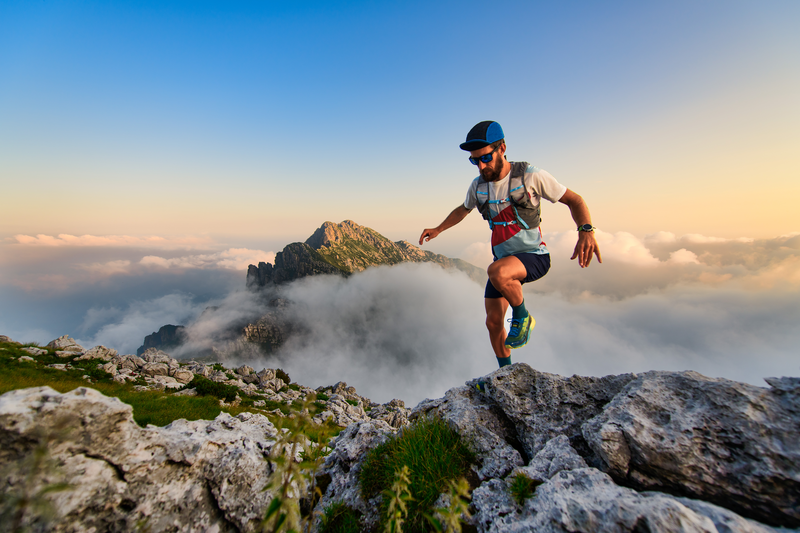
95% of researchers rate our articles as excellent or good
Learn more about the work of our research integrity team to safeguard the quality of each article we publish.
Find out more
ORIGINAL RESEARCH article
Front. Chem. , 25 March 2025
Sec. Catalytic Reactions and Chemistry
Volume 13 - 2025 | https://doi.org/10.3389/fchem.2025.1579108
Metal-organic frameworks (MOFs) are recognized as important Fenton-like materials for environmental remediation. However, their applications are often hindered by slow cycling between Fe (III) and Fe (II). This study aimed to address the slow Fe (III)/Fe (II) cycling limitation of Fe-MOFs through dual modification strategy: bimetallic modification and amino functionalization. A series of NH2-MOF(Fe, Co) catalysts with varying Fe/Co ratios were synthesized via a hydrothermal method and evaluated for sulfamethoxazole (SMX) degradation. The optimized NH2-MOF(Fe, Co) catalyst (Fe/Co ratio = 7:3) exhibited substantially enhanced catalytic performance, with SMX removal rate and rate constant in the H2O2 system being 3.2 and 43.5 times higher than those of the Fe-MOF/H2O2 system, respectively. The catalyst demonstrated robust performance across a wide pH range (3.05–7.00), addressing a common limitation of Fenton-like systems. Physicochemical characterization revealed that the enhanced performance was attributed to two key factors: the synergistic effect between Co and Fe in the bimetallic active center, and improved electron transfer to the central metal due to -NH2 functionalization. These modifications effectively addressed the Fe (III)/Fe (II) redox cycling limitation. The proposed reaction mechanism provides insights into SMX degradation pathways in the NH2-MOF(Fe, Co)/H2O2 system. This study presents an efficient and stable MOF-based Fenton-like catalyst with potential applications in wastewater treatment and environmental remediation.
Sulfamethoxazole (SMX) stands out as one of the most extensively used antibiotics in modern medicine, with its key information summarized in Supplementary Table S1 (Huang and Yang, 2021; Wang et al., 2024). Widely prescribed in hospital settings for the treatment a various diseases range of ailments, SMX has become a cornerstone of contemporary medical practice. However, its widespread use has led to significant environmental concerns. Conventional water and wastewater treatment processes often prove inadequate in completely remove it, resulting in its frequent detection in wastewater effluents (Pei et al., 2021; Da Silva Rodrigues et al., 2020). Consequently, SMX residues infiltrate both surface and groundwater systems, leading to a worrying accumulation of antibiotics in natural and human-habited environments, which harms non-target organisms and increases the risk of spreading drug-resistant bacteria and resistance genes (Mishra et al., 2023). Meanwhile, the high stability and antimicrobial properties of SMX further complicate its removal, particularly limiting the effectiveness of biological treatments (Suzuki et al., 2022; Kang et al., 2018).
Fenton-like technology, an advanced oxidation process, has emerged as one of the most effective chemical methods for treating organic pollutants (Wang J. F. et al., 2022; Zhang et al., 2023; Ikehata et al., 2008). Its efficacy stem from the generation of highly oxidative hydroxyl radicals (⋅OH), which rapidly and completely degrade pollutants without secondary pollution. Within this field, metal-organic frameworks (MOFs) have gained significant attention. These porous coordination polymers, formed by the self-assembly of metal ions or clusters with organic ligands, are particularly well-suited for Fenton-like technology due to their unique properties: high activity and selectivity, large specific surface area, adjustable porosity, and the ability to facility Fe2+/Fe3+ interconversion (Ma H. et al., 2021; Chen et al., 2024; Li S. et al., 2021). Recent years have seen a surge in the use of Fe-based MOFs as Fenton-like catalysts for treating recalcitrant organic pollutants. These catalysts effectively address the traditional challenges of Fenton processes, such as narrow pH range requirements and excessive iron sludge production, while achieving excellent degradation results (Sharma and Feng, 2019; Wang L. et al., 2022).
The strong interaction of H2O2 with the Fe sites in Fe-MOFs makes them react efficiently. The main challenge lies in significantly increasing the Fe (III)/Fe (II) redox rate to enhance Fe-MOFs Fenton-like properties. Various strategies have been explored, including incorporating heterogeneous materials (Du et al., 2022), construction of Fe (II)@MOFs materials (Lv et al., 2015), and utilizing light sources (Ke et al., 2019). These approaches have greatly advanced the development of MOFs materials in Fenton-like reactions and improved the efficiency of pollutant treatment. In recent years, research on bimetallic have gained popularity. Constructing a bimetallic synergistic reaction system by introducing mixed or doped metal centers (Fe/M, where M = Cu, Mn, Co, Ni) has proven effective in increasing catalytic activity. For instance, Kulandaivel synthesized Al/Fe-based MOF nanomaterials that rapidly degraded methylene blue dye within 45 min (Kulandaivel et al., 2024). Song increased the metal atom density by synthesizing Ni/Fe bimetallic cluster materials, utilizing inter-cluster for enhanced tetracycline removal (Song et al., 2023).
Functionalized of Fe-MOFs is another effective approach to enhance catalytic performance (Ma X. et al., 2021). Introducing substituents loke -OH and -NH2 improves pollutant adsorption by altering the catalysts’ electronic structure and increasing electron transfer (Kaur et al., 2024). For instance, NH2-MIL-101(Fe), NH2-MIL-53(Fe), and NH2-MIL-88B(Fe) have shown accelerated electron transfer and improved catalytic performance (Feng et al., 2023). The introduction of electrophilic amino groups the side chains can further accelerate the central metal’s redox activity and increase the catalyst’s redox potential (Chen et al., 2023). While Fe-MOFs show promise in environmental remediation, their widespread application is hindered by slow Fe (III)/Fe (II) cycling. Previous studies have explored either bimetallic synergy or functional modification separately, but few have investigated their combined effects.
Based on these considerations, we proposed a dual-modification strategy combining bimetallic synergy and amino functionalization to address the Fe (III)/Fe (II) cycling limitation in Fe-MOFs. NH2-MOF(Fe, Co) catalysts were synthesized via a facile hydrothermal method using 2-amino terephthalic acid as the organic linker, where the amino groups were expected to facilitate electron transfer while the Co incorporation could optimize the electronic structure of active centers. The catalytic performance was systematically evaluated using sulfamethoxazole (SMX) as a model pollutant, with particular emphasis on the optimization of Fe/Co ratio to achieve maximum synergistic effect. Comprehensive characterization techniques were employed to elucidate the structure-activity relationship, while single-factor experiments were conducted to assess the system’s adaptability to various environmental conditions. The degradation mechanism was investigated through reactive oxygen species (ROS) identification and electron paramagnetic resonance (EPR) analysis, supplemented by stability tests and interference studies to evaluate practical applicability. This study not only demonstrates an effective strategy for enhancing Fe-MOFs’ catalytic performance but also provides valuable insights into the design of efficient Fenton-like catalysts for environmental remediation, particularly in the treatment of pharmaceutical-containing wastewater under mild conditions.
Sulfamethoxazole (SMX), 2-Aminoterephthalic acid (2-NH2-BDC), tert-Butanol (TBA), p-Benzoquinone (pBQ), sodium chloride (NaCl), methanol (CH3OH), ethanol (C2H5OH), sodium bicarbonate (NaHCO3), and sodium nitrate (NaNO3) were procured from McLean Biochemicals Co., Ltd. N,N-Dimethylformamide (DMF), ferric chloride hexahydrate (FeCl3.6H2O), anhydrous sodium sulfate (Na2SO4), formic acid (HCOOH), and ρ-phthalic acid (H2BDC) were supplied by Aladdin Biochemical Technology Co., Ltd. Sinopharm Chemical Reagent Co., Ltd. Provided 30% hydrogen peroxide (H2O2), sodium hydroxide (NaOH), and nitric acid (HNO3). Cobalt nitrate hexahydrate (Co(NO3)2·6H2O) was obtained from Shanghai Acmec Biochemical Technology Co., Ltd. All chemicals and materials used in this study were analytical grade, except for methanol, which was superior purity.
The NH2-MOFs(Fe, Co) catalysts were prepared using a hydrothermal method, as illustrated in Figure 1. The detailed procedures were as follows.
1) Precursor preparation, dissolve 2.50 mmol of FeCl3·6H2O and Co(NO3)2·6H2O in a specific molar ratio dissolved in 30 mL DMF; add 1.25 mmol of 2-NH2-BDC to the solution; stir the mixture magnetically at 480 rpm for 35 min at 25°C until clear.
2) Hydrothermal reaction, transfer the clear solution to a 100 mL PTFE-lined stainless-steel autoclave; heat at 120°C for 20 h; cool to room temperature for 20 h.
3) Catalysts recovery and purification, recover the catalysts and wash three times with DMF, ethanol, and deionized water; centrifuge after each wash at 6,500 rpm for 4.5 min.
4) Dry the catalysts under vacuum at 110°C for 12 h.
To optimize the metal ratio, the total amount of FeCl3·6H2O and Co(NO3)2·6H2O was fixed at 2.50 mmol, with Fe:Co molar ratios of 9:1, 8:2, 7:3 and 6:4.
For comparison, MOFs(Fe) and MOFs(Fe, Co) were synthesis using the same method, substituting H2BDC for 2-NH2-BDC (Raw material compositions for diverse catalysts are shown in Supplementary Table S2).
1) scanning Electron Microscopy (SEM) by Gemini 300 thermal field emission SEM; 2) X-ray Diffraction (XRD) by D8 Advance diffractometer (Bruker), Cu-Kα radiation, 5°–25° 2θ range, 0.4°/min scan rate.
1) Fourier transform infrared (FTIR) spectra were obtained using a Nicolet 6700 FT-IR spectrometer with 4 cm-1 resolution and in range of 4,000–500 cm-1 at room temperature; 2) X-ray photoelectron spectroscopy (XPS) was analyzed by a Thermo Escalab 250XI (Thermo Fisher Scientific, USA) using monochromatic Al Kα radiation (hv = 1,486.6 eV) at 150 W power and a 650 µm beam spot; 3) Zeta potential at different pH values were determined by Malvern nanoparticle size potentiostat Zetasizer Pro (Zetasizer Nano ZS90, Malvern Panalytical, UK).
Impedance spectroscopy (EIS) was measured by an electrochemical analyzer (CHI760, Chenhua, China), 0.01 Hz to 100 kHz, 0.1 M Na2SO4 electrolyte, 12 mV with open-circuit voltage.
1) reactive oxidants (ROS) were analyzed by a Bruker A300-10/12 electron paramagnetic resonance technique (EPR, Germany). The radical trapping agent was 5,5-dimethyl-1-pyrroline-N-oxide (DMPO); 2). The concentration of SMX was determined by HPLC with a Waters, Arc-HPLC-2998 system. The analysis employed a SunFire@C18 column (4.6 × 250 mm, 5 μm) and a mobile phase consisting of methanol and 0.1% formic acid in a 70:30 volume ratio. The flow rate was set at 0.5 mL·min-1, with detection performed using a UV lamp of 269 nm and a temperature of 25°C. Intermediates were identified using liquid chromatography-mass spectrometry (LC-MS, AB Sciex Triple TOF®4,600). The pH of solutions was adjusted by 1 mM HNO3 and 0.1 mM NaOH. Unless otherwise specified, the Fe/Co ratios in both NH2-MOFs(Fe, Co) and MOFs(Fe, Co) were maintained at 7:3 throughout the experiments.
Fe and Co ion leaching after the initial cycling experiment was analyzed using AVIO 220 (PerkinElmer) inductively coupled plasma optical emission spectroscopy (ICP-OES).
The adsorption-catalyzed degradation experiments of SMX by NH2-MOFs(Fe, Co) was investigated at 25°C. The experiment began with 20 mL of the SMX (50 mg/L) diluted with 80 mL purified water in a 100 mL conical flask, achieving a final concentration of 10 mg/L. After 1 min of magnetic stirring at 380 rpm, a 2 mL sample was taken to determine the initial SMX concentration. The adsorption phase commenced upon adding certain concentrations of catalyst. After 30 min of adsorption of equilibrium, another 2 mL sample was collected. The catalyzed degradation was initialized by introducing H2O2. Subsequent samples were taken at 15, 30, 45, 60, 75, 90, 120, and 150 min. All samples were mixed with 200 μL of 0.5 mol/L sodium thiosulfate to quench the reaction, filtered through a 0.22 μm membrane to remove solid particles, and prepared for chromatographic analysis.
The catalyst’s reusability was assessed through multiple cycling experiments. After each use, the catalyst was recovered by filtration, washed with ultrapure water, and dried overnight at 60°C under vacuum conditions. The recovered catalyst was then reused in subsequent adsorption-catalyzed degradation experiments. This process was repeated several times to evaluate the catalyst’s longevity. To assess the structural and chemical changes induced by repeated use, the catalyst was analyzed before and after the reaction cycles using Fourier-transform infrared spectroscopy (FTIR), X-ray photoelectron spectroscopy (XPS), and X-ray diffraction (XRD) techniques.
Scanning Electron Microscopy (SEM) analyses revealed the morphological characteristics of the synthesized catalysts. MOFs(Fe) displayed a three-dimensional octahedral structure with diameter of approximately 1 μm (Figure 2a). The incorporation of Co atoms maintained overall morphology, the surface is concave inwards, and generally resulted in decreased particle size (Figure 2b). Amino-functionalized MOFs(Fe, Co) primarily preserved the three-dimensional octahedral structure, with a minor fraction exhibiting hexagonal micro-spindle morphology. This variation in geometric configuration suggested that ligand functionalization influenced the catalysts morphology (Figures 2c, d).
Figure 2. Structural and compositional analysis of MOF catalysts: (a) MOFs(Fe), (b) MOFs(Fe, Co) and (c, d) NH2-MOFs(Fe, Co). (e, f) XRD and FTIR patterns of MOFs(Fe), MOFs(Fe, Co) and NH2-MOFs(Fe, Co).
X-ray Diffraction (XRD) analysis provided insights into the crystalline structure of the synthesized catalysts (Figure 2e). MOFs(Fe) exhibited high crystallinity with distinct characteristic diffraction peaks at 2θ = 8.96°, 9.0°, 9.5°, 10.6°, and 16.5°, confirming successful synthesis of MOFs(Fe) and aligning with previous literature reports (Wu et al., 2020; Guo et al., 2019; Li et al., 2019; Gecgel et al., 2020). MOFs(Fe, Co) displayed a similar diffraction pattern to MOFs(Fe), indicating preservation of the overall structure during Co incorporation. However, slight peak shifts and intensity variations suggested successful Co doping into the MOFs(Fe) lattice.
NH2-MOFs(Fe, Co) exhibited distinct characteristic peaks at 2θ = 9.2°, 10.3°, 13°, 16.69°, and 18.5°, with significantly enhanced intensities in the 8°–10° and 16°–19° ranges due to -NH2 introduction. Compared to MOFs (Fe), peaks between 9 and 10°shifted right, while the 10.6°peak moved left, likely due to the incorporation of -NH2 groups and Co atoms. SEM electron micrographs corroborated the crystalline morphology of NH2-MOFs(Fe, Co), which showed similar alterations. Notably, NH2-MOFs(Fe, Co) demonstrated higher crystallinity than the other two materials (Figure 2e). Post-reaction XRD analysis revealed unchanged structures, confirming high catalyst stability and absence of significant structural disruption in NH2-MOFs(Fe, Co) materials.
Spectroscopic and elemental analyses of the synthesized MOF catalysts provided comprehensive characterization of their structural, compositional, and electronic properties. As shown in Figure 2f, FTIR spectra exhibited similar vibrational patterns across all samples. The absence distinct Co-O characteristic peaks after Co incorporation might be attributed to its relatively low content (He et al., 2022). Three critical spectral regions revealed essential structural information.
1) Hydroxyl Group Region (3,000–3,500 cm−1), the prominent absorption at 3,393 cm−1 corresponds to O-H stretching vibration (Yu et al., 2020), crucial for organic pollutants degradation in heterogeneous catalysis. Notably, -NH2 functionalization significantly enhanced this intensity in NH2-MOFs(Fe, Co), indicating increased surface hydroxyl groups on that provide additional reactive sites for SMX and H2O2 adsorption (Li et al., 2023). Complementary peaks at 3,458 cm−1 (asymmetric stretching) and 3,334 cm−1 (symmetric stretching) further confirmed amino group incorporation (Mohammadnezhad et al., 2021).
2) Characteristic vibrations at 1,619 cm−1 (N-H bending) and 1,256 cm−1 (C-N stretching) verified successful -NH2 grafting. The 1,577 cm−1 peak (C=O stretching) combined with bands at 1,423 cm−1 (symmetric O-H) and 1,382 cm−1 (asymmetric O-H) confirmed dicarboxylic acid ligands in the MOF architecture (Wu et al., 2021).
3) Metal-Oxygen Coordination Region (<800 cm−1): The 768 cm−1 absorption originated from C-H bending in benzene rings, while the 553 cm−1 feature confirmed Fe-O cluster formation in the crystalline framework (Fang et al., 2023).
X-ray Photoelectron Spectroscopy (XPS) offered detailed information on elemental compositions and electronic states. The incorporation of Co caused a shift of the Fe 2p orbital valence peaks, confirming the formation of bimetallic active center (Supplementary Figure S1) (Liang et al., 2021a). Strong N 1s signals in NH2-MOFs(Fe, Co) verified the successful incorporation of amino groups. Shifts in binding energies of C 1s, O 1s, and Fe 2p peaks further validated the synthesis of NH2-MOFs(Fe, Co). Notably, the introduction of Co and -NH2 groups enhanced electron transfer from ligands to metal centers, as evidenced by shifts in binding energies of the C 1s, O 1s, and Fe 2p peaks to varying extents.
High-resolution XPS spectra provided detailed insights into the electronic structure and chemical composition of NH2-MOFs(Fe, Co) catalysts before and after reaction. The C 1s spectrum revealed three distinct peaks corresponding to C=C/C-C, C-N, and C-O bonds (Figure 3a) (Zhou and Wang, 2023). Post-reaction changes in peak intensities indicated active involvement of carbon species in the catalytic process. After the reaction, the relative intensities of these peaks changed by 16.4%, 11.3%, and 5.1%, respectively. The N 1s spectrum (Figure 3b) demonstrated significant chemical transformation in NH2-MOF (Fe, Co.) during the Fenton reaction. Prior to the reaction, two distinct nitrogen species were identified: a C-NH configuration at 399.08 eV (32.5%) and a C=N-C at 400.14 eV (67.5%). Post-reaction analysis revealed a marked reduction in the contents of both components (C-NH: 32.5% → 28.1%; C=N-C: 67.5% → 65.1%), indicative of structural degradation or chemical modification. Notably, a new N-O species emerged at 402.67 eV (6.8%), confirming the oxidation of amino group and the formation of nitrogen-oxygen compounds. This evolution directly implies amino group participation in the Fenton reaction mechanism through electron transfer processes (Zeng et al., 2017). The O 1s spectrum (Figure 3c) displayed peaks corresponding to metal oxides, carboxylate C-O bonds, and O-H bonds. A reduction in metal-oxygen bond content after reaction indicated electron transfer from O2- to Fe (II) during oxidation.
Figure 3. XPS and EIS Analysis of NH2-MOFs(Fe, Co): (a) C 1s (b) N 1s (c) O 1s (d) Fe 2p (e) Co. 2p (f) survey scan and (g) EIS Nyquist plots of MOFs(Fe), MOFs(Fe, Co) and NH2-MOFs(Fe, Co).
Analysis of the Fe 2p orbital revealed peaks corresponding to Fe2+ and Fe3+ in both Fe 2p3/2 and Fe 2p1/2 regions. The stable distribution of Fe species before and after reaction demonstrated the redox stability of iron in the catalyst. Notably, the introduction of -NH2 groups caused a red-shift in Fe-O/Co-O peak binding energies (Supplementary Figure S1) and a slight shift of Fe 2p orbital valence peaks towards lower binding energies (Liang et al., 2021b). These shifts suggest that -NH2 functionalization alters the material’s electronic structure, enhancing electron transfer from the ligand to the central metal (Li et al., 2017).
The Co 2p spectrum (Figure 3e) showed two characteristic peaks attributed to Co3+ (780.7 eV and 789.3 eV) and Co2+ (783.1 eV and 792.6 eV), with a better cycling state of Co species before and after the reaction.
Additionally, EIS results demonstrated that the incorporation of dual reaction centers and -NH2 enhanced the material’s interfacial electron transfer capacity (Figure 3g).
These results collectively provide valuable insights into the electronic properties and reaction mechanisms of NH2-MOFs(Fe, Co) catalysts, highlighting the role of amino functionalization in modifying the catalyst’s electronic structure and potentially enhancing its catalytic performance.
A series of experiments were conducted to evaluate the performance of bimetallic NH2-MOFs(Fe, Co) as Fenton-like catalysts for SMX degradation (Test method for the concentration of SMX is given in Supplementary Text S1). The study revealed that adsorption played a minimal role, with less than 5% of SMX being removed across all metal ratios (Figure 4a). Similarly, H2O2 alone proved ineffective in degrading SMX, due to its relatively low oxidation potential (1.776 V) (Li et al., 2024; Ramos et al., 2021).
Figure 4. Degradation curves of SMX in different reaction systems (a) and the corresponding kinetic curves (b). The effect of (c) pH value for SMX degradation in the NH2-MOFs(Fe, Co)/H2O2 system. (d) The Zeta potential of NH2-MOFs(Fe, Co) under different pH value. The effect of (e) catalyst dosage, (f) concentration of SMX, and (g) concentration of H2O2 for SMX degradation in the NH2-MOFs(Fe, Co)/H2O2 system (Reaction conditions: natural pH value (ca. 5.4 ± 0.1), initial SMX concentration: 10 mg/L, H2O2 concentration: 1mM, and catalyst dosage: 0.3 g/L. where X represents the ratio of iron.).
Comparative analysis of MOFs(Fe) and MOFs(Fe, Co) under identical conditions demonstrated the significant impact of Co incorporation on catalytic performance. While MOFs(Fe) achieved 63.1% removal of SMX after 150 min, MOFs(Fe, Co) removed 87% of SMX after 120 min and completely removal within 150 min. This enhanced performance is attributed to the synergistic interaction between Fe and Co within the catalyst structure. The introduction of Co into MOFs(Fe) optimizes the electronic structure, lower the energy barrier for H2O2 activation, and promotes increased hydroxyl radicals (⋅OH). These factors contribute to more efficient SMX degradation (Liang et al., 2021a). Furthermore, Co can undergo redox reaction with H2O2 like Fe, further enhancing the catalytic process (Equation 1).
The introduction of -NH2 greatly to MOFs(Fe, Co) catalyst resulted in a remarkable improvement in their performance for SMX degradation. Comparative studies revealed that while MOFs(Fe, Co) removed only 33.5% of SMX in 75 min, the amino-functionalized NH2-MOFs(Fe, Co) achieved completely removal in same timeframe. Furthermore, in the presence of H2O2, NH2-MOFs(Fe, Co)x were all able to completely degrade SMX within 150 min. This significant enhancement in catalytic activity can be attributed to several factors. Firstly, the amino group facilitates accelerated electron transfer within the catalyst structure, promoting faster valence transitions of the central metal atoms. Secondly, it increases the electron density around the metal centers, enabling continuous and efficient production of hydroxyl radicals (⋅OH) (Chen et al., 2023). Furthermore, the incorporation of Co and -NH2 may facilitate the formation of Lewis acid sites, thereby providing more active sites for H2O2 activation (Liang et al., 2021b; Huang et al., 2022).
The Fe: Co ratio in metal-organic framework (MOF) catalysts significantly influences their performance in SMX degradation. Adjusting the ratio from 9:1 to 7:3 increased the SMX degradation rate from 91.8% to 94.5%, demonstrating a synergistic effect between Fe and Co However, further increasing the Co content to a 6:4 ratio led to a substantial decrease in catalytic performance, with only 54.2% SMX degradation at 60 min. This decline in performance with excessive Co doping can be attributed to two main factors: 1) excessive Co2+ occupying the active sites belonging to Fe2+, inhibiting the catalyst’s activity (Li et al., 2024); 2) high cobalt content could potentially compromise the material’s structural stability.
The catalytic efficiency evaluation revealed a clear performance hierarchy among the tested MOF catalysts in Fenton-like reactions: MOFs(Fe) < MOFs(Fe, Co) < NH2-MOFs(Fe, Co). This progression underscores the synergistic enhancement from bimetallic composition and amino functionalization. The NH2-MOFs (Fe, Co)/H2O2 system showed greater superiority over the existing catalysts (Supplementary Table S3). First, it achieved complete degradation of 10 mg/L SMX within 75 min under natural pH (5.4), surpassing MIL-53(Fe) (96% removal of 200 μg/L SMX at pH 4 with UV assistance) (Ortega-Moreno et al., 2022), CUCs-MIL-88 B-Fe/Ti3C2 (96% removal of 30 mg/L SMX requiring visible light) (Ahmad et al., 2020), and Fe@MesoC (100% removal of 20 mg/L SMX at pH 4) (Tang and Wang, 2018). Second, operational efficiency was optimized through reduced reagent demands—0.3 g/L catalyst dosage and 1 mM H2O2 concentration, significantly lower than literature values (200–500 mg/L catalyst, 2–10 mM H2O2) (Tang and Wang, 2020) while eliminating energy-intensive UV/visible light requirements. Third, the system demonstrated exceptional environmental adaptability by maintaining efficacy without pH adjustment, contrasting sharply with conventional Fenton systems constrained to acidic conditions (pH 2.8–3.5). These advancements position NH2-MOFs(Fe, Co) as a practical solution for wastewater remediation. Subsequent research prioritized two key aspects: rigorous stability evaluation through multi-cycle testing and mechanistic investigation via radical trapping/LC-MS analysis, establishing a foundation for industrial implementation.
The degradation kinetics of SMX were evaluated using pseudo-primary rate constants, calculated according to Equation 2. Figure 4b presents a comparative analysis of the kinetics across different reaction systems, offering insights into their relative efficiencies. Among the systems studied, the NH2-MOFs(Fe, Co)/H2O2 system exhibited the highest rate constant (k-value) of 0.0479 min-1, significantly outperforming the other ones. The observed enhancement can be attributed to several factors. Firstly, the amino group accelerates the cycling of the central metal, facilitating more efficient catalytic turnover. Secondly, it alters the electronic structure of the catalyst, potentially creating more favorable conditions for the degradation reaction. Lastly, the -NH2 group promotes more effective activation of H2O2, a crucial component in the degradation process
where Ct (mg/L) is the concentration of SMX at a certain reaction time t (min), and C0 (mg/L) refers to the concentration of SMX after the adsorption-desorption equilibrium is reached and before oxidative degradation begins.
The effectiveness of Fenton-like systems in degrading organic pollutants is heavily influenced by several key parameters, including initial pH, catalyst amount, initial pollutant concentration, and H2O2 content (Dapaah et al., 2022). To optimize these factors for SMX degradation, we conducted a series of controlled experiments using simulated wastewater, with results presented in Figures 4c–f.
As shown in Figure 4c, the initial pH plays a crucial role in the degradation process, though it has minimal impact on SMX adsorption by NH2-MOFs(Fe, Co). The system demonstrated optimal performance in the pH range of 3.0–5.4, achieving complete SMX removal within 75 min. Efficiency decreased at a higher pH level, primarily due to the formation and decomposition of hydroxides inhibiting iron species reactivity, as well as reduced H2O2 availability and its self-decomposition of H2O2 (Tang and Wang, 2019). Zeta potential analysis revealed a point of zero charge (pHPZC) 4.8 for NH2-MOFs(Fe, Co), indicating a negatively charged catalyst surface above this pH and a positively charged surface below it (Figure 4d). The ionization state of SMX also varies with pH, existing in an amphipathic form (SMX±) between pH 1.97 and 6.16, and as an anionic species (SMX-) above pH 6.16 (Brillas et al., 2009). Notably, even at a pH of 9, the system achieved nearly 90% SMX removal after 120 min reaction, significantly outperforming the typical optimal pH range (2.8–3.5) for conventional Fenton reactions (Milh et al., 2020). The reduced SMX degradation efficiency under alkaline conditions (pH > 8) likely arises from electrostatic repulsion between the negatively charged surface and deprotonated SMX molecules (pKa = 5.7), as a evidenced by the surface charge reversal observed in zeta potential measurements.
Based on these results, we selected the unadjusted initial pH of 5.4 for subsequent optimization studies, as it demonstrated excellent performance with complete SMX removal within 75 min. This pH level strikes a balance between optimal catalytic activity and practical applicability, potentially reducing the need for pH adjustment in real-world applications.
Figure 4e presents the experimental results of initial catalyst concentration (0.1–0.4 g/L) on pollutant removal. Notably, all tested concentrations achieved near-complete SMX degradation within 120 min, highlighting the system’s robustness across a range of catalyst loading. The study revealed a nuanced relationship between catalyst concentration and degradation efficiency. While the removal rate at 90 min initially increased with higher catalyst concentrations, this trend showed diminishing returns at higher levels. A catalyst concentration of 0.3 g/L emerged as the optimal point, achieving approximately 95% SMX removal, which was comparable to the performance at 0.4 g/L. Interestingly, while the catalyst concentration had a minimal effect on adsorption performance, it demonstrated a positive correlation with catalytic degradation efficiency. This relationship can be attributed to the increased availability of active sites at higher concentrations, which accelerates the production of OH radicals during SMX degradation, as noted by Guo et al. (2020). Therefore, a catalyst concentration of 0.3 g/L was selected as the condition for the subsequent experiments.
The impact of SMX concentration on the catalytic degradation efficiency was investigated (Figure 4f). The results revealed an inverse relationship between SMX concentration and removal efficiency. This phenomenon can be attributed to reduced contact efficiency between the catalyst’s active sites and SMX molecules at higher concentrations, as suggested by Li et al. (2024).
Figure 4g demonstrates the effect of H2O2 concentration on SMX degradation efficiency, showing a non-linear relationship. As the H2O2 concentration increased from 0.5 mM to 1 mM, the SMX removal rate increased. However, further increase in H2O2 concentration led to a gradual decrease in removal efficiency. This trend can be explained by the dual role of H2O2 in the process. At appropriate concentrations, H2O2 enhances the production of free radicals, improving SMX degradation. Conversely, excessive H2O2 can reduce Fenton-like activity and even quench ⋅OH radical, as described by Equations 3 and 4 (Yang et al., 2021). These findings highlight the importance of optimizing H2O2 dosage to balance treatment effectiveness and cost efficiency.
Based on the above results and cost-saving, the optimal conditions for SMX degradation were determined as follows: initial SMX concentration of 10 mg/L, NH2-MOFs(Fe, Co) concentration of 0.3 g/L, H2O2 concentration of 1 mM and an operation pH at 5.4.
The practical applicability of the NH2-MOFs(Fe, Co) catalyst was assessed through reusability tests and stability analyses. Consecutive cycling tests for SMX removal were conducted under consistent conditions (Initial SMX concentration: 10 mg/L, concentration of NH2-MOFs(Fe, Co): 0.3 g/L, H2O2 concentration: 1mM, pH = 5.4). Figure 5a illustrates the catalyst’s reusability performance. NH2-MOFs(Fe, Co) maintained stable catalytic efficiency though the first two cycles. A slight decrease in performance was observed in the third cycle, the catalyst activity stabilised and did not decrease during the cycle. This pattern indicates robust and sustainable catalytic activity over multiple uses.
Figure 5. (a) Recyclability of NH2-MOFs(Fe, Co) in Fenton-like degradation of SMX, (b) total metal content and 90 min dissolution. Experiment conditions: natural pH (ca.5.4 ± 0.1), initial SMX concentration:10 mg/L, H2O2 concentration: 1mM, catalyst dosage: 0.3 g/L, and temperature 25°C.
The stability of the catalyst was further confirmed by metal leaching tests and post-reaction analyses. As shown in Figure 5b, less than 10% metal leaching occurred after 90 min of reaction, suggesting high structural integrity of NH2-MOFs(Fe, Co). Moreover, XRD, FTIR, and XPS analyses of the used catalyst revealed no significant changes in its internal chemical bonding or electronic structure.
The presence of inorganic anions in natural waters can significantly influence H2O2 activation and organic pollutants removal (Wang et al., 2021; Su et al., 2022). This study examined the effects of common anions (Cl−, HCO3−, NO3−, and SO42-) on SMX removal in the NH2-MOFs(Fe, Co.)/H2O2 system. Cl− exhibited a concentration-dependent inhibitory effect on SMX removal within the 1–10 mM (Figure 6a). Despite this, the catalyst maintained high activity, achieving complete SMX degradation within 120 min even at 10 mM Cl−. This inhibition is attributed to Cl−—as a radical scavenger, forming less reactive Cl·, Cl2·, and HClO·-—upon reaction with ⋅OH (Goulart et al., 2021).
Figure 6. The effect of (a) Cl−,HCO3−; (b) NO3−, SO42- of NH2-MOFs(Fe, Co) in Fenton-like degradation of SMX. Reaction conditions: natural pH = 5.4 ± 0.1, initial SMX concentration: 10 mg/L, H2O2 concentration: 1mM, catalyst dosage: 0.3 g/L, and temperature 25°C.
HCO3− demonstrated a more pronounced inhibitory effect (Figure 6a). At just 1 mM HCO3−, SMX removal rate dropped below 5%. This strong inhibition results from HCO3− reacting with ⋅OH to form less effective radical species (Lin et al., 2019).
NO3− and SO42- showed contrasting effects (Figure 6b). NO3− exhibited a consistent inhibitory effect across the 1–10 mM range, though complete SMX removal was still achieved within 90 min. Conversely, SO42- promoted SMX degradation, with increasing effectiveness up to 5 mM concentration, further increases in SO42- concentration did not significantly enhance system performance.
In Fenton-like systems utilizing MOF materials, the activation of H2O2 primarily generates two reactive oxygen species (ROS): ⋅OH and ⋅O2−. Among these, ⋅OH is generally considered the principle active species responsible for pollutant degradation (Li et al., 2023). To elucidate the roles of these ROS in our NH2-MOFs(Fe, Co)/H2O2 system, tert-butanol (TBA) and p-benzoquinone (pBQ) were employed as specific scavengers to quench ⋅OH and ⋅O2− respectively. As shown in Figure 7a, the addition of 1 mM TBA reduced SMX rate, though completely degradation was still achieved within 120 min. Increasing TBA concentration to 10 mM significantly inhibited SMX degradation, with only 5% removal after 150 min. In contrast, the addition of 10 mM pBQ allowed for nearly 70% SMX degradation within 150 min. These results clearly demonstrate that ⋅OH is the primary active radical responsible for SMX elimination in this system.
Figure 7. Radical scavenging experiments and EPR analysis of the NH2-MOFs(Fe, Co)/H2O2 system for SMX degradation. (a) Effects of different concentrations of trapping agents TBA and pBQ on the degradation of SMX simulated wastewater by NH2-MOFs(Fe, Co)/H2O2 system; (b) Effect of catalysts and oxidizers on SMX degradation; (c) DMPO-⋅OH spin-trapping EPR spectra; (d) DMPO-⋅O2− spin-trapping EPR spectra. Reaction conditions: natural pH = 5.4 ± 0.1, initial SMX concentration:10 mg/L, H2O2 concentration: 1mM, catalyst dosage: 0.3 g/L, and temperature 25°C).
The synergistic action of the catalyst and oxidant in SMX degradation was demonstrated through control experiments and Electron Paramagnetic Resonance (EPR) spectroscopy (Specific test conditions are shown in Supplementary Text S2). Figure 7b shows that neither H2O2 nor the catalyst alone significantly degraded SMX, confirming that the degradation process relies on reactive radicals generated through their combined action.
EPR analysis was employed to identify and quantify the active species produced during H2O2 activation. DMPO (5,5-dimethyl-1-pyrroline N-oxide) was used as a spin-trapping agent for both hydroxyl (⋅OH) and superoxide (⋅O2−) radicals. As shown in Figure 7c, the characteristic 1:2:2:1 peak pattern of DMPO-⋅OH adducts was observed, with strong signal intensity maintained at 10 and 20 min. This aligns with the SMX degradation trends observed in previous experiments. Additionally, DMPO-⋅O2− signals were detected (Figure 7d), indicating the presence of superoxide radicals. Notably, the introduction of the -NH2 group in the MOF structure enhanced ⋅O2− production, potentially leading to increased generation of reactive radicals. This observation is consistent with previous studies (Huang et al., 2022) and suggests that the amino functionalization of the MOF plays a crucial role in the system’s catalytic efficiency.
Based on the above results, a rational mechanism for the Fenton-like NH2-MOFs(Fe, Co) reaction could be proposed (As shown in Figure 8). The catalyst’s dual active metal sites, Fe and Co, undergo valence changes between Fe (III)/Co (III) to Fe (II)/Co (II) states (Equations 5, 6) (Liang et al., 2021a). This process facilitates the activation of adsorbed H2O2, generating ⋅OH and ⋅O2− radicals through Fenton-like reactions (Equations 7, 8).
Figure 8. Schematic diagram of the proposed mechanisms involved for SMX degradation in NH2-MOFs(Fe, Co)/H2O2 system.
The efficiency of this process is enhanced by several factors: 1) π-bonds between SMX and NH2-MOFs(Fe, Co) improve the pollutant’s attachment to the catalyst surface (Cui et al., 2021), promoting reactions with surface-bound radicals (Liu et al., 2021; Xiao et al., 2023); 2) the electron-rich -NH2 group reduces the electron density of the intermediate metal, accelerating both the Fe (III)/Co (III) to Fe (II)/Co (II) transition and H2O2 decomposition; 3) XPS data confirm stable cycling of the central metals within NH2-MOFs(Fe, Co), with Fe (II) and Co (III) ratios remaining relatively constant post-reaction (Figure 3). The continuous electron extraction from NH2-MOFs(Fe, Co) by H2O2 produce sustains ⋅OH production. Subsequently, the combined action of ⋅OH and ⋅O2− radicals decomposes intermediates into CO2 and H2O (Huang et al., 2023).
To elucidate the degradation mechanism of SMX in the NH2-MOFs(Fe, Co)/H2O2 system, the formed intermediates were characterized using TOF (Analysis parameters are shown in Supplementary Text S3). Ten primary intermediates (N1-N10) were detected and characterized (Supplementary Table S4). The potential decomposition pathways of SMX were proposed based on the reaction properties of ⋅OH and ⋅O2− radicals, as illustrated in Figure 9. The strong redox capacity ⋅OH/⋅O2− primary targets the S-C and S-N bonds in SMX (Wang and Wang, 2017). S-C bond cleavage leads to the formation of N1 (m/z = 129) and N2 (m/z = 163.04), while S-N bond breakage produces N4 (m/z = 99.0553). These observations align with previous studies by Wang and Wang. (2017), Milh et al. (2020), and Liu et al. (2017) (Wang and Wang, 2017; Milh et al., 2020; Liu et al., 2017). N2 can further generate N3 (m/z = 183.08) by binding H+ from water and the same reaction has been reported in previous studies (Li T. et al., 2021). Hydroxylation of benzene and isoxazole rings by ⋅OH attack results in N5 and N6 (m/z = 270), influenced by the electron-donating effect of -NH2 and electron-withdrawing effect of -S(O2)-NH- groups, as noted by Ribeiro (Ribeiro et al., 2016). Further transformations include demethylation and hydroxylation leading to N8 (m/z = 256), and oxidative decarboxylation converting N6 to N7 (m/z = 173.0878), consistent with findings by Chen (Chen et al., 2023). The formation of nitro derivatives (N9, m/z = 284) occirs through ⋅OH radical attack on the -NH2 group, forming hydroxylamine and subsequently nitroso derivatives, as described by Milh (Milh et al., 2021). Additionally, isoxazole ring (N10, m/z = 304) opening followed by oxidation/decarboxylation or sulfonamide bond breakage leads to the formation of N7 (m/z = 173.0878), aligning with previous studies on H2O2 oxidation catalyzed by functionalized iron MOFs (Chen et al., 2023).
This study presents a significant advance in the development of Fenton-like catalysts by successfully combining bimetallic synergy with amino functionalization in MOF materials. Although this study has established the fundamental correlation between structural modifications and enhanced performance, several aspects warrant further investigation: (1) in-situ characterization of the electron transfer process between metal centers and amino groups; (2) molecular-level understanding of the Co-Fe synergistic mechanism; and (3) catalyst behavior in complex environmental matrices. Future research should focus on scaling up synthesis procedures, evaluating performance in real pharmaceutical wastewater treatment, and developing strategies for catalyst regeneration and recycling. Additionally, the catalytic performance and structural stability of NH2-MOFs(Fe, Co) exhibited strong dependence on vacuum drying temperatures during synthesis. Systematic optimization revealed elevated temperatures enhanced crystallinity and framework integrity, with 110°C identified as the optimal condition for balancing thermal stability and active site preservation.
The insights gained from this study not only contribute to the rational design of efficient MOF-based catalysts but also offer promising solutions for sustainable wastewater treatment, particularly in addressing emerging pharmaceutical pollutants under environmentally relevant conditions.
In this study, we successfully developed an amino-functionalized bimetallic MOF catalyst [NH2-MOF(Fe, Co)] through a facile hydrothermal synthesis method for enhanced Fenton-like degradation of sulfamethoxazole (SMX) pollutants. The optimized catalyst demonstrated remarkable advantages over conventional Fe-MOFs in SMX removal, achieving 95% degradation within 120 min under neutral pH conditions (0.3 g/L catalyst, 1 mM H2O2). Notably, the catalytic efficiency and kinetic rate constant were enhanced by 3.2 and 43.5 times, respectively, compared to the Fe-MOF/H2O2 system at natural pH, addressing a critical limitation of traditional Fenton processes.
Systematic investigation of operational parameters revealed the catalyst’s robust performance across a wide pH range and excellent stability over multiple cycles. The superior catalytic activity can be attributed to two synergistic mechanisms: (1) the cooperative interaction between Fe and Co creating dual active sites for enhanced hydroxyl radical generation, and (2) the electron-donating effect of amino groups facilitating rapid Fe (III)/Fe (II) cycling and efficient H2O2 activation. Mechanistic studies through intermediate identification elucidated the degradation pathways, confirming the crucial role of -NH2 functionalization in accelerating electron transfer and sustaining radical production.
This work provides valuable insights into the rational design of efficient MOF-based Fenton-like catalysts through dual modification strategies. The wide pH adaptability and excellent stability of NH2-MOF(Fe, Co) make it particularly promising for practical wastewater treatment applications. Future research should focus on scaling up synthesis procedures, evaluating performance in complex environmental matrices, and developing strategies for catalyst regeneration and recycling. These findings contribute significantly to advancing sustainable solutions for pharmaceutical pollution remediation through advanced oxidation processes.
The raw data supporting the conclusions of this article will be made available by the authors, without undue reservation.
XZ: Conceptualization, Data curation, Methodology, Validation, Writing–original draft. YL: Formal Analysis, Software, Writing–original draft. JY: Conceptualization, Methodology, Supervision, Writing–original draft.
The author(s) declare that financial support was received for the research, authorship, and/or publication of this article. This work was supported by Foundation for Young Talent Innovation in Higher Education of Guangdong, China [grant number 6022210124K], Post-doctoral Later-stage Foundation Project of Shenzhen [grant number 6022271006K] and Shenzhen Polytechnic University Lihu Elite Talent Project (LHRC20230405-2023-2025).
The authors declare that the research was conducted in the absence of any commercial or financial relationships that could be construed as a potential conflict of interest.
The author(s) declare that no Generative AI was used in the creation of this manuscript.
All claims expressed in this article are solely those of the authors and do not necessarily represent those of their affiliated organizations, or those of the publisher, the editors and the reviewers. Any product that may be evaluated in this article, or claim that may be made by its manufacturer, is not guaranteed or endorsed by the publisher.
The Supplementary Material for this article can be found online at: https://www.frontiersin.org/articles/10.3389/fchem.2025.1579108/full#supplementary-material
Ahmad, M., Quan, X., Chen, S., and Yu, H. (2020). Tuning Lewis acidity of MIL-88B-Fe with mix-valence coordinatively unsaturated iron centers on ultrathin Ti3C2 nanosheets for efficient photo-Fenton reaction. Appl. Catal. B Environ. 264, 118534. doi:10.1016/j.apcatb.2019.118534
Brillas, E., Sires, I., and Oturan, M. A. (2009). Electro-fenton process and related electrochemical technologies based on fenton's reaction chemistry. Chem. Rev. 109, 6570–6631. doi:10.1021/cr900136g
Chen, H., Guo, P., Huang, Z., Sun, J., Lei, Y., and Xu, J. (2024). Enhanced stability and conductivity of montmorillonite and sucrose loaded Fe-MOFs for degradation of chlortetracycline hydrochloride via electrochemically activated persulfate. Appl. Clay Sci. 249, 107231. doi:10.1016/j.clay.2023.107231
Chen, J., Qin, C., Mou, Y., Cao, Y., Chen, H., Yuan, X., et al. (2023). Linker regulation of iron-based MOFs for highly effective Fenton-like degradation of refractory organic contaminants. Chem. Eng. J. 459, 141588. doi:10.1016/j.cej.2023.141588
Cui, L., Li, Q., Chen, B., Qiu, J., Jing, W., and Gu, X. (2021). Metal-semiconductor electron-rich interface governs the enhanced activity of spinel ferrite toward heterogeneous electro-Fenton process. Appl. Surf. Sci. 567, 150874. doi:10.1016/j.apsusc.2021.150874
Dapaah, M. F., Niu, Q., Yu, Y.-Y., You, T., Liu, B., and Cheng, L. (2022). Efficient persistent organic pollutant removal in water using MIL-metal-organic framework driven Fenton-like reactions: a critical review. Chem. Eng. J. 431, 134182. doi:10.1016/j.cej.2021.134182
Da Silva Rodrigues, D. A., Rodrigues Ferreira da Cunha, C. C., Freitas, M. G., Correa de Barros, A. L., Neves e Castro, P. B., Pereira, A. R., et al. (2020). Biodegradation of sulfamethoxazole by microalgae-bacteria consortium in wastewater treatment plant effluents. Sci. Total Environ. 749, 141441. doi:10.1016/j.scitotenv.2020.141441
Du, X., Wang, S., Ye, F., and Zhang, Q. (2022). Derivatives of metal-organic frameworks for heterogeneous Fenton-like processes: from preparation to performance and mechanisms in wastewater purification - a mini review. Environ. Res. 206, 112414. doi:10.1016/j.envres.2021.112414
Fang, Z., Liu, Y., Qi, J., Xu, Z.-F., Qi, T., and Wang, L. (2023). Establishing a high-speed electron transfer channel via CuS/MIL-Fe heterojunction catalyst for photo-Fenton degradation of acetaminophen. Appl. Catal. B Environ. 320, 121979. doi:10.1016/j.apcatb.2022.121979
Feng, Z., Yang, J., Zhu, L., and Sun, T. (2023). Bromine functionalized Fe/Cu bimetallic MOFs for accelerating Fe (III)/Fe (II) cycle and efficient degradation of phenol in Fenton-like system. Colloids Surf. A 658, 130701. doi:10.1016/j.colsurfa.2022.130701
Gecgel, C., Simsek, U. B., Turabik, M., and Ozdemir, S. (2020). Synthesis of titanium doped iron based metal-organic frameworks and investigation of their biological activities. J. Inorg. Organomet. Polym. Mater. 30, 749–757. doi:10.1007/s10904-019-01329-3
Goulart, L. A., Moratalla, A., Lanza, M. R. V., Saez, C., and Rodrigo, M. A. (2021). Photocatalytic performance of Ti/MMO/ZnO at degradation of levofloxacin: effect of pH and chloride anions. J. Electroanal. Chem. 880, 114894. doi:10.1016/j.jelechem.2020.114894
Guo, B., Xu, T., Zhang, L., and Li, S. (2020). A heterogeneous Fenton-like system with green iron nanoparticles for the removal of bisphenol A: performance, kinetics and transformation mechanism. J. Environ. Manage. 272, 111047. doi:10.1016/j.jenvman.2020.111047
Guo, H., Guo, W., Liu, Y., and Ren, X. (2019). Quinone-modified metal-organic frameworks MIL-101(Fe) as heterogeneous catalysts of persulfate activation for degradation of aqueous organic pollutants. Water Sci. Technol. 79, 2357–2365. doi:10.2166/wst.2019.239
He, Y., Li, H., Wu, J., Liu, Z., Chen, Y., Guo, W., et al. (2022). In-situ formation of Au nanoparticles with surface plasmon resonance confined in the framework of Cu ions doped NH2-MIL-125 (Ti) to enhance photocatalytic hydrogen production and NO removal. Appl. Surf. Sci. 604, 154641. doi:10.1016/j.apsusc.2022.154641
Huang, P., Chang, Q., Jiang, G., Xiao, K., and Wang, X. (2023). MIL-101(Fe3II, Mn) with dual-reaction center as Fenton-like catalyst for highly efficient peroxide activation and phenol degradation. Sep. Purif. Technol. 306, 122582. doi:10.1016/j.seppur.2022.122582
Huang, P., Yao, L., Chang, Q., Sha, Y., Jiang, G., Zhang, S., et al. (2022). Room-temperature preparation of highly efficient NH2-MIL-101 (Fe) catalyst: the important role of -NH2 in accelerating Fe (III)/Fe (II) cycling. Chemosphere 291, 133026. doi:10.1016/j.chemosphere.2021.133026
Huang, Y., and Yang, J. W. (2021). Degradation of sulfamethoxazole by the heterogeneous Fenton-like reaction between gallic acid and ferrihydrite. Ecotoxicol. Environ. Saf. 226, 112847. doi:10.1016/j.ecoenv.2021.112847
Ikehata, K., Gamal El-Din, M., and Snyder, S. A. (2008). Ozonation and advanced oxidation treatment of emerging organic pollutants in water and wastewater. Ozone Sci. Eng. 30, 21–26. doi:10.1080/01919510701728970
Kang, A. J., Brown, A. K., Wong, C. S., and Yuan, Q. (2018). Removal of antibiotic sulfamethoxazole by anoxic/anaerobic/oxic granular and suspended activated sludge processes. Bioresour. Technol. 251, 151–157. doi:10.1016/j.biortech.2017.12.021
Kaur, B., Soni, V., Kumar, R., Singh, P., Selvasembian, R., Singh, A., et al. (2024). Recent advances in manipulating strategies of NH2-functionalized metallic organic frameworks-based heterojunction photocatalysts for the sustainable mitigation of various pollutants. Environ. Res. 259, 119575. doi:10.1016/j.envres.2024.119575
Ke, Q., Shi, Y., Liu, Y., Chen, F., Wang, H., Wu, X.-L., et al. (2019). Enhanced catalytic degradation of bisphenol A by hemin-MOFs supported on boron nitride via the photo-assisted heterogeneous activation of persulfate. Sep. Purif. Technol. 229, 115822. doi:10.1016/j.seppur.2019.115822
Kulandaivel, T., Rasappan, A. S., Savunthari, K. V., Samuel, M. S., Kumar, M., Dahms, H.-U., et al. (2024). Facile fabrication of amorphous Al/Fe based metal-organic framework as effective heterogeneous Fenton catalyst for environmental remediation. Environ. Geochem Health 46, 156. doi:10.1007/s10653-024-01940-5
Li, N., He, X., Ye, J., Dai, H., Peng, W., Cheng, Z., et al. (2023). H2O2 activation and contaminants removal in heterogeneous Fenton-like systems. J. Hazard. Mater. 458, 131926. doi:10.1016/j.jhazmat.2023.131926
Li, S., Shan, S., Chen, S., Li, H., Li, Z., Liang, Y., et al. (2021). Photocatalytic degradation of hazardous organic pollutants in water by Fe-MOFs and their composites: a review. J. Environ. Chem. Eng. 2021, 105967. doi:10.1016/j.jece.2021.105967
Li, T., Ge, L., Peng, X., Wang, W., and Zhang, W. (2021). Enhanced degradation of sulfamethoxazole by a novel Fenton-like system with significantly reduced consumption of H2O2 activated by g-C3N4/MgO composite. Water Res. 190, 116777. doi:10.1016/j.watres.2020.116777
Li, X., Pi, Y., Wu, L., Xia, Q., Wu, J., Li, Z., et al. (2017). Facilitation of the visible light-induced Fenton-like excitation of H2O2 via heterojunction of g-C3N4/NH2-Iron terephthalate metal-organic framework for MB degradation. Appl. Catal. B Environ. 202, 653–663. doi:10.1016/j.apcatb.2016.09.073
Li, Y., Ren, D., Guo, S., Wang, M., Zhai, J., Zhang, S., et al. (2024). Preparation of nickel-doped iron-based bimetallic organic framework carbonized derivatives for heterogeneous catalytic hydrogen peroxide degradation of 4-NP. J. Mol. Struct. 1299, 137058. doi:10.1016/j.molstruc.2023.137058
Li, Z., Liu, X., Jin, W., Hu, Q., and Zhao, Y. (2019). Adsorption behavior of arsenicals on MIL-101(Fe): the role of arsenic chemical structures. J. Colloid Interface Sci. 554, 692–704. doi:10.1016/j.jcis.2019.07.046
Liang, H., Liu, R., An, X., Hu, C., Zhang, X., and Liu, H. (2021a). Bimetal-organic frameworks with coordinatively unsaturated metal sites for highly efficient Fenton-like catalysis. Chem. Eng. J. 414, 128669. doi:10.1016/j.cej.2021.128669
Liang, H., Liu, R., Hu, C., An, X., Zhang, X., Liu, H., et al. (2021b). Synergistic effect of dual sites on bimetal-organic frameworks for highly efficient peroxide activation. J. Hazard. Mater. 406, 124692. doi:10.1016/j.jhazmat.2020.124692
Lin, H., Li, S., Deng, B., Tan, W., Li, R., Xu, Y., et al. (2019). Degradation of bisphenol A by activating peroxymonosulfate with Mn0.6Zn0.4Fe2O4 fabricated from spent Zn-Mn alkaline batteries. Chem. Eng. J. 364, 541–551. doi:10.1016/j.cej.2019.01.189
Liu, G., Li, X., Han, B., Chen, L., Zhu, L., and Campos, L. C. (2017). Efficient degradation of sulfamethoxazole by the Fe (II)/HSO5− process enhanced by hydroxylamine: efficiency and mechanism. J. Hazard. Mater. 322, 461–468. doi:10.1016/j.jhazmat.2016.09.062
Liu, L., Sim, S. F., Lin, S., Wan, J., Zhang, W., Li, Q., et al. (2021). Integrated structural and chemical analyses for HCl-supported hydrochar and their adsorption mechanisms for aqueous sulfachloropyridazine removal. J. Hazard. Mater. 417, 126009. doi:10.1016/j.jhazmat.2021.126009
Lv, H., Zhao, H., Cao, T., Qian, L., Wang, Y., and Zhao, G. (2015). Efficient degradation of high concentration azo-dye wastewater by heterogeneous Fenton process with iron-based metal-organic framework. J. Mol. Catal. A Chem. 400, 81–89. doi:10.1016/j.molcata.2015.02.007
Ma, H., Yu, B., Wang, Q., Owens, G., and Chen, Z. (2021). Enhanced removal of pefloxacin from aqueous solution by adsorption and Fenton-like oxidation using NH2-MIL-88B. J. Colloid Interface Sci. 583, 279–287. doi:10.1016/j.jcis.2020.09.034
Ma, X., Wang, W., Sun, C., Li, H., Sun, J., and Liu, X. (2021). Adsorption performance and kinetic study of hierarchical porous Fe-based MOFs for toluene removal. Sci. Total Environ. 793, 148622. doi:10.1016/j.scitotenv.2021.148622
Milh, H., Cabooter, D., and Dewil, R. (2021). Role of process parameters in the degradation of sulfamethoxazole by heat-activated peroxymonosulfate oxidation: radical identification and elucidation of the degradation mechanism. Chem. Eng. J. 422, 130457. doi:10.1016/j.cej.2021.130457
Milh, H., Schoenaers, B., Stesmans, A., Cabooter, D., and Dewil, R. (2020). Degradation of sulfamethoxazole by heat-activated persulfate oxidation: elucidation of the degradation mechanism and influence of process parameters. Chem. Eng. J. 379, 122234. doi:10.1016/j.cej.2019.122234
Mishra, S., Singh, A. K., Cheng, L., Hussain, A., and Maiti, A. (2023). Occurrence of antibiotics in wastewater: potential ecological risk and removal through anaerobic-aerobic systems. Environ. Res. 226, 115678. doi:10.1016/j.envres.2023.115678
Mohammadnezhad, F., Kampouri, S., Wolff, S. K., Xu, Y., Feyzi, M., Lee, J.-H., et al. (2021). Tuning the optoelectronic properties of hybrid functionalized MIL-125-NH2 for photocatalytic hydrogen evolution. ACS Appl. Mater. Interfaces. 13, 5044–5051. doi:10.1021/acsami.0c19345
Ortega-Moreno, G. A., Ayala-Duran, S. C., Barbero, B. P., Narda, G. E., Bernini, M. C., and Pupo Nogueira, R. F. (2022). Photo-Fenton degradation of sulfamethoxazole using MIL-53(Fe) under UVA LED irradiation and natural sunlight. J. Environ. Chem. Eng. 10, 107678. doi:10.1016/j.jece.2022.107678
Pei, X.-Y., Ren, H.-Y., Xing, D.-F., Xie, G.-J., Cao, G.-L., Meng, J., et al. (2021). Electron transfer mechanism of peroxydisulfate activation by sewage sludge-derived biochar for enhanced degradation of sulfamethoxazole. Environ. Sci. Water Res. Technol. 7, 1563–1575. doi:10.1039/d1ew00279a
Ramos, M. D. N., Santana, C. S., Velloso, C. C. V., da Silva, A. H. M., Magalhaes, F., and Aguiar, A. (2021). A review on the treatment of textile industry effluents through Fenton processes. Process Saf. Environ. Prot. 155, 366–386. doi:10.1016/j.psep.2021.09.029
Ribeiro, R. S., Frontistis, Z., Mantzavinos, D., Venieri, D., Antonopoulou, M., Konstantinou, I., et al. (2016). Magnetic carbon xerogels for the catalytic wet peroxide oxidation of sulfamethoxazole in environmentally relevant water matrices. Appl. Catal. B Environ. 199, 170–186. doi:10.1016/j.apcatb.2016.06.021
Sharma, V. K., and Feng, M. (2019). Water depollution using metal-organic frameworks-catalyzed advanced oxidation processes: a review. J. Hazard. Mater. 372, 3–16. doi:10.1016/j.jhazmat.2017.09.043
Song, Z., Zhang, X., Liu, B., Liu, J., and Wang, L. (2023). Efficient degradation of tetracycline residues in pharmaceutical wastewater by Ni/Fe bimetallic atomic cluster composite catalysts with enhanced electron transfer pathway. Chemosphere 335, 139181. doi:10.1016/j.chemosphere.2023.139181
Su, T., Wang, X., Chen, X., Cheng, Y., Wang, Z., Wu, Z., et al. (2022). Fe3+-NTA-Catalyzed homogenous fenton-like degradation of trichloroethylene in groundwater at natural pH (∼8.0): efficacy, by-products, and H2O2 utilization. J. Environ. Eng. 148. doi:10.1061/(asce)ee.1943-7870.0001951
Suzuki, N., Okazaki, A., Takagi, K., Serizawa, I., Hirami, Y., Noguchi, H., et al. (2022). Complete decomposition of sulfamethoxazole during an advanced oxidation process in a simple water treatment system. Chemosphere 287, 132029. doi:10.1016/j.chemosphere.2021.132029
Tang, J., and Wang, J. (2018). Fenton-like degradation of sulfamethoxazole using Fe-based magnetic nanoparticles embedded into mesoporous carbon hybrid as an efficient catalyst. Chem. Eng. J. 351, 1085–1094. doi:10.1016/j.cej.2018.06.169
Tang, J., and Wang, J. (2019). MOF-derived three-dimensional flower-like FeCu@C composite as an efficient Fenton-like catalyst for sulfamethazine degradation. Chem. Eng. J. 375, 122007. doi:10.1016/j.cej.2019.122007
Tang, J., and Wang, J. (2020). Iron-copper bimetallic metal-organic frameworks for efficient Fenton-like degradation of sulfamethoxazole under mild conditions. Chemosphere 241, 125002. doi:10.1016/j.chemosphere.2019.125002
Wang, J. F., Liu, Y., Shao, P., Zhu, Z.-Y., Ji, H.-D., Du, Z.-X., et al. (2022). Efficient ofloxacin degradation via photo-Fenton process over eco-friendly MIL-88A(Fe): performance, degradation pathways, intermediate library establishment and toxicity evaluation. Environ. Res. 210, 112937. doi:10.1016/j.envres.2022.112937
Wang, S., and Wang, J. (2017). Comparative study on sulfamethoxazole degradation by Fenton and Fe (II)-activated persulfate process. RSC Adv. 7, 48670–48677. doi:10.1039/c7ra09325j
Wang, X., Zhang, D., Ma, K., Bu, C., Wang, Y., Tang, Y., et al. (2024). Biochar and zero-valent iron alleviated sulfamethoxazole and tetracycline co-stress on the long-term system performance of bioretention cells: insights into microbial community, antibiotic resistance genes and functional genes. Environ. Res. 248, 118271. doi:10.1016/j.envres.2024.118271
Wang, Y., Gao, C.-Y., Zhang, Y.-Z., Leung, M. K. H., Liu, J.-W., Huang, S.-Z., et al. (2021). Bimetal-organic framework derived CoFe/NC porous hybrid nanorods as high-performance persulfate activators for bisphenol a degradation. Chem. Eng. J. 421, 127800. doi:10.1016/j.cej.2020.127800
Wang L., L., Jiang, H., Wang, H., Show, P. L., Ivanets, A., Luo, D., et al. (2022). MXenes as heterogeneous Fenton-like catalysts for removal of organic pollutants: a review. J. Environ. Chem. Eng. 10, 108954. doi:10.1016/j.jece.2022.108954
Wu, Q., Siddique, M. S., and Yu, W. (2021). Iron-nickel bimetallic metal-organic frameworks as bifunctional Fenton-like catalysts for enhanced adsorption and degradation of organic contaminants under visible light: kinetics and mechanistic studies. J. Hazard. Mater. 401, 123261. doi:10.1016/j.jhazmat.2020.123261
Wu, Q., Yang, H., Kang, L., Gao, Z., and Ren, F. (2020). Fe-based metal-organic frameworks as Fenton-like catalysts for highly efficient degradation of tetracycline hydrochloride over a wide pH range: acceleration of Fe (II)/Fe (III) cycle under visible light irradiation. Appl. Catal. B Environ. 263, 118282. doi:10.1016/j.apcatb.2019.118282
Xiao, K., Shu, B., Lv, K., Huang, P., Chang, Q., Wu, L., et al. (2023). Recent progress of MIL MOF materials in degradation of organic pollutants by Fenton reaction. Catalysts 13, 734. doi:10.3390/catal13040734
Yang, F., Jiang, G., Chang, Q., Huang, P., and Lei, M. (2021). Fe/N-doped carbon magnetic nanocubes toward highly efficient selective decolorization of organic dyes under ultrasonic irradiation. Chemosphere 283, 131154. doi:10.1016/j.chemosphere.2021.131154
Yu, J., Cao, J., Yang, Z., Xiong, W., Xu, Z., Song, P., et al. (2020). One-step synthesis of Mn-doped MIL-53(Fe) for synergistically enhanced generation of sulfate radicals towards tetracycline degradation. J. Colloid Interface Sci. 580, 470–479. doi:10.1016/j.jcis.2020.07.045
Zeng, T., Yu, M., Zhang, H., He, Z., Chen, J., and Song, S. (2017). Fe/Fe3C@ N-doped porous carbon hybrids derived from nano-scale MOFs: robust and enhanced heterogeneous catalyst for peroxymonosulfate activation. Catal. Sci. Technol. 7, 396–404. doi:10.1039/c6cy02130a
Zhang, Z., Xiao, S., Meng, X., and Yu, S. (2023). Research progress of MOF-based membrane reactor coupled with AOP technology for organic wastewater treatment. Environ. Sci. Pollut. Res. 30, 104958–104975. doi:10.1007/s11356-023-29852-4
Keywords: Fenton-like reaction, amino group introduction, dual active site, reaction mechanism, sulfamethoxazole
Citation: Zhang X, Liu Y and Yuan J (2025) Amino-functionalized Fe/Co bimetallic MOFs for accelerated Fe (III)/Fe (II) cycling and efficient degradation of sulfamethoxazole in Fenton-like system. Front. Chem. 13:1579108. doi: 10.3389/fchem.2025.1579108
Received: 18 February 2025; Accepted: 05 March 2025;
Published: 25 March 2025.
Edited by:
Xiulin Yang, Guangxi Normal University, ChinaReviewed by:
Benzhi Wang, Sungkyunkwan University, Republic of KoreaCopyright © 2025 Zhang, Liu and Yuan. This is an open-access article distributed under the terms of the Creative Commons Attribution License (CC BY). The use, distribution or reproduction in other forums is permitted, provided the original author(s) and the copyright owner(s) are credited and that the original publication in this journal is cited, in accordance with accepted academic practice. No use, distribution or reproduction is permitted which does not comply with these terms.
*Correspondence: Jiajia Yuan, NTAxNTE3ODc2QHFxLmNvbQ==
†ORCID: Xianbing Zhang, https://orcid.org/0000-0002-1637-4758
Disclaimer: All claims expressed in this article are solely those of the authors and do not necessarily represent those of their affiliated organizations, or those of the publisher, the editors and the reviewers. Any product that may be evaluated in this article or claim that may be made by its manufacturer is not guaranteed or endorsed by the publisher.
Research integrity at Frontiers
Learn more about the work of our research integrity team to safeguard the quality of each article we publish.