- 1Department of Environmental Politics, Helmholtz Centre for Environmental Research-UFZ (Helmholtz-Zentrum für Umweltforschung), Leipzig, Germany
- 2Department of Bioenergy, Helmholtz Centre for Environmental Research-UFZ (Helmholtz-Zentrum für Umweltforschung), Leipzig, Germany
- 3Climate Service Center Germany (GERICS) - Helmholtz-Zentrum Geesthacht, Hamburg, Germany
- 4Bioenergy Systems Department, Deutsches Biomasseforschungszentrum - DBFZ (German Biomass Research Centre), Leipzig, Germany
- 5Biogeochemical Modelling, GEOMAR Helmholtz Centre for Ocean Research Kiel (Helmholtz-Zentrum für Ozeanforschung Kiel), Kiel, Germany
The 2015 Paris Agreement aims to strengthen the global response to climate change, and to maintain an average global temperature well below 2°C, with aspirations toward 1.5°C, by means of balancing sources and sinks of greenhouse gas emissions. Following this, the importance of carbon dioxide removal in global emission pathways has been further emphasized, and Negative Emissions Technologies (NETs) that capture carbon from the atmosphere and remove it from the system have been put in the spotlight. NETs range from innovative, engineered technologies, to well-known approaches like afforestation/reforestation. These technologies essentially compensate for a shrinking carbon budget coupled with hard-to-abate future emissions, and a historical lack of action. However, none has been deployed at scales close to what is envisioned in emission pathways in line with the Paris Agreement goals. To understand the potential contribution of NETs to meet global emission goals, we need to better understand opportunities and constraints for deploying NETs on a national level. We examine 17 Long-Term Low Greenhouse Gas Emission Development Strategies (LT-LEDS), and discuss them in the context of available NETs feasibility assessments. Our mapping shows that most countries include NETs in their long-term strategies, and that enhancement of natural sinks is the most dominating type of NET in these strategies. In line with many feasibility assessments, LT-LEDS focus on technical and biophysical considerations, and neglect socio-cultural dimensions. We suggest that feasibility assessments at the national level need to be more holistic; context-specific and comprehensive in terms of aspects assessed.
Introduction
The 2015 Paris Agreement of the United Nations Framework Convention on Climate Change (UNFCCC) aims to strengthen the global response to climate change, limiting the increase in global temperature to “well below” 2°C, with aspirations toward 1.5°C (United Nations, 2015). Importantly, the agreement specifies that the long-term temperature goal should be achieved by means of balancing sources and sinks of greenhouse gas emissions. To understand options and emission pathways compatible with this objective, the Intergovernmental Panel on Climate Change (IPCC) produced the Special Report on 1.5°C global warming (SR15) (IPCC, 2018; Livingston and Rummukainen, 2020). SR15 shows that all emission pathways consistent with a 1.5°C warming limit require near-term carbon dioxide removal at large scale in addition to reduced emissions (also Fuhrman et al., 2019; Gough and Mander, 2019).
Negative Emissions Technologies (NETs) capture and remove carbon from the system (see Table 1 for a comprehensive list). Their rationale is: the more emissions that can be removed, the more room for maneuver in terms of (a) residual hard-to-abate emissions in the future and/or (b) closing the ambition gap, i.e., compensating for lack of action in the past (Renforth and Wilcox, 2019; Forster et al., 2020; Markusson et al., 2020). In this paper, we explore how the feasibility of NETs deployment is operationalized in assessments and analyze NETs coverage in national Long-Term Low Greenhouse Gas Emission Development Strategies (LT-LEDS), with an emphasis on how feasibility of NETs is understood, and argue that holistic assessments of NETs deployment at the national level are urgently needed.
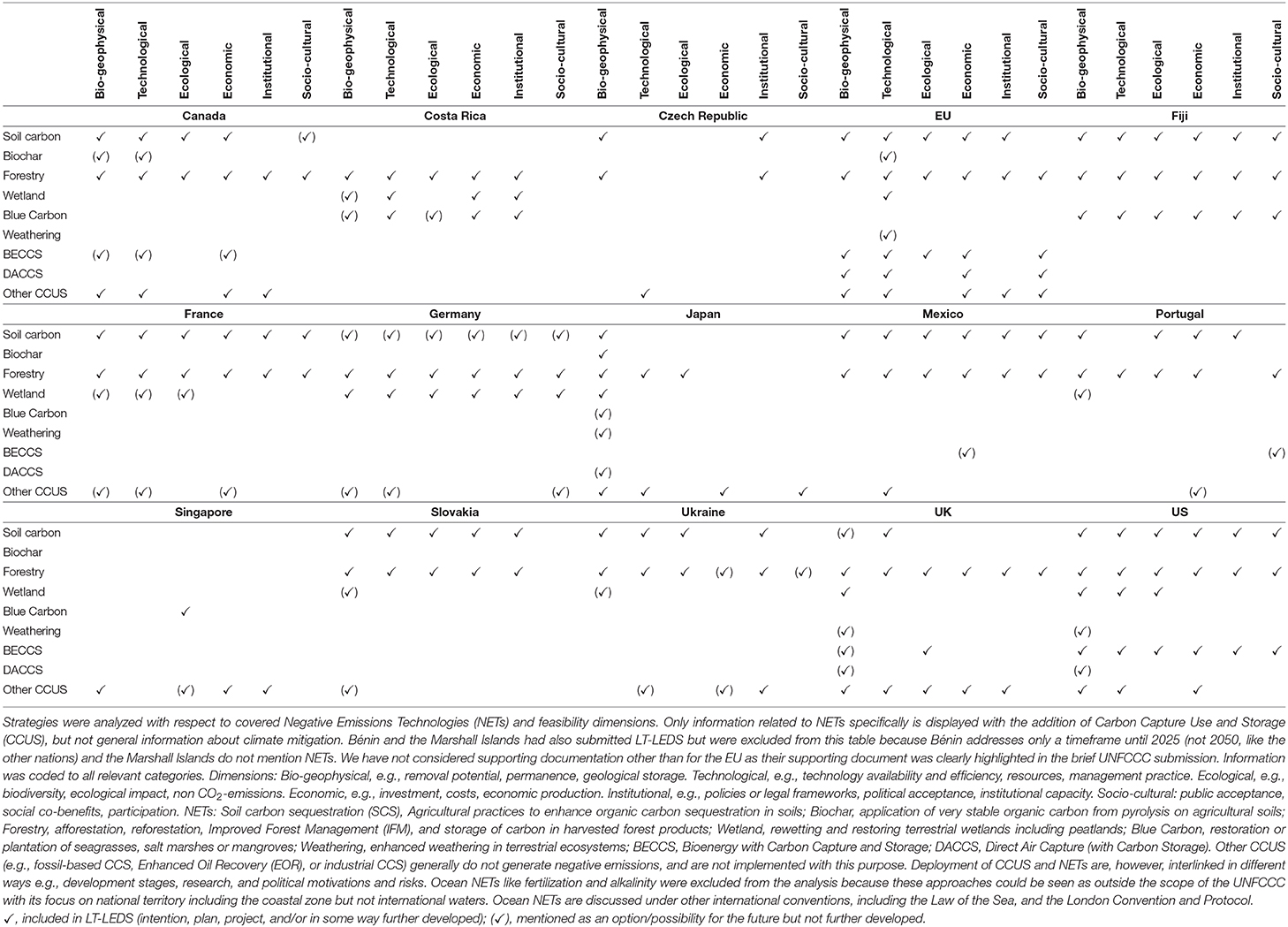
Table 1. Overview of 15 Long-Term Low Greenhouse Gas Emission Development Strategies (LT-LEDS) submitted to the United Nations Framework Convention on Climate Change (UNFCCC) as of July 2020.
Feasibility Operationalized in NETs Assessments
Within the last 10–15 years research into NETs and their feasibility has increased and multiple new ideas on how to remove carbon dioxide from the atmosphere have emerged. Emission pathways from Integrated Assessment Models (IAMs) most prominently feature Bioenergy with Carbon Capture and Storage (BECCS) and afforestation/reforestation (IPCC, 2018). While some approaches, such as afforestation/reforestation, have a long history in climate mitigation, the envisioned deployment scale in these pathways exceeds anything that has been deployed before (Fajardy et al., 2019; Carton et al., 2020). As NETs have not yet been scaled up, assessing the feasibility of deploying such technologies at a larger scale inevitably involves uncertainties about their implementation, effectiveness, and side effects (IPCC, 2018). These are so far mostly addressed by modeling studies, which bring about their own uncertainties (see section Feasibility Discussions, Oschlies and Klepper, 2017; Minx et al., 2018; Mengis et al., 2019).
Assessing the feasibility of NETs can be described as a process, with different assessments of feasibility carried out at different moments in time. These range from specific assessments like technology- or dimension-focused (e.g., Fuss et al., 2018; Nemet et al., 2018; Roe et al., 2019; Robb et al., 2020), reviews or syntheses of multiple dimensions or comparison of NETs (Oschlies and Klepper, 2017; Minx et al., 2018; Waller et al., 2020), and combined feasibility assessments (IPCC, 2018). These assessments accordingly differ in scope and how feasibility is operationalized.
The emergence of a new technology requires a scientific and technical evaluation. Technical assessments are conducted at all innovation stages to ensure solutions to emerging problems. Most attention in the scholarly literature has been given to research and development activities (R&D), whereas demonstration and upscaling have received less attention (Nemet et al., 2018). Technical assessments may focus on parameters related to technology efficiency and/or availability, and are important tools for assessing technological feasibility. Often technological feasibility is expressed as the maturity level of a certain technology, known as the Technology Readiness Level (TRL) (DOE, 2011). The TRL is composed of nine stages that progress sequentially from the conceptual stage (TRL 1), to commercial scale deployment (TRL 9)—a process that for complex technologies can take up to 30 years (Haszeldine et al., 2018). Progression to a higher TRL requires further research, financial investment and policy support (Bui et al., 2018). Currently, proposed NETs are at different maturity levels, but most have not advanced past the demonstration stage (TRL 6) (Lomax et al., 2015). In addition, separate components of individual NETs can range in TRL (Hepburn et al., 2019).
In contrast to technology-focused (focus on specific NET) or dimension-specific studies (e.g., focusing on technological, ecological, or economic aspects), reviews of NETs often compare multiple feasibility dimensions and/or NETs. Here, feasibility is often defined by carbon sequestration potential and efficiency, deployment costs and timeline, which correspond to the TRL and risks (Oschlies and Klepper, 2017). Combining multiple dimensions in the assessment can highlight temporal, spatial, and technological aspects enabling or hindering the scale-up potential of a technology (Minx et al., 2018; Forster et al., 2020). Assessments of NETs have been criticized for being too narrow in their evaluation of what enabling conditions need to be met, with most focusing on geophysical, technological, and economic aspects (Oschlies et al., 2017; Mengis et al., 2019; Kreuter et al., 2020; Waller et al., 2020).
Finally, a comprehensive assessment of a range of NETs across multiple feasibility dimensions has been carried out by the IPCC (see SR15). Here, feasibility is broken down into several dimensions, ranging from economic, technological, institutional, socio-cultural, ecological, and geophysical, operationalized with accompanying indicators (de Coninck et al., 2018a). Feasibility is assessed based on the barriers that exist for a specific NET. Enabling conditions, such as financial support, institutional capacity and innovation, are seen as affecting the feasibility of options (technologies, actions, and measures), and can accelerate and scale up systemic transitions. SR15 also identified where there was no or limited evidence for feasibility and underlined existing research gaps, the most obvious ones being the lack of evidence for institutional and socio-cultural feasibility for many NETs. Moreover, in order to enable an assessment of these dimensions, for this global scale assessment to be applicable at the national level, it must be adapted accordingly.
NETs at the National Level: Insights From 17 LT-LEDS
The Paris Agreement requests countries to submit Nationally Determined Contributions (NDCs), and invites them to submit LT-LEDS to the UNFCCC. Here, we focus on the LT-LEDS, as NETs are more likely to be addressed in long-term considerations. As of September 2020, 16 countries plus the European Union (EU) had submitted their LT-LEDS (Table 1, Supplementary Material)1.
NETs are highlighted as important for achieving the Paris Agreement's goal (e.g., Canada, EU, Japan, Slovakia, and UK), and in particular enhancements of natural sinks feature most heavily in the LT-LEDS examined in the present study (Table 1). It is important to note, however, that the land-use sector is often treated as a whole, without clearly separating negative emissions (cf. Dooley and Gupta, 2017; Minx et al., 2018). There is a degree of flexibility in the LT-LEDS with several countries including pathways where enhancement of natural sinks is essential for compensating for residual emissions, while BECCS feature in some pathways providing more time to transform society; without BECCS, emissions need to be reduced faster (e.g., EU, UK, and US). The notions of feasibility of NETs deployment vary in scope, level of detail and focus (see Table 1). For instance, Canada, the UK and the US consider a broad range of NETs, whereas Fiji focuses on enhancement of natural sinks including a comprehensive strategy for Blue Carbon and Germany focuses on forests, wetlands and peatlands.
The LT-LEDS feature examples of specific pilot studies, research, and deployment initiatives for NETs [e.g., dedicated research program (UK), funding for soil carbon potential and BECCS-pilot (US), and mangrove restoration projects (Singapore)]. However, the general level of detail on how NETs would be deployed is low. Such knowledge will be needed to scale up NETs deployment on a national level and it could also be instrumental in bridging the gap between global IAM assessments and action on the ground.
In terms of feasibility dimensions, national LT-LEDS generally incorporate a narrow view, i.e., focusing mostly on bio-geophysical and technological dimensions (Table 1). In addition, for NETs that focus on the enhancement of natural sinks, environmental dimensions are considered to a larger extent, while these dimensions are less pronounced for more technology-heavy NETs. For all NETs, socio-cultural feasibility is underrepresented. In summary, the LT-LEDS indicate which NETs are considered within the political reality of a country (indicating political feasibility), but they often do not provide a detailed assessment of the current status of research and implementation of specific NETs within a country (e.g., technical scalability or social acceptance).
Most strategies use a conditional understanding of NETs feasibility, identifying enabling conditions/barriers to deployment such as costs or knowledge gaps (e.g., Canada, Portugal, and US in the context of BECCS). However, countries differ in how they operationalize these conditions. For instance, Portugal currently excludes BECCS due to high costs, mentioning it only as a possible option for the future, while for instance the US and the UK explore different pathways with and without BECCS. In our mapping, we have also included Carbon Capture Usage and Storage (Table 1, “other CCUS”) without bioenergy even though they do not generate negative emissions because they are relevant for the development of BECCS and may impact their social acceptance (cf. Lock et al., 2014; Thomas et al., 2018).
Feasibility Discussions
The feasibility assessments and national LT-LEDS discussed herein foreground two different albeit interrelated discussions regarding feasibility that are relevant in the context of NETs deployment. The first discussion starts with a given policy goal and asks what is needed to reach this goal. This question is typically investigated using modeling, and the answer is typically that NETs are needed at a large scale to complement other mitigation approaches (e.g., IPCC, 2018). Examples include IAMs that look for pathways to limit temperature rise at a given level, and national strategies on how to reach net-zero emissions.
The second discussion instead starts with the NETs and asks questions regarding necessary enabling conditions or barriers to deploy NETs. This discussion can be narrow; focusing on a specific technology or dimension, or it can be broad comparing NETs and dimensions. As shown herein, both feasibility assessments and LT-LEDS tend to focus on bio-geophysical, technical, and to some extent economic dimensions, neglecting socio-cultural dimensions.
SR15 is perhaps the most prominent example of featuring both discussions. The report relies strongly on results from IAMs to identify feasible emission pathways to limit the increase of the average global temperature to 1.5°C, in turn feeding into the broader IPCC assessment of mitigation options (Low and Schäfer, 2020). It also, however, highlights a range of NETs and includes a comprehensive set of feasibility dimensions with indicators. That said, only some dimensions are comprehensively assessed in the context of NETs—most prominently geophysical, technological and economic dimensions (de Coninck et al., 2018b). Socio-cultural, institutional and to some extent ecological dimensions are not comprehensively assessed due to the lack of underlying research, instead highlighted as important uncertainties (de Coninck et al., 2018a,b).
Feasibility in the IAM-context equals model solvability, which in turn depends on model assumptions (Low and Schäfer, 2020). IAMs focus on a techno-economic context, excluding a range of other dimensions that could hinder or enable actual deployment (Fuss et al., 2014; Forster et al., 2020). If not revised carefully, such an approach may facilitate even highly improbable pathways to appear feasible. For example, when coupled with discounted future costs for action on climate change, a narrow understanding of NETs feasibility can fabricate a high reliance on future NETs and justify delayed action (Köberle, 2019; Rogelj et al., 2019). A recent study found that assumptions built into IAMs about NETs deployment could amount to an additional temperature rise of 1.4°C if these technologies do not deliver as assumed (McLaren, 2020).
In terms of the diversity of NETs considered in IAMs, BECCS, and afforestation/reforestation dominate. This can partly be understood based on traditions in IAM climate modeling focusing on the energy sector and emissions, rather than suggesting that these approaches are more feasible or more desirable than others (Fuhrman et al., 2019). This predominance, however, introduces a bias in the assessments toward a higher perceived feasibility for these technologies.
The LT-LEDS are developed following the Paris Agreement, “mindful of” its long-term temperature goal (Articles 4 and 2 of the Paris Agreement). Consequently, they feed into and relate to a feasibility-of-the-goal discussion. As illustrated in Table 1, NETs have a role to play in most LT-LEDS communicated thus far. While some countries communicate that the deployment of NETs is important to fulfill the Paris Agreement, they also highlight a number of hindering conditions that need to be addressed, including costs and knowledge-gaps (e.g., Canada, EU, and US). When feasibility of NETs deployment is addressed, this is typically narrowly framed, excluding most prominently socio-cultural dimensions (Figure 1). This leaves many important questions unanswered and thereby limits the credibility of the underlying assumptions made in the LT-LEDS.
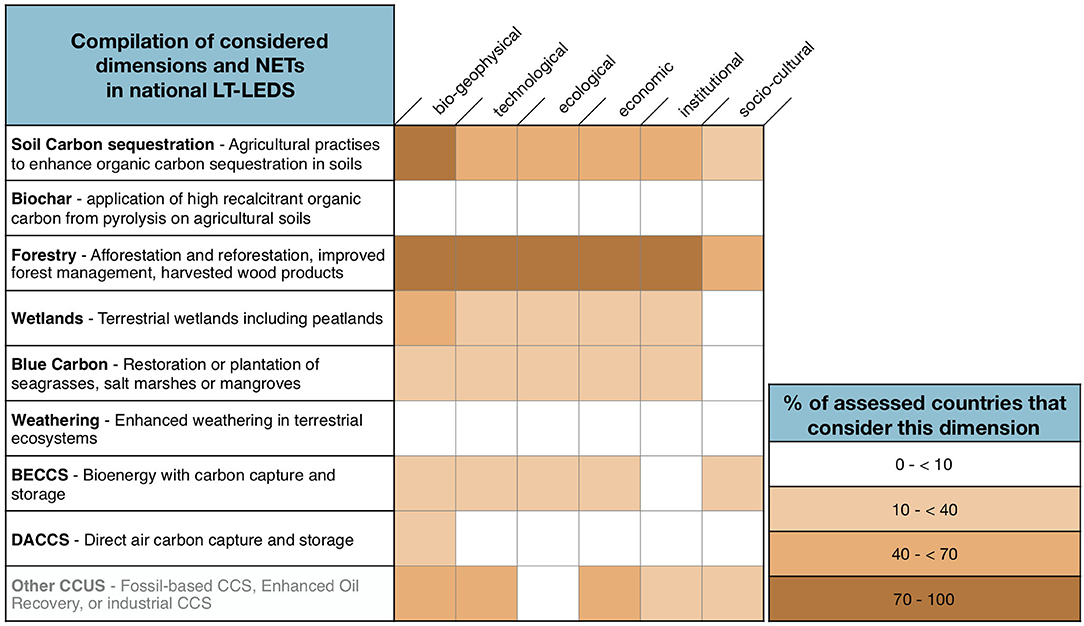
Figure 1. Percentage of Long-Term Low Greenhouse Gas Emissions Development Strategies (LT-LEDS) that cover a given NET, considering one of six feasibility dimensions. This is a compilation of the analysis from Table 1, the percentage is calculated as the number of LT-LEDS that include (=1) or mention (=0.5) a NET and feasibility dimension, relative to the total number of considered LT-LEDS, excluding Benin and the Marshall Islands (N = 15). The category “other CCUS” in gray refers to CCUS techniques without bioenergy. These do not produce negative emissions, but are included as reference based on their interlinkage with BECCS (see also section NETs at the National Level: Insights From 17 LT-LEDS).
A narrow understanding of feasibility generated by, for example, considering the potential of NETs in isolation from one another rather than as a portfolio, or not considering socio-cultural or institutional dimensions, risks creating unrealistic expectations regarding the potential of NETs deployment (Fajardy et al., 2019; Low and Schäfer, 2020). This is problematic, because it has been shown that for example social acceptance can be an important barrier to the deployment of new technologies (Lock et al., 2014; Dowd et al., 2015). A narrow feasibility discussion focusing on what can be done (technically), risks losing sight of the normative foundation of climate policy discussions, namely the future we want. While there is a growing social science and humanities literature on NETs, it is important that these disciplines are properly integrated into research projects and policy assessments, complementing or challenging dominating narratives (Markusson et al., 2020, Waller et al., 2020). This would provide breadth to consider the risk that NETs might not be feasible at large scale, as well as adequately exploring alternative futures (Buck, 2016; Beck and Mahony, 2018; McLaren et al., 2019; Low and Buck, 2020). Asking critical questions about feasibility is a first step to opening up the debate regarding the way forward, rather than taking it for granted. Moreover, to better understand socio-cultural dimensions of NETs, such as participation and acceptance, a broader range of actors included in the knowledge-making process could help us better understand local realities of NETs deployment [e.g., Markusson et al. (2020)].
Moving Forward: Holistic Feasibility Assessments at the National Level
Because many NETs are still at an early stage of development, uncertainties remain regarding the feasibility and implications of their large-scale deployment (Fuss et al., 2014, 2018; Low and Schäfer, 2020). Thus far, the debate on NETs has predominantly been held at a global level, and it has previously been suggested that we need to better understand feasibility of NETs deployment at the national level (de Coninck et al., 2018a; Fajardy et al., 2019). In this paper, we have seen that many national strategies include NETs in their long-term mitigation portfolio to meet their national goals and contribute to the fulfillment of the Paris Agreement. However, when it comes to feasibility, focus is given to some dimensions (bio-geophysical, technological), while others, primarily socio-cultural, are neglected (Table 1, Figure 1). Turning to feasibility assessments, we found that these range in scope from focusing on specific components of technologies, to broad, global, assessments. Regardless of the type of assessment, these too tend to provide little information on socio-cultural as well as institutional dimensions. Going forward, new tools are needed to inform and catalyze a discussion with and for national policy that are: (1) scaled down and context-specific and (2) comprehensive in terms of dimensions covered.
The LT-LEDS analyzed for this paper generally specify a goal and a pathway, but lack the comprehensive assessment that would help to improve our understanding about concrete challenges and trade-offs at the national level, and the realistic potential of NETs at the global level. In theory, a holistic feasibility assessment could cover an almost endless number of enabling and/or hindering conditions. Holistic feasibility assessments therefore need to reflect their specific purpose, and be tailored to the national context (Oschlies and Klepper, 2017; Fajardy et al., 2019). NETs vary in nature, and not all will be suitable for all countries, as the LT-LEDS also indicate. For instance, some countries are geologically not suitable for CO2-storage (e.g., Singapore's LT-LEDS), while others face social or institutional barriers for certain NETs (Fridahl and Lehtveer, 2018; Geden et al., 2018). Moreover, NETs are neither static nor singular and can be broken down into components or procedural steps (e.g., TRLs as described in section Feasibility Operationalized in NETs Assessments). In addition, they need to be understood in relation to other societal goals such as energy security and sustainable development at the national level, as well as trade-offs resulting from the maximization of one ecosystem-service (carbon sequestration) before others (Dooley and Kartha, 2018; Fajardy and Mac Dowell, 2018; Carton et al., 2020). Moreover, socio-cultural dimensions are not only potential barriers to deployment, but can also be potential drivers (Beck and Mahony, 2018; Fajardy et al., 2019; Waller et al., 2020) and thus holistic assessments need to embrace this dynamism.
Finally, it is important to recognize that the discussion on feasibility of NETs deployment is bound up with discussions regarding the feasibility of reaching specific policy goals in the future. NETs are commonly described as a necessary means to reaching these goals, supported by emission pathways generated by IAMs. However, IAMs assume NETs can be deployed at a large scale. Looking at the technology development rate of NETs, it is uncertain if NETs can be timely scaled-up in line with model assumptions (Nemet et al., 2018). It is therefore important that assumptions made about future deployments of NETs are complemented with holistic feasibility assessments. With new NDCs due in 2020 and the submission of LT-LEDS further encouraged, now is the time to holistically assess NETs deployment, so that in the future these strategies are more firmly anchored to the national context.
Data Availability Statement
All datasets generated for this study are included in the article/Supplementary Material.
Author Contributions
SB, MB, JF, TT, and DT conceived of and designed the study. JF, KG, AH, AS, and TT analyzed the national strategies. JF and TT designed Table 1. NM conceived of and created Figure 1. SB, MB, NM, DT, and TT reviewed feasibility assessments. TT led the drafting of the paper, with contributions from all co-authors. AS edited the text. All authors contributed to the article and approved the submitted version.
Funding
The Helmholtz-Climate-Initiative (HI-CAM) is funded by the Helmholtz Associations Initiative and Networking Fund. The authors are responsible for the content of this publication.
Conflict of Interest
The authors declare that the research was conducted in the absence of any commercial or financial relationships that could be construed as a potential conflict of interest.
The handling editor declared a past co-authorship with one of the authors DT.
Acknowledgments
The authors would like to thank Till Markus, Klaas Korte, Romina Luz Schaller, and Erik Gawel for valuable discussions, and Anne Wessner for support with layout. We would also like to thank the three reviewers whose comments helped improve and clarify this manuscript.
Supplementary Material
The Supplementary Material for this article can be found online at: https://www.frontiersin.org/articles/10.3389/fclim.2020.590305/full#supplementary-material
Footnote
1. ^At the moment of writing, it is unclear how many more LT-LEDS are to be expected, as they are not mandatory. Once/if more LT-LEDS are made available, a more comprehensive analysis would be possible. The current paper should thus be seen as a contribution to a rapidly unfolding debate, not a comprehensive and final overview.
References
Beck, S., and Mahony, M. (2018). The politics of anticipation: the IPCC and the negative emissions technologies experience. Glob. Sustain. 1:e8. doi: 10.1017/sus.2018.7
Buck, H. J. (2016). Rapid scale-up of negative emissions technologies: social barriers and social implications. Clim. Change 139, 155–167. doi: 10.1007/s10584-016-1770-6
Bui, M., Adjiman, C. S., Bardow, A., Anthony, E. J., Boston, A., Brown, S., et al. (2018). Carbon capture and storage (CCS): the way forward. Energy Environ. Sci. 11, 1062–1176. doi: 10.1039/C7EE02342A
Carton, W., Asiyanbi, A. P., Beck, S., Buck, H. J., and Lund, J. F. (2020). Negative emissions and the long history of carbon removal. Wiley Interdisciplinary Rev. Clim. Change. 11:e671. doi: 10.1002/wcc.671
de Coninck, H., Revi, A., Babiker, M., Bertoldi, P., Buckeridge, M., Cartwright, A., et al. (2018a). “Strengthening and implementing the global response,” in Global Warming of 1.5°C. An IPCC Special Report on the Impacts of Global Warming of 1.5°C above Pre-Industrial Levels and Related Global Greenhouse Gas Emission Pathways, in the Context of Strengthening the Global Response to the Threat of Climate Change, Sustainable Development, and Efforts to Eradicate Poverty. eds V. MassonDelmotte, P. Zhai, H. O. Pörtner, D. Roberts, J. Skea, P. R. Shukla, et al. 313–443.
de Coninck, H. A., Revi, M., Babiker, P., Bertoldi, M., Buckeridge, A., Cartwright, W., et al. (2018b). “Strengthening and implementing the global response supplementary material,” in Global Warming of 1.5°C. An IPCC Special Report on the Impacts of Global Warming of 1.5°C above Pre-Industrial Levels and Related Global Greenhouse Gas Emission Pathways, in the Context of Strengthening the Global Response to the Threat of Climate Change, Sustainable Development, and Efforts to Eradicate Poverty. eds V. Masson-Delmotte, P. Zhai, H. O. Pörtner, D. Roberts, J. Skea, P. R. Shukla, et al. Available online at: https://www.ipcc.ch/sr15
DOE, (2011). Technology Readiness Assessment Guide. Washington, DC: U.S. Department of Energy. Available online at: https://www.directives.doe.gov/directives-documents/400-series/0413.3-EGuide-04 (accessed October 18, 2020).
Dooley, K., and Gupta, A. (2017). Governing by expertise: the contested politics of (accounting for) land-based mitigation in a new climate agreement. Int. Environ. Agreements 17, 483–500. doi: 10.1007/s10784-016-9331-z
Dooley, K., and Kartha, S. (2018). Land-based negative emissions: risks for climate mitigation and impacts on sustainable development. Int. Environ. Agreements 18, 79–98. doi: 10.1007/s10784-017-9382-9
Dowd, A. M., Rodriguez, M., and Jeanneret, T. (2015). Social science insights for the BioCCS industry. Energies 8, 4024–4042. doi: 10.3390/en8054024
Fajardy, M., and Mac Dowell, N. (2018). The energy return on investment of BECCS: is BECCS a threat to energy security? Energy Environ. Sci. 11, 1581–1594. doi: 10.1039/C7EE03610H
Fajardy, M., Patrizio, P., Daggash, H. A., and Mac Dowell, N. (2019). Negative emissions: priorities for research and policy design. Front. Clim. 1:6. doi: 10.3389/fclim.2019.00006
Forster, J., Vaughan, N. E., Gough, C., Lorenzoni, I., and Chilvers, J. (2020). Mapping feasibilities of greenhouse gas removal: key issues, gaps and opening up assessments. Glob. Environ. Change. 63:102073. doi: 10.1016/j.gloenvcha.2020.102073
Fridahl, M., and Lehtveer, M. (2018). Bioenergy with carbon capture and storage (BECCS): Global potential, investment preferences, and deployment barriers. Energy Res. Soc. Sci. 42, 155–165. doi: 10.1016/j.erss.2018.03.019
Fuhrman, J., McJeon, H., Doney, S. C., Shobe, W., and Clarens, A. F. (2019). From zero to hero?: Why integrated assessment modeling of negative emissions technologies is hard and how we can do better. Front. Clim. 1:11. doi: 10.3389/fclim.2019.00011
Fuss, S., Canadell, J. G., Peters, G. P., Tavoni, M., Andrew, R. M., Ciais, P., et al. (2014). Betting on negative emissions. Nat. Clim. Change. 4, 850–853. doi: 10.1038/nclimate2392
Fuss, S., Lamb, W. F., Callaghan, M. W., Hilaire, J., Creutzig, F., Amann, T., et al. (2018). Negative emissions—Part 2: costs, potentials and side effects. Environ. Res. Lett. 13:063002. doi: 10.1088/1748-9326/aabf9f
Geden, O., Scott, V., and Palmer, J. (2018). Integrating carbon dioxide removal into EU climate policy: Prospects for a paradigm shift. WIREs Climate Change 9:e521. doi: 10.1002/wcc.521
Gough, C., and Mander, S. (2019). Beyond social acceptability: applying lessons from CCS social science to support deployment of BECCS. Curr. Sustain. Renew. Energy Rep. 6, 116–123. doi: 10.1007/s40518-019-00137-0
Haszeldine, R. S., Flude, S., Johnson, G., and Scott, V. (2018). Negative emissions technologies and carbon capture and storage to achieve the Paris Agreement commitments. Philos. Trans. R Soc. A Math. Phys. Eng. Sci. 376:20160447. doi: 10.1098/rsta.2016.0447
Hepburn, C., Adlen, E., Beddington, J., Carter, E. A., Fuss, S., Mac Dowell, N., et al. (2019). The technological and economic prospects for CO2 utilization and removal. Nature 575, 87–97. doi: 10.1038/s41586-019-1681-6
IPCC (2018). Global warming of 1.5°C. An IPCC Special Report on the Impacts of Global Warming of 1.5° C Above Pre-industrial Levels and Related Global Greenhouse Gas Emission Pathways, in the Context of Strengthening the Global Response to the Threat of Climate Change, Sustainable Development, and Efforts to Eradicate Poverty, eds V. Masson-Delmotte, P. Zhai, H. O. Pörtner, D. Roberts, J. Skea, P. R. Shukla, A. Pirani, W. Moufouma-Okia, C. Péan, R. Pidcock, S. Connors, J. B. R. Matthews, Y. Chen, X. Zhou, M. I. Gomis, E. Lonnoy, T. Maycock, M. Tignor, and T. Waterfield. 1–562.
Köberle, A. C. (2019). The value of BECCS in IAMs: a review. Curr. Sustain. Renew. Energy Rep. 6, 107–115. doi: 10.1007/s40518-019-00142-3
Kreuter, J., Matzner, N., Baatz, C., Keller, D. P., Markus, T., Wittstock, F., et al. (2020). Unveiling assumptions through interdisciplinary scrutiny: observations from the German Priority Program on Climate Engineering (SPP 1689). Clim. Change. 162, 57–60. doi: 10.1007/s10584-020-02777-4
Livingston, J. E., and Rummukainen, M. (2020). Taking science by surprise: the knowledge politics of the IPCC Special Report on 1.5 degrees. Environ. Sci. Policy 112, 10–16. doi: 10.1016/j.envsci.2020.05.020
Lock, S. J., Smallman, M., Lee, M., and Rydin, Y. (2014). “Nuclear energy sounded wonderful 40 years ago”: UK citizen views on CCS. Energy Pol. 66, 428–435. doi: 10.1016/j.enpol.2013.11.024
Lomax, G., Lenton, T. M., Adeosun, A., and Workman, M. (2015). Investing in negative emissions. Nat. Clim. Change 5, 498–500. doi: 10.1038/nclimate2627
Low, S., and Buck, H. J. (2020). The practice of responsible research and innovation in “climate engineering”. WIREs Clim. Change 11:e644. doi: 10.1002/wcc.644
Low, S., and Schäfer, S. (2020). Is bio-energy carbon capture and storage (BECCS) feasible? The contested authority of integrated assessment modeling. Energy Res. Soc. Sci. 60:101326. doi: 10.1016/j.erss.2019.101326
Markusson, N., Balta-Ozkan, N., Chilvers, J., Healey, P., Reiner, D., and McLaren, D. (2020). Social science sequestered. Front. Clim. 2:2. doi: 10.3389/fclim.2020.00002
McLaren, D. (2020). Quantifying the potential scale of mitigation deterrence from greenhouse gas removal techniques. Clim. Change. doi: 10.1007/s10584-020-02732-3
McLaren, D. P., Tyfield, D. P., Willis, R., Szerszynski, B., and Markusson, N. O. (2019). Beyond “net-zero”: a case for separate targets for emissions reduction and negative emissions. Front. Clim. 1:4. doi: 10.3389/fclim.2019.00004
Mengis, N., Keller, D. P., Rickels, W., Quaas, M., and Oschlies, A. (2019). Climate engineering–induced changes in correlations between Earth system variables—implications for appropriate indicator selection. Clim. Change 153, 305–322. doi: 10.1007/s10584-019-02389-7
Minx, J. C., Lamb, W. F., Callaghan, M. W., Fuss, S., Hilaire, J., Creutzig, F., et al. (2018). Negative emissions—Part 1: research landscape and synthesis. Environ. Res. Lett. 13:063001. doi: 10.1088/1748-9326/aabf9b
Nemet, G. F., Callaghan, M. W., Creutzig, F., Fuss, S., Hartmann, J., Hilaire, J., et al. (2018). Negative emissions—Part 3: Innovation and upscaling. Environ. Res. Lett. 13:063003. doi: 10.1088/1748-9326/aabff4
Oschlies, A., Held, H., Keller, D., Keller, K., Mengis, N., Quaas, M., et al. (2017). Indicators and metrics for the assessment of climate engineering. Earth's Future 5, 49–58. doi: 10.1002/2016EF000449
Oschlies, A., and Klepper, G. (2017). Research for assessment, not deployment, of climate engineering: the German research foundation's priority program SPP 1689. Earth's Future 5, 128–134. doi: 10.1002/2016EF000446
Renforth, P., and Wilcox, J. (2019). Specialty grand challenge: negative emission technologies. Front. Clim. 1:1. doi: 10.3389/fclim.2019.00001
Robb, S., Joseph, S., Abdul Aziz, A., Dargusch, P., and Tisdell, C. (2020). Biochar's cost constraints are overcome in small-scale farming on tropical soils in lower-income countries. Land Degrad. Dev. 31, 1713–1726. doi: 10.1002/ldr.3541
Roe, S., Streck, C., Obersteiner, M., Frank, S., Griscom, B., Drouet, L., et al. (2019). Contribution of the land sector to a 1.5°C world. Nat. Clim. Change 9, 817–828. doi: 10.1038/s41558-019-0591-9
Rogelj, J., Huppmann, D., Krey, V., Riahi, K., Clarke, L., Gidden, M., et al. (2019). A new scenario logic for the Paris Agreement long-term temperature goal. Nature 573, 357–363. doi: 10.1038/s41586-019-1541-4
Thomas, G., Pidgeon, N., and Roberts, E. (2018). Ambivalence, naturalness and normality in public perceptions of carbon capture and storage in biomass, fossil energy, and industrial applications in the United Kingdom. Energy Res. Soc. Sci. 46, 1–9. doi: 10.1016/j.erss.2018.06.007
United Nations (2015). “Paris Agreement”. (UNFCCC). Available online at: https://unfccc.int/process-and-meetings/the-paris-agreement/the-paris-agreement (accessed July 30, 2020).
Keywords: Negative Emissions Technology (NET), net-zero, UNFCCC (United Nations Framework Convention on Climate Change), Long-Term Low Greenhouse Gas Emission Development Strategies (LT-LEDS), feasibility assessment, integrated assessment modeling (IAM), pathway, IPCC SR15
Citation: Thoni T, Beck S, Borchers M, Förster J, Görl K, Hahn A, Mengis N, Stevenson A and Thrän D (2020) Deployment of Negative Emissions Technologies at the National Level: A Need for Holistic Feasibility Assessments. Front. Clim. 2:590305. doi: 10.3389/fclim.2020.590305
Received: 31 July 2020; Accepted: 29 September 2020;
Published: 20 November 2020.
Edited by:
Patrick Lamers, National Renewable Energy Laboratory (DOE), United StatesReviewed by:
José Luis Vicente-Vicente, Leibniz Center for Agricultural Landscape Research (ZALF), GermanyPhillip Williamson, University of East Anglia, United Kingdom
Jose Moreira, University of São Paulo, Brazil
Copyright © 2020 Thoni, Beck, Borchers, Förster, Görl, Hahn, Mengis, Stevenson and Thrän. This is an open-access article distributed under the terms of the Creative Commons Attribution License (CC BY). The use, distribution or reproduction in other forums is permitted, provided the original author(s) and the copyright owner(s) are credited and that the original publication in this journal is cited, in accordance with accepted academic practice. No use, distribution or reproduction is permitted which does not comply with these terms.
*Correspondence: Terese Thoni, terese.thoni@ufz.de