Editorial: Climate risks, resilience and adaptation in coastal systems
- 1Laboratorio de Ecología del Paisaje, Instituto de Ciencias Ambientales y Ecológicas, Facultad de Ciencias, Universidad de Los Andes, Mérida, Venezuela
- 2Programa de Ciencias Ambientales, Universidad Nacional Experimental Francisco de Miranda Santa Ana de Coro, Estado Falcón, Venezuela
- 3Facultad de Ciencias, Universidad de la República (UdelaR), Montevideo, Uruguay
- 4Texas Sea Grant at Texas A&M University, College Station, TX, United States
- 5Cawthron Institute, Nelson, New Zealand
- 6Instituto de Zoología y Ecología Tropical, Facultad de Ciencias, Universidad Central de Venezuela, Caracas, Venezuela
- 7Consejo Nacional de Investigaciones Científicas y Técnicas (CONICET), Buenos Aires, Argentina
- 8Centro Interdisciplinario de Investigaciones y Estudios Sobre Medio Ambiente y Desarrollo, Instituto Politécnico Nacional, Ciudad de México, Mexico
- 9Facultad de Ciencias, Universidad de la República (UdelaR), Instituto de Ecología y Ciencias Ambientales (IECA), Oceanografía y Ecología Marina, Montevideo, Uruguay
Natural and human-induced hazards and climatic risks threaten marine and coastal ecosystems worldwide, with severe consequences for these socio-ecological systems. Therefore, assessing climate vulnerability (exposure, sensitivity and adaptive capacity) and the cumulative environmental impacts of multiple hazards are essential in coastal planning and management. In this article, we review some approaches used in climate vulnerability assessment and marine and coastal cumulative environmental impacts to learn about state-of-the-art on the subject. Besides, we qualitatively evaluated the climatic vulnerability of five coastal regions of Venezuela using the IPCC concept of Reasons for Concern (RFCs) to determine their level of climatic exposure. We also assessed the cumulative environmental impact of multiple stressors on marine and terrestrial ecosystems using a well-known impact assessment method partially modified to explore the feasibility of this model in data-poor areas. However, we found no standardization of the methodologies used in evaluating Coastal Climate Vulnerability or Cumulative Environmental Impacts in coastal landscapes or frameworks that operationally link them with socio-ecological systems. Most studied coastal regions are at risk from at least three RFCs, loss of unique ecosystems (RFC1), risks associated with extreme events (RFC2) and risks associated with global aggregate impacts (RFC4). Furthermore, the assessment showed that areas with accumulated impact cover about 10 percent ranging from moderate to high in urban areas, growth zones, industrial oil settlements, port areas and aquaculture areas with fishing activity. Moreover, areas with moderate to low cumulative impact cover half of the study area, dominated by uninhabited regions and vegetation of the thorny scrub and coastal grassland types. Therefore, we consider it essential to implement regional climate risk management that incorporates these assessments into the ordinance in countries that are particularly vulnerable to climate change, such as Venezuela, which has an extensive line of low-lying coastlines (where 60% of the country's population lives) and coastal regions with harsh climates and poor economic conditions. Finally, we present the scope and limitations of implementing these evaluations and highlight the importance of incorporating them into regional strategies for adaptation to climate change.
Introduction
The increase in human pressures and exposure to the impacts and threats of climate change intensify the Climate Vulnerability of Coastal areas CVC, affecting biological diversity, contributing to the loss of habitats, and increasing their vulnerability to variations in sea level and erosion (Spalding et al., 2014; Newton et al., 2020).
This research assumes vulnerability as people and places are susceptible to disturbances resulting from coastal hazards (Bevacqua et al., 2018). Measured by the level of readiness or state of the system to anticipate or respond to risks (IPCC., 2014; Sharma and Ravindranath, 2019; O'Neill et al., 2022) and as the tendency or predisposition of assets to be negatively affected by threats, and its evolution incorporates exposure, sensitivity, possible consequences and adaptability (Nagy et al., 2019), as shown in Figure 1.
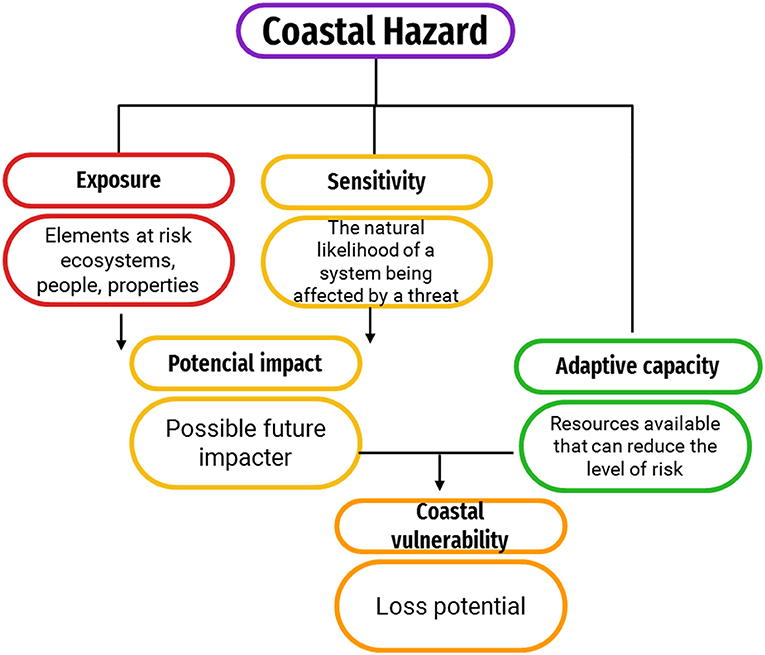
Figure 1. Conceptual framework and terms related to coastal vulnerability [Source: Developed from Wamsley et al. (2015)].
We know that CVC is intensified by human pressures and by its exposure to the impacts of climate change (Spalding et al., 2014; Newton et al., 2020) due to human population growth and the increase in economic activities in the coastal areas. For this reason, governments need management decisions or development projects to assess how human activities contribute to Cumulative Environmental Impacts CEI to determine how they interact to affect a species or ecosystem (Hollarsmith et al., 2021).
On the other hand, human pressures and exposure to the impacts of climate change (for example, rising sea levels and rising global surface temperatures) intensify climate vulnerability that has effects on biological diversity by contributing to the loss of habitat that protects human communities by increasing their vulnerability to variations in sea level and erosion (Spalding et al., 2014; Newton et al., 2020).
At the same time, the cumulative impacts are increasing in number and intensity, mainly due to the growth of the human population and the increase in economic activities in coastal areas. Consequently, governments need management decisions or development projects to evaluate how human activities Contribute to Cumulative Environmental Impacts CAIA to determine how multiple human activities may interact to affect a species or ecosystem (Hollarsmith et al., 2021).
In this way, various methods have emerged to evaluate them, but each has its limitations (Hodgson et al., 2019).
Apart from the CEIA, the Climate Vulnerability Assessment CVA; allows understanding of how economic sectors and communities are affected by current and potential climate changes, helping to inform actions that contribute to human and ecosystem wellbeing, guide environmental policies, and achieve Climate Change Adaptation, ensuring the prioritization of areas with a higher risk of a negative impact in coastal adaptation planning.
The Climate Vulnerability Assessment CVA and the Cumulative Environmental Impact Assessment CEIA are central elements of Ecosystem-Based Management as they contribute to climate adaptation planning, aligned with the methodologies and approaches of the sustainable development goals. Such elements show a complex and intricate relationship between the Sustainable Development Goals and adaptation to climate change's impacts at the global level (Fuldauer et al., 2022).
Notwithstanding the preceding, the choice of a particular methodological approach depends on application site conditions, data availability and scale, and access to reliable information.
The issue of climate vulnerability assessments and cumulative environmental impacts is of particular interest, mainly due to the need to have baseline information to develop efficient management and coastal management plans for adaptation to climate change.
Due to this, we set the objectives in this research as follows a) To review the different methodological approaches used in evaluating climatic vulnerability and the cumulative marine and coastal environmental impacts to know state of the art on the subject. b) To evaluate the climatic vulnerability of five coastal regions of Venezuela by carrying out a conceptual, theoretical analysis of the RFC indicators for each of these regions. The IPCC Reasons of Concern (RFC) concept helps to determine the level of climate exposure for each region and c) To test the cumulative environmental impact assessment using an impact model to explore the feasibility of this model in data-poor areas.
For the climate vulnerability analysis, we selected five coastal regions of Venezuela due to their exposure to changing climatic conditions. The IPCC Reasons of Concern (RFC) indicators were analyzed for each region to document the level of climate exposure in each; we found that five regions are exposed to at least three (RFCs), namely loss of unique ecosystems (RFC1), risks associated with extreme events (RFC2), and risks associated with global aggregate impacts (RFC4).
We selected the Central Coast of Falcón state to analyze the Cumulative Environmental Impacts and present the sustained impact over time of various anthropogenic activities with effects on the variety of marine and coastal ecosystems in the area.
In this area, we implemented the Halpern Cumulative Impact Model (Halpern et al., 2008) both on land and at sea to document existing threats and explore the feasibility of applying this impact model in data-poor areas and found that regions with accumulated impact cover about 10 percent ranging from moderate to high in urban areas, growth zones, industrial oil settlements, port areas and aquaculture areas with fishing activity. Moreover, areas with moderate to low cumulative impact cover half of the study area, dominated by uninhabited regions and vegetation of the thorny scrub and coastal grassland types.
Impacts of climate change on coastal landscapes
We define coastal seascapes as a mosaic of patches with benthic or pelagic environments, variable in distribution, nutrients and depth (Boström et al., 2011; Pittman et al., 2011), composed of different marine and terrestrial coverages (Kim et al., 2017), with high complexity in the provision of ecological services (refuge and habitat for many species) (Barbier et al., 2011); protection against storms and hurricanes (Sevilla and Le Bail, 2017); erosion and flood prevention.
Coastal landscapes are subject to multiple disturbances, such as climate change; which affects their functionality and provision of services (Singh et al., 2019; Smale et al., 2019), conditioning their resilience (Bernhardt and Leslie, 2013; He and Silliman, 2019) and affecting marine biodiversity at different temporal and spatial scales (IPCC., 2018, 2019).
According to the IPCC AR6 report of WG I (IPCC., 2021), all regions of the Earth are being affected by climate change caused by man, so many of the low-lying coasts are subject to severe risks from climate change.
Several recent studies have documented rapid and accelerating losses of coastal landscape components, such as the loss of coastal forested wetlands (White et al., 2022). The main drivers of change in the value of wetland ecosystem services in coastal areas; are relative sea level rise, coastal erosion, increased cyclone frequency, and rising sea temperatures (Mehvar et al., 2019).
It also highlights the degradation and transformation of landscapes due to sea level rise and extreme events due to climate change with implications for the function of wetlands, wildlife habitats, and the global carbon cycle (Ury et al., 2021).
Methodological framework
For the review, we followed a narrative literature-based approach, and the scope of the existing literature focused on an overview of the methods used for CVA, emphasizing biophysical and environmental aspects to understand the state of knowledge about the different approaches used in the CVA and the CEIA. To evaluate the climatic vulnerability of five coastal regions of Venezuela, we analyzed the sensitivity, exposure, and adaptive capacity and used indicators related to the Reasons for Concern of the IPCC; RFC-1, RFC2, RFC4, and RFC5 (O'Neill et al., 2022). While applying the impact model, we use the method of Halpern et al. (2008), where the index is a function of (i) relevant human pressures expressed by intensity maps, (ii) representative ecosystem components augmented as value maps, and (iii) a sensitivity index that defines how sensitive each ecosystem component is to every human pressure. We use sensitivity scores based on expert judgment to weigh the potential effects of anthropogenic pressures on selected ecosystems and verify all information in the field.
Methodological approaches used in the CVA and the CEIE
Methodological approaches used in the CVA
The concept of vulnerability has evolved since the second assessment report of the IPCC. (1996), which describes vulnerability as “the extent to which climate change can damage or impair a system, suggesting that it depends not only on the system's sensitivity but also on its ability to adapt to new climatic conditions”.
Later in the IPCC Fourth Assessment Report (AR4), the definition of vulnerability was established as a function of three factors; exposure, sensitivity, and adaptability (Schneider et al., 2007; Hahn et al., 2009). AR4 refers to the vulnerable system (for example, low-lying islands or coastal cities).
More recently, in the IPCC Fifth Assessment Report (AR5), a new approach and terminology similar to the concept of disaster risk is introduced, which differs from the current understanding of vulnerability as mentioned in IPCC AR4 (Fritzsche et al., 2014).
In the literature, the main methodological approaches to CVA offer three ways of perceiving vulnerability (Lampis, 2013): a) as a result of a process (external to the system), b) as an internal characteristic of the system (exposed to the threat making it susceptible to damage) and c) as the combination of internal vulnerability (social; a population, a place) with its exposure to external biophysical risk factors.
The latter is called the “Integrated Approach” or “Strategic Environmental Assessment” EAE or SEA2 (Espinoza, 2007), represented in Figure 2. The SEA2 has a proactive view of vulnerability assessment and considers cumulative, additive, synergistic effects, saturation thresholds, and induced and indirect impacts. They are used at the policy, planning and programming levels.
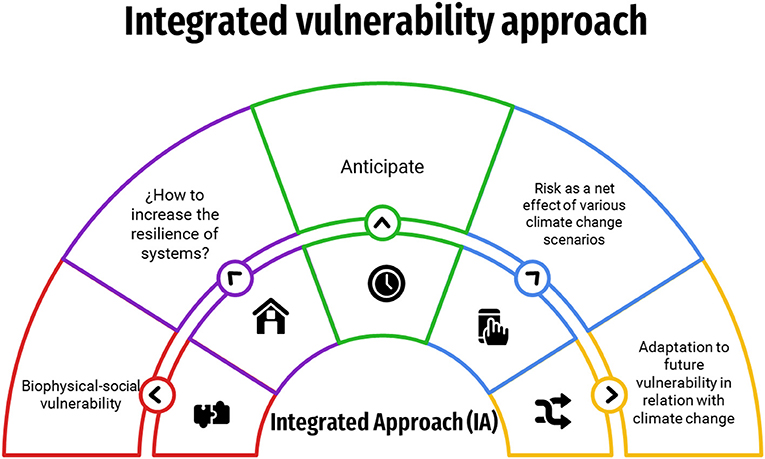
Figure 2. An integrated vulnerability approach was created from Lampis (2013) approach.
According to AR5, risk (risks from climate change impacts) (Oppenheimer et al., 2014) is the potential for consequences where something of value is at stake and where the outcome is uncertain, recognizing the diversity of values.
Vulnerability in AR5 includes the concepts of sensitivity (susceptibility to damage) and adaptive capacity. Therefore, it can be said that the terminology used in these last two IPCC assessment reports is different, but the basic assumptions follow the same underlying principles (Das et al., 2020).
On the other hand, the AR6 assessment incorporates the complex nature of climate risk, integrating key risks, including how these risks vary with the magnitude of global warming, socioeconomic development pathways, and levels of adaptation (Begum et al., 2022).
The AR6 provides new information on risks under levels of warming, including cascading, composite, and transboundary risks. The reasons for concern consider unique and threatened ecosystems (RFC1) with high vulnerability and low adaptation capacity. Extreme weather events (RFC2) include heat waves, heavy rains, droughts, coastal flooding, or wildfires. The reasons of interest address both the distributional and aggregate impacts of climate change (RFC3, RFC4). The final cause for concern relates to large-scale singularities such as Events, Non-linearities, and Tipping Points (RFC5), including ice sheet collapse and ecosystem regime changes (Begum et al., 2022).
Other CVA methods, known as statistical or empirical models, involve using climate variables (e.g., global surface temperature) to make future predictions based on past observations. Some use indicators like; exposure to socioeconomic threats, land use, and land cover (Wu et al., 2020). For example, the Coastal Vulnerability Index to sea level rise combines variables from different categories (Gallego and Selvaraj, 2019). They also highlight some research that integrates physical and social aspects (Aral et al., 2012; Hoque et al., 2019).
CVA in coastal regions of Venezuela
With 3,726 km and direct access to the Atlantic Ocean and the Caribbean Sea, Venezuela is physically and environmentally vulnerable to sea level rise (Naveda, 2010; Villamizar et al., 2017; Villamizar, 2020). Furthermore, with more than 50% of the total population concentrated in the northern zone, occupying 10% of the national territory, the country is also environmentally and socioeconomically vulnerable due to the high proportion of the population that inhabits its coasts (Marrero and Rodríguez-Olarte, 2017).
Table 1 shows a qualitative analysis of the Reasons for Concern RFC due to climate change for five regions of Venezuela (Northwest, Northcentral, Northeast, East, and Federal Dependencies).
Methodological approaches used in the CEIA
Currently, the CEIA of natural and anthropic pressures on marine and coastal ecosystems is a topic of great importance in strategic planning, conservation, and coastal management (Stelzenmüller et al., 2018). However, it remains an exploratory field (Quemmerais-Amice et al., 2020) because conceptual models and tools are still under development (Korpinen and Andersen, 2016; Stelzenmüller et al., 2018).
Recent reviews (Korpinen and Andersen, 2016; Gissi et al., 2021) offer evidence of the combined effects of climate change on ecological responses (Figure 3). From human activities to the ecological components of the system (Hodgson et al., 2019), such as the effect of temperature changes on industrial fishing, sea level rise with artisanal fishing, change in sediment load, and introduction of alien species.
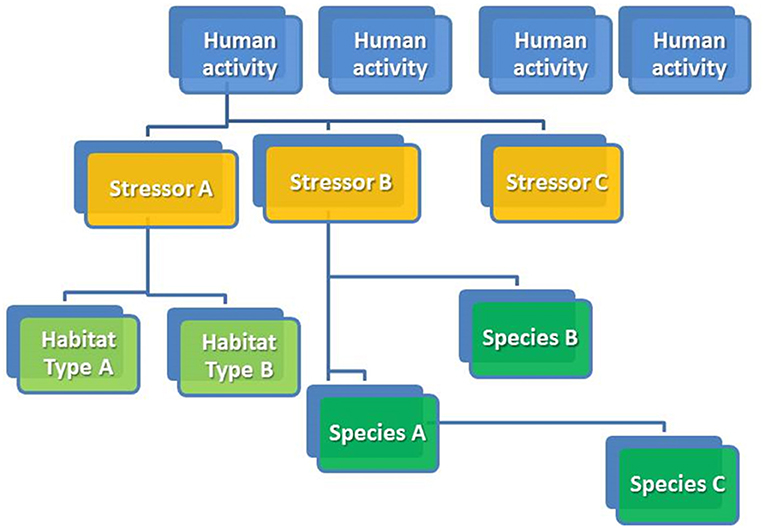
Figure 3. Hypothetical pathways followed by cumulative effects from human activities on the ecological components of the system [Modified from Hodgson et al. (2019)].
Cumulative impact assessments apply at different ecological scales; species (Marcotte et al., 2015), communities (Giakoumi et al., 2015), and also at the landscape level (Harker et al., 2021), integrating history and space, temporal scales such as habitat connectivity (Tarabon et al., 2019) and network analysis to detect changes (Harvey and Altermatt, 2019).
It seems that one of the most widely used general approaches in the assessment of cumulative impacts (Korpinen and Andersen, 2016) is the method of Halpern et al. (2008) which has frequently been applied, making minor adaptations of the method when treating the input data and integrating them into the accumulated pressure or impact score or the ecosystem sensitivity score.
More recently, research has emerged using landscape ecological approaches and geographic information systems (GIS) that combine information on a landscape scale and history, providing a valuable tool to support management in marine and coastal environments (Micheli et al., 2013; Singh et al., 2019; Harker et al., 2021).
In the South American case, up to now, CEIA has only been carried out in Brazil (Magris et al., 2018; Vilardo and La Rovere, 2018; Blakley and Russell, 2022).
CEIA in coastal regions of Venezuela
Area case of study. The area is located in northwestern Venezuela (Figure 4) (11° 56' 19 0.97” N) and (70 17' 9.42” W), (11° 29' 23 0.12” N) and (69° 24' 35.25” O) and covers a total area of 14,498.14 km2. It presents a flat relief with a limestone basement, calcareous clay rocks, and active dune and paleodune systems (Audemard, 1996).
The climate is arid or semi-arid, with an average annual rainfall of 330.5 mm, with maximums between October and December and minimums between January and July. The yearly average wind speed is 6.4 km/h, reaching a maximum speed in July (7.9 km/h) and a minimum speed in November (4.9 km/h) (Ministry of Environment and Renewable Natural Resources).
The area has a diversity of marine and coastal ecosystems (Carmona, 1989; Aguilera et al., 2003; Rodríguez et al., 2010), such as mangroves, seagrass meadows, coral reefs, coastal lagoons, and coastal forests (Matteucci et al., 1999; Rodríguez et al., 2010).
Ecosystems and stressors
The ecosystems (n = 16; Supplementary Table S1), stressors (n = 14; Supplementary Table S2) shown in Figure 5, and resulting impacts were mapped on a grid with 100 × 100 m cells (a hectare). Ecosystems and binary stressors were mapped based on their presence or absence in each grid cell. Non-binary stressors were log [X+1] transformed to reduce the effect of extreme outliers and then readjusted between 0 and 1 to allow stressors with different distributions and different units of measure to be compared with each other. Standardization requires choosing a maximum value to set equal to 1.0. In our study, we used the highest observed value within the study area, plus an additional 10%, as recommended by Halpern and Fujita (2013) (see Supplementary Table S2 for details).
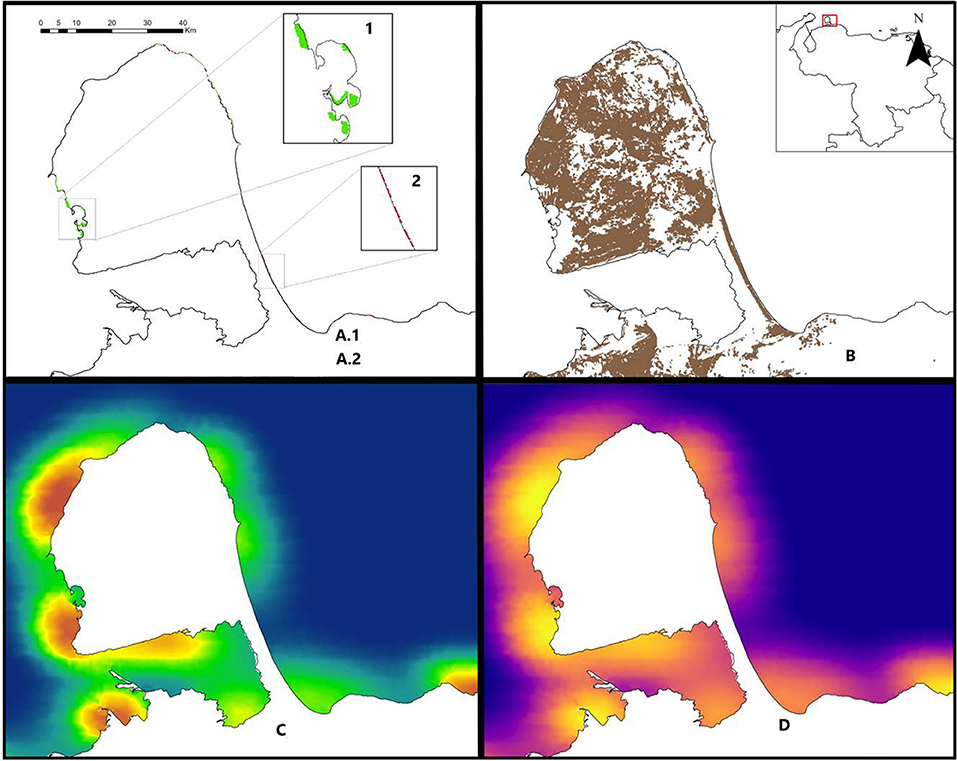
Figure 5. Layers of ecosystems for the model of cumulative impacts and aquatic stressors on the central coast of Falcón state, Venezuela. (A) 1) Seagrass 2) Rocky shoreline (B) Bush (C) Nutrients (D) Chemical pollution.
Impact weights
An essential step in the cumulative impact model is estimating the impact of each stressor on each ecosystem. Impact weight (sometimes called vulnerability score or vulnerability weight in other studies) is the value that transforms a stressor into an impact for a given ecosystem and is determined by the vulnerability of the ecosystem to that stressor. The impact weights were derived from three sources that used expert judgment (through a questionnaire, their opinion value judgment based on knowledge, experience, on the subject of analysis) to estimate the vulnerability of ecosystems to anthropogenic stressors (Halpern et al., 2007, 2015). In addition, when impact weights were unavailable for a given ecosystem and stressor combination, we consulted experts from the case study area to provide impact weights. Finally, weighted scores for the ecosystem/stressor combinations addressed in the present study were extracted and used as impact weights in the cumulative impact model (Supplementary Table S3).
Cumulative impact model
Matrices representing distributions of ecosystems and stressors in the case study area were assembled in ArcMap 10.6 and multiplied by the corresponding impact weights (Supplementary Table S3). Cumulative Impact Score (CI) was calculated for each one-hectare grid cell using the following formula described in Halpern et al. (2008).
where Di is the log[X+1] intensity transformed and standardized (with a scale between 0 and 1) of a stressor i, Ej is the presence or absence (1 or 0, respectively) of the ecosystem j, and μi,j is the impact weight for stress factor i and ecosystem j (range 0 to 4), given n = 14 stress factors and m = 16 ecosystems.
Results
By compiling available information on indicators of Vulnerability and Reasons for Concern (RFCs) to climate change for marine and coastal ecosystems in different regions of Venezuela, we obtained that five coastal regions of Venezuela are at risk of at least three of the RFCs analyzed, which include the loss of unique ecosystems and risks associated with extreme events, as shown in Figure 6.
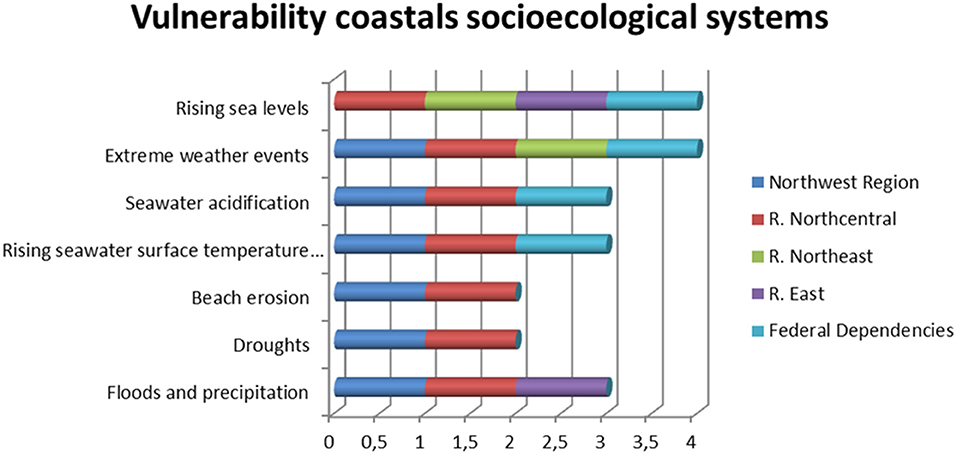
Figure 6. Vulnerability of the coastal socio-ecological systems of Venezuela to climate change indicators. Regions of Venezuela: Northwest, Northcentral, Northeast, East, and Federal Dependencies.
Among the coastal regions of Venezuela that show exposure to coastal risks related to climate change, the regions; Northwest, North Center, Northeast, East, and Federal Dependencies, as shown in Figure 7.
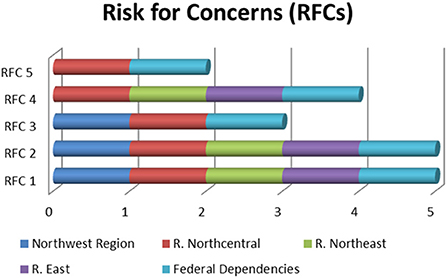
Figure 7. Coastal risks related to climate change. Regions of Venezuela: Northwest, Northcentral, Northeast, East, and Federal Dependencies.
Table 1 shows the coastal regions of Venezuela that are exposed to climatic risks.
Risks to unique and threatened systems (RFC-1)
We found that under scenarios of an increase of 0.5 m and 1 m to the SRL (Olivo-Garrido et al., 2011), several coastal units of Venezuela (Miranda state) present environmental sensitivity, being the Laguna de Tacarigua National Park “Northeast Region” NER, the most affected, with estimated land losses due to flooding between 8.97 and 17.75 km2. In addition, projections from the US Climate Central Association (climatecentral.org) estimate that by the year 2100, the surroundings of ML and the city of Tucupita (DA state) will be submerged due to SLR.
Risks associated with extreme weather events (RFC2)
Another critical event related to the extreme variations of the climate on the coasts of Venezuela is the event of extraordinary rains in 1999 that caused the desalination of shallow water masses in one of the extensive seagrass meadows of the Morrocoy National Park “Noroccidental Region” (NWR) and Las Aves, the northernmost island of Venezuela, significantly affected by hurricanes (Olivares and Piñero, 2010). Compared to the rest of the Caribbean, this has been one of the few extreme events (storms, cyclones, and hurricanes) that have hit Venezuela's coasts.
Impacts related to aggregate risks (RFC4), and RFC5
North-central region Landslides and threat to people's lives (mainly Vargas); loss of coastal area due to sea level rise (in Miranda); (in Aragua 1987 and 2020 tragedies of the Limón river), López et al. (2011), Olivo-Garrido et al. (2011), ACFIMAN-SACC (2018). North-eastern region: Decline in fisheries (ie sardines); Gómez et al. (2012, 2014), Gaspar (2022), Taylor et al. (2012), Gómez (2019), González et al. (2020). Eastern region: a threat to the property of indigenous populations in the alluvial plains of the Orinoco Delta Medina et al. (2015), MPPEA-IICNCC (2017). Federal Dependencies: changes in the coverage and structure of mangrove and seagrass ecosystems, affectation of organisms with calcareous shells; Villamizar et al. (2003, 2008, 2014), Cróquer and Weil (2009), Bastidas et al. (2012), Yranzo et al. (2014), Laso (2015).
In the North-western region, which presents impacts of desertification; beach erosion, shoreline retreat; mangrove degradation; changes in the composition of the vegetation; degradation of reef habitats, we apply the model of Crain et al. (2008) in their marine and coastal ecosystems using multiple natural and anthropogenic pressures.
Obtained “Moderate to High” cumulative impact levels that cover 9.93% (102,471, 522Ha) of the area where there are urban growth zones, industrial oil settlements, port areas, and aquaculture areas with fishing activity on the western coast of the Peninsula of Paraguaná, the Golfete de Coro and the flood zone of the Isthmus of Los Médanos (see Figure 8).
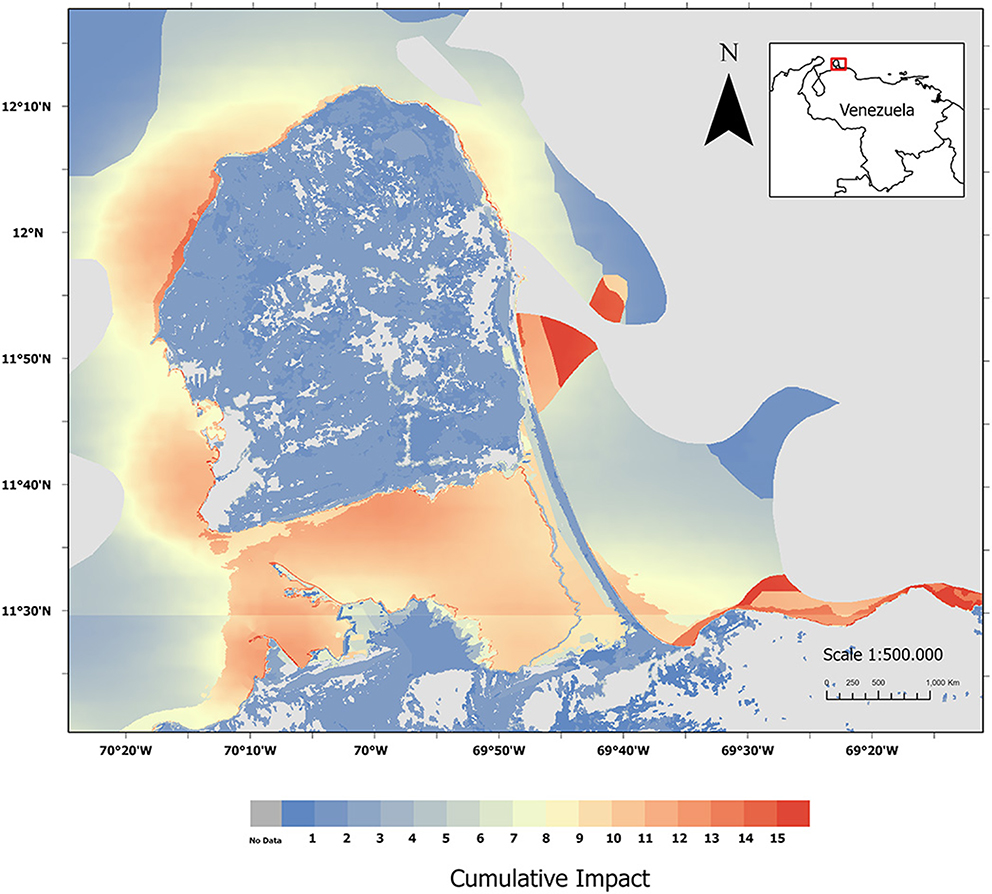
Figure 8. Map of cumulative impacts of multiple natural and anthropic disturbances on the central coast of Falcón state, Venezuela.
“High” cumulative impacts were located in populated coastal areas. They require special attention in their comprehensive management due to their growth; the areas with “moderate” cumulative impacts destined for aquaculture and fishing activities require compliance with the established management and planning parameters.
The model also yielded “Low to moderate” cumulative impact levels that cover around 43.74% (455,345.95 Ha) of the area in coastal areas with “thorn scrub” and “coastal grassland” type vegetation located on the Peninsula de Paraguaná and flood-prone areas of the Mitare Delta. In contrast, moderate pressures decreased in the direction of the Gulf of Venezuela and inland.
The areas with a “low or moderate” cumulative impact located in the Paraguaná Peninsula and the flood-prone areas of the Mitare Delta require comprehensive management to ensure the sustainability and conservation of the ecosystems.
In general, the intensity levels of the impacts were distributed heterogeneously along the east coast of the Paraguaná Peninsula, with “Medium to high” impact focusing on rocky shores with hard bottoms, sandy beaches, and coral communities (in Adícora-Buchuaco to Cabo San Román) and in the “Golfete de Coro” with “Medium to low” impact levels in areas with coastal lagoons, sandy beaches, and mangroves.
On the other hand, the stress factors measured for all the marine and coastal ecosystems of the central coast of the state of Falcón, Venezuela, that contributed the most to the cumulative impact score were: “Nutrients” (contribution of nitrogen and phosphorus from urban discharges), “Chempoll” (Contaminants from sewage, urban runoff, industrial effluent, agricultural residue) and “Shipping” (Maritime traffic trajectories and frequency) as shown in Supplementary Table S4.
Detailed per-pixel results were not obtained for the contribution of the rest of the threats; since most of the layers were rasters with a single value (1) denoting a polygon extent (e.g., urban areas, oil spill); yielded average, min, max, and std dev = 1 values.
Regarding the stress factors associated with climate change (sea level rise, sea surface temperature, land temperature, and precipitation), the model showed uniform distributions in the study area. However, it is essential to note that different ecosystems may be more sensitive to these threats than others, leading to differences in the cumulative impact that needs to be measured.
Discussion
We found no standardization in the types of assessments, procedures, or precise definitions of the scope of impact or vulnerability assessments. Neither are the frameworks that operationally link the ecological, socioeconomic (Pavlickova and Vyskupova, 2015) or cultural aspects of socio-ecological systems.
Based on our review and following Leslie et al. (2015) and Jozaei et al. (2022), we believe that the lack of standardization in evaluations and procedures could be because there are still few approaches that incorporate science in CVA (Leslie et al., 2015; Jozaei et al., 2022).
CVA helps to determine the potential effects of climate change on a biophysical system based on information obtained from impact assessments (Dudley et al., 2021) to reduce its risks. This framework assumes risk levels for each RFC supported by identifying “key risks” that represent severe consequences for socio-ecological systems.
A practical climate risk assessment requires information on climate hazards at multiple spatial and temporal scales, exposure, vulnerability, their associates, and uncertainty to help communicate risk and prioritize investments (Arribas et al., 2022).
Due to its geographical location (the northernmost in South America), with 3,726 km of continental coastline and direct access to the Atlantic Ocean and the Caribbean Sea, Venezuela is physically and environmentally highly vulnerable to sea level rise and its effects of erosion and flooding, especially on low-lying coasts (Naveda, 2010; Villamizar et al., 2017; Villamizar, 2020) highlights that it is severe for areas subject to subsidence, such as the south and east coast of Lake Maracaibo (ML) in the state Zulia and the Orinoco Delta in the Delta Amacuro state (DA) (see Table 1).
Villamizar (2020) points out that in trajectories of higher greenhouse gas emissions (temperature increase greater than 1.5°C), as projected by RCP6.0 and RCP8.5, sea level rise by 2100 will reach two meters or even more.
Sea surface water temperature rise (SWTI) has significant negative ecological and socioeconomic consequences for coastal communities. For example, massive bleaching of corals (and other sessile coral reef invertebrates) have been observed during years of very high SWTI, disease outbreaks and worse, the death of many coral colonies.
In the late 1990s, the average coral cover on the Dos Mosquises Sur reef (southwest of the archipelago) was approximately 60%. However, by February 2011, after 2010, it was around 29% (Bastidas et al., 2012; Villamizar et al., 2014).
Thus, it is evident that the Venezuelan coast is environmentally and socioeconomically vulnerable to climate change due to its environmental complexity and the combination of uses (Castillo et al., 2011); such as coastal development (airport, oil, commercial, industrial, and tourist infrastructure) and the high proportion of the coastal population in areas composed of unique or fragile ecosystems such as the western coastal zone of Venezuela.
The western coastal area of Venezuela presents high human pressures such as oil spills (Caporusso et al., 2022), unprocessed open-pit solid waste dumping, soil salinization (Mogollón et al., 2017), and suspended sediments (Ramos et al., 2021) that could endanger the integrity of its high ecosystem heterogeneity (Carmona, 1989).
The first Inventory of Classification of Lands and Resources in the state of Falcón Venezuela (Matteucci et al., 1982) carried out between 1975 and 1985 showed that more than 42% of the surface of the state of Falcón had suffered deterioration and that 41% was damaged in danger of severe or very severe desertification. The inventory followed a landscape approach defining physiognomic units in terms of relief, soil, vegetation, drainage pattern, and climate.
After 37 years, we have found more significant localized pressures in coastal areas with a higher concentration of anthropic activities when evaluating the cumulative effect of human and natural pressures in the coastal region of Falcón state, using the method of Crain et al. (2008).
The coastal landscapes of Falcón state are composed of different classes of connected land and marine cover; artificial surfaces, agricultural areas, forests and semi-natural areas, wetlands, dunes, and bodies of water with a complex dynamic in the provision of ecological services, which gives them a high value as a social and ecological integrator (Kim et al., 2017). However, they are currently subject to multiple disturbances that condition their resilience (Bernhardt and Leslie, 2013; He and Silliman, 2019).
According to our model, the intensity of the impacts increases toward the eastern region of Falcón state from “Moderate to high” varies heterogeneously along the coast and toward the open sea, possibly due to the development of coastal port infrastructure and more remarkable development of population centers (Figure 8).
Along the eastern coast of the Paraguaná Peninsula, the impacts' intensity is distributed heterogeneously with “Medium to high” impact sources. The hard bottoms, the sandy beaches, the coralline communities (located between Adícora and Buchuaco up to Cabo San Román) with modified dunes with road layout, infrastructure development, bush forestation, and intensive agriculture present “high impact”.
The Golfete de Coro shows “Moderate to low” impacts in almost unpopulated areas of the central Mitare River and in the Mitare Delta, where after fishing, the main economic activity is aquaculture, which affects the coastal lagoons, the sandy beach line, and the mangroves due to the removal of soil for the expansion of the areas of cultivation pools and the construction of roads to the facilities.
While in the Western Region, the intensity of the impacts varies from continent to sea, with contributions of sediment, variation in water temperature, and chemical contamination. On the coast, the SRL generates “High” impacts on the mangroves and the flooded areas moderately threatened by the variation of the SRL under the RCP 6.2 scenario.
A marked variation was likely not observed for indicators of climate change due to the homogeneous data of the rasters used in a small area. Therefore, regionalized climate information layers may be required to use these indicators in conjunction with the Halpern model.
Gradients are challenging to map because they require spatial data with sufficient resolution to represent the influence of individual pressures and their combinations on the landscapes of the central coast of Falcón state.
In this coastal region, the complex dynamics of socio-ecological systems make it difficult to estimate the cumulative effects of multiple stress factors on various ecosystems, at least at a local scale (Clark et al., 2016), so visualizing the coastal region as an integral system contributes to understanding the complex interactions between terrestrial and marine ecosystems.
Countries like Venezuela that are particularly vulnerable to climate change due to their large coastal areas with varied climatic conditions and poor economic conditions should consider the cumulative effects of multiple stress factors in the management of their coastal environments and benefit from the advantages that a map of cumulative impacts offers to help communicate the coastal management issues required by decision-makers.
In 1992, Venezuela incorporated Environmental Impact studies into its Natural Resources Code. Around 2010, it began implementing the Strategic Environmental Assessment (Espinoza, 2007), which considers the cumulative, additive, synergistic effects, saturation thresholds, induced impacts, and indirect impacts.
The Venezuelan regulation of the EIA under Decree 1,257 of the “Standards on environmental evaluation of activities likely to degrade the environment” expresses the need to include the CEIA in its presentation (Osorio and Sebastiani, 2013) but does not require it, ignoring in the evaluation of projects perhaps due to the difficulty of mitigating them or because they consider the responsibility of their management solely to the regional or national government (Watkins et al., 2015).
Conclusion
Approaches to assessing climate vulnerability and the cumulative impacts of multiple pressures on coastal zones have diversified as the scope of research broadens.
However, despite advances in research on these assessments, socio-ecological and resilience approaches seem to be lacking, possibly because strategies incorporating social sciences into climate vulnerability assessment are still relatively rare (Leslie et al., 2015).
Although landscape ecological approaches are not yet widely adopted in cumulative impact assessments, they should be done on coastal landscapes under the socio-ecological systems (SES) framework as it provides an interdisciplinary view.
Opportunities also exist to incorporate the principles and tools of landscape ecology, select appropriate spatial and temporal scales for these analyses, and provide tools to inform decisions (Harker et al., 2021).
Given the broad spectrum of research oriented toward CVA and CEIA, we consider the use of SEA2 and CEIA in CVA beneficial because they are adaptable when considering additive, synergistic, or saturation thresholds, as well as induced or indirect impacts.
On the other hand, the method of Halpern et al. (2008) allowed us to identify possible cumulative impacts of multiple natural and anthropic disturbances on the arid coasts of Venezuela. Its application in data-poor areas requires method modifications and adjustments to make it helpful in improving understanding of the current environmental and socioeconomic conditions of other affected regional coastal areas.
They also highlight the difficulties Latin American countries face in regulating their coastline from an integrated perspective since only half of the Latin American nations have legislation on this subject (Botero et al., 2022).
Adaptation measures such as community-based adaptation and ecosystem-based adaptation are not fully integrated into national plans yet (Nagy et al., 2019).
Due to the persistent lack of inclusion of these assessments in regional coastal planning, it is urgent to respond to the need to implement measures to address climate risks and the cumulative effect of multiple pressures on coastal areas.
At the regional level, among the main adaptation actions carried out by Brazil, to a lesser extent, Chile and Uruguay highlight the Strengthening of the National System of Protected Areas Systems, restoration, and maintenance of coastal ecosystem services, continuous monitoring, mapping of risks and loss and damage assessment (Nagy et al., 2019).
It is urgent to have availability, quality, and homogeneity of the scale of the data used to build models of cumulative impacts that offer valuable and applicable information for an Integrated Management of the Coastal Zones of the region. In addition to guaranteeing the reliability of the expert's evaluation, ensuring their participation throughout the development of the study.
Data availability statement
The original contributions presented in the study are included in the article/Supplementary material, further inquiries can be directed to the corresponding author/s.
Author contributions
IO-A was responsible for the project, conceived the manuscript, the writing, figures and tables, and was also responsible for preparing data on habitats/threats and matrices. GS-D was responsible for mapping and applying climate models. NW coordinated the preparation of the maps and the selection of tools. DC and LF were in charge of developing the methodological tool, using the impact model, and reviewing the draft. EV was in charge of preparing the section on vulnerability and risks for Venezuela and revision. SM contributed to the writing of the article. NM supported the section on vulnerability. GN contributed to the conception, writing, and revision of the manuscript. All authors contributed to the article and approved the submitted version.
Acknowledgments
We thank Gina Colónico for collaborating with the transcription of the text and Mario Bidegain for his suggestions on tools for constructing temperature anomaly maps.
Conflict of interest
The authors declare that the research was conducted in the absence of any commercial or financial relationships that could be construed as a potential conflict of interest.
Publisher's note
All claims expressed in this article are solely those of the authors and do not necessarily represent those of their affiliated organizations, or those of the publisher, the editors and the reviewers. Any product that may be evaluated in this article, or claim that may be made by its manufacturer, is not guaranteed or endorsed by the publisher.
Supplementary material
The Supplementary Material for this article can be found online at: https://www.frontiersin.org/articles/10.3389/fclim.2022.1018182/full#supplementary-material
Abbreviations
CEIA, Cumulative Environmental Impact Assessment; CVA, Climate Vulnerability Assessment; SEA2, Strategic Environmental Assessment; RFC, Reasons for Concern about climate change; CVC, Climate vulnerability of coastal areas; SWTI, Sharpening Water Thermal Imagery.
References
ACFIMAN-SACC. (2018). “Primer Reporte Académico de Cambio Climático 2018: Contribución de los Grupos de Trabajo I, II y III al Primer Reporte Académico de Cambio Climático (PRACC) de la Secretaría Académica de Cambio Climático (SACC) de la Academia de Ciencias Físicas, Matemáticas y Naturales (ACFIMAN) de Venezuela,” in EDICIONES ACFIMAN - CITECI, eds A. Villamizar, E. Buroz Castillo, R. Lairet Centeno, and J. A. Gómez (Caracas), 455. Available online at: http://appportal.rect.ucv.ve/vrac_pasantias/vrac_ucv/documentos/PRACC%2023_01_2018.pdf
Aguilera, M., Azócar, A., González-Jiménez, E. (2003). “Venezuela: Un país megadiverso,” in Aguilera, M., Azócar, A., González-Jiménez, E.Biodiversidad en Venezuela. Tomo II. Fundación Polar y Ministerio de Ciencia y Tecnología – FONACIT Caracas 10561074.
Aral, M., Guan, J., and Chang, B. (2012). Dynamic system model to predict global sea-level rise and temperature change. J. Hydrol. Eng. 17, 237–242. doi: 10.1061/(ASCE)HE.1943-5584.0000447
Arribas, A., Fairgrieve, R., Dhu, T., Bell, J., Cornforth, R., Gooley, G., et al. (2022). Climate risk assessment needs urgent improvement. Nat. Commun. 13, 4326. doi: 10.1038/s41467-022-31979-w
Audemard, F. A. M. (1996). Paleoseismicity studies on the Oca-Ancón fault system, north-western Venezuela. Tectonophysics. 259, 67–80. doi: 10.1016/0040-1951(95)00144-1
Barbier, E. B., Hacker, S. D., Kennedy, C., Koch, E. W., Stier, A. C., et al. (2011). The value of estuarine and coastal ecosystem services. Ecol. Monogr. 201, 169–193. doi: 10.1890/10-1510.1
Bastidas, C., Bone, D., Croquer, A., Debrot, D., Garcia, E., et al. (2012). Massive hard coral loss after a severe bleaching event in 2010 at Los Roques, Venezuela. Rev. Biol. Trop. 60, 23–32. doi: 10.15517/rbt.v60i0.19843
Begum, R., Lempert, R., Ali, E., Benjaminsen, T., Bernauer, T., Cramer, W., et al. (2022). “Point of departure and key concepts,” in H.-O. Pörtner, D. C. Roberts, E. S. Poloczanska, K. Mintenbeck, M. Tignor, A. Alegría, M. et al. (Eds.), Climate change 2022: Impacts, adaptation, and vulnerability. Contribution of working group II to the sixth assessment report of the intergovernmental panel on climate change. Cambridge: Cambridge University Press.
Bernhardt, J., and Leslie, H. (2013). Resilience to climate change in coastal marine ecosystems. Annu. Rev. Mar. Sci. 5:371–392. doi: 10.1146/annurev-marine-121211-172411
Bevacqua, A., Yu, D., and Zhang, Y. (2018). Coastal vulnerability: evolving concepts in understanding vulnerable people and places. Environ. Sci. Policy. 82, 19–29. doi: 10.1016/j.envsci.2018.01.006
Blakley, J., and Russell, J. (2022). International progress in cumulative effects assessment: a review of academic literature 2008–2018. J. Environ. Plann. Manage. 65, 186–215. doi: 10.1080/09640568.2021.1882408
Boström, C., Pittman, S., Simenstad, C., and Kneib, R. (2011). Seascape ecology of coastal biogenic habitats: Advances, gaps and challenges. Mar. Ecol. Prog. Ser. 427, 191–217. doi: 10.3354/meps09051
Botero, C., Milanes, C., Cuker, B., and Anfuso, G. (2022). Latin America and the search for a coastal law: lessons from the legislative procedure in Colombia. Sustainability 14, 5168. doi: 10.3390/su14095168
Caporusso, G., Gallo, C., and Tarantino, E. (2022). “Change Detection Analysis Using Sentinel-1 Satellite Data with SNAP and GEE Regarding Oil Spill in Venezuela,” in Gervasi, O., Murgante, B., Misra, S., Rocha, AMAC, Garau, C. (eds) Computational Science and Its Applications – ICCSA 2022 Workshops. ICCSA 2022. Lecture Notes in Computer Science, vol. 13379. Cham: Springer. doi: 10.1007/978-3-031-10545-6_27
Carmona, C. (1989). Caracterización de las costas del estado Falcón. Bol. Inst. Oceanogr. Univ. Oriente 28, 127–134.
Castillo, A., García, M., Padrón, A., Abogado, M., Pino, A., and Pérez, F. (2011). “Integrated Coastal Zone Management in Venezuela: A space with its own identity,” in Proceedings of the 11th International Coastal Symposium. Szczecin, Poland. p. 1320–1325.
Clark, D., Goodwin, E., Sinner, J., Ellis, J., and Singh, G. (2016). Validation and limitations of a cumulative impact model for an estuary. Ocean Coast. Manage. 120, 88–98. doi: 10.1016/j.ocecoaman.2015.11.013
Crain, C., Kroeker, K., and Halpern, B. S. (2008). Interactive and cumulative effects of multiple human stressors in marine systems. Ecol. Lett. 11, 1304–1315. doi: 10.1111/j.1461-0248.2008.01253.x
Cróquer, A., and Weil, E. (2009). Changes in Caribbean coral disease prevalence after the 2005 bleaching event. Dis. Aquat. Org. 87, 33–43. doi: 10.3354/dao02164
Das, S., Ghosh, A., Hazra, S., Ghosh, T., Safra de Campos, R., and Samanta, S. (2020). Linking IPCC AR4 and AR5 frameworks for assessing vulnerability and risk to climate change in the Indian Bengal Delta. Prog. Disaster Sci. 7, 100110. doi: 10.1016/j.pdisas.2020.100110
Del Monaco, C., Haiek, G., Narciso, S., and Galindo, M. (2012). Blanqueamiento masivo de arrecifes de coral inducido por el ENSO 2010, Puerto Cabello, Venezuela. Rev. Biol. Trop.
Dudley, P. N., Rogers, T. L., Morales, M. M., Stoltz, A. D., Sheridan, C. J., Beulke, A. K., et al. (2021). A more comprehensive climate vulnerability assessment framework for fisheries social-ecological systems. Front. Mar. Sci. 8, 674. doi: 10.3389/fmars.2021.678099
Espinoza, G. (2007). Gestión y Fundamentos de Evaluación de Impacto Ambiental. Santiago de Chile: BIC/CED. p. 287.
Fritzsche, K., Schneiderbauer, S., Bubeck, P., Kienberger, S., Buth, M., Zebisch, M., et al. (2014). The Vulnerability Sourcebook: Concept and guidelines for standardized vulnerability assessments. BMZ: Federal Ministry for Economic Cooperation and Development.
Fuldauer, L., Thacker, S., Haggis, R., Fuso-Nerini, F., Nicholls, R. J., and Hall, J. W. (2022). Targeting climate adaptation to safeguard and advance the sustainable development goals. Nat. Commun. 13, 3579. doi: 10.1038/s41467-022-31202-w
Gallego, B. E., and Selvaraj, J. J. (2019). Evaluation of coastal vulnerability for the District of Buenaventura, Colombia: A geospatial approach. Rem. Sens. Applic. 16, 100263. doi: 10.1016/j.rsase.2019.100263
Gaspar, A. G. (2022). Nueve décadas de explotación de sardina Sardinella aurita en Venezuela, revisión crítica: Nine decades of Spanish sardine Sardinella aurita exploitation in Venezuela, a critical revision. Bol. Investig. Mar. Costeras. Available online at: http://boletin.invemar.org.co/ojs/index.php/boletin/article/view/1102
Giakoumi, S., Halpern, B. S., Michel, L. N., Gobert, S., Sini, M., Boudouresque, C. F., et al. (2015). Towards a framework for assessment and management of cumulative human impacts on marine food webs. Conserv. Biol. 29, 1228–34. doi: 10.1111/cobi.12468
Gissi, E., Manea, E., Mazaris, A. D., Fraschetti, S., Almpanidou, V., Bevilacqua, S., et al. (2021). A review of the combined effects of climate change and other local human stressors on the marine environment. Sci. Total Environ. 755, 142564. doi: 10.1016/j.scitotenv.2020.142564
Gómez, A. (2019). Variación a largo término (2002-2016) en la densidad y composición del mesozooplancton indica cambio de régimen en isla Margarita (Venezuela). Boletín de Investigaciones Marinas y Costeras. 47, 85–106.
Gómez, A., Barceló, A., and Mata, E. (2012). Condiciones hidrográficas (2007-2009) al sur de isla Margarita y Península de Araya como indicio de disminución de la fertilidad regional y posible afectación del recurso sardinero venezolano. Boletín del Instituto Oceanográfico de Venezuela Universidad de Oriente. 51, 173–186
Gómez, A., Mata, E., and Gómez, O. (2014). Crisis de la pesca de sardina en Venezuela: variación de la biomasa del fitoplancton en el este de Margarita, una década de estudio (2003-2012). Boletín del Instituto Oceanográfico de Venezuela Universidad de Oriente. 53, 25–36.
González, L. W., Núñez, E., Eslava, N., and Guevara, F. (2020). Estimación del índice de abundancia relativa de la sardina (Sardinella aurita) del estado Nueva Esparta, Venezuela. Boletín del Instituto del Mar del Perú. 35, 127–133.
Hahn, M., Riederer, A., and Foster, S. (2009). The Livelihood Vulnerability Index: a pragmatic approach to assessing risks from climate variability and change—a case study in Mozambique. Global Environ. Change 19, 74–88. doi: 10.1016/j.gloenvcha.2008.11.002
Halpern, B. S., Frazier, M., Potapenko, J., Casey, K. S., Koenig, K., Longo, C., et al. (2015). Spatial and temporal changes in cumulative human impacts on the world's ocean. Nat. Commun. 6, 7615. doi: 10.1038/ncomms8615
Halpern, B. S., and Fujita, R. (2013). Assumptions, challenges, and future directions in cumulative impact analysis. Ecosphere 4, 131. doi: 10.1890/ES13-00181.1
Halpern, B. S., Selkoe, K. A., Micheli, F., and Kappel, C. V. (2007). Evaluating and ranking the vulnerability of global marine ecosystems to anthropogenic threats. Conserv. Biol. 21, 1301–1315. doi: 10.1111/j.1523-1739.2007.00752.x
Halpern, B. S., Walbridge, S., Selkoe, K. A., Kappel, C. V., Micheli, F., D'Agrosa, C., et al. (2008). A global map of human impact on marine ecosystems. Science 319, 948–952. doi: 10.1126/science.1149345
Harker, K. J., Arnold, L., Sutherland, I. J., and Gergel, S. E. (2021). Perspectives from landscape ecology can improve environmental impact assessment. FACETS 6, 358–378. doi: 10.1139/facets-2020-0049
Harvey, E., and Altermatt, F. (2019). Regulation of the functional structure of aquatic communities across spatial scales in a major river network. Ecology 100, e02633. doi: 10.1002/ecy.2633
He, Q., and Silliman, B. R. (2019). Climate change, human impacts, and coastal ecosystems in the anthropocene. Curr. Biol. 29, R1021–R1035. doi: 10.1016/j.cub.2019.08.042
Hodgson, E., Halpern, B., and Essington, T. (2019). Moving beyond silos in cumulative effects assessment. Front. Ecol. Evol. 7, 211. doi: 10.3389/fevo.2019.00211
Hollarsmith, J. A., Therriault, T. W., and Côt,é, I. M. (2021). Practical implementation of cumulative- effects management of marine ecosystems in western North America. Conserv. Biol. 36, e13841. doi: 10.1111/cobi.13841
Hoque, M., Cui, S., Xu, L., Islam, I., Tang, J., and Ding, S. (2019). Assessing agricultural livelihood vulnerability to climate change in coastal Bangladesh. Int. J. Environ. Res. Public Health 16, 4552. doi: 10.3390/ijerph16224552
IPCC. (1996). “Climate change 1995. Impacts, adaptations and mitigation of climate change: scientific-technical analyses,” Contribution of working group II to the second assessment report of the Intergovernmental Panel on Climate Change. edited by Watson, R.T., Zinyowera, M. C., Moss, R. H., and Dokken, D. J. Cambridge: Cambridge University Press.
IPCC. (2014). “Summary for policy makers,” in Field, C. B., Barros, V. R., Dokken, D. J., Mach, K. J., Mastrandrea, M. D., Bilir, T. E., et al. (Eds.). Climate Change 2014: Impacts, Adaptation and Vulnerability. New York: Cambridge University Press. p. 1–34.
IPCC. (2018). “Summary for policymakers,” in MassonDelmotte, V., et al. (Eds). Global warming of 1.5°C. An IPCC Special Report on the impacts of global warming of 1.5°C above pre-industrial levels and related global greenhouse gas emission pathways, in the context of strengthening the global response to the threat of climate change, sustainable development, and efforts to eradicate. Geneva: World Meteorological Organization.
IPCC. (2019). Summary for Policymakers. Pörtner H. O., Roberts, D.C., Masson-Delmotte, V., Zhai, P., Tignor, M., Poloczanska, E., et al. (Ed.), IPCC Special Report on the Ocean and Cryosphere in a Changing Climate.
IPCC. (2021). “Summary for policymakers. In Climate Change 2021: The Physical Science Basis,” in Contribution of Working Group I to the Sixth Assessment Report of the Intergovernmental Panel on Climate Change. Masson-Delmotte, V., Zhai, P., and Pirani, A., et al., eds. (Cambridge: Cambridge University Press). p. 1–41.
Jozaei, J., Chuang, W.-C., Allen, C. R., and Garmestani, A. (2022). Social vulnerability, social-ecological resilience and coastal governance. Global Sustain. 5, e121–9. doi: 10.1017/sus.2022.10
Kasperson, J. X., Kasperson, R. E., and Turner, II, B. L. (Eds.). (1995). Regions at Risk: Comparisons of Threatened Environments. New York, NY: United Nations University Press.
Kim, M., You, S., Chon, J., and Lee, J. (2017). Sustainable land-use planning to improve the coastal resilience of the social-ecological landscape. Sustainability. 9, 1086. doi: 10.3390/su9071086
Korpinen, S., and Andersen, J. (2016). A global review of cumulative pressure and impact assessments in marine environment. Front. Mar. Sci. 3, 153. doi: 10.3389/fmars.2016.00153
Laboy-Nieves, E. N., Klein, E., Conde, J. E., Losada, F., Crus, J. J., and Bone, D. (2001). Mass mortality of tropical marine communities in Morrocoy, Venezuela. Bull. Mar. Sci. 68, 163–179.
Lampis, A. (2013). Vulnerabilidad y adaptación al cambio climático: debates acerca del concepto de vulnerabilidad y su medición. Cuadernos de Geogr. 22, 17–33. doi: 10.15446/rcdg.v22n2.37017
Leslie, H. M., Basurto, X., Nenadovic, M., Sievanen, L., Cavanaugh, K. C., Cota-Nieto, J. J., et al. (2015). Operationalizing the social-ecological systems framework to assess sustainability. PNAS 112, 5979–5984. doi: 10.1073/pnas.1414640112
López, B., Barreto, M. B., and Conde, J. E. (2011). Caracterización de los manglares de zonas semiáridas en el noroccidente de Venezuela. Interciencia. 36, 888–893. Available online at: http://www.redalyc.org/articulo.oa?id=33921507004
Magris, R., Grech, A., and Pressey, R. (2018). Cumulative human impacts on coral reefs: assessing risk and management implications for Brazilian Coral Reefs. Diversity 10, 1–14. doi: 10.3390/d10020026
Marcotte, D., Hung, S. K., and Caquard, S. (2015). Mapping cumulative impacts on Hong Kong's pink dolphin population. Ocean Coast. Manag. 109, 51–63. doi: 10.1016/j.ocecoaman.2015.02.002
Marrero, C., and Rodríguez-Olarte, D. (2017). “Los humedales costeros venezolanos en los escenarios de cambios climáticos: vulnerabilidad, perspectivas y tendencias,” in Botello A.V., S. Villanueva, J. Gutiérrez y J.L. Rojas Galaviz (eds.). Vulnerabilidad de las zonas costeras de Latinoamérica al cambio climático. UJAT, UNAM, UAC. p. 461–476.
Matteucci, S., Colma, A., and Pla, L. (1982). Análisis ecológico regional del estado Falcón. Acta Científica Venezolana. 33, 78–87.
Matteucci, S. D., Colma, A., and Pla, L. (1999). Biodiversidad vegetal en el árido falconiano. Venezuela. Interciencia 24, 300–330.
Medina, E., Velázquez, G., and Hernández, I. (2015). Impacto del calentamiento global y enriquecimiento atmosférico de CO2 sobre cultivos tropicales: la perspectiva para Venezuela. Rev. Fac. Agron. (UCV). 42, 25–37. Available online at: https://www.researchgate.net/publication/306013946_Impacto_del_calentamiento_global_y_enriquecimiento_atmosferico_de_CO2_sobre_cultivos_tropicales_la_perspectiva_para_Venezuela
Mehvar, S., Filatova, T., Sarker, M., Dastgheib, A., and Ranasinghe, R. (2019). Climate change-driven losses in ecosystem services of coastal wetlands: a case study in the West coast of Bangladesh. Ocean Coast. Manag. 169, 273–283. doi: 10.1016/j.ocecoaman.2018.12.009
Micheli, F., Halpern, B. S., Walbridge, S., Ciriaco, S., Ferretti, F., Fraschetti, S., et al. (2013). Cumulative human impacts on Mediterranean and Black Sea marine ecosystems: assessing current pressures and opportunities. PLoS ONE 8, e79889. doi: 10.1371/journal.pone.0079889
Mogollón, J. P., Rivas, W., Rivas, J. G., and Martínez, A. (2017). Procesos de degradación de suelos asociados a la desertificación en la península de Paraguaná, Venezuela. Ágora de Heterodoxias. 3, 94–110.
MPPEA-IICNCC. (2017). Ministerio del Poder Popular para el Ecosocialismo y Aguas. Segunda Comunicación sobre Cambio Climático.
Nagy, G. J., Gutiérrez, O., Brugnoli, E., Verocai, J., Gómez-Evache, M., Villamizar, A., et al. (2019). Climate vulnerability, impacts and adaptation in Central and South America coastal areas. Reg. Stud. Mar. Sci. 29, 100683. doi: 10.1016/j.rsma.2019.100683
Naveda, J. (2010). Variación en el nivel del mar como consecuencia del cambio climático global: una evaluación de la costa venezolana. Rev. Temas de Coyuntura 61, 63–87.
Newton, J., Icely, J., Cristina, S., Perillo, G. M. E., Turner, R. E., Ashan, D., et al. (2020). Anthropogenic, direct pressures on coastal wetlands. Front. Ecol. Evol. 8, 144. doi: 10.3389/fevo.2020.00144
Olivares, L., and Piñero, A. (2010). Los ciclones tropicales en las costas de Venezuela e Isla de Aves. Período 1851-2008. Tecnocolor.
Olivo-Garrido, M. L., Martín-Zazo, A., Sáez-Sáez, V., and Soto-Olivo, A. (2011). Vulnerabilidad al incremento del nivel del mar. Pérdidas de tierra en el área Cabo Codera-Laguna de Tacarigua, estado Miranda, Venezuela. Terra 27, 125–145.
O'Neill, B., van Aalst, M., Zaiton Ibrahim, Z., Berrang Ford, L., Bhadwal, S., Buhaug, H., et al. (2022). “Key risks across sectors and regions,” in Pörtner, H.-O., Roberts, D.C., Tignor, M., Poloczanska, E.S., Mintenbeck, K., Alegría, A. et al. (Eds.) Climate change. 2022. impacts, adaptation, and vulnerability. Contribution of Working Group II to the Sixth Assessment Report of the Intergovernmental Panel on Climate Change. Cambridge University Press. In press.
Oppenheimer, M., Campos, M., Warren, R., Birkmann, J., Luber, G., O'Neill, B., et al. (2014). “Emergent Risks and Key Vulnerabilities,” in Field, C.B., Barros, V., Mach, K., Mastrandrea, M. (Eds.), Contribution of Working Group II to the IPCC Fifth Assessment Report.
Osorio, P., and Sebastiani, M. (2013). Propuesta para la fase inicial de los estudios de impacto ambiental y sociocultural en el contexto legal venezolano. Rev. Geográfica Venezolana. 54, 67–83.
Pavlickova, K., and Vyskupova, M. (2015). A method proposal for cumulative environmental impact assessment based on the landscape vulnerability evaluation Environ. Impact Assess. Rev. 50, 74–84. doi: 10.1016/j.eiar.2014.08.011
Pérez, D., and Galindo, L. (2000). Effects of hyposalinity in Thalassia testudinum (Hydrocharitaceae) from Parque Nacional Morrocoy, Venezuela. Rev. Biol. Trop. 48, 251–260.
Pittman, S., Kneib, R., and Simenstad, C. A. (2011). Practicing coastal seascape ecology. Mar. Ecol. Prog. Ser. 427, 187–90. doi: 10.3354/meps09139
Quemmerais-Amice, F., Barrere, J., La Rivière, M., Contin, G., and Bailly, D. A. (2020). Methodology and tool for mapping the risk of cumulative effects on benthic habitats. Front. Mar. Sci. 7, 7. doi: 10.3389/fmars.2020.569205
Ramos, R., Verde, A., and García, E. M. (2021). Heavy metals in Venezuelan marine sediments: concentrations, degrees of contamination and distribution. Cienc. Mar. 47, 185–189. doi: 10.7773/cm.v47i3.3124
Rodríguez, J. P., and Rojas-Suárez, F., Giraldo, D. (2010). Libro Rojo de los ecosistemas terrestres de Venezuela. Caracas: Provita, Lenovo, Shell de Venezuela.
Schneider, S. H., Semenov, S., Patwardhan, A., Burton, I., Magadza, C. H. D., Oppenheimer, M., et al. (2007). “Assessing key vulnerabilities and the risk from climate change,” in Climate Change 2007: Impacts, Adaptation and Vulnerability Contribution of Working Group II to the Fourth Assessment Report of the Intergovernmental Panel on Climate Change. Cambridge, UK: Cambridge University Press. p. 779–810.
Sevilla, N. P. M., and Le Bail, M. (2017). Latin American and caribbean regional perspective on ecosystem based management (EBM) of large marine ecosystems goods and services. Environ. Dev. 22, 9–17. doi: 10.1016/j.envdev.2017.01.006
Sharma, J., and Ravindranath, N. (2019). Applying IPCC, 2014 framework for hazard-specific vulnerability assessment under climate change. Environ. Res. Commun. 1, 51004. doi: 10.1088/2515-7620/ab24ed
Singh, C., Tebboth, M., Spear, D., Ansah, P., and Mensah, A. (2019). Exploring methodological approaches to assess climate change vulnerability and adaptation: reflections from using life history approaches. Reg. Environ. Chang. 19, 2667–82. doi: 10.1007/s10113-019-01562-z
Smale, D. A., Wernberg, T., Oliver, E. C. J., Thomsen, M., Harvey, B. P., Straub, S. C., et al. (2019). Marine heatwaves threaten global biodiversity and the provision of ecosystem services. Nat. Clim. Chang. 9, 306–312. doi: 10.1038/s41558-019-0412-1
Spalding, M. D., Ruffo, S., Lacambra, C., Meliane, I., Hale, L. Z., Shepard, C. C., et al. (2014). The role of ecosystems in coastal protection: adapting to climate change and coastal hazards. Ocean Coastal Manage. 90, 50–57. doi: 10.1016/j.ocecoaman.2013.09.007
Stelzenmüller, V., Coll, M., Mazaris, A. D., Giakoumi, S., Katsanevakis, S., Portman, M. E., et al. (2018). A risk-based approach to cumulative effect assessments for marine management. Sci. Total Environ. 612, 1132–1140. doi: 10.1016/j.scitotenv.2017.08.289
Tarabon, S., Bergès, L., Dutoit, T., and Isselin-Nondedeu, F. (2019). Environmental impact assessment of development projects improved by merging species distribution and habitat connectivity modelling. J. Environ. Manage. 241, 439–449. doi: 10.1016/j.jenvman.2019.02.031
Taylor, G., Müller-Karger, F., Thunell, R., Scranton, M., Astor, Y., Varela, R., et al. (2012). Ecosystem responses in the southern Caribbean Sea to global climate change. Proc. Nat. Acad. Sci. 109, 19315–19320. doi: 10.1073/pnas.1207514109
Ury, E., Yang, X., Wright, J., and Bernhardt, E. (2021). Rapid deforestation of a coastal landscape driven by sea level rise and extreme events. Ecol. Appl. 31, e02339. doi: 10.1002/eap.2339
Vilardo, C., and La Rovere, E. (2018). Multi-project environmental impact assessment: insights from offshore oil and gas development in Brazil. Impact Assess Project Appraisal 36, 358–370. doi: 10.1080/14615517.2018.1475615
Villamizar, A. (2020). Medidas de adaptación al aumento del nivel del mar considerando las trayectorias de concentración representativa. Boletín Acad. Cienc. Físicas, Matemáticas Natur. 73, 41–77.
Villamizar, A., Gutiérrez, M., Nagy, G., Caffera, R., and Leal, W. (2017). Climate adaptation in South America with emphasis in coastal areas: the state-of-the-art and case studies from Venezuela and Uruguay. Clim. Dev. 9, 364–382. doi: 10.1080/17565529.2016.1146120
Villamizar, E., Camisotti, H., Rodríguez, B., Pérez, J., and Romero, M. (2008). Impacts of the 2005 Caribbean bleaching event at Archipiélago de Los Roques National Park, Venezuela. Rev. Biol. Trop. 56, 255–270.
Villamizar, E., Posada, J. M., and Gómez, S. (2003). “Rapid assessment of coral reefs in the Archipiélago de Los Roques National Park, Venezuela (Part I: stony corals and algae),” in Status of Coral Reefs in the Western Atlantic: Results of Initial Surveys, Atlantic and Gulf Rapid Reef Assessment, ed J. C. Lang. p. 512–529.
Villamizar, E., Yranzo, A., González, M., Herrera, A. T., and Pérez, J., Camissotti, H. (2014). Diversidad y condición de salud de corales pétreos en algunos arrecifes del Parque Nacional Archipiélago de los Roques, Venezuela. Acta Biol. Venezuelica. 34, 257–279.
Wamsley, T. V., Collier, Z. A., Brodie, K., Dunkin, L. M., Raff, D., and Rosati, J. D. (2015). Guidance for developing coastal vulnerability metrics. J. Coast. Res. 31, 1521–1530. doi: 10.2112/JCOASTRES-D-14-00015.1
Watkins, G., Atkinson, R., Canfield, E., Corrales, D., Dixon, J., et al. (2015). Guía para evaluar y gestionar los impactos y riesgos para la biodiversidad en los proyectos respaldados por el Banco Interamericano de Desarrollo.| Publications. Biblioteca Felipe Herrera del Banco Interamericano de Desarrollo.
White, E., Ury, E., Bernhardt, E., and Yang, X. (2022). Climate change driving widespread loss of coastal forested wetlands throughout the North American coastal plain. Ecosystems 25, 812–827. doi: 10.1007/s10021-021-00686-w
Wu, X., Hao, Z., Zhang, X., Li, C., and Hao, F. (2020). Evaluation of severity changes of compound dry and hot events in China based on a multivariate multi-index approach J. Hydrol. 583, 124580. doi: 10.1016/j.jhydrol.2020.124580
Keywords: coastal ecosystems, threats, adaptation, socio-ecological systems, environmental impact, marine ecosystems, climate risks
Citation: Olivares-Aguilar IC, Sánchez-Dávila G, Wildermann NE, Clark D, Floerl L, Villamizar E, Matteucci SD, Muñoz Sevilla NP and Nagy GJ (2022) Methodological approaches to assess climate vulnerability and cumulative impacts on coastal landscapes. Front. Clim. 4:1018182. doi: 10.3389/fclim.2022.1018182
Received: 12 August 2022; Accepted: 03 October 2022;
Published: 26 October 2022.
Edited by:
Rajiv Pandey, Indian Council of Forestry Research and Education (ICFRE), IndiaReviewed by:
Surendra Singh Jatav, Babasaheb Bhimrao Ambedkar University, IndiaManob Das, University of Gour Banga, India
Copyright © 2022 Olivares-Aguilar, Sánchez-Dávila, Wildermann, Clark, Floerl, Villamizar, Matteucci, Muñoz Sevilla and Nagy. This is an open-access article distributed under the terms of the Creative Commons Attribution License (CC BY). The use, distribution or reproduction in other forums is permitted, provided the original author(s) and the copyright owner(s) are credited and that the original publication in this journal is cited, in accordance with accepted academic practice. No use, distribution or reproduction is permitted which does not comply with these terms.
*Correspondence: Isabel C. Olivares-Aguilar, cGFsZW9lY29sb2dpYS5jaW1hckBnbWFpbC5jb20=