- 1Department of Sustainable Development, Environmental Science and Engineering, KTH Royal Institute of Technology, Stockholm, Sweden
- 2TERRA, Gothenburg, Sweden
- 3Department of Technology Management and Economics, Chalmers University of Technology, Göteborg, Sweden
- 4IVL Swedish Environmental Research Institute, Stockholm, Sweden
- 5Department of Technology Management and Economics, Swedish Life Cycle Center, Chalmers University of Technology, Gothenburg, Sweden
Biofuel producers and other commodity suppliers are increasingly affected by conflicting rules for life cycle assessment (LCA). They may get multiple requests for LCAs to be used in various contexts, which require the application of different methodological approaches that vary in scope, system boundaries, data demand, and more. This results in increased cost and competence requirements for producers, as well as confusion among other actors including their customers. Differences in methodologies might also lead to various outcomes, conclusions and conflicting guidance regarding which fuels to prioritize or develop. We have analyzed the actual differences when applying three different frameworks: the EU Renewable Energy Directive (RED), the EU framework for Product Environmental Footprints (PEF), and the framework of Environmental Product Declarations (EPD), which have different modeling requirements. We analyzed the methods from a conceptual point of view and also applied the methods to estimate the carbon footprint on a wide range of biofuel production pathways: (i) ethanol from corn, (ii) fatty acid methyl ester (FAME) from rapeseed oil, (iii) biogas from food waste, (iv) hydrogenated vegetable oil (HVO) from rapeseed oil, and (v) HVO from used cooking oil. Results obtained for a specific fuel could differ substantially depending on the framework applied and the assumptions and interpretations made when applying the different frameworks. Particularly, the results are very sensitive to the modeling of waste management when biofuel is produced from waste. Our results indicate a much higher climate impact for, e.g., biogas and HVO produced from used cooking oil when assessed with the PEF framework compared to the other frameworks. This is because PEF assigns at least part of the production of primary materials and energy to the use of recycled material and recovered energy. Developing Category Rules for biofuels for PEF and EPD ought to help clarifying remaining ambiguities.
Introduction
Life cycle assessment (LCA) approaches are increasingly used to guide decisions aimed at improved environmental performance of products or services over their supply chains and across a range of impacts. General LCA practice is standardized by ISO (2006a,b, 2020a,b). More specific calculation rules for assessing biofuels in specific contexts are given by guidelines such as the European Commission's (EC's) Renewable Energy Directive (RED; EC, 2018a), the Product Environmental Footprint (PEF; EC, 2018b), the Environmental Product Declaration (EPD, 2021), the UN Carbon Offsetting and Reduction Scheme for International Aviation (CORSIA; ICAO, 2020), and the UK Renewable Transport Fuel Obligation (DfT, 2008), etc. They are also embedded in tools, such as the CA-GREET3.0, which is used for calculating the climate impact of fuels within the California Low Carbon Fuels Standard (CARB, 2015).
Fuel producers may be required to calculate the carbon footprint of their products which, in turn, requires the application of one or more of these methodological frameworks. Three frameworks might be particularly important for biofuel producers in Europe: RED, PEF, and EPD. The RED framework is commonly applied by biofuel producers in Europe for compliance reporting. It stipulates rules for calculating the reduction in life cycle greenhouse gas (GHG) emissions when using renewable fuels, as well as national targets for EU member states' share of renewable energy, and the eligibility for financial support, which rests on meeting specific sustainability criteria. The RED framework also forms the basis for the methodology in the UK Renewable Transport Fuel Obligation.
In the future, it is likely that the application of PEF will be required. It has been developed by the EC with environmental product declarations and possibly policy-making as intended applications. Detailed rules (PEF Category Rules; PEFCRs) are being developed within this framework, but no PEFCR for biofuel exists yet.
Fuel producers are likely to be asked for input data to EPDs, mainly because their fuel is used in the life cycle of other products. Companies develop life-cycle based EPDs produced for marketing purposes. The detailed rules are specified by Product Category Rules (PCR) that differ between product groups and sometimes among programme operators. PCRs have been developed for an expanding number of product groups. No PCR for biofuel currently exists, although the need has been identified (Hallberg et al., 2013). When a PCR for biofuel is developed, fuel producers can opt to develop their own EPDs.
The three frameworks (RED, PEF, and EPD) vary in scope, system boundaries or modeling requirements (e.g., data and methodological choices). As a result, they may also vary in terms of the information they can provide in relation to the environmental performance of the product. These variations may lead to conflicting outcomes and conclusions but also demand increased competence and resource requirements for the LCA practitioner.
This paper aims at clarifying the degree to which the application of RED, PEF, and EPD to biofuel systems leads to different results, identifying the most important methodological differences between the frameworks, and discussing the extent to which each framework leads to reproducible assessments. An assessment is automatically less capable of being reproduced if the framework explicitly allows for the use of different methods, or if the calculation rules in the framework are unclear.
For this purpose we compile information about the methodology in each framework (section Materials and methods). We apply all three frameworks to a wide range of fuels produced from biomass and/or waste. The calculations are made for the climate impact only, since this is the impact category the three frameworks have in common (section Results). Finally, we analyse the case-study results and calculations to explain differences between the results in terms of methodological differences between the frameworks (section Discussion).
All underlaying assumptions and initial data are presented in Poulikidou et al. (2022). The full study, including inventoris and assessments of eight production pathways, is presented in a report (Poulikidou et al., 2022). This paper summarizes our findings as our goal is to compare different methods and to identify the source of their differences, which is novel. It includes assessments of the five production pathways (Table 1) with the greatest explanatory value. These include fuels produced from dedicated crops (3) and waste (2).
Materials and methods
An overview of the three frameworks analyzed and applied in this work: RED, PEF, and EPD can be found in Poulikidou et al. (2022; Table 2).
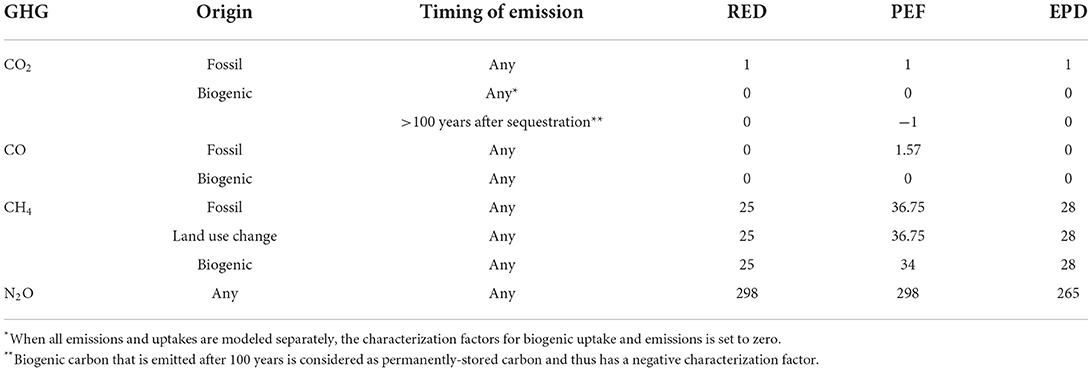
Table 2. GHG characterization factors in RED (EC, 2018a, p. 152), PEF (EC, 2018b, p. 70), and EPD (2019).
EU Renewable Energy Directive (RED)
The RED was established in 2009 and updated in 2018 as a regulatory framework to support the increased use of renewable energy in the European Union, while reducing emissions of GHGs (EC, 2018a). The Directive states that 32% of the gross final energy use in the year 2030 shall be renewable. It also includes a minimum share of renewable energy for each Member State from the year 2020. To count as renewable energy, and to be eligible for associated financial support, a fuel must meet several sustainability criteria, which are laid out directly in the directive, its annexes and supporting delegated acts.
The RED sustainability criteria include a minimum reduction of GHG emissions from the production and use of the fuel, compared to the fossil alternative. The required savings in GHG emissions for transport biofuels depend on when the plant in which they are produced was inaugurated. Newer plants have higher requirements: for plants starting operation from 1st January 2021, a minimum of 65% reduction is required, which is raised to 70% until the end of 2025, and to 80% thereafter.
The Directive includes default values for the climate impacts of common bio-based fuels and many production pathways (EC, 2018a, Annex V, Sections A, B; Annex VI, Section A). These can be used when the fuel is not associated with a negative climate impact from land-use change.
The Directive also includes rules for calculating the actual climate impact of fuels in a life-cycle perspective, distinguishing between transport biofuels and bioliquids (Annex V, Section C) and solid and gaseous biomass fuels (Annex VI, Section B). In this paper we refer to the methodology for calculating actual climate impacts of fuels in the updated directive from 2018: RED II.
Modeling approach, system boundary and functional unit
The methodology for calculating GHG emissions of individual fuels is essentially attributional: average data are used for modeling the production of, e.g., electricity used in the biofuel production, and environmental impacts of processes with multiple products are partitioned between these products. In other words, the methodology quantifies the share of the impacts of the electricity system and co-production processes that belong to the biofuel life cycle. This is typical for an attributional LCA (ALCA), which estimates the share of the global environmental impact that belongs to the product under study. A consequential LCA (CLCA), in contrast, estimates how the global environmental impacts are affected when the demand for the product is changed (Brandão et al., 2021).
RED II makes an exception from the attributional methodology for biogas produced from the anaerobic digestion of manure. In this case, it gives a credit of 45 g CO2eq/MJ for the avoided emissions arising from energy recovery instead of an alternative manure management. Accounting for avoided emissions due to avoided waste management is a consequential element in the calculations.
RED II guidance includes consequential elements that consider how a change in the production and use of a biofuel affects systems beyond the biofuel life cycle. For example, RED II considers it appropriate in a policy analysis to use the substitution approach, i.e., to expand the system to account for substitution when the production and use of fuels generate co-products (EC, 2018a, p. 99). The Directive also argues for a limited use of biofuels with a high risk for indirect land-use change (iLUC; see section Environmental impact assessment) with large climate impacts (ibid. p. 94). This aspect is further specified in a Delegated Regulation (EC, 2019).
In RED II, the GHG emissions of the fuel (E) are calculated with a life cycle perspective, where the functional unit is 1 MJ of fuel. The revised emission factor for the fossil fuel comparator is 94 g CO2-eq./MJ.
Co-production, recycling, reuse and energy recovery
The directive states that emissions of co-production processes are to be partitioned (i.e., allocated) between all co-products in proportion to their energy content, which is determined by their lower heating value (LHV). For cogeneration of electricity and heat, the allocation is instead based on the exergy (i.e., the useful part of energy that is able to do work) of the electricity and heat. Waste and residues from fuel production are not considered as co-products in RED II and thus have no upstream emissions (i.e., emissions taking place prior in the life cycle of the waste or residue being generated) allocated to them.
Consistently, fuels produced from waste and residues from forestry, agriculture and biomass processing are not assigned any GHG emissions from the processes or systems that generate these residues. The same holds for waste recycled from other life cycles. All waste and residues enter the fuel life cycle free from GHG emissions. The RED II calculations account, however, for emissions from the collection and further processing of the residues.
Environmental impact assessment
Since the RED framework targets GHG emissions, it only measures the impact on climate change, which is expressed as grams of CO2 equivalent (gCO2eq). The climate-impact calculation accounts for emissions of CO2, CH4, and N2O; their characterization factors are specified in the framework as shown in Table 2, based on IPCC's fourth Assessment Report (Myhre et al., 2013).
The RED II framework does not account for the sequestration of CO2 by energy crops. Similarly, CO2 emissions from fuel in use, e(u), is to be considered as zero for biofuels and bioliquids.
Emissions from direct land-use change (dLUC) and indirect land-use change (iLUC) are not insignificant in the carbon footprint of biofuels (Brandão et al., 2021). While dLUC refers to the GHG emissions that arise directly when land is converted from one use to another (e.g., from grassland or forest to arable land), iLUC refers to those emissions that arise as an indirect result of diverting food crops to biofuel; the market-mediated effects of which implying that LUC takes place elsewhere to compensate for the loss of food production.
LUC is modeled in the same manner between the RED II and EPD approaches, but differently in PEF. Indirect land-use change (iLUC), on the other hand, is only considered in RED II and is mutually exclusive to dLUC. While REDII considers iLUC, it is not part of the methodology for calculating GHG emissions of individual fuels. We included iLUC in the results as an illustration of the magnitude of its contribution to the results. The factors given in RED II for iLUC are applied: 12 gCO2eq/MJ for corn ethanol and 55 gCO2eq/MJ for oilseed rape FAME and HVO (EC, 2018a, p. 203).
Data quality
The directive states that primary data or a combination of data collected by the biofuel producer and default values shall be used in the calculation. Average data is allowed for estimating the average value for the GHG emissions from cultivation and stipulated for modeling the production and distribution of electricity in a defined region. When emission factors cannot be obtained from primary data, RED II has also provided typical values and default values for emission parameters, such as e(ec), e(p), and e(td). Typical values are representative values of the GHG emissions and emission saving for different types of biofuel, bioliquid or biomass fuel production pathway, consumed in the EU. Default values are derived from the typical values by applying pre-determined factors.
Product Environmental Footprint (PEF)
The Product Environmental Footprint or PEF was developed by the European Commission and provides a common and harmonized methodology for quantitatively assessing and communicating the environmental impacts of products and services at a European level (EC, 2012). In order to conduct a PEF, a PEFCR is required, which provides the rules for performing a PEF for a specific product category. However, the PEF framework has not been fully developed yet. Between 2013 and 2018, the framework was under a pilot phase, when more than 280 companies were voluntarily testing it and developing specific rules for their products or services. PEF is currently undergoing a transition phase, before the policies regarding the implementation of PEF can be adopted.
The PEF framework is described in general in the PEF Guide (EC, 2012). An updated and elaborated methodology is presented in the guidance for developing PEFCRs (EC, 2018b). Zampori and Pant (2019) suggest further updates of the methodology. However, no PEFCR for biofuels exists yet. In this paper we refer to the methodology in the PEFCR Guidance from 2018. The parts of the methodology that are important for our study are essentially the same as suggested by Zampori and Pant (2019).
Modeling approach, system boundary and functional unit
The modeling approach in the PEF framework can be described as a combination of attributional and consequential LCA. The framework allows for the use of average data, which is consistent with ALCA and, in parallel, encourages the use of substitution for handling co-production (see section Modeling approach, system boundary and functional unit), which reflects a consequential approach.
The PEF framework defines the system boundary as being from cradle to grave, i.e., including the stages of raw material extraction, processing, production, distribution, use and the end-of-life treatment of the product.
In PEF, the functional unit is termed “unit of analysis.” The unit of analysis defines the function(s) and duration of the product, both qualitatively and quantitively. As stated, it answers the questions of “what function,” “how much,” “how well,” and “how long” (EC, 2018b, p. 44). The functional unit in PEF is 1 MJ for biofuels.
Co-production, recycling, reuse and energy recovery
The PEF guidance on how to model multifunctional processes is an interpretation and modification of the allocation hierarchy of the International Standardization Organization (ISO, 2006b; EC, 2018b, pp. 61–64):
• Avoid allocation through subdivision or system expansion. Subdivision indicates that multifunctional activity is divided so that input flows associated with each process output can be separated. The PEF framework interprets system expansion to mean the inclusion of additional functions so that a system with multiple functional outputs is assessed.
• If allocation cannot be avoided through subdivision or system expansion, then allocation based on a relevant underlying physical relationship should be applied. The PEF framework modifies this part of the hierarchy by stating that physical allocation can also be modeled through direct substitution if such substitution can be verified empirically. For example, manure applied on land can directly substitute an equivalent amount of a specific fertilizer nitrogen.
• Allocation based on other relationship can be applied as a third option, e.g., based on economic values. Here, the PEF methodology includes indirect substitution as an additional option where the co-product is assumed to replace an average equivalent product on the market.
The notion that allocation can be modeled through substitution is not just a deviation from ISO (2006b) but also from the common notion that substitution is an approach to avoid allocation.
For product life cycles that involve recycling and/or energy recovery, the PEF uses the so-called Circular Footprint Formula (CFF) for allocating burdens to previous and subsequent life cycles. The CFF can be divided into three parts: material production and recycling, energy recovery, and waste disposal:
The parameters used in the CFF include the allocation factors A and B, the quality of primary and secondary material (Qp, Qsin, and Qsout), the recycled content of the product (R1), the proportion of material going to recycling or energy recovery (R2 and R3), specific emissions from and resources used in various processes and subsystems (Ev, E, Erecycled, ErecyclingEoL, EER, ED), the efficiency of the energy recovery process for heat and electricity (XER, heat and XER, elec) and lastly the lower heating value (LHV) of the material in energy recovery.
The A factor for dividing burdens and credits between supplier and user of recycled materials is predefined for different types of products and can have values of 0.2, 0.5, or 0.8. For products with unspecified A factor, 0.5 shall be used. Factor B is zero, which means that all burdens and benefits of energy recovery are assigned to the product generating waste for energy recovery. For consistency, the recovered energy is given the same burdens as the primary energy it substitutes (i.e., substitution approach).
More details on the definitions of each parameter can be found in the PEFCR Guidance v.6.3 (EC, 2018b, pp. 113–130).
Environmental impact assessment
The PEF framework includes 16 environmental impact categories, for example climate change indicated by GWP100 (kg CO2eq). This particular impact is divided into three sub-categories, which should be separately reported if important to the PEF results (EC, 2018b, pp. 65–69):
• fossil: GHG emissions from oxidation or reduction of fossil substances through, e.g., combustion, digestion, landfilling.
• biogenic: carbon emissions to air (CO2, CO, and CH4) from aboveground biomass through oxidation or reduction and CO2 uptakes from the atmosphere via photosynthesis.
• land use and land transformation: carbon uptakes and emissions (CO2, CO, and CH4) arising from changes in carbon stock via land use and land use change. This includes biogenic carbon exchanges from deforestation, road construction, soil carbon emissions, and other soil activities.
The characterization factors for the GHGs are adapted from the IPCC 2013 (Myhre et al., 2013), where the factor for fossil methane is corrected by taking into consideration that in a longer time horizon, all of the fossil methane is converted into CO2. Myhre et al. (2013) account for the conversion into CO2 for biogenic metane only (see Table 2).
LUC is modeled differently in PEF compared to the other two approaches, as the LUC here includes the marginal crops that balance DDGS or rapeseed cake. ILUC is not considered in this approach.
Data quality
In general, company-specific data should be used whenever possible, especially for the foreground system, which represents activities that are under the direct influence of the decision maker. Generic data can be used and should be used only for background system, which represents activities that are not under the direct influence of the decision maker (e.g., fertilizer manufacture is not under the direct influence of a farmer).
When company-specific data are used, the electricity shall be modeled according to the following hierarchy: (1) Supplier-specific electricity product; (2) Supplier-specific total electricity mix and (3) “Country-specific residual grid mix, consumption mix”—data available from Sphera (2022).
Environmental Product Declaration (EPD)
The EPD is a Type III environmental declaration, compliant with the ISO 14025 standard (ISO, 2006c) that, in turn, rests on ISO 14040/14044 (ISO, 2006a,b). It provides independently-verified environmental information for products or services based on LCA.
An EPD is performed voluntarily by a company and the information is used primarily for business-to-business communication. There are several programme operators that publish EPDs and the framework's methodology can sometimes vary among operators (Del Borghi, 2013; Hunsager et al., 2014; Minkov et al., 2015).
In this study, the framework followed is described in the General Programme Instruction (GPI) documentation of the programme operator EPD International (EPD, 2019). In addition, an EPD normally follows a PCR, which contains specific guidelines and rules for a specific product type. A PCR for biofuels has not yet been developed, but the general method description provided by the GPI is applied to the case studies in section Results, unless otherwise stated. We have used GPI Version 3.01 (EPD, 2019) but refer also to Version 4.0 (EPD, 2021), which was developed during the course of our study.
Modeling approach, system boundary and functional unit
The default methodology in the EPD framework is attributional. An EPD normally has a cradle-to-grave perspective. However, an EPD of a material or component that is used in the production of other products can be an information module that accounts for only part of the life cycle of the material or component (ISO, 2006c, p. 5), for example its production, or its production and waste management. All relevant processes should be included in the information module to prevent the loss of information at the assessment of the final product.
The International EPD® System divides the life cycle of a product into three stages: (1) Upstream processes—from cradle-to-gate; (2) Core processes—from gate-to-gate; and (3) Downstream processes—from gate-to grave. This division is made because each stage has different data quality rules, and to facilitate clear the presentation of results. Furthermore, this approach supports the modularity required to combine information modules and EPDs into EPDs for other products (ISO, 2006c, p. 5).
The choice of functional unit in the EPD framework is specified in the PCR. If a full life cycle is not represented or the function of the product is unknown, a so-called “declared unit” can be used in lieu of the functional unit. A declared unit is defined as a quantity of the product related to the typical use e.g., 1 kg, 1 m, or 1 m3 of a product (EPD, 2019, p. 52). In an EPD of a fuel, 1 MJ can be both declared unit and functional unit.
Co-production, recycling, reuse and energy recovery
The EPD (2021, p. 66) framework includes an allocation procedure that is similar to ISO (2006b), except for system expansion:
• When possible, allocation shall be avoided through sub-dividing the processes, so that the input and output data related to the sub-processes can be obtained. System expansion according to the ISO14044 is not allowed due to the nature of the framework being strictly attributional.
• When allocation cannot be avoided, a partitioning of input and output to different products or services shall be done based on their underlying physical relationship.
• If allocation based on a physical relationship cannot be applied, partitioning based on another relationship is also possible. A sensitivity analysis needs to be performed when economic value is used as a basis for allocation.
When the product system involves reuse, recycling or energy recovery, the EPD applies the Polluter Pays Principle (PPP) to separate the impact of the secondary material from its previous life cycle. The PPP indicates that the polluter or the waste generator carries the full environmental impact of the product system, including the reuse/recycling or recovery activity as well as its transport. The impact from the processes that occur subsequently, i.e., after the point where waste is processed for reuse, recycled, or recovered (as energy), is then attributed to the subsequent product/life cycle (cf. EPD, 2021, pp. 66–67).
Environmental impact assessment
The EPD (2022) framework includes seven default environmental impact categories in addition to global warming.
GWP over 100 years (GWP100) is used to indicate the climate-change impact category. The GWP100 shall be calculated according to the CML 2001 baseline, version 2016, which was originally from IPCC 2013 (Myhre et al., 2013). EPD requires four types of GHG emissions and removals to be reported, which are based on their origins: fossil, biogenic, land use and land use change (LULUC) and total. The total is the sum of the three aforementioned types of GHGs. The characterization factors of the GHGs are indicated in Table 2.
The emissions and removals of GHGs from human food and animal feed that are associated with the ingested part of the product are excluded. GHG emissions (except CO2) originating from the degradation food/feed waste and enteric fermentation are included. When a secondary material is used, the stored carbon that enters the system boundary of the second product is accounted for as a primary material.
LUC is modeled in the same manner as the RED II approach. ILUC is not considered in the EPD approach.
Data quality
Specific data are to be used whenever possible. For the core activities, it is mandatory to use primary data. Generic data can be used for upstream and downstream when specific data are not available. The generic data used should be representative for the activity in question. Electricity shall be modeled in the following order: (1) with specific electricity mix as generated or purchased; (2) with national residual electricity mix; and (3) with national electricity production mix or electricity mix on the market.
Results
Five case studies (see Table 1) were modeled using the three frameworks: RED II, PEF, and EPD. Within each case study, the same activity data were used for the assessment. Case-study details on the system boundaries, key input data, assumptions, multifunctional activities, and rules for material recycling, reuse and energy recovery are summarized in this section, while further details and further case studies can be found in Poulikidou et al. (2022).
Biofuels from dedicated crops: Ethanol from corn, FAME and HVO from oilseed rape
Ethanol is a gasoline substitute that can be made from sugar and starch crops. Corn is the most common feedstock in the USA [Renewable Fuels Association (RFA), 2021]. FAME and HVO can be used as a diesel partial substitute in vehicles. FAME can be produced via esterification, and both FAME and HVO can be produced from various vegetable oils and fats which contain triglycerides and fatty acids. Rapeseed oil is the dominant biodiesel feedstock in the European Union, accounting for around half of the total production (Flach et al., 2019). In this study, we have modeled the cultivation, transport and storage, processing, as well as the direct and indirect land use change phases of the life cycle of the ethanol, FAME and HVO.
The cultivation, processing, transport and storage phases are considered equivalent across the RED II and EPD approaches. The impact from the use stage is set to zero, as biogenic CO2 emissions are assumed to be balanced with the sequestration of CO2 as the feedstocks grew. We allocate based on the energy content of co-products whenever these are generated: dried distillers' grains with solubles (DDGS) are a co-product of ethanol produced from corn, while rapeseed cake and glycerol are a co-product of rapeseed oil. However, when the PEF approach is applied, we use the option it offers to model/avoid allocation through substitution. The DDGS and rapeseed cake are both used as animal feed, where they substitute a mix of soybean meal and barley, which are considered to be the marginal sources of feed protein and feed energy, respectively. Avoided soybean meal results in the reduced production of soybean oil, which in our model is replaced by palm oil, following an algorithm similar to the approach adopted by Dalgaard et al. (2008) and Schmidt and Weidema (2008). Accounting for these indirect effects is an option only in the PEF approach.
Figure 1 shows the system boundaries applied to the three case studies on (i) corn ethanol, (ii) rapeseed oil FAME, and (iii) rapeseed oil HVO.
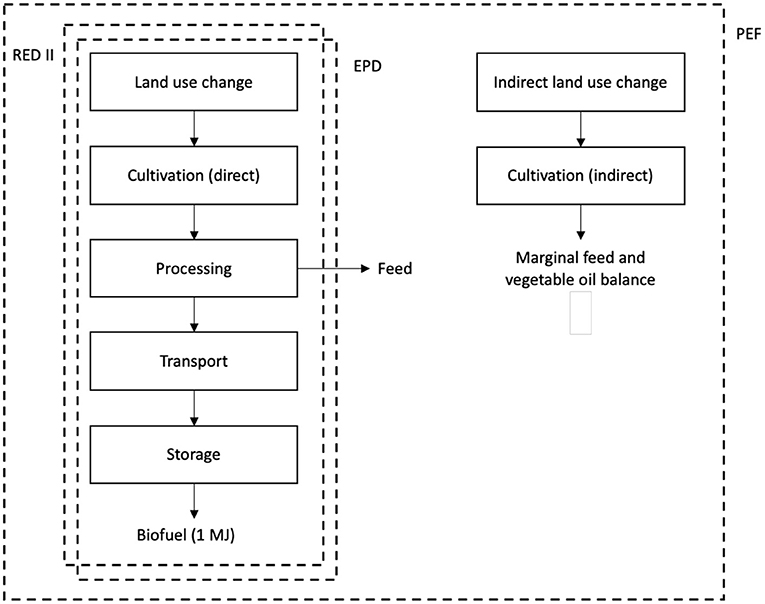
Figure 1. System boundaries in relation to the methods applied: RED II, EPD, and PEF. RED II and EPD exclude by-products and their fate by applying energy allocation, while PEF allows for the substitution approach and considers changes in the cultivation and LUC of the marginal crops affected by the DDGS or rapeseed cake, which is used as an animal feed. As a result, the balancing of the feed and vegetable oil markets is included in our PEF calculations.
The updated data from BioGrace found in Brandão et al. (2021) were used as inventory data. The processing of corn into ethanol at the ethanol plant results in the co-production DDGS. The energy content of the co-products give an allocation factor of 54.6 and 45.4% to ethanol and DDGS, respectively, when allocation by energy is applied. The extraction of oil from rapeseed results in two co-products: crude vegetable oil and rapeseed cake (which also can be used as animal feed). Their energy-based allocation factors are 61.3 and 38.7%, respectively. The processing of rapeseed oil into FAME at the esterification stage results in the co-production glycerol. Applying energy allocation gives a factor of 95.7 and 4.3%, respectively, to FAME and glycerol.
Figure 2 illustrates the results calculated by applying the different frameworks and elucidates the differences between these.
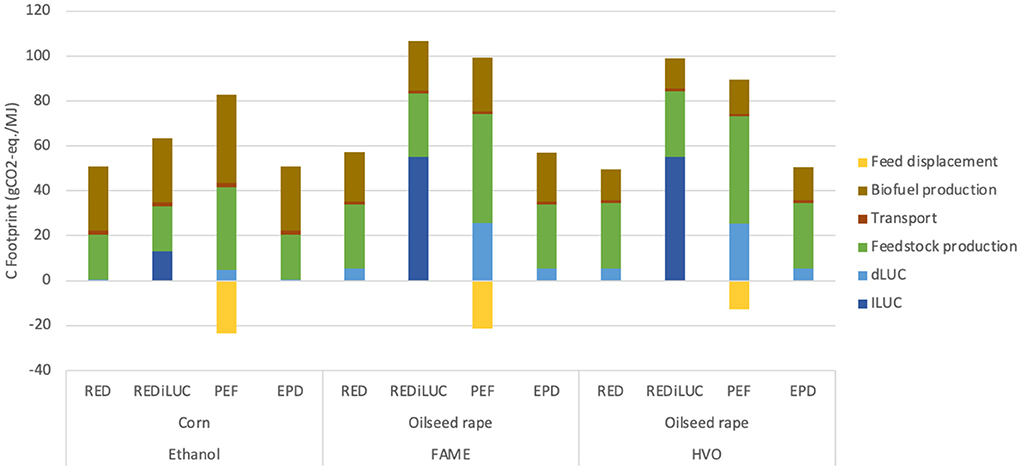
Figure 2. Carbon footprint of corn ethanol, oilseed rape FAME, oilseed rape HVO, as modeled according to RED II, PEF, and EPD.
Biofuels from waste
HVO from used cooking oil
In addition to vegetable oils, HVO can be produced from a wide range of by-products, such as animal fats from slaughterhouse wastes or from used cooking oil (UCO). This case study represents HVO from UCO, which is assumed to be collected within Sweden.
The production of the HVO uses hydrogen to treat UCO in two stages. Firstly, the UCO, which contains triglycerides, is saturated and then treated further to produce fatty acids. In a second stage oxygen is removed from the fatty acids, with or without hydrogen, to produce the paraffinic hydrocarbons which is the HVO (Jogner and Nojpanya, 2021). The production processes produce propane, excess steam and a small amount of methane as co-products.The propane is a marketable by-product. However, in this case study both propane and methane are used as fuel gas in the HVO production process. Thus, excess steam was the only co-product considered. The produced HVO is shipped to the five major depots in Sweden and then distributed to smaller fuel stations. The life cycle considered includes: (i) collection of UCO; (ii) pre-treatment of UCO; (iii) transport of UCO to the HVO production site; (iv) HVO production; (v) transportation and distribution; and (vi) combustion of HVO in a heavy-duty truck (class EURO 5). The system boundaries in relation to different frameworks applied to the case study on HVO are shown in Figure 3. Site-specific data are used for the HVO production. Generic and average data, obtained from scientific literature databases and published company reports, are used for most other parts of the system.
Since UCO is used as feedstock to produce HVO, the product system involves material/waste recycling. The RED II and EPD frameworks consider the UCO as waste, while the PEF framework considers it as a secondary material. The PEF approach treats recycled material by applying the CFF. As a result, the application of the three frameworks yield different system boundaries: the PEF framework includes the substituted virgin material (as part of the CFF formula);. the EPD framework excludes the collection of UCO. Common to all approaches, no upstream emissions prior to the point where UCO is collected are included. Hence, the production and use of virgin cooking oil are excluded from the calculations in all frameworks.
Useful steam is produced in excess in the HVO production. As stated in section Materials and methods, the three frameworks allows for different approaches for handling multifunctional processes. We used the substitution approach in the PEF calculations. The excess steam is assumed to substitute steam produced in a boiler fuelled by natural gas. In the RED II and EPD calculations we applied energy allocation, resulting in an allocation factor of 0.94 and 0.06 to HVO and steam, respectively.
Figure 4 illustrates the results calculated by applying the different frameworks and elucidates the differences between these.
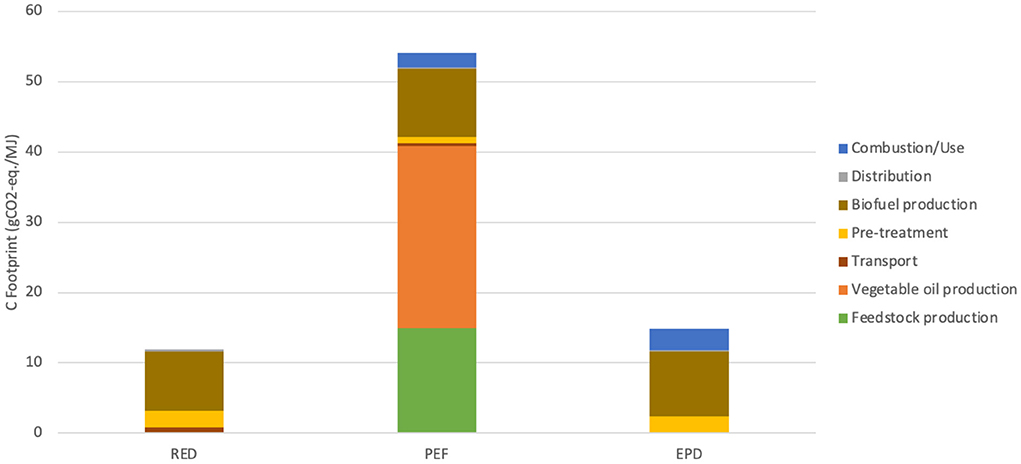
Figure 4. Carbon footprint of UCO HVO, as modeled by RED II, PEF, and EPD. The CFF results includes part of the climate impact of virgin oil production.
Biogas from food waste
This case study represents biomethane produced from biogas via anaerobic digestion of municipal food waste from households. The life cycle includes: (i) collection of food waste from households; (ii) transport of food waste to biogas plant; (iii) pre-treatment of food waste; (iv) anaerobic digestion of food waste to biogas and digestate; (v) upgrade of biogas to vehicle fuel (biomethane); (vi) distribution of biomethane to gas station; and (vii) combustion of biomethane in a passenger car. Figure 5 shows the system boundaries in relation to the different approaches applied.
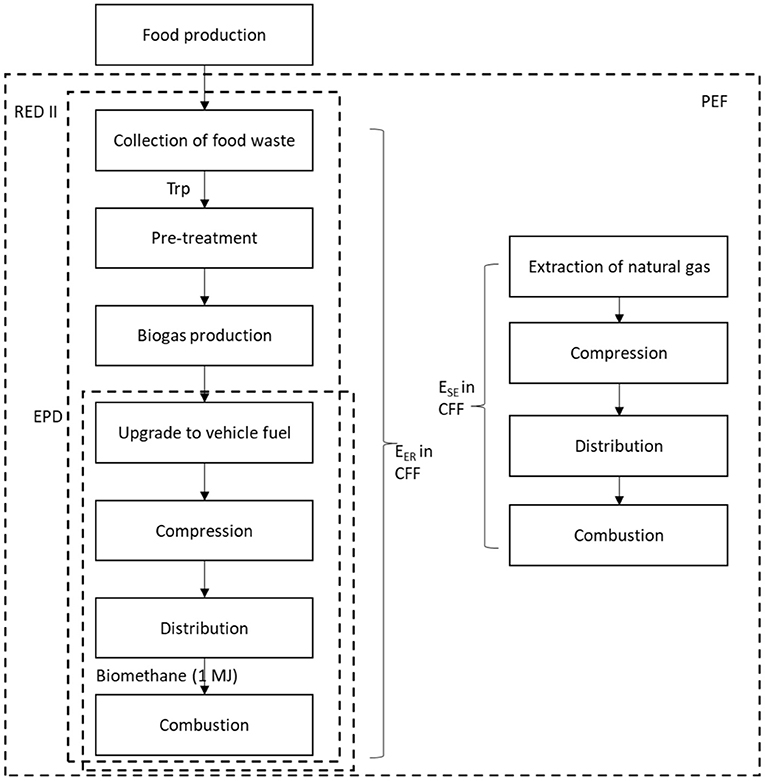
Figure 5. System boundaries in relation to the approaches applied; RED II, PEF, and EPD. CO2 emissions from combustion are excluded in RED II, while non-CO2 GHGs (i.e., CH4 and N2O) are included. An alternative interpretation of the CFF excludes the combustion and possibly the distribution from EER and ESE.
The calculation rules in RED II (EC, 2018a, p. 185) state that emissions of N2O and CH4 from the use of biomass fuels in terms shall be included in the calculations. Biomass fuels are defined as gaseous and solid fuels from biomass. Consistent with this rule, we included the combustion of the biogas in the RED II system (Figure 5). This contrasts with the calculation rules for liquid biofuels, where RED II (EC, 2018a, p. 154) excludes the use phase from the calculations.
As currently there are no PCRs for biogas production within EPD International, we instead interpreted and applied the broader set of rules in the General Programme Instructions (GPI, EPD, 2021). In addition, we consulted the existing PCR for electricity, steam and hot water that specifies how to model biogas production for electricity generation (EPD, 2020). This PCR states that when biogas is produced from waste through anaerobic digestion, the burdens of the digestion plant shall be allocated to the life cycle generating the waste. Consistent with this rule, we excluded the digestion plant from the EPD calculations on biogas (see Figure 5).
Similarly, we used the general PEF 6.3 guidance, since no PEFCR exists for biofuels. The general PEF guide considers biogas from anaerobic digestion a form of energy recovery (EC, 2018b, p. 125). This means Equation 2 applies to the PEF calculations on biogas. This equation gives a credit to the food products generating the waste for environmental burdens avoided when the recovered energy substitutes another energy source. To avoid double-counting of the environmental benefits of energy recovery, the PEF rules (ibid. p. 114) require that the recovered energy be modeled as the primary energy substituted by the biogas. If a PEF of the food product assumes that the biogas substitutes production and use of natural gas, the biogas must be modeled as production and use of natural gas (ESE in Figure 5) rather than as biogas (EER).
The PEF calculations depend on how the point of substitution is defined, because they include a credit for avoided processes up to the point of substitution only. If the food PEF defines the point of substitution as the point where biogas is fed into the gas grid, it includes substitution of the production of natural gas but not substitution of the use of natural gas. Consistently, the life cycle of the biogas should be modeled as production of natural gas and distribution and use of biogas.
The general PEF guide allows for both these interpretations. For this reason, we make two PEF calculations for biogas: one where the production and use of biogas is modeled as production and use of natural gas, and one where just the production of biogas is modeled as production of natural gas. In the former case, the biogas is assigned the same climate impact as natural gas (see Figure 6).
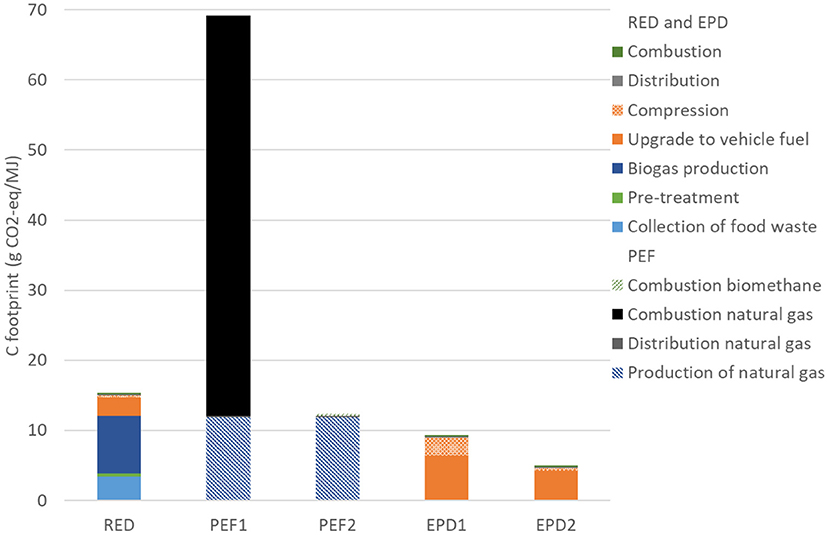
Figure 6. Carbon footprint of food-waste biogas, as modeled by RED II, PEF, and EPD. PEF results are calculated under the assumption that the biogas substitutes the production and use of natural gas (PEF1, tall bar) or only the production of natural gas (PEF2), EPD results are calculated with two sets of electricity data representing the residual mix for the Nordic region (EPD1) and Sweden (EPD2), respectively.
The application of the three approaches resulted in a markedly different system boundaries, which affects the parts of the life cycle that are considered to be part of the biogas/biomethane product system, as opposed to those considered part of the food system (see Figure 5).
Primary data sources include Börjesson et al. (2016), ecoinvent (Wernet et al., 2016) and Sphera (2021). To investigate the significance of electricity data, the EPD calculations are made with two sets of electricity data: average data for the Nordic countries (EPD1 in Figure 6), and average data for Sweden (EPD2).
RED II applies allocation according to the lower heating value (LHV) of co-products. Two products are produced in this case study: biogas and digestate. Digestate is used as a fertilizer and is thus an important product from the system to recover the nutrients in the food waste but since the digestate has a high water content the LHV is 0 and thus all emissions are allocated to the biogas. Both RED II and EPD apply the cut-off method for the modeling of energy recovery (and material recycling), while PEF uses the CFF (EC, 2012, 2018a; EPD, 2019).
Figure 6 illustrates the results calculated by applying the different frameworks and elucidates the differences between these.
Discussion
It is outside the scope of this paper to identify the best approach. Our aim was simply to identify and quantify the differences between approaches. However, we can summarize the differences between the different approaches, relative to three important aspects, as follows:
• Estimating environmental consequences: PEF is the framework that best reflects the foreseeable consequences.
• Consistency of methods: EPD is fully attributional; RED II is mainly attributional (e.g., biogas from manure is an exception); PEF is a mix of CLCA and ALCA.
• Reproducibility and robustness: RED II is reproducible because the methods are specified in detail; EPD needs a PCR before it can reach the same level of reproducibility; PEF would need both a PEFCR and further development of the general guidelines before it can be considered to be reproducible. No particular approach is considered robust in estimating the climate-change impacts of producing and consuming biofuels.
Differences in results
The application of the frameworks to the biofuel case studies illustrates that the results can vary widely between the three frameworks, and between different interpretations of an individual LCA framework. The most drastic difference in our results—a factor six—is between the two PEF calculations on biogas (Figure 6), where the climate impact of the fuel use depends on how the CFF is applied. The second largest difference is in the calculations on HVO produced from UCO (Figure 4), where PEF indicates a much higher climate impact compared to the other two frameworks. This is because the CFF brings part of the climate impact of the primary production of substituted crops and vegetable oil into the PEF calculations.
Other differences in total results are less dramatic, but still important. Our EPD calculations for biogas exclude collection of food-waste, pretreatment, and the digestion process (Figure 5). As a consequence, the EPD results indicate much less climate impact compared to RED II (Figure 6). The total results from RED II can vary by a factor two depending on whether the calculations include the direct or indirect land-use change (dLUC or iLUC in Figure 2).
Even when the difference in total results is not large, significant differences can occur in the modeling of subsystems. Production of crops affects the climate much more in our PEF calculations, compared to the other frameworks; on the other hand, the PEF calculations include a climate benefit from feed displacement (Figure 2). The biogas results are dominated by different subsystems, depending on what framework is applied (Figure 6).
Most of these differences are further explained and discussed below.
Modeling of land-use change
The RED II, PEF, and EPD frameworks diverge in the methods applied for modeling land use. This can be very important for the assessment results when the biofuel is produced from crops such as rapeseed and corn.
RED II emphasizes the risk that the use of biofuel produced from crops can result in the extension of agricultural land into forests, wetlands and peatland, causing additional greenhouse gas emissions (EC, 2018a, p. 94). For oil-rich crops such as rapeseed, they estimate the climate impact of indirect land-use change (iLUC) to be 55 g CO2 eq per MJ of fuel (ibid. p. 203). If this impact were included in the calculations, RED II results would indicate that RME and HVO produced from rapeseed oil have a climate impact of approximately 100 g CO2-eq./MJ (see Figure 2). This is greater than the impact of the fossil fuel comparator, which is 94 g CO2-eq./MJ (EC, 2018a, p. 155).
However, the iLUC impacts are highly uncertain. The RED II framework for calculating the climate impacts of individual biofuels excludes these uncertain impacts, but instead includes direct land-use change (dLUC; EC, 2018a, pp. 179, 184). The same holds for PEF and EPD. When iLUC is not accounted for, the crop-based biofuels in our study have less climate impact than fossil fuel. RED II and EPD calculations without iLUC lead to quite similar results for the crop-based fuels (Figure 2). Our PEF results are higher, mainly because of how we model the multifunctional processes (see section Modeling of multifunctional processes).
The PEF calculations also indicate a greater climate impact from the cultivation, but this is off-set by a climate benefit from indirect climate benefits of cultivation. This is because PEF includes in the system boundary the land required and displaced by balancing the feed and vegetable oil markets as a result of additional feed co-produced with biofuels.
Modeling of multifunctional processes
The RED II, PEF, and EPD frameworks diverge in the approach to modeling co-products. In particular, the PEF framework allows for system expansion with substitution (EC, 2018b, pp. 61–64), which is prohibited in the two other frameworks. This can very be important for the total result when the biofuel has co-products. In our calculations, DDGS is a coproduct of ethanol produced from corn, rapeseed cake is a co-product of rapeseed oil, etc. We applied system expansion with substitution in our PEF calculations of crop-based fuels, where DDGS and rapeseed cake are both used as animal feed. In our model, this reduces the production of soybeans and barley but increases the production of palm oil. Since palm oil has a large climate impact, the system expansion made the PEF results significantly higher, compared to RED II without iLUC and compared to the EPD results.
However, PEF allows for a free choice between allocation and substitution. This means that PEF calculations do not necessarily diverge from EPD and RED II calculations on this point. The free choice between allocation and substitution contributes to making it difficult to compare results from different PEFs of biofuel. The PEF calculations could be made more reproducible through the development of PEFCR for biofuel that stipulates how the most common or important multifunctional processes should be modeled.
Even if the EPD framework prohibits substitution, it allows for a broad range of allocation approaches. This can contribute to making comparisons difficult between different EPDs of biofuel. The EPD calculations can also be made more reproducible through the development of a PCR for biofuel.
The RED II methodology is more well-defined than PEF and EPD, because it stipulates what method should be used: allocation should be based on the energy content of products, except for the co-production of electricity and heat where allocation should be based on the exergy.
Modeling of waste management
The RED II, PEF, and EPD frameworks diverge in the methods applied for modeling waste management. This can be very important for the assessment results when the biofuel is produced from by-products or residues. The main difference between by-products and residues is that the former has economic value while the latter does not. In the case of co-production, the process under assessment is considered a multi-functional process and therefore the inputs or emissions linked to that process need to be handled so as to isolate the product of interest. RED II defines “residue” as “a substance that is not the end product(s) that a production process directly seeks to produce; it is not a primary aim of the production process and the process has not been deliberately modified to produce it.” EPD and PEF differentiate co-products and residues in the same manner. Waste can be defined as the substances or flows which the holder intends or is required to dispose (PEF guide). Similarly to residues, wastes are not considered an intended outcome of the production process.
One important difference is that the PEF framework models the supply of recycled material as part recycling and part primary production (cf. Equation 1). When primary production has much more climate impact than recycling, this can make the PEF results indicate a much higher climate impact compared to the other frameworks. This is the main reason why PEF results are higher than RED II and EPD results for HVO from UCO (see Figure 4).
A PEF of a fuel produced from waste includes a model of primary production up to the point of substitution, i.e., the point where the flow of recovered material displaces a flow of primary material. This point is also where the quality ratio of the secondary material relative to the primary (QS/QP) is estimated. Hence, the point of substitution can be important for the PEF results. The PEFCR guide (EC, 2018b, pp. 115–116) explains how to identify this point when a product is produced from a mix of recycled and primary material. However, when a fuel is produced from waste only, the point of substitution is difficult to identify. For HVO from UCO, several options are possible in the PEF model.
Our calculations assume that the pretreated UCO substitutes virgin vegetable oils as input to the HVO production. In this case, available price data suggest that the quality ratio QSin/QP is nearly 1 (Poulikidou et al., 2022). Since the allocation factor A is 0.5, this means that the PEF of the HVO produced from UCO includes nearly half the burdens of virgin oil production, plus approximately half the impacts of collecting and pretreating UCO.
The HVO produced from UCO might instead substitute HVO produced from primary raw materials. Since these fuels compete on the same market, they are likely to have similar price, and the quality ratio QSin/QP will be at or near 1. This means that the PEF of the HVO produced from UCO will include half the burdens of recycled HVO production and half the impacts of virgin HVO production. With this point of substitution, the PEF result depends on the climate impacts of HVO production from UCO and directly from vegetable oils.
The purpose of producing HVO from UCO is to substitute fossil fuel. If the point of substitution is at the tank station, the PEF of the HVO will not include any production of virgin vegetable oil or HVO. Instead, it will include half of the production and distribution of fossil diesel (as long as the HVO is not cheaper than the fossil diesel). Production and distribution of diesel has little climate impact, compared to the impact of producing the vegetable oils in our study: a mix of rapeseed and palm oil (see Figure 3). Hence, the PEF result will with this point of substitution be closer to the RED II and EPD results than the PEF result in Figure 4.
Finally, transportation based on HVO might substitute transportation based on fossil fuel. The cost and quality of transportation is not likely to be noticeably lower when HVO is used as fuel in the vehicle. This means the PEF model of the HVO will include half the impacts of producing and using recycled HVO and half the impacts of producing and using the fossil fuel. This will increase the climate impact indicated by the PEF to approximately the level indicated in Figure 4.
Similar options for the point of substitution are open for biogas and other waste-based fuels. For biogas, the point of substitution is vital for the PEF results. When transportation with biogas substitutes transportation with natural gas, the production and use of biogas is modeled as production and use of natural gas. This is because the PEFCR guide redards biogas production as a case of energy recovery (EC, 2018b, p. 125) and states that recovered energy should be modeled as the primary energy it substitutes (ibid., p. 114). As a result, biogas is assigned the same climate impact as natural gas. If the point of substitution is instead when the biogas is fed into the gas grid, or at the tank station, the PEF calculations include the production of substituted natural gas but the use of biogas. This makes the total PEF results similar to the results obtained when applying RED II (see Figure 6).
Our biogas results obtained with the EPD framework are lower still (Figure 6). This is because emissions from the anaerobic digestion process were not included (cf. Figure 5).
Modeling of electricity
The RED II, PEF, and EPD frameworks diverge slightly in how electricity production is modeled. RED II stipulates that electricity bought from the grid should be modeled to reflect the average emissions from “the production and distribution of electricity in a defined region” (EC, 2018a, p. 154).
Version 3.01 of the General Programme Instructions (GPI) of EPD International stipulates that electricity production should be modeled using data for a supplier and technology if the origin can be guaranteed (EPD, 2019, pp. 57–58). In other cases, data reflecting the residual mix in the country or market should be used, if possible. When data on the residual mix cannot be found, data reflecting the total production mix in the country or market should be used. The recent Version 4.0 of the GPI, stipulates the use of residual or consumption mix on the markets, and does not allow for the use of data reflecting the national or production mix (EPD, 2021, pp. 64–65).
The PEFCR guide (EC, 2018b, pp. 89–94) states, similar to the EPD GPI, that electricity production should be modeled using data for a technology or a supplier if the origin can be guaranteed. In other cases, data reflecting the national residual consumption mix should be used. However, for electricity used in Sweden national data are replaced by Nordic data (EI, 2021).
There is room for interpretations in the guidance on electricity modeling. When RED II is applied, for example, decisions must be made on how to define the region and on whether to use data on the production or consumption mix in this region. The EPD GPI leaves the question open how the market should be defined: if electricity is used in Sweden, is the market national or Nordic, or does it include a wider range of European countries? The definition of the market is currently discussed within EPD International and European Standardization. It might be clarified in coming versions of the EPD GPI and/or in a PCR for biofuel.
In the case the market is defined to be Nordic, EPD and PEF give quite similar guidance on how to model the supply of electricity used in Sweden.
The climate impact of electricity production can vary significantly depending on what data are used. The choice of electricity data can be important for the assessment results when other climate impacts in the system are very small. In an EPD of biogas, for example, the results are dominated by emissions from production of electricity used in the upgrading of the gas, because the emissions from digestion are excluded from the EPD calculations. In this case, the choice between electricity data representing a Nordic residual mix (EPD1 in Figure 5) or the Swedish residual mix (EPD2) is important for the total results.
The RED II calculations are here based on the average Swedish consumption mix, which has very little climate impact. This means the upgrading of the biogas affects the climate much less according to RED II, compared to an EPD (Figure 5). The total results from the RED calculations are still higher than the EPD results, because RED II includes methane leakage from the digestion process.
In most of our calculations, however, the climate impact of the fuel is not significantly affected by the choice of electricity data, because the production of most of the biofuels requires little electricity. The choice of electricity data can be more important for other fuels, especially synthetic fuels (such as bio-electro-fuels) or hydrogen produced with electricity.
Conclusions and recommendations
As the LCA field develops and other sectors tend to customize their LCA tools and modeling approaches, fuel producers may need to provide input data and calculation results that are specifically adapted to certain LCA frameworks. Our study focusses on three different frameworks that can be used for estimating the environmental performance of products, and in particular of transport fuels: RED II, PEF, and EPD. It is clear that the different frameworks have different scopes and can be used for different purposes.
The RED II methodology is commonly applied and highly linked to regulatory measures in the EU. The scope of RED, however, is limited to GHG emissions. With increased need for holistic approaches and to avoid burden-shifting between different environmental impacts, additional categories should be included; thus, the need for applying a broader LCA framework increases.
Based on the work performed in this study and the results obtained, it can be concluded that applying all three frameworks is not a straightforward task. The associated approaches include fundamental differences and have different levels of maturity and adoption. Their application can lead to conflicting results, thus influencing decision making in opposing directions. Understanding the differences and underlying assumptions can be important for understanding the variations in outcome.
Moreover, this study confirms that applying a framework like PEF or EPD in addition to RED II would require significant additional efforts—not only because of different rules (which were often contradicting or difficult to interpret) but also because of additional data and reporting requirements. The need for expertise and resources is increasing for fuel producers to be able to provide EPD- and PEF-compliant assessments.
The results obtained for a specific fuel could differ substantially depending on the framework applied and the assumptions and interpretations made when applying this framework. Certain methodological parameters were identified to have a greater impact on the results than others. In summary, the three frameworks diverge in how LUC and waste management are modeled. This is important for the results when the fuel is produced from waste. In addition, the frameworks diverge in what approaches are allowed for modeling processes with multiple products. This can be very important for the results when the fuel is co-produced with other products. Finally, the frameworks also diverge in how the electricity supply is modeled. As opposed to the previous points, this is not very important for the results in our case studies because the production of these biofuels does not require a significant amount of electricity.
Attributional LCA has been critizised for not accounting for iLUC. Our RED II calculations confirm that results are indeed very sensitive to LUC considerations, particularly when modeling dediated crops. Neither EPD nor PEF include iLUC (only dLUC). Sseveral other frameworks for assessing the climate-change impacts of biofuels do include iLUC, for example, CORSIA (ICAO, 2020), the US Renewable Fuels Standard (U.S. Government, 2005), and the California Low-Carbon Fuel Standard (CARB, 2015).
The PEF guidelines, in particular the CFF that guides the modeling of material production and waste management, proved to be challenging to interpret. The CFF is designed to give clear and specific methodological guidance, and the PEFCR guide gives support in much of the interpretation of the formula (EC, 2018b, pp. 113–130); however, a few aspects of the CFF need further qualification. Examples identified in this work include the distinction between energy recovery and material recycling, the definition of the specific factors included in the CFF, the point of substitution etc.
A PEFCR for biofuels can make the methodology more robust by focusing on identifying points of substitution that are specific for biofuels. We recommend that the industry develops a PEFCR for biofuel with support from experts on PEF and on LCA in general.
Similarly, PCRs for biofuels would help making the EPD methodology more well-defined to clarify, for example, how to partition burdens in the most important multifunctional activities. A biofuel PCR could also more clearly establish whether emissions from digestions should be included in an EPD of biogas (as indicated by Version 3.01 of the General Programme Instructions) or excluded from the EPD (as indicated by Version 4.0 and by the PCR for electricity and heat). We recommend that the industry develops such a PCR with support from experts on EPD and on LCA in general.
To enhance the development and harmonization of studied LCA approaches, this study stresses the need for product specific rules (in the form of PEFCR and PCR) for renewable fuels. The variety of promising feedstock alternatives in biofuel production (industrial residues, waste, electricity, or other type of energy carriers) indicates that future transport fuels are likely to involve complex, interconnected, and sometimes circular value chains making the need for updated and comprehensive rules of paramount importance.
Future versions of all three studied frameworks should also be clearer on how specific methodological choices are to be applied, for example when it comes to allocation and multifunctional processes, as well as modeling electricity supply. RED, for example, could be clearer on how to define the electricity region, as could the EPD guidelines for defining the electricity market.
Although it may not realistic to aim for a single unified LCA framework, the biofuel PCR and PEFCR can be developed with reference to RED. Some aspects of the PEF methodology could also be integrated into subsequent versions of the RED, for example the modeling of waste management and the production of waste-based fuels. This would enhance the broader adoption of the framework among fuel producers.
Finally, the involvement and engagement of the industry, including fuel producers, is necessary. A recently finalized project on the application of PEF and EPD on paper and steel products stressed the benefits of industry engagement, not only in terms of capacity building and increased awareness, but also in terms of preparedness for future developments and requirements (Palander et al., 2021). The actors involved in that project identified similar methodological and data challenges to the ones described in this study. Industry initiatives are therefore essential for the development of biofuel PCR and PEFCR, while the general development of the three frameworks can also be influenced.
Data availability statement
The raw data supporting the conclusions of this article will be made available by the authors, without undue reservation.
Author contributions
MB, TE, SP, and TR contributed to conception and design of the study. MB, JN, and PN conducted the case studies. MB wrote the first draft of the manuscript. TE, SP, JN, and PN wrote sections of the manuscript. All authors contributed to manuscript revision, read, and approved the submitted version.
Acknowledgments
For the funding of this project, the authors would like to thank the Renewable Transportation Fuels and Systems 2018–2021, which is a collaborative research programme between the Swedish Energy Agency and f3 The Swedish Knowledge Center for Renewable Transportation Fuels.
Conflict of interest
The authors declare that the research was conducted in the absence of any commercial or financial relationships that could be construed as a potential conflict of interest.
Publisher's note
All claims expressed in this article are solely those of the authors and do not necessarily represent those of their affiliated organizations, or those of the publisher, the editors and the reviewers. Any product that may be evaluated in this article, or claim that may be made by its manufacturer, is not guaranteed or endorsed by the publisher.
References
Börjesson, P., Lantz, M., Andersson, J., Björnsson, L., Möller, B.F., Fröberg, M., et al. (2016). Methane as Vehicle Fuel—A Well-to-Wheel Analysis (MetDriv). Report No 2016:06, f3. The Swedish Knowledge Centre for Renewable Transportation Fuels, Sweden. Available online at: www.f3centre.se
Brandão, M., Azzi, E., Novaes, R. M. L., and Cowie, A. (2021). The modelling approach determines the carbon footprint of biofuels: the role of LCA in informing decision makers in government and industry. Clean. Environ. Syst. 2:100027. doi: 10.1016/j.cesys.2021.100027
CARB (2015). Low Carbon Fuel Standard. Final Regulation Order. Title17, California Code of Regulations, California Air Resources Board. Available online at: http://www.arb.ca.gov/re~gact/2015/lcfs2015/lcfsfinalregorder.pdf
Dalgaard, R., Schmidt, J., Halberg, N., Christensen, P., Thrane, M., and Pengue, W. A. (2008). LCA of soybean meal. Int. J. Life Cycle Assess. 13, 240–254. doi: 10.1065/lca2007.06.342
Del Borghi, A. (2013). LCA and communication: environmental product declaration. Int. J. Life Cycle Assess. 18, 293–295. doi: 10.1007/s11367-012-0513-9
DfT. (2008). Carbon and Sustainability Reporting Within the Renewable Transport Fuel Obligation. Requirements and Guidance Government Recommendation to the Office of the Renewable Fuels Agency. London: DfT. Available online at: http://www.dft.gov.uk/pgr/roads/environment/rtfo/govrecrfa.pdf
EC (2012). Product Environmental Footprint (PEF) Guide. Italy: European Commission, Joint Research Centre (JRC).
EC (2018a). Directive (EU) 2018/2001 of the European Parliament and of the Council of 11 December 2018 on the promotion of the use of energy from renewable sources. O. J. Eur. Union L. 328, 82–209. European Commission.
EC (2018b). PEFCR Guidance Document - Guidance for the Development of Product Environmental Footprint Category Rules (PEFCRs), Version 6.3. European Commission.
EC (2019). COMMISSION DELEGATED REGULATION (EU) …/… of 13.3.2019 Supplementing Directive (EU) 2018/2001 As Regards the Determination of High Indirect Land-Use Change-Risk Feedstock for Which A Significant Expansion of the Production Area into Land With High Carbon Stock is Observed and the Certification of Low Indirect Land-Use Change-Risk Biofuels, Bioliquids and Biomass Fuels. European Commission.
EI (2021). Nu finns information om residualmix för 2019. The Swedish Energy Market Inspectorate. Available online at: https://www.ei.se/sv/nyhetsrum/nyheter/nyheter-2020/nu-finnsinformation-om-residualmix-for-2019/ (in Swedish) (accessed July 5, 2022).
EPD (2019). General Programme Instructions for the International EPD ® System. Version 3.01. EPD International.
EPD (2020). Product Category Rules (PCR): Electricity, Steam and Hot Water Generation and Distribution.
EPD (2021). General Programme Instructions for the International EPD® System. Version 4.0. EPD International.
EPD. (2022). Environmental Performance Indicators. Available online at: https://environdec.com/resources/indicators (accessed 5 July, 2022).
Flach, B., Lieberz, S., and Bolla, S. (2019). EU-28 Biofuels Annual EU Biofuels Annual 2019. GAIN Report. Washington, DC.
Hallberg, L., Rydberg, T., Bolin, L., Dahllöf, L., Mikaelsson, H., Iverfeldt, E., et al. (2013). Well-to-Wheel LCI Data for Fossil and Renewable Fuels on the Swedish Market. Report No 2013:29, f3 The Swedish Knowledge Centre for Renewable Transportation Fuels, Sweden. Available online at: www.f3centre.se
Hunsager, E. A., Bach, M., and Breuer, L. (2014). An institutional analysis of EPD programs and a global PCR registry. Int. J. Life Cycle Assess. 19, 786–795. doi: 10.1007/s11367-014-0711-8
ICAO (2020). Resolution A40-19: Consolidated Statement of Continuing ICAO Policies and Practices Related to Environmental Protection - Carbon Offsetting and Reduction Scheme for International Aviation (CORSIA). International Civil Aviation Organisaiton.
ISO (2006a). ISO 14040. Environmental Management—Life Cycle Assessment—Principles and Framework. International Organisation for Standardisation.
ISO (2006b). ISO 14044. Environmental Management—Life Cycle Assessment—Requirements and Guidelines. International Organisation for Standardisation.
ISO (2006c). ISO 14025. Environmental Labels and Declarations—Type III Environmental Declarations. International Organisation for Standardisation.
ISO (2020a). ISO 14040:2006/Amd 1:2020(EN). Environmental Management—Life Cycle Assessment—Principles and Framework. International Organisation for Standardisation, AMENDMENT 1.
ISO (2020b). ISO 14044:2006/Amd 2:2020(EN). Environmental Management—Life Cycle Assessment—Requirements and Guidelines. International Organisation for Standardisation, AMENDMENT 2.
Jogner, C., and Nojpanya, P. (2021). A Comparison of Different Frameworks for Product Environmental Performance: A Life-Cycle-Based Environmental Assessment of HVO from Used Cooking Oil (UCO) Based on EPD, PEF and RED II Frameworks. Report No. E2021: 060. Chalmers University of Technology, Gothenburg, Sweden.
Minkov, N., Schneider, L., Lehmann, A., and Finkbeiner, M. (2015). Type III environmental declaration programmes and harmonization of product category rules: status quo and practical challenges. J. Clean. Prod. 94, 235–246. doi: 10.1016/j.jclepro.2015.02.012
Myhre, G. D., Shindell, F.-M., Bréon, W., Collins, J., Fuglestvedt, J., Huang, D., et al. (2013). “Anthropogenic and natural radiative forcing,” in Climate Change 2013: The Physical Science Basis. Contribution of Working Group I to the Fifth Assessment Report of the Intergovernmental Panel on Climate Change, eds T. F. Stocker, D. Qin, G.-K. Plattner, M. Tignor, S. K. Allen, J. Boschung, A. Nauels, Y. Xia, V. Bex, and P.M. Midgley (Cambridge, UK; New York, NY: Cambridge University Press) p. 710–714.
Palander, S., Lorentzon, K., Hammar, T., Sanne, K., Nilsson, J., Hallberg, L., et al. (2021). Environmental Footprint in Sweden—Increased Competence and Communication. Lessons Learned of Working with Product Environmental Footprint. Report number: 2022:03, Gothenburg, Sweden.
Poulikidou, S., Johansson, K., Lassesson, H., Nilsson, J., Nojpanya, P., Rydberg, T., et al. (2022). Impacts on fuel producers and customers of conflicting rules for Life Cycle Assessment. Publ. No FDOS 30:2022. Available online at: https://f3centre.se/en/renewabletransportation-fuels-and-systems/
Renewable Fuels Association (RFA) (2021). Markets and Statistics. Available online at: https://ethanolrfa.org/markets-and-statistics/annual-ethanol-production (accessed 6 July, 2022).
Schmidt, J. H., and Weidema, B. P. (2008). Shift in the marginal supply of vegetable oil. Int. J. Life Cycle Assess. 13, 235–239. doi: 10.1065/lca2007.07.351
Sphera (2021). GaBi Software System and Database for Life Cycle Engineering 1992–2018 Version 10. Leinfelden-Echterdingen, Germany.
Sphera (2022). Environmental Footprint (EF) Secondary Data Sets Versions EF 2.0 and 3.0. Available online at: https://lcdn.thinkstep.com/Node/ (accessed 9 June, 2022).
U.S. Government (2005). Energy Policy Act of 2005 Public Law 109-59, 109th Congress. Washington, DC.
Wernet, G., Bauer, C., Steubing, B., Reinhard, J., Moreno Ruiz, E., and Weidema, B. (2016). The ecoinvent database version 3 (part I): overview and methodology. Int. J. Life Cycle Assess. 21, 1218–1230. doi: 10.1007/s11367-016-1087-8
Keywords: life cycle assessment (LCA), biofuels, RED, EPD, PEF, carbon footprint
Citation: Brandão M, Ekvall T, Poulikidou S, Johansson K, Nilsson J, Nojpanya P, Wikström A and Rydberg T (2022) RED, PEF, and EPD: Conflicting rules for determining the carbon footprint of biofuels give unclear signals to fuel producers and customers. Front. Clim. 4:988769. doi: 10.3389/fclim.2022.988769
Received: 07 July 2022; Accepted: 26 September 2022;
Published: 12 October 2022.
Edited by:
Catherine Butler, University of Exeter, United KingdomReviewed by:
Mariarosaria Lombardi, University of Foggia, ItalyVille Uusitalo, Lappeenranta University of Technology, Finland
Copyright © 2022 Brandão, Ekvall, Poulikidou, Johansson, Nilsson, Nojpanya, Wikström and Rydberg. This is an open-access article distributed under the terms of the Creative Commons Attribution License (CC BY). The use, distribution or reproduction in other forums is permitted, provided the original author(s) and the copyright owner(s) are credited and that the original publication in this journal is cited, in accordance with accepted academic practice. No use, distribution or reproduction is permitted which does not comply with these terms.
*Correspondence: Miguel Brandão, miguel.brandao@abe.kth.se