020-00183-1
Watson, A. J., Schuster, U., Shutler, J. D., Holding, T., Ashton, I. G. C., Landschutzer, P., et al. (2020). Revised estimates of ocean-atmosphere CO2 flux are consistent with ocean carbon inventory. Nat. Commun. 11, 4422. doi: 10.1038/s41467-020-18203-3
Wedding, L. M., Moritsch, M., Verutes, G., Arkema, K., Hartge, E., Reiblich, J., et al. (2021). Incorporating Blue Carbon sequestration benefits into sub-national climate policies. Global Environ. Change 69, 102206. doi: 10.1016/j.gloenvcha.2020.102206
Wernberg, T., and Filbee-Dexter, K. (2018). Grazers extend Blue Carbon transfer by slowing sinking speeds of kelp detritus. Sci. Rep. 8, 17180(2018). doi: 10.1038/s41598-018-34721-z
Williamson, P., Boyd, P., Harrison, D., Reynard, N., and Mashayekhi, A. (2021). “Biologically-based negative emissions in the open ocean and coastal seas,” in Negative Emission Technologies, eds M. Bui and N. Mac Dowell (London: Royal Society of Chemistry).
Williamson, P., and Gattuso, J.-P. (2022). Carbon removal using coastal blue carbon ecosystems is uncertain and unreliable, with questionable climatic cost-effectiveness. Front. Clim. 4, 853666. doi: 10.3389/fclim.2022.853666
Witherell, D., and Coon, C. (2001). “Protecting gorgorian corals off Alaska from fishing impacts,” in First International Symposium on Deep-Sea Corals, eds J. H. M. Willison, J. Hall, S. Gass, E. Kenchington, M. Butler, P. Doherty (Halifax, Nova Scotia: Ecology Action).
Wood, M. C. (2014). Nature's Trust: Environmental Law for a New Ecological Age. New York, NY: Cambridge University Press.
Yasuhara, M., and Deutsch, C. (2022). Paleobiology provides glimpses of future ocean. Science 375, 25–26. doi: 10.1126/science.abn2384
Yasuhara, M., Wei, C. L., Kucera, M., Costello, M. J., Tittensor, D. P., Kiessling, W., et al. (2020). Past and future decline of tropical pelagic biodiversity. Proc. Natl. Acad. Sci. 117, 12891–12898. doi: 10.1073/pnas.1916923117
Yong, W. T. L., Thien, V. Y., Rupert, R., and Rodrigues, K. F. (2022). Seaweed: a potential climate change solution. Renew. Sustain. Ener. Rev. 159, 112222. doi: 10.1016/j.rser.2022.112222
Yoon, J. E., Yoo, K. C., Macdonald, A. M., Yoon, H. I., Park, K. T., Yang, E. J., et al. (2018). Reviews and syntheses: Ocean iron fertilization experiments – past, present, and future looking to a future Korean Iron Fertilization Experiment in the Southern Ocean (KIFES) project. Biogeosciences 15, 5847–5889. doi: 10.5194/bg-15-5847-2018
Keywords: deep sea, nature-based solutions, blue carbon, climate change, ecosystem services
Citation: Hilmi N, Sutherland M, Farahmand S, Haraldsson G, van Doorn E, Ernst E, Wisz MS, Claudel Rusin A, Elsler LG and Levin LA (2023) Deep sea nature-based solutions to climate change. Front. Clim. 5:1169665. doi: 10.3389/fclim.2023.1169665
Received: 19 February 2023; Accepted: 29 May 2023;
Published: 06 July 2023.
Edited by:
Soheil Shayegh, European Institute of Economics and the Environment, ItalyReviewed by:
Jesse Van Der Grient, South Atlantic Environmental Research Institute, Falkland IslandsCopyright © 2023 Hilmi, Sutherland, Farahmand, Haraldsson, van Doorn, Ernst, Wisz, Claudel Rusin, Elsler and Levin. This is an open-access article distributed under the terms of the Creative Commons Attribution License (CC BY). The use, distribution or reproduction in other forums is permitted, provided the original author(s) and the copyright owner(s) are credited and that the original publication in this journal is cited, in accordance with accepted academic practice. No use, distribution or reproduction is permitted which does not comply with these terms.
*Correspondence: Nathalie Hilmi, aGlsbWlAY2VudHJlc2NpZW50aWZpcXVlLm1j
Disclaimer: All claims expressed in this article are solely those of the authors and do not necessarily represent those of their affiliated organizations, or those of the publisher, the editors and the reviewers. Any product that may be evaluated in this article or claim that may be made by its manufacturer is not guaranteed or endorsed by the publisher.
Research integrity at Frontiers
Learn more about the work of our research integrity team to safeguard the quality of each article we publish.