- 1National Institute for Space Research, São José dos Campos, Brazil
- 2Department of Meteorology and Oceanography, Andhra University, Visakhapatnam, India
- 3Centre for Earth, Ocean and Atmospheric Sciences, University of Hyderabad, Hyderabad, India
India, one of the most disaster-prone countries in the world, has suffered severe economic losses as well as life losses as per the World Focus report.1 More than 80% of its land and more than 50 million of its people are affected by weather disasters. Disaster mitigation necessitates reliable future predictions, which need focused climate change research. From the climate change perspective, the summer monsoon, the main lifeline of India, is predicted to change very adversely. The duration of the rainy season is going to shrink, and pre-monsoon drying can also occur. These future changes can impact the increase of vector-borne diseases, such as malaria, dengue, and others. In another recent study, 29 world experts from various institutions found that the largest exposure to disasters, such as tropical cyclones (TCs), river floods, droughts, and heat waves, is over India. For improved and skillful prediction, we suggest a three-stage cumulative method, namely, K is for observational analysis, U is for knowledge and understanding, and M is for modeling and prediction. In this brief note, we report our perspective of imminent weather disasters to India, namely, monsoons and TCs, and how the weather and climate forecasting can be improved, leading to better climate change adaptation.
Introduction
The Indian economy still significantly depends on agriculture, which, in turn, depends on the summer monsoon rains occurring from June to September. In the present scenario of climate change, it is essential to know how the Indian summer monsoon rainfall is going to change in the future. In a recent detailed study with regional climate model projections, Ashfaq et al. (2020) suggest that an important adverse signal of future climate change over the Indian monsoon region in the RCP8.5 scenario (Krishnan et al., 2020; Jyoteeshkumar Reddy et al., 2021) can occur. The sinking of the Indian monsoon rainy season onset is projected to delay by five to eight pentads and a shrinking of the monsoon rainy season. India can experience pre-monsoon drying as well.
In a recent innovative study, 29 world experts (Lange et al., 2020) from different institutions and different countries, reached some important conclusions. These inferences deserve urgent attention and action plans by policymakers. They considered six categories of extreme climate impact events, namely, river floods, cyclones, crop failures, wildfires, heat waves, and droughts. These authors (Lange et al., 2020) quantified the pure effect of climate change on the exposure of the global population to the events mentioned. One important conclusion, which is of grave concern to India, is that the largest increase in exposure is projected here. Thus, to avoid huge damages due to these disasters, such as deaths and loss of property, urgent and more reliable predictions are needed. We, however, must clarify that there has been tremendous improvement in numerical prediction of tropical cyclones (TCs) in the last few decades in India [e.g., Pattanaik and Mohapatra, 2021; Saranya Ganesh et al., 2021; Sarkar et al., 2021, and all other papers in January 2021 of Mausam, a special issue on the state of the art on TC prediction in the North Indian Ocean (NIO)], but what we claim is that applying theory can enhance the skills from the current day model outputs substantially more as discussed in the following section. To provide an analogy, in a recent study, Rao et al. (2021) attempted to connect observations, theory, and a prediction plan for heat waves. This prediction method can be applied to a numerical weather prediction model to predict deadly heat waves; thus, Rao et al. (2021) used a K, U, and M approach for the prediction of deadly heat waves over India.
From the context of the three-pronged K, U, and M method (hereafter, KUM), there are sufficient observational studies, or K, and also some attempts have been made using highly sophisticated, state-of-the-art (atmosphere and ocean) coupled models for predictions, M. What is most lacking, however, are theoretical studies (U) aiming to find out the causes for disastrous TCs or the highly complex regional monsoons.
According to a recent 2021 overview of current research results by the Geophysical Fluid Dynamics Laboratory of Global Warming and Hurricanes2, the severity and frequency of TCs are increasing globally. A recent study (Balaguru et al., 2015) also suggests an increase of TCs globally even over the NIO. Essentially, the increase in the strong TCs has far-reaching implications for society because these include the most harmful aspects, namely, storm surges and heavy rains with intense wind speeds. Indeed, TC rainfall rates will possibly increase in the future due to various anthropogenic effects and accompanying increases in atmospheric moisture. Rapid intensification of TCs poses forecast challenges and increased risks for coastal communities (Emanuel, 2017). Recent modeling studies (Emanuel, 2020) show an increase of 10–15% for precipitation rates averaged within about 100 km of the cyclone for a 2°C global warming scenario. As per IPCC AR5, higher levels of coastal flooding due to TCs are expected to occur, all else assumed to be constant due to rising sea levels. In this situation, together with the rise in sea level, the impact due to the strong TCs deteriorates the conditions of the increasing coastal population across India and the neighborhood. As the NIO is one of the typical regions with a population of 1.353 billion (2018), about 18% of the global population by 2020, it is highly susceptible to strong TCs causing adverse living conditions, and the implication is that stronger TCs will be worse.
According to reports from a respected BBC newspaper3,4, and a potential report5 from the Indian Meteorological Department, Amphan is a very severe cyclone that transited the west coast of India in 2020 and also caused a lot of damage. The super cyclonic storm Amphan is the costliest case in the recorded history of TCs with damage of US$15.78 billion and also total fatalities of 269. Similarly, in the year 2019, a loss of US$11 billion occurred due to TCs. In the year 2020, there was a record-breaking occurrence of eight TCs over the NIO: five cyclones and three major cyclones compared to the climatology of 4.9, 1.5, and 0.7. We note a drastic increase in category 3 and beyond hurricanes occurring in the NIO and also a significant increase in the Northern and Southern hemispheres (Figure 1). Also, there is a substantial increase in accumulated cyclone energy (ACE) in the last two decades in the NIO and Northern and Southern hemispheres (Figure 2). In 2019, record-breaking ACE of 85 × 104 knots2, occurred in the NIO, nearly twice the previous record (Singh et al., 2021; Wang et al., 2021, BAMS). The decrease in the projected number of TCs found in some studies (Sugi et al., 2017) is overcompensated by the huge increase in intensity similar to that found over the NIO in 2019 and 2020. Furthermore, as if to worsen the situation in a colloquial sense, Wang and Murakami (2020) show that the general atmospheric and ocean parameters, which show a high global correlation with the number of TCs, nevertheless show only a very low correlation with TCs of the NIO. Thus, urgent research should be carried out to understand the causes of the occurrence of TCs over the NIO. Even globally, in the last 39 years (1980–2018), weather disasters caused about 23,000 fatalities and US$100 billion in damages worldwide. Each year, weather events displace huge populations, drive people into poverty, and dampen economic growth globally (Kousky, 2014; Munich, 2020; Hoegh-Guldberg et al., in press). The underlying causes show a marked signal of anthropogenic roots and global warming (e.g., Sobel et al., 2016; Im et al., 2017).
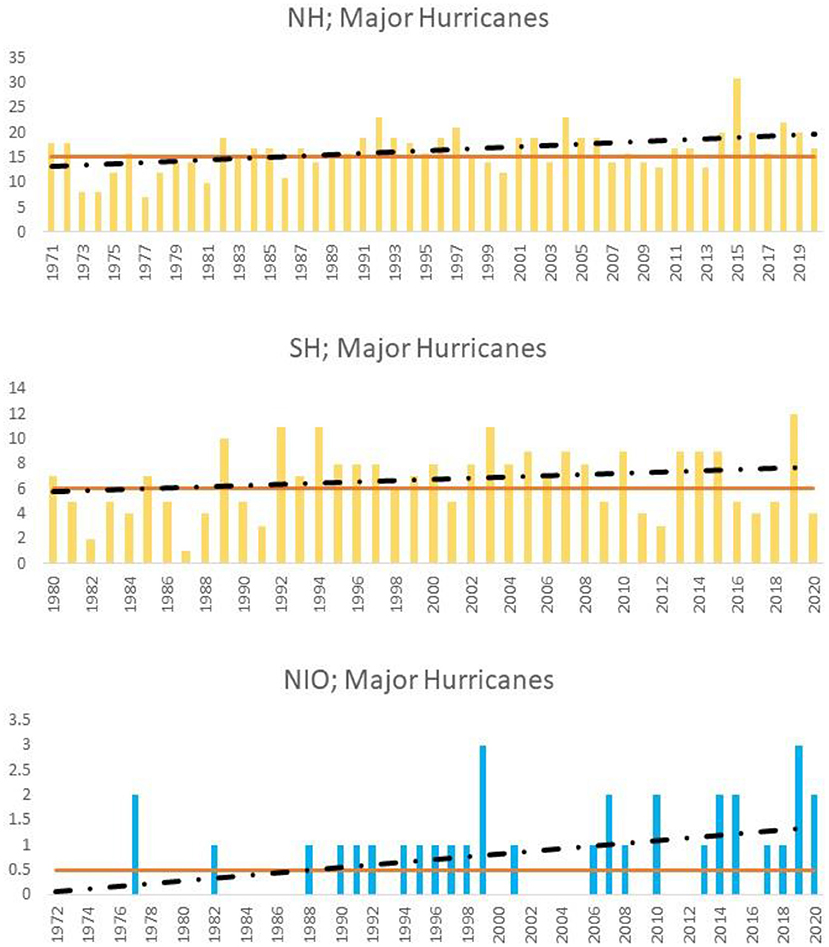
Figure 1. The number of category 3+ hurricanes that occurred in the Northern and Southern hemispheres and the NIO (black dotted line indicates a linear trend, and orange line indicates significance at the 95% confidence level) (http://tropical.atmos.colostate.edu/Realtime/index.php?archandloc=northindian).
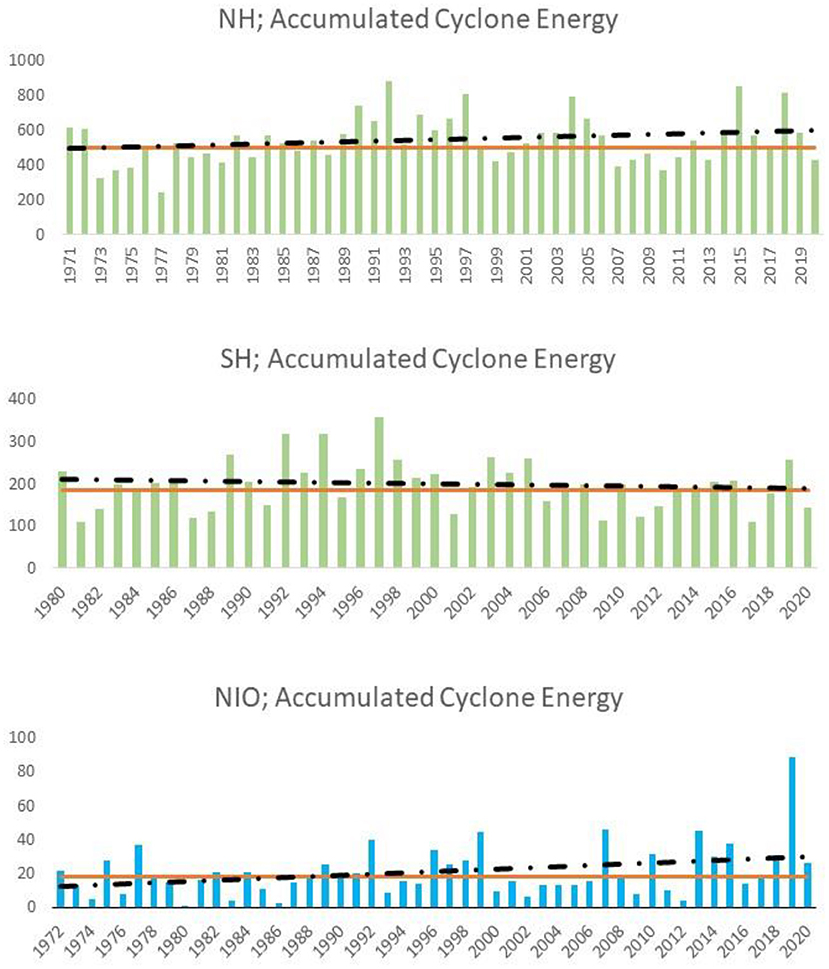
Figure 2. ACE (in 104 Knots2) in the Northern and Southern hemispheres and the NIO (black dotted line indicates a linear trend, and orange line indicates significance at 95% confidence level) (http://tropical.atmos.colostate.edu/Realtime/index.php?archandloc=northindian).
Henceforth, we focus on the TCs as well as summer monsoons, which are the two most relevant weather and climate phenomena for the Indian region.
A Three-Stage Method to Study and Plan Reliable Prediction
Because India is rigorously prone to natural disasters as well as impacts due to anticipated changes in the summer monsoon in the future, there is indeed a serious question as to how to study the causal mechanisms of these disasters and plan to mitigate them. In this context, the late Gill (1985), an accomplished geosciences expert, suggested almost 35 years ago the KUM method, namely, knowledge, understanding, and modeling, a three-pronged approach. The first step (K) is to improve observational knowledge of calamity-causing weather events and next a theoretical understanding to find out the cause of a specific effect, probably utilizing linear analytical mathematical solutions (U). Finally, the third one (M), using the presently available highly complex coupled (atmosphere and ocean) models giving numerical solutions to non-linear equations, pioneered by Phillips (1956), predicting future occurrences. The order of KUM seems to be important. Although relatively substantial observational results are available in the Indian context for meteorological and oceanographic events, very few theoretical studies have been made delineating the causal mechanisms. Thus, this aspect should be given priority. In a recent comment, Emanuel (2020) also stressed the need for theoretical studies. Finally, only after acquiring the observational, knowledge, and cause-and-effect relationships in theoretical studies, only then, should one embark on numerical or climate modeling to successfully predict the future.
In this context, it is illuminating to recall the comments of Phillips (1970), one of the founding fathers of theoretical meteorology and numerical weather prediction: “in making a numerical forecast, one takes a set of numbers.regardless of.synoptic structures.by another set of numbers, representing the forecast. The computation of a set of numbers depicting the formation of a front, is of course, not a theory of fronts (unless one is content to point to the equation of motion as theory!!!!!)” Thus, one should be very careful using numerical models to develop a theory of TCs, and in the Indian context, monsoon depressions (MDs) are crucial for monsoon rainfall. Today, many students and scientists worldwide spend most of their valuable time dealing with huge data sets and running numerical models to simulate rather than to develop a theory. Tellingly, Emanuel (2020), mentions that presently there is “computing too much and thinking too little.” Indeed, there is an urgent need for curiosity-driven theoretical research even in the Indian context. One interesting example to stress the importance of theory is, today, that the best numerical weather prediction is in mid and high latitudes in winter. This is because the basic theory behind the mechanism of winter weather changes, the baroclinic instability, was discovered more than 70 years ago by Charney (1947), and models and observations evolved accordingly. Thus, it is important to realize, without the correct understanding of the causal mechanisms through theory, one will never be able to predict correctly and completely the required weather or climate or its changes with just the brute force of computers available today!!!
TCs Over the NIO
Regarding the theory of the generation mechanisms of TCs, there are two well-known hypotheses, namely, (a) the conditional instability of the second kind (CISK) and (b) wind-induced surface heat exchange (WISHE) (please refer to Tomassini, 2020 for a comprehensive discussion of these two processes). A detailed discussion of these two is beyond the scope of the present short article. However, the authors quickly discuss these two mechanisms in the context of TCs over NIO.
In the case of TCs, the pre-synoptic disturbances get their energy by the complex interaction of two different horizontal scales, namely, cumulus convection of about 1 km and synoptic systems of about 500 km. How this interaction happens is a topic of debate, though, and most of the research in the published literature is about TCs in tropical ocean basins other than the NIO region.
Briefly, we discuss the basic characteristics of CISK and quasi-equilibrium (or WISHE). In the process of CISK, the buoyant convection can occur only when low-level stability is weakened (see Figure 2; Ooyama, 1969), and in the other, moist convection is governed by the vertically integrated measure of instability. As noted by Tomassini (2020), meteorological conditions vary greatly from one region to the other in the tropics and also in the same region from one season to another (see Ashok et al., 2000; Rao et al., 2000; Raymond et al., 2015). Raymond mentions two tropical places, Sahel and the Western Pacific, where conditions are very different. Now, how do the conditions vary, during (i) pre-monsoon, (ii) MDs, and (iii) post-monsoon TCs? Similar to Bony et al. (2017), we suggest that more detailed observations of both satellite measurements and data developed in field programs should be used to understand the convection and circulation coupling of TCs over NIO. For example, the INCOMPASS IOP field program, which collects data from strategically installed ground-based instruments in India, is one such program (Fletcher et al., 2018).
Another, synoptic disturbance of importance is a MD. Despite several observational and theoretical studies by many authors (for example, Sikka, 1977; Mishra and Salvekar, 1979; Aravequia et al., 1995; Boos et al., 2017) trying to understand the basic mechanism of origin, some fundamental questions remain unanswered. Similar to TCs, the lack of understanding of how convection and MD circulation couple hinders the prediction. For both TCs and MDs, we suggest analyzing time vertical sections of potential temperature, equivalent potential temperature, and saturated equivalent potential temperature such that one can get an idea of the relative importance of CISK or the quasi-equilibrium hypothesis discussed briefly above.
Another method for elucidating the study is to examine the system's energetics, i.e., TCs or MDs. Lorenz (1960) mentions, “one enlightening method of studying the behaviour of the atmosphere, or a portion of it, consists of examining the behaviour of the energy involved.” Earlier Mishra and Rao (2001) used limited area energetics to infer the mechanism of generation of Northeast Brazil's upper tropospheric vortices. Also, Rao and Rajamani (1972) examined the energetics of MDs. These methods of energy analysis, for example, can be used to isolate or single out the basic mechanism of generation of TCs or MDs, using more recent well-covered data, such as the INCOMPASS IOP program (Turner et al., 2019). Later, targeted numerical model studies should be used to not only verify the process/processes identified in energetic and diagnostic studies, but to design dynamics-based indices related to TC formation that are relatively easier to predict. For example, a CISK parameter may be easier to predict with a longer lead as compared with the TC rainfall. These methods are again akin to the KUM approach. Such carefully verified and designed indices, when operationalized, will substantially help in extending the lead prediction time. Probabilistic dynamical-statistical downscaling tools can also be developed to relate local rainfall with these indices. This will also potentially enhance the lead time of the TC-related deluge. Similarly, a better understanding of model ability in capturing the conversions between different forms of energy.
Monsoons
Again, several aspects of monsoons, particularly, the Indian Monsoon are still not completely clear and hinder the mechanisms of prediction. In a recent exhaustive study, Geen et al. (2020), discussed several aspects, primarily from a theoretical standpoint even though this study was developed based on the concept of a global monsoon, Figure 2 of Geen et al. (2020) shows only a very low correlation in interannual variations of rainfall, the main meteorological element that must be predicted. However, the different regions of monsoons with different geographical boundaries raise serious objections about the global monsoon concept.
Several studies exist in the literature regarding the observed aspects of the Indian summer monsoon (the K part of the three-pronged method), and modern numerical models are employed to improve prediction skills (Sahai et al., 2016; Rao et al., 2019; Mohanty et al., 2020). From an almost zero skill, we have reached a stage at which the skills for predicting the area-averaged Indian summer monsoon are found to be statistically significant. This is great progress. Having said that, there is a great scope for further improvement. Although the broad regionally averaged skills are statistically significant, they are modest. Further, improving the skills such that they are locally useful is the obvious goal but still a long way ahead. Although the prediction skill improved through better methods of, for example, data assimilation and parametrization schemes, to improve the predictions further, we need to diagnose the improved representation (e.g., Halder et al., 2016; Saha et al., 2019; Hazra et al., 2020), better replication of physical processes and scale interactions.
Notwithstanding all these technical improvements, the large-scale physical causal mechanisms are not clear yet. This can only be done with the studies aiming to understand the cause-and-effect relation or the U in the three-pronged method. As mentioned earlier, with more observational studies aiming to identify the correct interaction mechanism over NIO between convection and large-scale monsoon circulation (either CISK or WHISE), then this mechanism can be included in the numerical models. Also, controlled experiments using simple models, such as the one by Rao et al. (2000), can be used to identify relative roles of mountains and thermal contrast in generating the Indian summer monsoon. In the state-of-the-art coupled models, because of extremely complex non-linear interactions among various physical mechanisms, it is almost impossible to isolate the cause of a specific effect.
Again, the diagnostic study based on energetics, such as the generation of available potential energy (PE) by latent heat and the baroclinic conversions, for example, may reveal relative roles of some physical processes, such as convection in the Indian monsoon. In a recent companion study (Rao et al., under review), comparing the South American and Indian monsoons, we found that, in the Indian monsoon, the baroclinic conversions (mean available PE) to P′ (eddy PE) to kinetic energy (KE) is non-existent, and the KE of monsoon is mainly furnished by the generation of perturbation PE by latent heating (rainfall) and subsequent conversion to KE. In contrast, over the South American monsoon, both the baroclinic conversions and generation terms are equally important. This is probably because the Himalayas extend from East to West across the cardinal northern border of the country, which does not allow mid-latitude baroclinic waves to penetrate at lower levels while the Andes mountains in South America extend along North to South, permit these waves to penetrate even as low latitude as Manaus, where even austral summer cold waves (FRAIAGENS) are noted. Furthermore, studies are necessary to verify how energetics vary between wet and dry monsoons in these two regions.
In a review article by Geen et al. (2020), the authors discuss attempts to understand fundamental dynamics (U in our three-pronged method). Geen et al. (2020) mention a very similar KUM approach for monsoons (their section 3). Such efforts are urgently needed from the context of the Indian monsoon. They even discuss the south Asian monsoon (their section 3.1.2). Although they tried to reconcile between global and regional monsoon features, the differences are more striking as we mentioned earlier, regarding the Indian and South American monsoons. In the case of the East Asian monsoon, at least one author (Molnar et al., 2010) mentions, “‘monsoon' is somewhat of a misnomer.”
Although there are some uncertainties in the methods used by Lange et al. (2020), the importance of their conclusion is unambiguous. They mention that “anthropogenic” climate change has already substantially increased the exposure to extreme global climatic impacts, and anthropogenic warming is projected to exacerbate the pattern of climate change that we are already noticing nowadays. Thus, it is urgent to restrain the increase in global average temperature well below 2°C, which would significantly reduce the risks and impacts of climate change6 (Benitez, 2009; Dash et al., 2013). All this, therefore, underscores the urgency for climate action expressed in the Paris agreement of 2015. Even in a climate change context, using the KUM approach will help in a better diagnosis of the changes in regional implications for large-scale instabilities to diabatic processes. These can help in design model-based indices that can inform the stakeholders working on climate change mitigation and adaptation.
Recommendations
We are in an era in which observational data availability in the tropics has improved significantly and is going to be further improved. In this context, it is recommended that the forecasters and researchers of Indian weather and climate use this excellent opportunity to build theoretical knowledge unique to the regional weather and climate. The knowledge gained should be translated to identify tangible, large-scale dynamical process indices. Such indices will be very useful to extend the lead prediction skills of important weather and climate phenomenon, such as TCs, MDs, etc. Similarly, (i) evaluating the model capacity in predicting and calibration of association between hindcast perturbation PE, latent heating, and subsequent conversion to KE, and (ii) comparing the observations will potentially provide us with indices that can be directly used to predict subseasonal monsoonal rainfall with longer leads. The above recommendations are just examples. In summary, identifying the key dynamics behind important weather and climate processes at discernible time scales and designing useful dynamical indices that can be used to extend the lead forecast envelope will be the way forward.
Data Availability Statement
The datasets presented in this study can be found in online repositories. The names of the repository/repositories and accession number(s) can be found below: http://tropical.atmos.colostate.edu.
Author Contributions
VB conceived the idea. VB wrote the manuscript with inputs from KA and using the results from DG analysis. KA comprehensively revised the article. All authors contributed to the article and approved the submitted version.
Funding
The publication charge of this article is fully funded by the Frontiers in Climate Journal.
Conflict of Interest
The authors declare that the research was conducted in the absence of any commercial or financial relationships that could be construed as a potential conflict of interest.
Publisher's Note
All claims expressed in this article are solely those of the authors and do not necessarily represent those of their affiliated organizations, or those of the publisher, the editors and the reviewers. Any product that may be evaluated in this article, or claim that may be made by its manufacturer, is not guaranteed or endorsed by the publisher.
Acknowledgments
The authors thank Prof. Matthew Collins, Specialty Chief Editor, Frontiers in Climate Journal, and reviewers for their helpful feedback and recommendations in improving the manuscript quality. The authors are grateful to the Frontiers in Climate Journal Committee for waiving the article's publishing fees. We thank the reviewers for their critical comments, which helped to improve the quality of the article.
Footnotes
1. ^World focus-special issue July 2014, editorial (peer-reviewed, refereed research journal).
2. ^https://www.gfdl.noaa.gov/global-warming-and-hurricanes/
3. ^https://www.bbc.com/news/world-asia-india-52749935
4. ^https://en.wikipedia.org/wiki/2020_North_Indian_Ocean_cyclone_season
5. ^https://mausam.imd.gov.in/Forecast/marquee_data/indian111.pdf
6. ^https://unfccc.int/process-and-meetings/the-paris-agreement/the-paris-agreement
References
Aravequia, J. A., Brahmananda Rao, V., and Bonatti, J. P. (1995). The role of moist baroclinic instability in the growth and structure of monsoon depressions. J. Atmos. Sci. 52, 4393–4409. doi: 10.1175/1520-0469(1995)052<4393:TROMBI>2.0.CO;2
Ashfaq, M., Cavazos, T., Reboita, M. S., Torres-Alavez, J. A., Im, E.-S., Olusegun, F. M., et al. (2020). Robust late twenty-first century shift in the regional monsoons in RegCM-CORDEX simulations. Clim. Dyn. 57, 1463–1488. doi: 10.1007/s00382-020-05306-2
Ashok, K., Soman, M., and Satyan, V. (2000). Simulation of monsoon disturbances in a GCM. Pure Appl. Geophys. 157, 1509–1539. doi: 10.1007/PL00001131
Balaguru, K., Foltz, G. R., Leung, L. R., D'Asaro, E, Emanuel, K. A., Liu, H., et al. (2015). Dynamic potential intensity: an improved representation of the ocean's impact on tropical cyclones. Geophys. Res. Lett. 42, 6739–6746. doi: 10.1002/2015GL064822
Benitez, M. A. (2009). Climate change could affect mosquito - borne diseases in Asia. Lancet. 373, 1070. doi: 10.1016/S0140-6736(09)60634-6
Bony, S., Stevens, B., Ament, F., Bigorre, S., Chazette, P., Crewell, S., et al. (2017). EUREC4A: a field campaign to elucidate the couplings between clouds convection and circulation. Surv. Geophys. 38, 1529–1568. doi: 10.1007/s10712-017-9428-0
Boos, W. R., Mapes, B. E., and Murthy, V. S. (2017). Potential vorticity structure and propagation mechanism of Indian monsoon depressions. Glob Monsoon Syst Res Forecast 2017, 187–199. doi: 10.1142/9789813200913_0015
Charney, J. G. (1947). The dynamics of long waves in a baroclinic westerly current. J. Meteor. 4, 135–162. doi: 10.1175/1520-0469(1947)004<0136:TDOLWI>2.0.CO
Dash, A. P., Bhatia, R., Suyoto, T., and Mourya, D. T. (2013). Emerging and re-emerging arbovorial diseases in South-east Asia. J. Vector. Borne Dis. 50 77–84.
Emanuel, K. (2017). Assessing the present and future probability of Hurricane Harvey's rainfall. Proc. Natl. Acad. Sci. U.S.A. 114, 12681–12684. doi: 10.1073/pnas.1716222114
Emanuel, K. (2020). The relevance of theory for contemporary research in atmospheres, oceans, and climate. AGU Adv. 1 e2019AV000129. doi: 10.1029/2019AV000129
Fletcher, J. K., Parker, D. J., Turner, A. G., Menon, A., Martin, G. M., et al. (2018). The dynamic and thermodynamic structure of the monsoon over southern India: new observations from the INCOMPASS IOP. Q. J. R. Meteorol. Soc. 146, 2876–2890. doi: 10.1002/qj.3439
Geen, R., Bordoni, S., Battisti, D. S., and Hui, K. (2020). Monsoons, ITCZs, and the concept of the global monsoon. Rev. Geophys. 58, e2020RG000700. doi: 10.1029/2020RG000700
Gill, A. E. (1985). “An overview of the dynamics of the tropical oceans and global atmosphere,” in International Conference on TOGA. WCRP. NO 4 WMO/TD-5 (Paris).
Halder, S., Saha, S. K., Dirmeyer, P. A., Chase, T. N., and Goswami, B. N. (2016). Investigating the impact of land-use land-cover change on Indian summer monsoon daily rainfall and temperature during 1951–2005 using a regional climate model. Hydrol. Earth Syst. Sci. 20, 1765–1784. doi: 10.5194/hess-20-1765-2016
Hazra, A., Chaudhari, H. S., Saha, S. K., Pokhrel, S., Dutta, U., Goswami, B. N., et al. (2020). Role of cloud microphysics in improved simulation of the Asian monsoon quasi-biweekly mode (QBM). Clim. Dyn. (2020) 54, 599–614. doi: 10.1007/s00382-019-05015-5
Hoegh-Guldberg, O., Jacob, D., Taylor, M., Bindi, M., Brown, S., and Camilloni, I. (in press). “Impacts of 1.5°C Global Warming on Natural Human Systems,” in Global Warming of 1.5°C. An IPCC Special Report on the Impacts of Global Warming of 1.5°C Above Pre-industrial Levels Related Global Greenhouse Gas Emission Pathways in the Context of Strengthening the Global Response to the Threat of Climate Change, Sustainable Development, Efforts to Eradicate Poverty, eds V. Masson-Delmotte, P. Zhai, H.-O. Pörtner, D. Roberts, J. Skea, P. R. Shukla, A. Pirani, W. Moufouma-Okia, C. Péan, R. Pidcock, S. Connors, J. B. R. Matthews, Y. Chen, X. Zhou, M. I. Gomis, E. Lonnoy, T. Maycock, M. Tignor, T. Waterfield. Available online at: https://www.ipcc.ch/sr15/chapter/chapter-3/ .
Im, E. S., Pal, J. S., and Eltahir, E. A. B. (2017). Deadly heat waves projected in the densely populated agricultural regions of South Asia. Sci. Adv. 3, e1603322. doi: 10.1126/sciadv.1603322
Jyoteeshkumar Reddy, P., Sriram, D., Gunthe, S. S., and Balaji, C. (2021). Impact of climate change on intense Bay of Bengal tropical cyclones of the post-monsoon season: a pseudo global warming approach. Clim. Dyn. 56, 2855–2879. doi: 10.1007/s00382-020-05618-3
Kousky, C. (2014). Informing climate adaptation: a review of the economic costs of natural disasters. Energy Econ. 46, 576–592. doi: 10.1016/j.eneco.2013.09.029
Krishnan, R., Sanjay, J., Gnanaseelan, C., Mujumdar, M., Kulkarni, A., and Chakraborty, S. (2020). Assessment of Climate Change Over the Indian Region: A Report of the Ministry of Earth Sciences (MoES), Government of India. Singapore: Springer. doi: 10.1007/978-981-15-4327-2
Lange, S., Volkholz, J., Geiger, T., Zhao, F., Vega, I., and Veldkamp, T. (2020). Projecting exposure to extreme climate impact events across six event categories and three spatial scales. Earth Fut. 8, e2020EF001616. doi: 10.1029/2020EF001616
Lorenz, E. N. (1960). Energy and numerical weather prediction. Tellus 12, 364–373. doi: 10.1111/j.2153-3490.1960.tb01323.x
Mishra, S. K., and Rao, V. B. (2001). The energetics of an upper tropospheric cyclonic vortex over north-east Brazil. Q. J. R. Meterol. Soc. 127, 2329–2351. doi: 10.1002/qj.49712757707
Mishra, S. K., and Salvekar, P. S. (1979). Role of baroclinic instability in the development of the monsoon disturbances. J. Atmos. Sci. 37, 383–394. doi: 10.1175/1520-0469(1980)037<0383:ROBIIT>2.0.CO;2
Mohanty, U. C., Mohapatra, M., Ashok, K., and Raghavan, K. (2020). Indian monsoons variability and extreme weather events: recent improvements in observations and modelling. Proc. Ind. Natl. Sci. Acad. 86, 503–524. doi: 10.16943/ptinsa/2020/49817
Molnar, P., Boos, W. R., and Battisti, D. S. (2010). Orographic controls on climate and paleoclimate of Asia. Thermal and mechanical roles of the Tibetan Plateau. Annu. Rev. Earth Planet. Sci. 38, 77–102. doi: 10.1146/annurev-earth-040809-152456
Munich, R. E. (2020). NatCatSERVICE Analysis Tool. Available online at: https://natcatservice.munichre.com/
Ooyama, K. (1969). Numerical simulation of the life cycle of tropical cyclones. J. Atmos. Sci. 26, 3–40. doi: 10.1175/1520-0469(1969)026<0003:NSOTLC>2.0.CO
Pattanaik, D. R., and Mohapatra, M. (2021). Evolution of IMD's operational extended range forecast system of tropical cyclogenesis over North Indian Ocean during 2010–2020. Mausam 72, 35–56. doi: 10.54302/mausam.v72i1.124
Phillips, A. (1956). The general circulatin of the atmosphere: a numerical experiment. Q. J. R. Meterol. Soc. 82, 123–164. doi: 10.1002/qj.49708235202
Rao, K. V., and Rajamani, S. (1972). Study of heat sources and sinks and the generation of available potential energy in the Indian region during the southwest monsoon season. Mon. Weath. Rev. 100, 383–388. doi: 10.1175/1520-0493(1972)100<0383:SOHSAS>2.3.CO
Rao, S. A., Goswami, B. N., Sahai, A. K., Rajagopal, E. N., Mukhopadhyay, P., Rajeevan, M., et al. (2019). Monsoon mission: a targeted activity to improve monsoon prediction across scales. Bull. Amer. Meteorol. Soc. 100, 2509–2532. doi: 10.1175/BAMS-D-17-0330.1
Rao, V. B., Rao, K. K., Mahendranath, B., Lakshmi Kumar, T. V., and Govardhan, D. (2021). Large-scale connection to deadly Indian heatwaves. Q. J. R. Meterol. Soc. 147, 1419–1430. doi: 10.1002/qj.3985
Rao, V. B., Reyes Fernandez, J. P., and Franchito, S. H. (2000). Monsoonlike circulations in a zonally averaged numerical model with topography. Month. Weath. Rev. 128, 779–794. doi: 10.1175/1520-0493(2000)128<0779:MCIAZA>2.0.CO;2
Raymond, D., Fuchs, Z., Gjorgjievska, S., and Sessions, S. (2015). Balanced dynamics and convection in the tropical atmosphere. J. Adv. Model. Earth Syst. 7, 1093–1116. doi: 10.1002/2015MS000467
Saha, S. K., Hazra, A., Pokhrel, S., Chaudhari, H. S., Sujith, K., Rai, A., et al. (2019). Unraveling the mystery of Indian summer monsoon prediction: improved estimate of predictability limit. J. Geophys. Res. Atmos. 124, 1962–1974. doi: 10.1029/2018JD030082
Sahai, A. K., Chattopadhyay, R., Joseph, S., Phani, R., and Abhilash, S. (2016). Extended range prediction system and its application. Vayu Mandal 42, 75–96. Available online at: http://imetsociety.org/wpcontent/pdf/vayumandal/2016422/2016422_3.pdf
Saranya Ganesh, S., Abhilash, S., Joseph, S., Kaur, M., Dey, A., Mandal, R., et al. (2021). A review of the development and implementation of tropical cyclone prediction system for North Indian Ocean in a multi-model ensemble framework. Mausam 72, 57–76. doi: 10.54302/mausam.v72i1.126
Sarkar, A., Kumar, S., Dube, A., Prasad, S. K., Mamgain, A., Chakraborty, P., et al. (2021). Forecasting of tropical cyclone using global and reginal ensemble prediction systems of NCMRWF: a review Mausam 72, 77–86. doi: 10.54302/mausam.v72i1.131
Sikka, D. R. (1977). Some aspects of the life history, structure and movement of monsoon depressions. Pageoph 115, 1501–1529. doi: 10.1007/BF00874421
Singh, V. K., Roxy, M. K., and Deshpande, M. (2021). Role of warm ocean conditions and the MJO in the genesis and intensification of extremely severe cyclone Fani. Sci. Rep. 11:3607. doi: 10.1038/s41598-021-82680-9
Sobel, A. H., Camargo, S. J., Hall, T. M., Lee, C.-Y., Tippett, M. K., and Wing, A. A. (2016). Human influence on tropical cyclone intensity. Science 353, 242–246. doi: 10.1126/science.aaf6574
Sugi, M., Murakami, H., and Yoshida, K. (2017). Projection of future changes in the frequency of intense tropical cyclones. Clim. Dyn. 49, 619–632 doi: 10.1007/s00382-016-3361-7
Tomassini, L. (2020). The interaction between moist convection and the atmospheric circulation in the tropics. Bull. Amer. Meteorol. Soc. 101, E1378–E1396. doi: 10.1175/BAMS-D-19-0180.1
Turner, A. G., Bhat, G. S., Martin, G. M., Parker, D. J., Taylor, C. M., Mitra, A. K., et al. (2019). Interaction of convective organization with monsoon precipitation, atmosphere, surface and sea: the 2016 INCOMPASS field campaign in India. Q. J. R. Meteorol. Soc. 146, 2828–2852. doi: 10.1002/qj.3633
Wang, B., Biasutti, M., Byrne, M. P., Castro, C., Chang, C., Cook, K., et al. (2021). Monsoons climate change assessment. Bull. Am. Meteorol. Soc. 102, E1–E19. doi: 10.1175/BAMS-D-19-0335.1
Keywords: KUM method, extreme weather, human suffering, tropical cyclone, monsoon, Indian summer monsoon (ISM)
Citation: Brahmananda Rao V, Ashok K and Govardhan D (2021) Unprecedented Climate Change in India and a Three-Pronged Method for Reliable Weather and Climate Prediction. Front. Clim. 3:716507. doi: 10.3389/fclim.2021.716507
Received: 28 May 2021; Accepted: 04 October 2021;
Published: 15 November 2021.
Edited by:
Bai-Lian Li, University of California, Riverside, United StatesReviewed by:
Ladislaus Benedict Chang'A, Tanzania Meteorological Agency, TanzaniaZablon W. Shilenje, Charles University, Czechia
Copyright © 2021 Brahmananda Rao, Ashok and Govardhan. This is an open-access article distributed under the terms of the Creative Commons Attribution License (CC BY). The use, distribution or reproduction in other forums is permitted, provided the original author(s) and the copyright owner(s) are credited and that the original publication in this journal is cited, in accordance with accepted academic practice. No use, distribution or reproduction is permitted which does not comply with these terms.
*Correspondence: Vadlamudi Brahmananda Rao, cmFvdmFkbGFtdWQmI3gwMDA0MDtnbWFpbC5jb20= orcid.org/0000-0001-5905-9806