- 1European Commission, Joint Research Centre (JRC), Ispra, Italy
- 2Department of Physical Geography, Utrecht University, Utrecht, Netherlands
- 3Department of Geosciences, University of Oslo, Oslo, Norway
The Mediterranean is an area where the balance between water demand and abstractions vs. water availability is often under stress already, as demonstrated here with the Water Exploitation Index. In this work, model estimates on how different proposed measures for water resources management would affect different indicators. After a review of the current water resources status in the Mediterranean and the definition of indicators used in this study, aspects interlinked with water in the Water-Energy-Food-Ecosystems Nexus are briefly discussed, focusing on problems linked with water scarcity and depletion of groundwater resources as well as with climate change projections. Subsequently, the proposed measures for water efficiency are detailed—irrigation efficiency, urban water efficiency, water reuse and desalination—that might be effective to reduce the growing water scarcity problems in the Mediterranean. Their effects that result from the LISFLOOD model, show that wastewater reuse, desalination and water supply leakage reduction lead to decreased abstractions, but do not affect net water consumption. Increased irrigation efficiency does decrease consumption and reduces abstractions as well. We deduct however that the current envisaged water efficiency measures might not be sufficient to keep up with the pace of diminishing water availability due to climate change. More ambition is needed on water efficiency in the Mediterranean to keep water scarcity at bay.
Introduction
The Mediterranean region has already long been recognized as a region where water resources are limited by the climate. Already in their “Blue Plan—Futures for the Mediterranean Basin” Grenon and Batisse (1989) stated that “solutions (for inland waters) are rather well known” and “adaptations” would be “necessary sooner or later.” They also warned to “closely follow the consequences of the trend toward a heating up of the climate due to the greenhouse effect,” already more than three decades ago (1989!). The “Blue Plan” also recognized the interconnections between climate, soils, water constraints, the energy sector, agriculture, demographics and economics. But actually, already the Romans found a way to thrive in the water-limited environment of the Mediterranean with smart agricultural practices and water transfers (Dermody et al., 2014).
This is exactly what the Water-Energy-Food-Ecosystem Nexus (WEFE) approach aims to demonstrate: energy production and food production depend on available water resources, and they need to be examined together. Once water is abstracted and consumed by one sector it is not available anymore for the other sectors: the ecosystem, energy sector, agricultural sector, industrial sector, and the public sector (for drinking water and sanitation). Water abstraction as such needs to be differentiated in water that is eventually returned to the hydrological system—e.g., return flows of cooling water from thermal power plants, deeper infiltration of irrigation water in soils, treated waste water return flow to rivers -, and water that is “lost” to the atmosphere by evaporation of cooling towers or irrigation water.
WEFE—sometimes called Water, Energy and Food Security (WEF) Nexus, but we added the “Ecosystem” here—originated from a conference in Bonn in 2011, after which the concept was followed on by several organizations, including the UNECE, FAO, World Economic Forum (2011) and the JRC. WEFE builds on the Integrated Water Resources Management (IWRM) principles, but differs from IWRM by not explicitly focusing on water, and takes food security and energy security on board as well.
Studies in the past, have focused on a more systemic approach of the nexus in a river basin (Lawford et al., 2013; Shi et al., 2020), or a whole country (Daher and Mohtar, 2015), but in this study, a different approach starts from a physically based model, to ensure the best available precision of water availability and irrigation requirement. This precision is necessary to subsequently being able to simulate different scenarios with exact locations of measures. The focus on water related indices in this study does not mean that the other components of the WEFE Nexus have been neglected. On the contrary, based on previous experience (De Roo et al., 2020), the model incorporates data or modules for climatic projections, energy production, agricultural practices, land use, data concerning planned interventions on infrastructure (e.g., urban efficiency) at a very detailed scale throughout the European Union.
Especially where water resources are scarce during—parts of—a year, it is of crucial importance that we understand the water interdependencies of the various WEFE sectors with respect to the availability of water resources. Timely adaptation to future challenges of climate change and its effects on water resources is essential. How will economic growth and demographic changes influence public and industrial water demand? Will the energy transition toward renewable sources have an effect on water demand and consumption? Will the climate get wetter or drier and are there seasonal variations that affect water availability? Will agriculture practices under a warmer climate lead to increased irrigation water demand, or can farmers change to less water-demanding crop-types?
This paper aims to provide insight in some of the current water resources problems focusing on the Mediterranean—as a WEFE hotspot in Europe -, with areas of groundwater depletion, and rivers with low flow issues. We describe projections of future water resources as a consequence of climate change comparing them to current water resources, and envisaged changes in water demands due to higher temperatures and population changes. Thereafter, we describe possible measures in the field of agriculture and energy that might reduce water scarcity and would help to adapt to the changing water resources, and we demonstrate their effects on water resources in the Mediterranean.
Current Water Resources in the Mediterranean
The water cycle in the Mediterranean is characterized by dry summers frequently associated with long drought episodes, alternated by autumn and winter rainfall that can be very intense at times (Ducrocq et al., 2016). Precipitation but also seasonal mean temperature have a high spatial and temporal variability.
Water scarcity was and is a situation familiar to the entire Mediterranean region. However, a lack of water is also for growing concern in many other EU Member States, many of which until recently believed to have sufficient water. At present, there are ~52 million people or 11% of the population of the 27 states of the European Union plus the United Kingdom living in water scarce regions (Bisselink et al., 2020). This means that at least during part of the year, water demand can scarcely be satisfied by the available freshwater. Of the aforementioned 52 million people, the majority live in Southern European countries including Spain (22 million; 50% of the national population), Italy (15 million; 26%), Greece (5.4 million; 49%) and Portugal (3.9 million; 41%). Furthermore, the entire population of Cyprus and Malta is considered to be living in conditions of water scarcity. During the summer, the exploitation of water in the Mediterranean area approaches 100%, meaning that all possible freshwater is being used, often including a substantial amount of fossil groundwater resources, causing groundwater depletion.
Water is being employed not only for drinking and sanitation, but also for agricultural irrigation, the cooling of electricity-production facilities, for industrial manufacturing (e.g., paper, textiles, soft drinks) and for the rearing of livestock. Furthermore, water is required to produce hydropower, while sufficient water needs to remain in rivers, lakes, and groundwater for ecological reasons. During episodes of low water availability, the demand for water may exceed the capacity to supply, which can lead to a series of conflicting situations.
Indicators of Quantitative Pressures on Water Resources
We express the ratio between water consumption and water availability with the so-called Water Exploitation Index (WEI+). WEI+ illustrates the pressure on renewable freshwater resources due to water demand.
Water availability in a region is the local precipitation minus the evapotranspiration, but if appropriate added with river inflow coming from upstream countries. Best example here is Egypt, which has few annual runoff from local precipitation, but does have inflow water through the River Nile.
Net water consumption equals all water abstractions minus return flows. Water used for cooling power plants is partially returned back to the hydrological system, be it slightly warmer. Drinking water abstractions in the end typically largely flow back as treated wastewater.
WEI+ values above 20% (we use fraction in this paper, thus WEI+ >0.20) are generally considered as an indication of water scarcity, while values equal or bigger than 40% (WEI+ > 0.40) indicate situations of severe water scarcity (EEA, 2015). In the case of WEI+ values larger than 40%, the use of freshwater resources is likely unsustainable, with likely areas of groundwater depletion.
In this paper, we also use the WEI indicator, which the Water Exploitation Index based on abstraction vs. availability, which we will also refer to as WEIabstraction to distinguish it from WEI+. WEIabstraction is always larger than WEI+. The difference between them is the accounting for the return flows of irrigation, cooling water, and wastewater.
In Figure 1 we have estimated the WEI+ using the pan-European version of the integrated agro-hydrological LISFLOOD model (De Roo et al., 2000; Van Der Knijff et al., 2010; Burek et al., 2013; Gelati et al., 2020) forced with observed weather data 1990-2018, water demand data, and relevant soils, land use and vegetation data. The latest version of LISFLOOD has an integrated irrigation and crop yield module, thus irrigation water demand is estimated from the specific crop demand. LISFLOOD is calibrated and validated using observed river flows from more than thousand gauging stations, and is constantly updated with improved data and physics. LISFLOOD uses an ecological flow threshold—in this study set as the local 10th percentile of river flow—below which abstractions are interrupted to allow for minimum flow conditions of a river.
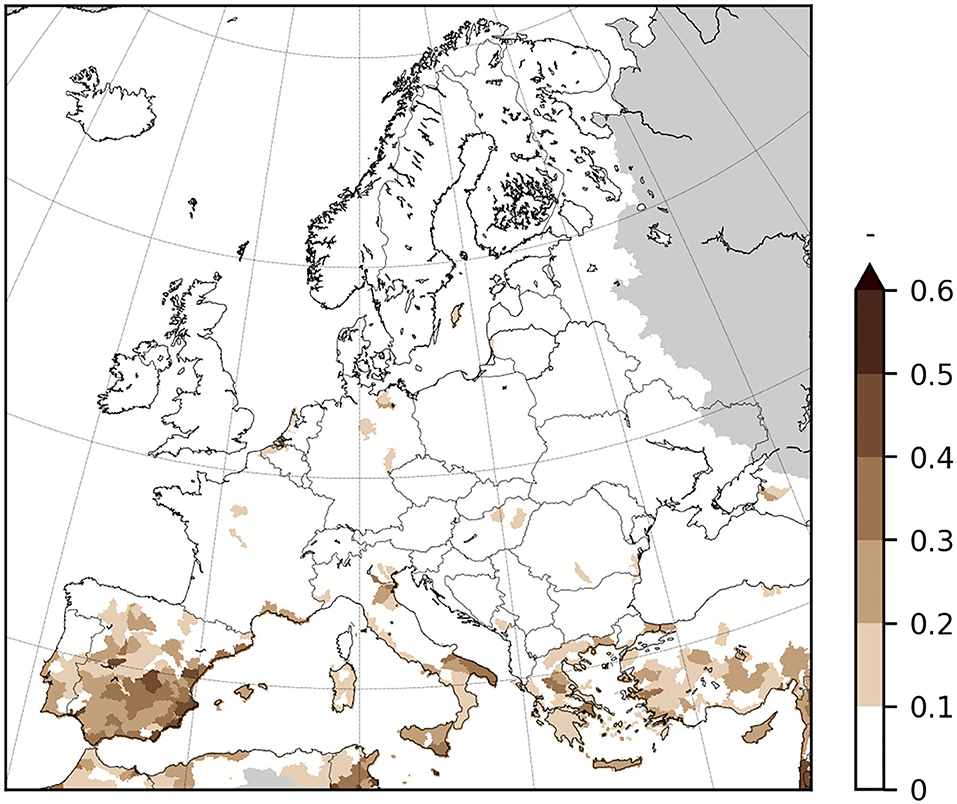
Figure 1. The Water Exploitation Index (WEI+) for present day climate (1990–2018) as simulated with the LISFLOOD model.
WEI+ is calculated monthly at sub-riverbasin district level, as visible by the regions in Figure 1.
Figure 2 shows a country average WEI+ values, indicating the countries with the largest values: Cyprus, Spain, Greece, Italy, Malta, and Portugal. Several other European countries have local hotspots of increased WEI+ values. In this paper, we will focus on the Mediterranean countries.
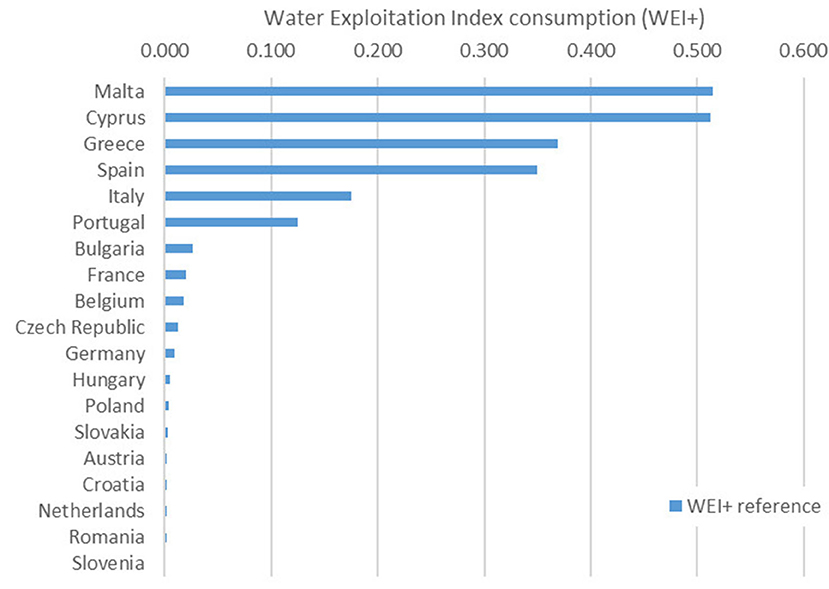
Figure 2. The Water Exploitation Index (WEI+), estimated using the LISFLOOD model, averaged for European countries for 1990–2018.
Water Scarcity and Groundwater Depletion
The WEI+ calculations show that in several parts of Europe, the annual renewable freshwater is used in an unsustainable way, and this is thus further confirmed by the estimates of groundwater depletion.
In addition to the WEI+ indicator, we have been assessing possible areas of longterm groundwater depletion with the LISFLOOD model (Gelati et al., 2020). LISFLOOD consists of a groundwater storage component, fed by recharge, and releasing water as baseflow to the river system. From the groundwater storage, water abstractions are possible. We use FAO Aquastat and Eurostat data to compute percentages of surface and groundwater being abstracted in a country. For irrigation, such percentages are available at sub-national administrative level (Eurostat, 2021). The various sectorial water demands determine then where water is abstracted.
Groundwater depletion is deducted in the model if there is a statistically significant decreasing trend in groundwater storage over the 1990–2018 simulation period, using the Mann-Kendall trends test. This test not only accounts for differences in groundwater resources at the end of the simulation vs. the start of the simulation, but also for the variability of the time series. Gelati et al. (2020) also evaluated the likely source of groundwater depletion. When we excluded irrigation—and separately also other water abstractions—from the model simulation, only a few areas were left with some groundwater depletion, which we assume to originate from climatic changes between 1990 and 2018.
Figure 3 shows estimates of groundwater depletion for Europe averaged over sub-riverbasin districts, with some major groundwater depletion regions in southern Spain, Sicily, Greece, Turkey and Cyprus. We estimate rates of depletion up to 92 mm water per year (Crete). Gelati et al. (2020) compared these groundwater depletion estimates with reported groundwater level measurements in Spain and found a reasonable agreement between large scale patterns.
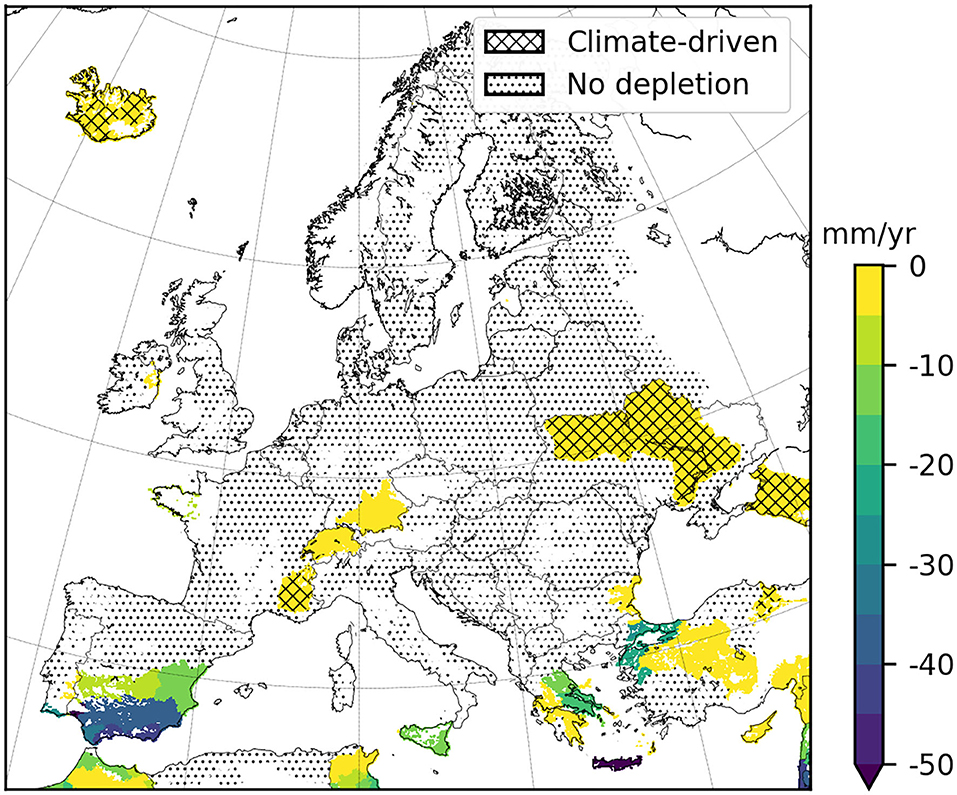
Figure 3. Groundwater depletion between 2018 and 1990, as estimated with the LISFLOOD model (from Gelati et al., 2020).
Climate Change Projections of Water Resources
While we already demonstrated issues of water scarcity and groundwater depletion, with some major challenging areas in the Mediterranean for present climate, we assessed that climate change might result in even larger problems (Bisselink et al., 2020). While forcing the LISFLOOD model with 11 Euro-Cordex climate scenarios and various RCP emission scenarios, we deducted that the Mediterranean is projected to face diminishing water availability.
In a projected future climate, the southern part of Europe shows a strong increase in annual average temperature with an even stronger increase in summer temperature. For most parts of the Mediterranean, the annual precipitation is projected to decline with a much stronger decrease in summer precipitation. More details about future climate projection for Europe can be found in Dosio (2020).
The projected change in meteorological conditions results in an increase of the frequency and severity of hydrological droughts with a progressively decrease in water availability in the southern European regions.
Figure 4 represents the spatial pattern of change in WEI+ for the global warming level of 2°C relative to the baseline (Figure 1). The largest increases in WEI+ of 30% or more take place in the Mediterranean region with WEI+ values exacerbating in already existing water scarce areas. Apart from an intensification of water scarcity under global warming, the duration of the water scarcity period in a year is projected to increase as well with an increase up to more than 1 month per year for the southern part of Spain.
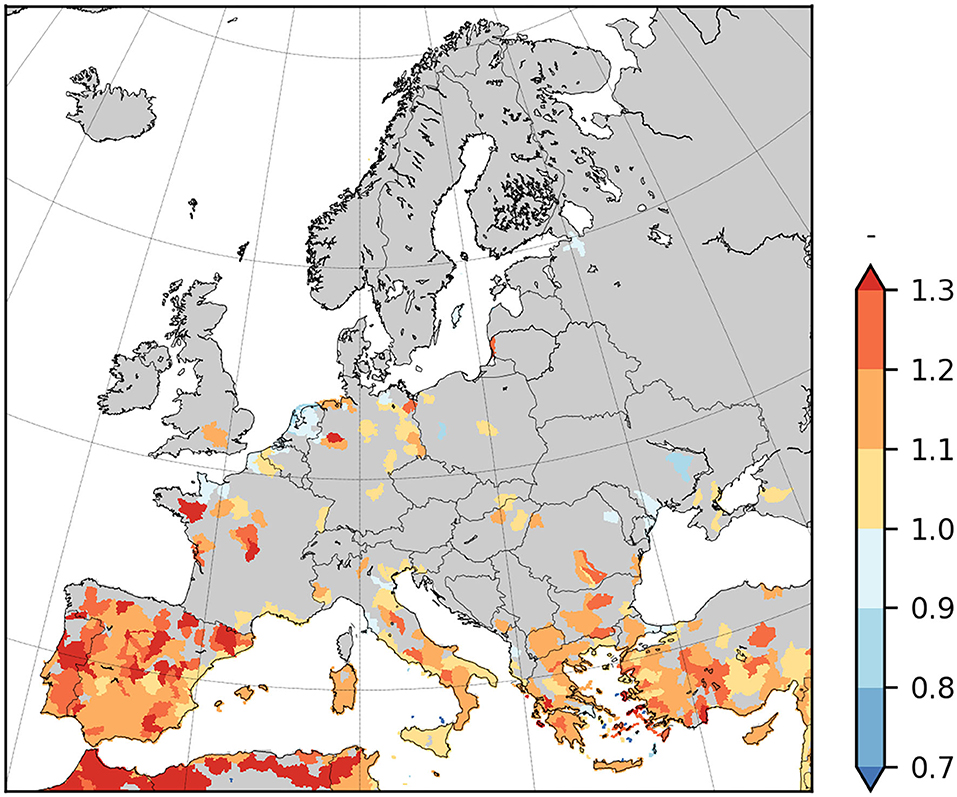
Figure 4. Changes in Water Exploitation Index (WEI+) due to a 2-degree warmer climate as compared to the 1981–2010 climate (from Bisselink et al., 2020). Ratios are shown here, indicating increases in WEI+ due to climate change (ratios larger than 1) or decreases in WEI+ (ratios smaller than 1).
Consequently, Spain sees the largest absolute increase in the number of people living in areas considered to be water scarce. In the 2°C warming scenario these amount to over 1 million additional people relative to present. In other countries around the Mediterranean, an increase in the number of people is projected to have serious water scarcity exposure as well (Bisselink et al., 2020).
Measures of Water Efficiency
With the balance of water demand and water availability changing toward increased water scarcity in various parts of Europe, measures need implementing that reduce net water consumption. From Eurostat reported data on water abstractions (Figure 5) we can deduct that the agricultural and energy sector are major water abstractors. A part of the abstracted water is returned to the hydrological system, which is especially the case in the energy and industrial sector, where cooling water—depending on the cooling method applied—is returned with a slightly higher temperature to surface water bodies.
If we therefore look at net water consumption—abstractions minus return flows—we estimate that the agricultural sector is by far the largest net consumer of water, mainly for crop irrigation, and a small part for livestock.
Therefore, measures of water savings would likely be most effective in the agricultural sector, and next in the energy sector.
In this paper, we evaluate four different measures that may reduce water abstraction and net water consumption:
• Increasing irrigation efficiency in agriculture
• Re-using treated urban wastewater for irrigated agriculture
• Increasing urban water efficiency by reducing leakage
• Use of desalination of sea water for public water use
We describe the measures below. In the next chapter we will then evaluate the impact of these measures on water resources in Europe, with a special focus on the Mediterranean.
Irrigation Efficiency
As part of the European Member States (MS) River Basin Management Plans and Regional Investment Plans, countries are planning to invest in their irrigation areas to make them more water efficient, often changing the irrigation method from surface and sprinkling toward drip irrigation. The reported investments in irrigation efficiency as planned by MS and collected by Benitez Sanz et al. (2018) were used in the LISFLOOD model (Gelati et al., 2020), where we can distinguish between the type of irrigation applied: surface including rice paddies, sprinkling and drip. Within LISFLOOD we account in every single model pixel of 5 × 5 km for the percentage of forests, urban area, open water, crops (32 crops including paddy rice), and other land uses. For each crop in each pixel we can indicate rainfed and irrigated areas and, within the latter, the extent to which each irrigation method is applied.
Figure 6 shows the areal percentage of planned irrigation efficiency improvements in the most relevant European countries up to 2030. Most MS plan only marginal new investments in irrigation efficiency up to 2030. We have implemented these changes as shifts from sprinkling irrigation toward drip irrigation. Spain, Greece, Italy, and Portugal plan more changes, but still restricted to 5–10% of the total irrigated area.
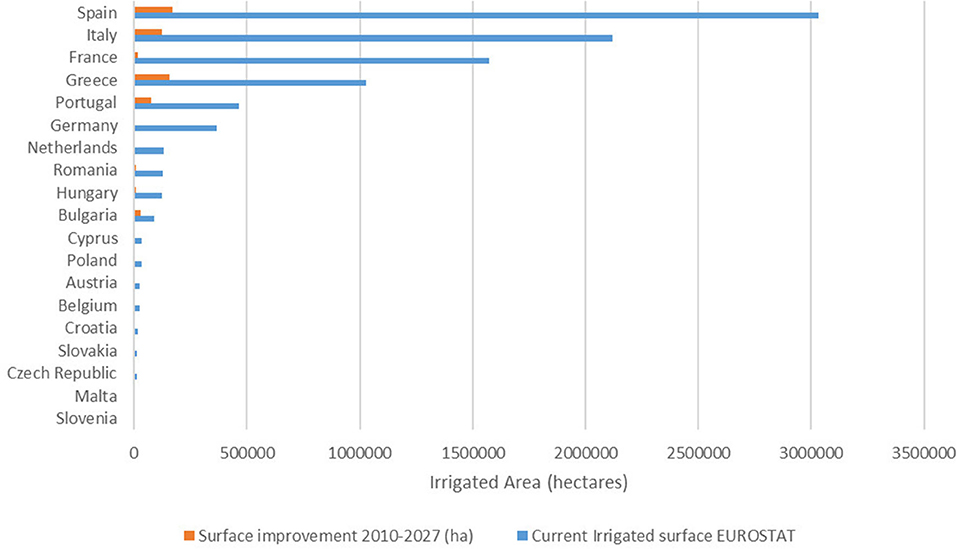
Figure 6. Planned investments in irrigation to improve water efficiency (source data from Benitez Sanz et al., 2018).
Water Re-use
The second type of measure we considered in this work is water re-use. Reuse of treated wastewater for agricultural irrigation is a possible measure to reduce water stress. In a European scale hydro-economic analysis, Pistocchi et al. (2017) quantified the volumes of treated domestic wastewater that could be reused in agriculture at a cost below given thresholds. The costs were appraised including the additional treatment of wastewater effluents in order to meet European quality standards, as well as the cost of transport and storage of water. The volume of wastewater that may be reused economically depends for a large part on the distance between a wastewater treatment plant and the irrigated areas, and varies significantly across Europe. Pistocchi et al. (2017) estimated the volumes of treated wastewater available for irrigation in all European regions at leveled costs—including Capital expenditure (CAPEX) and Operational Expenditure (OPEX)—below 0.25, 0.50, 0.75, and 1.00 euro/m3. The scenario with costs not exceeding a threshold of 0.25 euro/m3 yields in very little water re-use, and has been omitted in this study. In this study, we evaluate the amount of water re-use with costs not exceeding a threshold of 0.50 euro/m3 (Figure 7), which is still a rather conservative scenario.
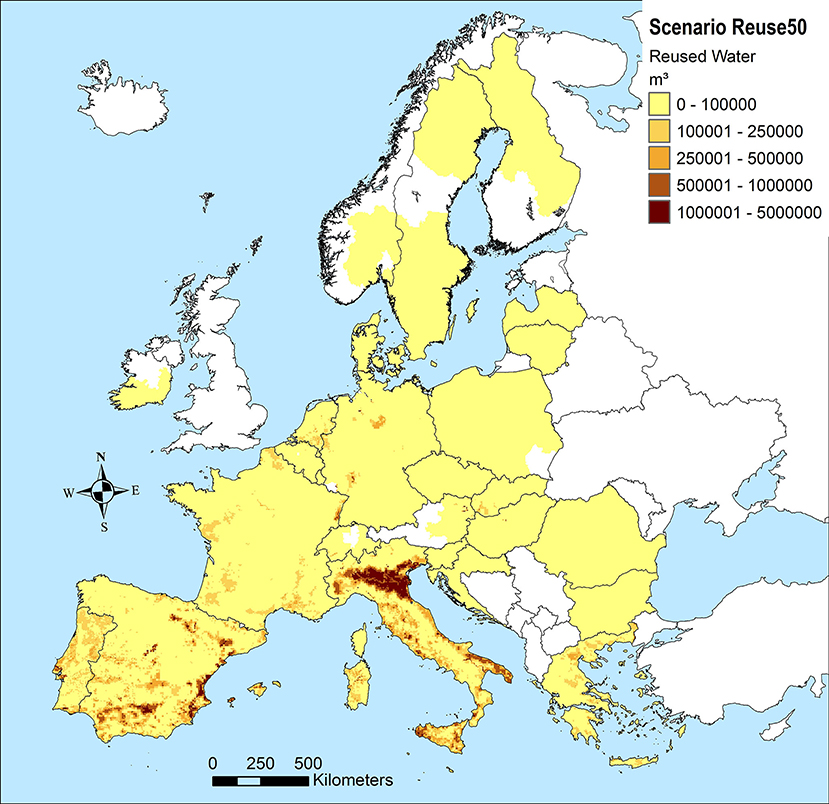
Figure 7. Amounts of water re-use (m3 per 25 km2) under the 0.50 euro/m3 scenario. See text. Data from Pistocchi et al. (2017).
If the treated wastewater to be reused is taken from freshwater bodies, reuse reduces abstractions. However, in principle it does not affect the overall consumptive water use. Therefore, water re-use will not effect the WEI+ (consumption), but it will reduce the WEIabstraction.
Desalination
A net reduction of consumptive water use is provided by desalination. Although largely regarded as a “brute force”—last resort solution—until recent times, desalination is increasingly affordable and may be turned into a sustainable solution with appropriate planning (Pistocchi et al., 2020).
Pistocchi et al. (2018), estimated the cost of supplying desalinated water across the Mediterranean region, taking into account the costs of developing and maintaining the infrastructure, and of pumping desalinated water inland from coastal areas. While desalinated water is in principle unlimited, its supply is constrained by the acceptable costs. The authors estimated the population potentially served by desalination in all European regions, at levelized costs not exceeding 0.50, 1.00 Euro/m3, and higher costs. This corresponds to a volume of wastewater potentially available for reuse, determined by the supply per capita and the percentage of the supply that ends up in the wastewater treatment plant. In this exercise, we estimate the supply per capita at 200 l/person/day, and the percentage ending in wastewater at 80%.
Urban Water Use Efficiency: Leakage Reduction
The fourth measure to potentially reduce water scarcity issues that is considered in this study is a reduction of leakage of the public water supply network. Losses from urban water networks in Europe range from around 5% in Germany and The Netherlands up to 40–50% in Malta and Ireland (Benitez Sanz et al., 2018). Water scarce countries such as Spain (29% leakage), Italy (38%), Greece (21%) and Cyprus (24%) all lose considerable amounts of water in the public supply network.
Benitez Sanz et al. (2018) used the public investments plans by the European countries envisaged between 2016 and 2027 to estimate the efficiency improvements that could be reached in the urban water supply. We have been using the baseline and leakage reduction scenario here to assess the potential improvement in water efficiency that could be achieved.
Effects of Measures on Water Resources
With the LISFLOOD model we have assessed the reference water resources situation 1990–2018. For this reference period, LISFLOOD is calibrated against observed discharge using 1,137 gauging stations from 215 river basins in Europe. State of the art observed meteorological data and water demand datasets are used.
For this paper, we assessed the measures individually, to examine their effects on water resources. Simulations with combined measures, which will have some cross over effects, will be dealt with in a subsequent paper.
Figure 8 shows the effect of the four examined measures on the WEIabstraction. While the WEI and WEI+ are calculated on a sub-riverbasin basis (see Figure 1), the results are averaged here per country to achieve a better insight in the results.
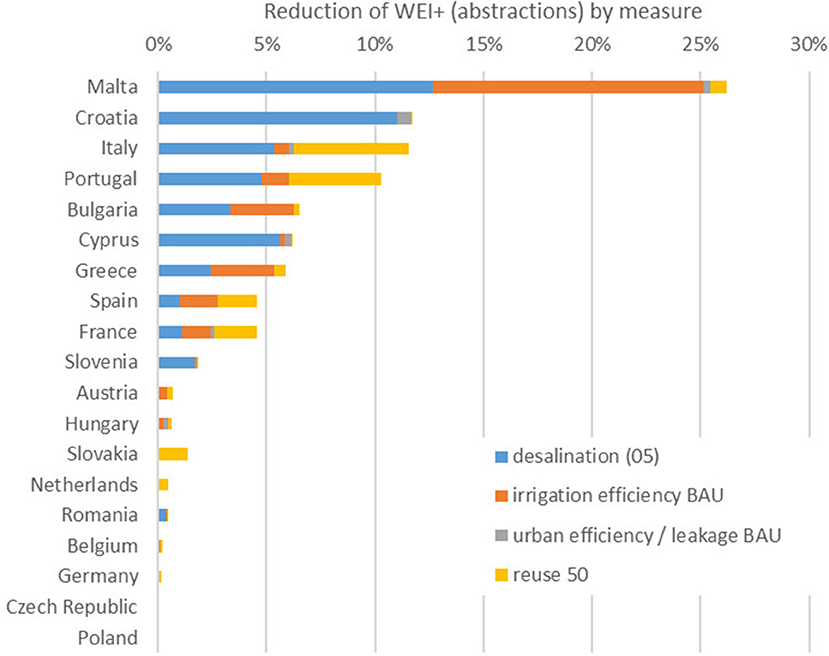
Figure 8. Effects of four water efficiency measures on WEI-abstraction, as estimated with the LISFLOOD model.
What can be deducted from the results in Figure 8 is that irrigation efficiency improvements and desalination—in sea bordering countries—are the most effective measures. Water reuse is helpful in some countries to reduce the WEIabstraction, in particular Italy, Portugal, Spain and France. Increasing urban water efficiency—reducing leakage—is on country scale little effective, but might help at local level.
Evaluating the effects of the four measures on net consumption, as visualized with the WEI+ indicator (Figure 9), we see that water re-use is not reducing net consumption. Water re-use is reducing new abstractions needed for irrigation, by double using the urban water later for irrigated agriculture. Leakage reduction also does not help to reduce net consumption, as the leakage is entering the subsurface hydrological system and eventually will be part of the soil and groundwater resources.
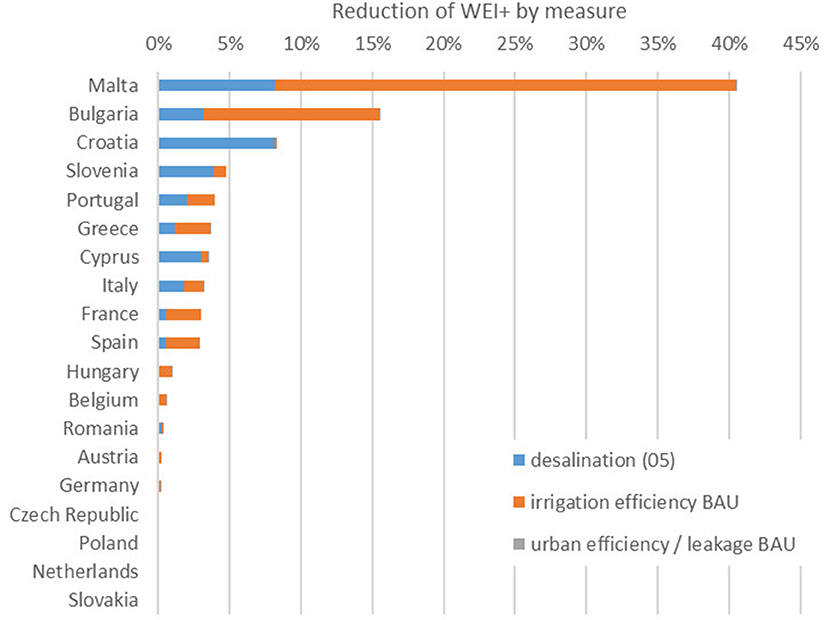
Figure 9. Effects of three water efficiency measures on WEI+ (consumption) as estimated with the LISFLOOD model. Note: water reuse does not influence WEI+ as it is used by irrigation.
Increasing irrigation efficiency and desalination are effective measures to reduce the WEI+ in various countries. Particularly in Malta, Bulgaria, Croatia and Slovakia the WEI+ could be reduced by 10–40% applying these measures. In many other countries however, the effect of the measures on net water consumption and thus reduction of the WEI+ is in the order of 4% (Greece) to 6% (Italy, Portugal). It should be noted that these are country averages, and local effects may be larger.
Challenges for the Mediterranean
The Mediterranean is already under current climate and water use conditions a water scarce region with high values of WEI+ and regional groundwater depletion issues, as demonstrated above. With climate change projections of a 2°C climate indicating that the water availability in the Mediterranean could decrease by 10–30% locally, we estimate consequently that the WEI+ indicator could increase by that same amount (Figure 4).
Given the results on potential measures presented in this paper, we observe that investments in irrigation efficiency in this decade will likely reduce the WEI+ only by 3–4% in countries such as Italy, Spain, and Greece. Desalination is as yet not much implemented. Water re-use could reduce new abstractions—and thus the WEIabstraction–but will not reduce consumption—the WEI+.
Depending on how fast we will reach the 2° global temperature increase, there might still be time to implement one or more additional cycles of investments of increasing irrigation efficiency and other effective water efficiency measures. In case the 2° warming is reached already earlier, the current level of investments on water efficiency might need to be increased to keep up with the decreasing water availability caused by global warming. Alternatively, farmers might need to be stimulated to change to different crops or crop varieties that consume less water, and require less irrigation.
In ongoing work we are assessing these additional investments in measures combined with the climate change signal, and we will report on that in subsequent papers and reports.
Citizen Engagement, Key to Solve the Riddle
Scientific studies such as these are helpful to convince policy makers at national and perhaps regional level, but different efforts are needed to convince and engage the layperson. If knowledge is power, as scientists we must share this power and allow the interested layperson to access it. Indeed, the aforementioned interconnections are at the heart of the climate change debate and the Mediterranean is one of the regions which lives the direct consequence is the most dramatic way.
Just to illustrate, the WEFE nexus affects other socioeconomic factors such as health, mass migration, conflict and gender inequality to name but a few. Science and technology are part of the solution, but require a broad understanding, and awareness in the society affected. Awareness leads to concern, concern leads to engagement, engagement leads to consensus and consensus leads to continuity of potential solutions. But awareness must be based on knowledge, engagement must be the route to solutions and consensus must be founded on an understanding of society's scientific and technological capacity to support what has often been considered as simply a socio-political debate (Elelman and Feldman, 2018).
Technology centers and research institutions in Europe are beginning to recognize that through its scientific and technical investigations they have a social responsibility to work in close collaboration and cooperation with society as a whole, to join forces and unite the so-called Quintuple Helix: Research and academia, the public and private sectors, and even arts and ultimately the citizen (Carayannis and Campbell, 2011; Gawlik et al., 2018).
The Gateway to the Future of the Mediterranean (Gawlik, 2021) is a unique experience that aims at explaining the complex nexus between water, energy, food and ecosystems in the Mediterranean region using a language accessible to the interested layperson and engaging with the public using novel techniques of communication made accessible through printed media. It offers both a scientific but also cultural perspective regarding the issues addressed while demonstrating that the most important challenge that the region faces, i.e., the lack of water, can become a source of dialogue and peace rather than conflict and confrontation.
The Gateway provides hard sciences: a spatial baseline analysis and projection of water-energy-food requirements of agricultural and urban areas in the Mediterranean region, but also technological solutions and non to face the challenge. The approach, addresses inter-sectorial flows of energy and water as sources of stability and socio-economic development in the Mediterranean area, and relates it to its historic past. Case studies and solutions are then evaluated through an SDG framework (Malagó et al., 2021). A series of interactive tools given access to further, animated information, illustration, arts and social media opens then a two way channel of engagement with the reader.
Conclusions
The Mediterranean is already under current climate and water use conditions a water scarce region with high ratios of water abstraction and consumption as compared to water availability, expressed here with the Water Exploitation Index (WEI and WEI+). Regional groundwater depletion is already an issue in the Mediterranean, and becoming an issue elsewhere in Europe as well.
With climate change projections of a 2°C climate indicating that the water availability in the Mediterranean could decrease by 10–30% locally, we estimate consequently that the WEI+ indicator could increase by that same amount.
In this paper, we assess that investments in irrigation efficiency in this decade will likely reduce the WEI+ only by 3–4% in countries such as Italy, Spain, and Greece. Desalination and water re-use are as yet not much implemented. Planned investments in urban water efficiency might reduce local water scarcity issues, but hardly reduce the WEI+ at larger scale. Water re-use could reduce new abstractions—and thus the WEIabstraction–but will not reduce consumption—the WEI+.
Depending on how fast we will reach the 2°C climate change situation globally, there might still be time to implement one or more additional cycles of investments of increasing irrigation efficiency and other effective water efficiency measures. In case the 2°C warming is reached already earlier, the current level of investments on water efficiency might need to be increased to keep up with the decreasing water availability in the Mediterranean, as caused by global warming. Alternatively, farmers might need to be stimulated to change to different crops or crop varieties that consume less water, and require less irrigation.
Water reuse can be an important measure to reduce abstractions, hence protect ecosystems. The costs of treatment for reuse—as per the new EU standards—may however exceed the current willingness to pay for water in agriculture. However, the current price of water very often does not reflect its actual costs (Pistocchi et al., 2017).
Desalination could be an increasingly central option. It is essential that it is put on track to be carbon-neutral—which is feasible—and coupled with re-use for irrigation in order to maximize its benefits (Pistocchi et al., 2020).
A stronger citizen engagement, as attempted with the Gateway to the Future of the Mediterranean, is required to bridge the gap between science and technology and public awareness of the water nexus issues.
Data Availability Statement
The raw data supporting the conclusions of this article will be made available by the authors, without undue reservation.
Author Contributions
AD: main writer of the manuscript. IT: executed the scenarios analysis and assessed the indicators. BB: executed the scenario modeling and wrote text on climate change. EG: maintains the LISFLOOD code and contributed on irrigation and groundwater depletion. AP: contributed on desalination and water reuse. BG: contributed on the citizen engagement. All authors contributed to the article and approved the submitted version.
Conflict of Interest
The authors declare that the research was conducted in the absence of any commercial or financial relationships that could be construed as a potential conflict of interest.
Publisher's Note
All claims expressed in this article are solely those of the authors and do not necessarily represent those of their affiliated organizations, or those of the publisher, the editors and the reviewers. Any product that may be evaluated in this article, or claim that may be made by its manufacturer, is not guaranteed or endorsed by the publisher.
References
Benitez Sanz, C., Wolters, H., Mart,í B., and Mora, B. (2018). “EU Water and Marine Measures Data base.” Deliverable to Task B2 of the BLUE2 project “Study on EU integrated policy assessment for the freshwater and marine environment, on the economic benefits of EU water policy and on the costs of its non-implementation.” Report to DG ENV—BLUE2 study; INTECSA-INARSA S.A., Madrid, Spain.
Bisselink, B., Bernhard, J., Gelati, E., Adamovic, M., Guenther, S., Mentaschi, L., et al. (2020). Climate change and Europe's water resources, EUR 29951 EN. Luxembourg: Publications Office of the European Union.
Burek, P., Ad de, R, and Johan van der, K. (2013). LISFLOOD—Distributed Water Balance and Flood Simulation Model—Revised User Manual. EUR 26162 10/2013; Publications Office of the European Union. Directorate-General Joint Research Centre, Institute for Environment and Sustainability. ISBN: 978-92-79-33190-9
Carayannis, E. G., and Campbell, D. F. J. (2011). Open innovation diplomacy and a 21st Century fractal research, education and innovation (FREIE) ecosystem: building on the quadruple and Quintuple Helix innovation concepts and the “Mode 3” knowledge production system. J. Knowl. Econ. 2, 327–372. doi: 10.1007/s13132-011-0058-3
Daher, B.T., and Mohtar, R.H. (2015). Water–Energy–Food (WEF) Nexus Tool 2.0: guiding integrative resource planning and decision-making. Water Int. 40, 748–771. doi: 10.1080/02508060.2015.1074148
De Roo, A., Bisselink, B., Guenther, S., Gelati, E., and Adamovic, M. (2020). Assessing the effects of water saving measures on Europe's water resources; BLUE2 project—Freshwater quantity, EUR 30361 EN. Luxembourg: Publications Office of the European Union.
De Roo, A. P. J., Wesseling, C. G., and Van Deursen, W. P. A. (2000). Physically-based river basin modelling within a GIS: The LISFLOOD model. Hydrol. Processes. 14, 1981–1992. doi: 10.1002/1099-1085(20000815/30)14:11/12andlt;1981::AID-HYP49andgt;3.0.CO;2-F
Dermody, B. J., van Beek, E., Meeks, K., Klein Goldewijk, W., Scheidel, Y., van der Velde, M., et al. (2014). A virtual water network of the Roman world. Hydrol. Earth Syst. Sci. 18:5025 doi: 10.5194/hess-18-5025-2014
Dosio, A. (2020). Mean and extreme climate in Europe under 1.5, 2, and 3°C global warming, EUR 30194 EN. Luxembourg: Publications Office of the European Union.
Ducrocq, V., Philippe, D., Silvio, G., and Patrick, R. (2016). “The water cycle in the Mediterranean,” in The Mediterranean region under climate change. Jean-Paul Moatti and Stéphane Thiébault (eds). IRD Editions. Available online at: https://books.openedition.org/irdeditions/23073
EEA (2015). https://www.eea.europa.eu/data-and-maps/explore-interactive-maps/water-exploitation-index-for-river-2
Elelman, R., and Feldman, D. (2018). The future of citizen engagement in cities—The council of citizen engagement in sustainable urban strategies (ConCensus). Futures 101, 80–91, doi: 10.1016/j.futures.2018.06.012
Eurostat (2021). Irrigation: number of farms, areas and equipment by size of irrigated area and NUTS 2 regions. Available online at: https://ec.europa.eu/eurostat/web/products-datasets/-/ef_poirrig, accessed 2021-09-21
Gawlik, B. (2021), The Gateway to the Future of the Mediterranean. Luxembourg: European Commission, Joint Research Centre.
Gawlik, B. M., Głowacka, N., Feldman, D., and Elelman, R. (2018). The scientist, the politician, the artist and the citizen: how water united them. Environ. Sci. Europe 30:12. doi: 10.1186/s12302-018-0141-5
Gelati, E., Zuzanna, Z., Andrej, C., Simona, B., Bernard, B., Marko, A., et al. (2020). Assessing groundwater irrigation sustainability in the Euro-Mediterranean region with an integrated agro-hydrologic model. Adv. Sci. Res. 17, 227–253. doi: 10.5194/asr-17-227-2020
Grenon, M., and Batisse, M. (1989). Futures for the Mediterranean Basin—The Blue Plan. Oxford: Oxford University Press, 279.
Lawford, R., Bogardi, J., Marx, S., Jain, S., Wostl, C. P., Knüppe, K., et al. (2013). Basin perspectives on the water–energy–food security nexus. Curr. Opin. Environ. Sustain. 5, 607–616. doi: 10.1016/j.cosust.2013.11.005
Malagó, A., Comero, S., Bouraoui, F., Kazezyilmaz-Alhan, C. M., Gawlik, B. M., Easton, P., et al. (2021). An analytical framework to assess SDG targets within the context of WEFE nexus in the mediterranean region, resources, conservation. Recycling 164:105205. doi: 10.1016/j.resconrec.2020.105205
Pistocchi, A., Aloe, A., Dorati, C., Alcalde Sanz, L., Bouraoui, F., Gawlik, B., et al. (2017). The potential of water reuse for agricultural irrigation in the EU: a hydro-economic analysis. EUR 28980 EN. Luxembourg (Luxembourg). Publications Office Of The European Union.
Pistocchi, A., Bleninger, T., Breyer, C., Caldera, U., Dorati, C., Ganora, D., et al. (2020). Can seawater desalination be a win-win fix to our water cycle? Water Res. 182:20. doi: 10.1016/j.watres.2020.115906
Pistocchi, A., Dorati, C., Huld, T., and Salas Herrero, M. (2018). Hydro-economic assessment of the potential of PV-RO desalinated seawater supply in the Mediterranean region: Modelling concept and analysis of water transport costs, EUR 28982 EN. Luxembourg: Publications Office of the European Union.
Shi, H., Luo, G., Zheng, H., Chen, C., Bai, J., Liu, T., et al. (2020). Coupling the water-energy-food-ecology nexus into a bayesian network for water resources analysis and management in the Syr Darya river basin. J. Hydrol. 581:124387, doi: 10.1016/j.jhydrol.2019.124387
Keywords: water quantity, Mediterranean, hydrology, water resources management, WEFE Nexus, modeling, climate change impact, water efficiency
Citation: De Roo A, Trichakis I, Bisselink B, Gelati E, Pistocchi A and Gawlik B (2021) The Water-Energy-Food-Ecosystem Nexus in the Mediterranean: Current Issues and Future Challenges. Front. Clim. 3:782553. doi: 10.3389/fclim.2021.782553
Received: 24 September 2021; Accepted: 25 November 2021;
Published: 22 December 2021.
Edited by:
Marco Gaetani, University Institute of Higher Studies in Pavia, ItalyReviewed by:
Rabi Mohtar, American University of Beirut, LebanonMaría Cleofé Valverde, Federal University of ABC, Brazil
Copyright © 2021 De Roo, Trichakis, Bisselink, Gelati, Pistocchi and Gawlik. This is an open-access article distributed under the terms of the Creative Commons Attribution License (CC BY). The use, distribution or reproduction in other forums is permitted, provided the original author(s) and the copyright owner(s) are credited and that the original publication in this journal is cited, in accordance with accepted academic practice. No use, distribution or reproduction is permitted which does not comply with these terms.
*Correspondence: Ad De Roo, YWQuZGUtcm9vJiN4MDAwNDA7ZWMuZXVyb3BhLmV1