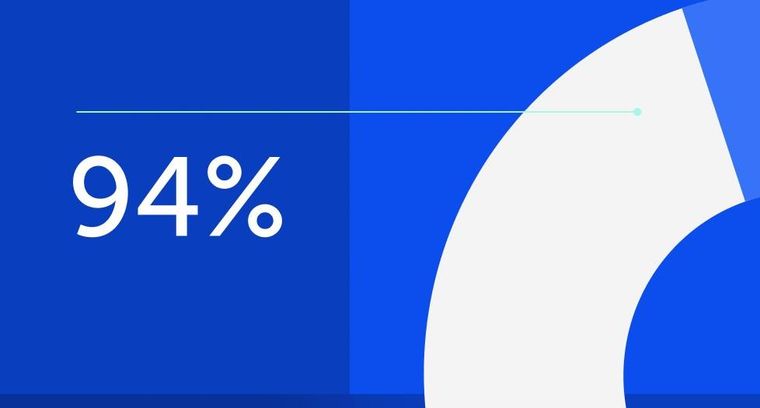
94% of researchers rate our articles as excellent or good
Learn more about the work of our research integrity team to safeguard the quality of each article we publish.
Find out more
ORIGINAL RESEARCH article
Front. Chem., 17 March 2025
Sec. Medicinal and Pharmaceutical Chemistry
Volume 13 - 2025 | https://doi.org/10.3389/fchem.2025.1552298
This article is part of the Research TopicNatural and Artificially-Engineered Medicines: Design, Characterization and Diseases ManagementView all 6 articles
The extract of medicinal plants is increasingly popular around the whole world due to its attractive therapeutic effects. However, the bioavailability of the extract of bioactive compounds was barely satisfactory due to its easily deactivated and untargeted properties. The use of nanotechnology to develop novel carrier delivery techniques for bioactive extracts has been proven to have significant potential and provides an amazing improvement in the therapeutic effect. Calcium carbonate nanoparticles (CaCO3 NPs), as representative biodegradable materials, are well recognized as environmentally responsive delivery vehicles for disease treatment. In this study, extracts of the root of ginseng, the fruit of Alpinia oxyphylla Miq., and the stem of Millettia speciosa Champ. were developed as a CaCO3 nanoparticle loading drug. All of the three composite nanoparticles exhibited spherical shapes with a narrow size distribution. Notably, the ginseng extract-loaded CaCO3 NPs hold a relatively higher entrapment efficiency of up to 55.2% ± 6.7% and excellent release performance under acidic conditions (pH = 5.5). Moreover, intravenous injection of ginseng CaCO3 NPs resulted in significantly enhanced therapeutic effects in the treatment of glioma. The results demonstrate that CaCO3-based composite nanoparticles are ideal for the delivery of plant extracts, and the systems are expected to be effective against various types of diseases in the future.
Throughout history, medicinal plants have demonstrated efficacy in augmenting immune function and cognitive abilities when used appropriately. Bioactive compounds such as polysaccharides, saponins, flavonoids, polyphenols, and volatile oils found in medicinal plant extracts significantly contribute to their health-promoting properties (Harvey et al., 2015). With continuous research and development of techniques, medicinal plant extracts have become increasingly prominent in the global market as plant-based herbal remedies for a range of therapeutic applications owing to their anticancer, anti-aging, anti-inflammatory, and hepatoprotective properties and play a role in immune response, cognition, and neurological disorders (Zhang et al., 2024; Zhao et al., 2021). Most traditional herbal medicines contain crude extracts that have multiple potencies in treating various diseases. Within the extensive array of traditional medicinal plants, several Millettia species within the Leguminosae family have been investigated to identify bioactive constituents, including phenylpropanoids, terpenoids, alkaloids, flavonoids, and chalcones (Huang et al., 2022; Chen et al., 2016). Millettia speciosa Champ. stands out as one of the most extensively studied species, with its roots serving as a folk medicine of considerable economic and medicinal significance. This species is acknowledged for its dual-purpose application as both a culinary herb and a medicinal plant, and it is predominantly found in tropical and subtropical regions, particularly in southeastern China. More than 50 active compounds have been isolated successfully to verify the immunomodulatory, antioxidant, analgesic, and anti-hepatitis activities of the aqueous extracts of the stem of M. speciosa Champ. (Xie et al., 2024; Zhang et al., 2021; Lai et al., 2023). In clinical settings, M. speciosa Champ. is predominantly used for the treatment of various disorders, including lumbar muscle strain, cough associated with lung deficiency, rheumatoid arthritis, traumatic injuries, and other pathologies (Chen J. et al., 2021; Zhao et al., 2017). Recently, active compounds derived from M. speciosa Champ. were applied in cancer therapy and have shown excellent therapeutic effects (Xie et al., 2024; Chen J. et al., 2021). Another medically valuable understory plant in southern China is Alpinia oxyphylla Miq., which is expected to exert broadly therapeutic effects in disease treatment. The fruits of the A. oxyphylla are recognized as one of “the four famous south medicines” and are used in neuroprotective, anti-inflammatory, anti-nociceptive, and anti-diuretic applications (Yu et al., 2003; Zhang et al., 2018). Among plant species whose roots are employed for medicinal purposes, ginseng is one of the most popular and acclaimed herbs around the world (Chang-Xiao and Pei-Gen, 1992; Wong et al., 2015). Due to its medicinal properties, which include anticancer, antidiabetic, and immunomodulatory effects, as well as its contribution to stress relief (Kim et al., 2018; Huang et al., 2023; Balusamy et al., 2023), ginseng is extensively utilized as dietary supplements, personal care products, pharmaceuticals, and oral care products. Recent clinical investigations have demonstrated the prophylactic and therapeutic potential of ginseng against cancer. Numerous in vitro studies have elucidated the high anticancerous and immunomodulatory efficacy of ginsenosides, including ginsenoside, derived from ginseng. (Balusamy et al., 2023). Although the safety and efficacy of the aforementioned plant-derived extracts have been established, their clinical application is limited by the suboptimal pharmacokinetic properties, including low solubility, inadequate membrane permeability, and metabolic lability. Improving the pharmacokinetic profile of the therapeutic agents by enhancing the solubility, providing protection, facilitating intracellular transport, and enabling sustained and controlled release is important for clinical application.
To enhance the drug efficacy and improve the bioavailability of active agents, nanotechnology-based formulations are extensively used to construct novel nanoagents (Mitchell et al., 2021; Nance et al., 2022). The encapsulation of herbal extracts within polymeric nanoparticles has been extensively validated to enhance the solubility, modulate drug release, reduce side effects, and enhance drug targeting and frequently results in increased therapeutic efficacy while shielding the active compounds from premature degradation (Kim et al., 2018; Chen X. et al., 2021; Wang et al., 2024). However, the application of encapsulation was commonly restricted from drug leakage and undesirable release performance. Hence, there is an urgent need for the development of a carrier that is relatively easy to prepare, has ideal release performance, and has excellent biocompatibility for delivery of plant-derived extracts. Among different nanocarriers, calcium carbonate nanoparticles (CaCO3 NPs) have gained much attention recently because of their excellent biocompatibility and biodegradability, easy preparation, and pH sensitivity (Qi et al., 2018). Many important studies have used CaCO3 NPs to deliver small-molecule drugs, genes, and proteins (Zhao et al., 2022; Kim et al., 2013). Thus, we hypothesized that CaCO3 NPs might be a suitable carrier for plant-derived extract delivery.
In this study, we use the extract of the root of ginseng, the fruit of A. oxyphylla Miq., and the stem of M. speciosa champ. as active agents to prepare a composite delivery system for achieving enhanced therapeutic efficacy. Glioblastoma multiforme is the most common malignant primary brain tumor with a median survival. We utilized CaCO3 NPs as carriers and optimized the synthesis of the extract-loaded composite nanoparticles for enhanced delivery performance in glioma treatment. The size and distribution, release curve, and therapeutic efficacy of ginseng CaCO3 NPs were verified, and they demonstrated an excellent anti-glioma effect. This is the first CaCO3 NP-based delivery strategy for naturally extracted ingredients of root, fruit, and stem, and it provides a promising strategy for glioma therapy using ginseng extract. We hope this study can provide inspiration for the development of novel delivery vehicles for plant extracts.
Ginseng, A. oxyphylla Miq., and M. speciosa Champ. extracts were purchased from National Institutes for Food and Drug Control. 3-(4,5-Dimethylthiazol-2-yl)-2,5-diphenyltetrazolium bromide, dimethyl sulfoxide, cyclohexane, IGEPAL® CO-520, calcium chloride, sodium carbonate, and chloroform were purchased from Sigma-Aldrich Chemical Co. (St. Louis, MO, United States). Cell culture plates and round coverslips were purchased from NEST Biotechnology Co., Ltd. (Wuxi, China). Fetal bovine serum (FBS) and high-glucose Dulbecco’s modified Eagle’s medium (DMEM) were obtained from Thermo Fisher Scientific (Chicago, IL, United States). C57BL/6 mice (5–6 weeks old, female) were purchased from Beijing Vital River Laboratory Animal Technology Company (Beijing, China). The experimental protocol was approved by the Committee on Ethical Animal Experiment at Hainan Medical University (HYLL-2023-379).
The CaCO3 NPs loaded with ginseng, A. oxyphylla Miq., or M. speciosa Champ. were prepared by using a water in-oil reverse emulsion method (Qi et al., 2018; Li et al., 2010). In brief, 500 μL of 300 mM CaCl2 and the aqueous extracts of ginseng, A. oxyphylla Miq., or Milletia speciosa Champ. were dispersed in 50 mL of the cyclohexane/IGEPAL CO-520 (70/30, V/V) “solution to form a water-in-oil reverse microemulsion. The “carbonate microemulsion” was prepared by combining 500 μL of 300 mM Na2CO3 in another 50-mL oil phase. Two microemulsions were combined and mixed overnight at room temperature. Then, 100 mL of absolute ethanol was added to the microemulsion and centrifuged at 10,000 g for 40 min to pellet to remove the cyclohexane and surfactant. The drug-loaded CaCO3 NPs werewashed 2–3 times using ethanol and suspended in water for storage.
The hydrodynamic sizes of CaCO3 NPs were measured via dynamic light scattering (Zetasizer Nano ZS, Malvern Instruments Ltd., UK). The morphologies of NPs were observed via transmission electron microscopy (TEM, JEOL 100CX II, Japan). The extent of entrapment efficiency was quantified using a spectrophotometer (UV-2600, Shimzadu, Kyoto, Japan). The absorbance values of ginseng, A. oxyphylla Miq., and M. speciosa Champ. aqueous extracts were measured at 540 nm, 485 nm, 256 nm, respectively. The entrapment efficiency was calculated based on the following equation:
The pH-dependent release of ginseng, A. oxyphylla Miq., or M. speciosa Champ from CaCO3 NPs was studied using a dialysis method at 37°C. Phosphate-buffered saline (PBS) solutions with pH 7.4 and pH 5.5 were used as the media for simulating normal blood/tissue and tumoral lysosomal acidic conditions. CaCO3 NPs loaded with ginseng, A. oxyphylla Miq., or M. speciosa Champ. were placed into pretreated dialysis bags (MW cutoff of 14 kDa). The dialysis bags were placed into brown bottles containing 100 mL of PBS solutions of different pH values. These bottles were shaken at 37°C while being shielded from light. Samples were withdrawn at various intervals and replaced with an equal volume of fresh buffer. The amount of the released drug was analyzed using a spectrophotometer.
Cell viability was determined via MTT assay. GL261 cells were seeded in 96-well plates at 2 × 104 cells per well for overnight culture. After 24 h of treatment with the drugs (ginseng aqueous extracts, A. oxyphylla Miq. aqueous extracts, M. speciosa Champ. aqueous extracts, ginseng CaCO3 NPs, A. oxyphylla Miq. CaCO3 NPs, and M. speciosa Champ. CaCO3 NPs). The MTT solution was added. After an additional 4 h of incubation, the supernatants were removed carefully, and 150 μL of DMSO was added to each well. The absorbance value was measured at 570 nm using the SpectraMax M5 microplate reader.
For apoptosis analysis via flow cytometry, GL261 cells (5 × 105 per well) were seeded on six-well plates. Then, cells were treated with CaCO3 NPs for 12 h. The cells were harvested by trypsinization and were stained using the Annexin V-FITC Apoptosis Detection Kit (KeyGEN Biotech, Nanjing, China) based on the manufacturer’s protocol. Stained cells were immediately analyzed on a BD Accuri C6 Flow Cytometer.
GL261 cells were transformed using the luciferase gene (GL261-Luc) for constructing the glioma mouse model. To construct the GL261 intracranial orthotopic glioblastoma mouse model, C57BL/6 mice were anesthetized with isoflurane and placed in a stereotactic instrument. Then, 1.0 × 105 GL261 cells in a 5 µL volume were injected into the right striatum (1 mm anterior, 2 mm right lateral from bregma, and 3.5 mm deep). The skin was sealed using surgical glue. Real-time bioluminescence imaging was used to evaluate the therapeutic efficiency. The GL261-Luc mice were randomly divided into three groups (n = 5 per group), which were intravenously injected with three injections of PBS, ginseng aqueous extract, and ginseng CaCO3 NPs on days 5, 8, and 11 after implantation, containing ginseng (5 mg kg−1) per dose. The bioluminescence signal was used to evaluate the therapeutic efficiency at fifth, 10th, and 15th days after tumor implantation through the IVIS Spectrum system. Throughout the study, mice were weighted regularly. For safety detection, 500 μL of blood was collected from the tail vein of each mouse, and the blood serum was isolated to analyze some biochemical indicators containing aspartate transaminase (ALT), aspartate aminotransferase (AST), blood urea nitrogen (BUN), and creatinine (CREA). Major organs were collected and processed for immunohistochemical analysis.
All data were collected from three independent experiments and then expressed as means ± standard deviation (SD). Statistical significance was analyzed using an unpaired, two-tailed Student’s t-test, as well as one-way or two-way ANOVA analysis using GraphPad Prism 5.0 (San Diego, CA, United States). Statistical significance thresholds were set at *p < 0.05, **p < 0.01, and ***p < 0.001.
To construct the composite nanoparticles, we chose the aqueous extracts derived from the root of the ginseng, the fruit of A. oxyphylla Miq., and the stem of M. speciosa champ. as the active agents. CaCO3 NPs loaded with the aqueous extracts were prepared through a reverse microemulsion method (Figure 1). The particle size and polydispersity indexes (PDI) of three kinds of CaCO3 NPs were determined via dynamic light scattering (DLS). As shown in Figure 1, the results showed that the average particle sizes of ginseng, A. oxyphylla Miq., and M. speciosa Champ. CaCO3 NPs were 50.7 ± 2.5 nm, 48.3 ± 3.1 nm, and 56.4 ± 5.3 nm, respectively. The PDI measured in PBS were 0.12 ± 0.01, 0.11 ± 0.02, and 0.18 ± 0.10, respectively, indicating a uniform particle size and ideal distribution in the physiological environment. We next measured the loading efficacy of the composite nanoparticles. The entrapment efficiencies (EE) of ginseng, A. oxyphylla Miq., and M. speciosa Champ. were measured as 55.2% ± 6.7%, 45.9% ± 12.1%, and 40.2% ± 4.8%, respectively, implying that the extract of ginseng had relatively higher EE compared with the other two kinds of extracts. By optimizing the preparation conditions, we achieved high loading efficiency, providing assurance for subsequent applications.
Figure 1. Schematic illustration of the construction of CaCO3-based composite nanoparticles. The aqueous extracts derived from the root of the ginseng, the fruit of A. oxyphylla Miq., and the stem of M. speciosa champ. were prepared as loaded drugs for the construction of composite nanoparticles. The size, polydispersity (PDI), and entrapment efficiency (EE) of the corresponding composite nanoparticles (ginseng CaCO3 nanoparticles, A. oxyphylla Miq. CaCO3 nanoparticles, and M. speciosa champ. CaCO3 nanoparticles) were measured for the characterization of the load performance. Data are expressed as mean ± SD (n = 5).
We next characterize the morphology and release performance of the nanoparticles. The stability of the nanoparticles was demonstrated to be good, allowing for stable storage at room temperature over a 2-week period. The results of transmission electron microscopy (TEM) revealed that ginseng, A. oxyphylla Miq., and M. speciosa Champ. CaCO3 NPs were spherical in shape, with a relatively small size of approximately 30 nm, as shown in Figure 2A and Supplementary Figure S1. The relatively larger sizes measured via DLS than those via TEM may be attributed to the surface hydration of NPs. In the release performance test, the pH-sensitive release of ginseng, A. oxyphylla Miq., and M. speciosa Champ. from CaCO3 NPs was analyzed via dialysis. Phosphate-buffered saline (PBS) solutions at pH 7.4 and 5.5 were utilized to replicate the physiological conditions of normal healthy tissue and the acidic environment of tumor tissues, respectively. After 48 h, 93.7% of ginseng, 98.3% of A. oxyphylla Miq., and 77.2% of M. speciosa Champ. were released from the corresponding CaCO3 NPs at pH 5.5. In contrast, only 13.3% of ginseng, 30.0% of A. oxyphylla Miq., and 12.9% of M. speciosa Champ. were released at pH 7.4 (Figure 2B). These data revealed that low pH (5.5) triggered drug release from CaCO3 NPs. Moreover, ginseng CaCO3 NPs have a better controlled-release performance. The stability of M. speciosa Champ. CaCO3 NPs was assessed in physiological environments and PBS. As shown in Figure SX, there was no significant change in size after 7 days (Supplementary Figure S2). We next used Cy5-loaded CaCO3 NPs to simulate drug internalization. As shown in Figure 3, the effectiveness of internalization was tested in GL261 cells. Through the systematic analysis of the abovementioned experimental results, we found that the size distribution and release properties of ginseng particles make them most suitable as a therapeutic carrier among the three types of particles.
Figure 2. Preparation and characterization of CaCO3-based composite nanoparticles. (A) TEM image of CaCO3 NPs. Scale bar, 100 nm. (B) Cumulative drug release from CaCO3 NPs.
Figure 3. Confocal fluorescence images of GL261 cells after incubation with Cy5-loaded CaCO3 NPs. Scale bar: 50 µm.
Next, the cytotoxicities of ginseng, A. oxyphylla Miq., and M. speciosa Champ. aqueous extracts and ginseng, A. oxyphylla Miq., and M. speciosa Champ. CaCO3 NPs were evaluated in GL-261 cells via MTT assay. As can be seen in Figure 4, the treatment of the ginseng aqueous extract exerted greater inhibition of cell viability compared with A. oxyphylla Miq. or M. speciosa Champ. in GL-261 cells. At 50 μg of ginseng, only 53.5% and 46.4% cells survived with the treatment of ginseng aqueous extract and ginseng CaCO3 NPs, respectively. In contrast, A. oxyphylla Miq. and M. speciosa Champ. only had mild toxicity. Furthermore, the loading of drugs into CaCO3 NPs could partly increase the toxicity. Thus, ginseng CaCO3 NPs were chosen for further evaluation.
Figure 4. Viabilities of GL261 cells after different treatments for 24 h. (A) Cell viability of ginseng aqueous extracts and ginseng CaCO3 NPs. (B) Cell viability of A. oxyphylla Miq. aqueous extracts and A. oxyphylla Miq. CaCO3 NPs. (C) Cell viability of M. speciosa Champ. aqueous extracts and M. speciosa Champ. CaCO3 NPs.
To further quantify cell apoptosis caused by the treatment with ginseng CaCO3 NPs against GL261 cells, flow cytometry analysis was utilized. After incubation with ginseng CaCO3 NPs for 12 h, GL261 cells were stained with annexin V and propidium iodide (PI). As shown in Figure 5, ginseng CaCO3 NPs induced up to 30.6% overall apoptosis, further proving the cytotoxicity in GL261 cells.
Encouraged by the antitumor effect in vitro of ginseng CaCO3 NPs, we next investigated the antitumor efficacy in vivo through an orthotopic GL261-Luc glioma mouse model. As shown in Figure 6A, IVIS Spectrum demonstrated rapid tumor growth in the PBS or ginseng aqueous extract-treated group, whereas the bioluminescence signals of the ginseng CaCO3 NP group were obviously weaker than those of the PBS or ginseng aqueous extract-treated groups, indicating ginseng CaCO3 NPs hold the strongest antitumor effect. Moreover, the survival study also proved that ginseng CaCO3 NPs could prolong mice survival and lead to a 60% survival rate in 30 days (Figure 6B). The body weight of mice was deeply affected by different treatments, which is similar to the trend of survival rate (Figure 6C). No significant alterations were observed in the blood biochemical markers of liver and kidney toxicity, including alanine aminotransferase (ALT), aspartate aminotransferase (AST), blood urea nitrogen (BUN), and creatinine (CREA), in all therapy groups, indicating that no significant renal and liver toxicity was observed after ginseng CaCO3 NP treatment (Supplementary Figure S3). Furthermore, no significant histopathological changes were observed in the harvested heart, liver, spleen, lung, and kidney via HE staining (Figure 7). All these results proved that ginseng CaCO3 NPs could be a safe and effective strategy for glioma treatment.
Figure 6. In vivo anti-glioma activity of ginseng CaCO3 NPs. (A) Representative bioluminescence images of GL261-Luc glioma-bearing mice treated via different groups. (B) Survival curve for the mice (n = 5 mice per group). (C) Body weight change in different groups.
We used extracts from the roots of ginseng, the fruits of A. oxyphylla Miq., and the stems of M. speciosa Champ. as bioactive constituents to fabricate a composite delivery system that aims to improve the therapeutic efficacy. We explored a novel approach using CaCO3 NPs to combat the defects in the development of the plant extract-involved drug delivery carriers. The size, distribution, release kinetics, and therapeutic efficacy of the composite nanoparticles were characterized, and ginseng CaCO3 NPs exhibited great potential in glioma therapy. This study represents the first to use a CaCO3 NP-based delivery strategy for the natural extracts of roots, fruits, and stems, offering a promising therapeutic approach for glioma treatment using ginseng extract. We believe that the development of novel nanoformulations can promote the application of natural products in disease treatment.
The original contributions presented in the study are included in the article/Supplementary Material; further inquiries can be directed to the corresponding authors.
The animal study was approved by the Committee on Ethical Animal Experiment at Hainan Medical University. The study was conducted in accordance with the local legislation and institutional requirements.
XZ: conceptualization, data curation, formal analysis, and writing–original draft. TM: writing–review and editing and validation. RL: writing–review and editing, formal analysis, and validation. XF: writing–review and editing and funding acquisition. LH: writing–review and editing, investigation, and supervision.
The author(s) declare that financial support was received for the research, authorship, and/or publication of this article. This work supported by Joint Program on Health Science and Technology Innovation of Hainan Province (WSJK2024QN117), Hainan Provincial Natural Science Foundation of China (824QN261). The authors are grateful to the support of instruments and facilities provided by Public Research Center of Hainan Medical University.
The authors declare that the research was conducted in the absence of any commercial or financial relationships that could be construed as a potential conflict of interest.
The author(s) declare that no Generative AI was used in the creation of this manuscript.
All claims expressed in this article are solely those of the authors and do not necessarily represent those of their affiliated organizations, or those of the publisher, the editors and the reviewers. Any product that may be evaluated in this article, or claim that may be made by its manufacturer, is not guaranteed or endorsed by the publisher.
The Supplementary Material for this article can be found online at: https://www.frontiersin.org/articles/10.3389/fchem.2025.1552298/full#supplementary-material
Balusamy, S. R., Perumalsamy, H., Huq, M. A., Yoon, T. H., Mijakovic, I., Thangavelu, L., et al. (2023). A comprehensive and systemic review of ginseng-based nanomaterials: synthesis, targeted delivery, and biomedical applications. Med. Res. Rev. 43 (5), 1374–1410. doi:10.1002/med.21953
Chang-Xiao, L., and Pei-Gen, X. (1992). Recent advances on ginseng research in China. J. Ethnopharmacol. 36 (1), 27–38. doi:10.1016/0378-8741(92)90057-x
Chen, J., Lyu, X., Zhai, D., and Cai, J. (2021a). Advances in chemical constituents and pharmacological effects of Millettia speciosa Champ. J. Holist. Integr. Pharm. 2 (4), 287–300. doi:10.1016/s2707-3688(23)00072-9
Chen, S.-L., Yu, H., Luo, H.-M., Wu, Q., Li, C.-F., and Steinmetz, A. (2016). Conservation and sustainable use of medicinal plants: problems, progress, and prospects. Chin. Med. 11 (1), 37. doi:10.1186/s13020-016-0108-7
Chen, X., Huang, Z., Luo, S.-Y., Zong, M.-H., and Lou, W.-Y. (2021b). Multi-functional magnetic hydrogels based on Millettia speciosa Champ residue cellulose and Chitosan: highly efficient and reusable adsorbent for Congo red and Cu2+ removal. Chem. Eng. J. 423, 130198. doi:10.1016/j.cej.2021.130198
Harvey, A. L., Edrada-Ebel, R., and Quinn, R. J. (2015). The re-emergence of natural products for drug discovery in the genomics era. Nat. Rev. Drug Discov. 14 (2), 111–129. doi:10.1038/nrd4510
Huang, L., Li, H.-J., and Wu, Y.-C. (2023). Processing technologies, phytochemistry, bioactivities and applications of black ginseng-a novel manufactured ginseng product: a comprehensive review. Food Chem. 407, 134714. doi:10.1016/j.foodchem.2022.134714
Huang, Z., Zong, M.-H., and Lou, W.-Y. (2022). Effect of acetylation modification on the emulsifying and antioxidant properties of polysaccharide from Millettia speciosa Champ. Food Hydrocoll. 124, 107217. doi:10.1016/j.foodhyd.2021.107217
Kim, H., Lee, J. H., Kim, J. E., Kim, Y. S., Ryu, C. H., Lee, H. J., et al. (2018). Micro-/nano-sized delivery systems of ginsenosides for improved systemic bioavailability. J. Ginseng Res. 42 (3), 361–369. doi:10.1016/j.jgr.2017.12.003
Kim, S. K., Foote, M. B., and Huang, L. (2013). Targeted delivery of EV peptide to tumor cell cytoplasm using lipid coated calcium carbonate nanoparticles. Cancer Lett. 334, 311–318. doi:10.1016/j.canlet.2012.07.011
Lai, L.-H., Zong, M.-H., Huang, Z., Ni, Z.-F., Xu, P., and Lou, W.-Y. (2023). Purification, structural elucidation and biological activities of exopolysaccharide produced by the endophytic Penicillium javanicum from Millettia speciosa Champ. J. Biotechnol. 362, 54–62. doi:10.1016/j.jbiotec.2022.12.008
Li, J., Chen, Y.-C., Tseng, Y.-C., Mozumdar, S., and Huang, L. (2010). Biodegradable calcium phosphate nanoparticle with lipid coating for systemic siRNA delivery. J. Control. Release 142 (3), 416–421. doi:10.1016/j.jconrel.2009.11.008
Mitchell, M. J., Billingsley, M. M., Haley, R. M., Wechsler, M. E., Peppas, N. A., and Langer, R. (2021). Engineering precision nanoparticles for drug delivery. Nat. Rev. Drug Discov. 20 (2), 101–124. doi:10.1038/s41573-020-0090-8
Nance, E., Pun, S. H., Saigal, R., and Sellers, D. L. (2022). Drug delivery to the central nervous system. Nat. Rev. Mater. 7 (4), 314–331. doi:10.1038/s41578-021-00394-w
Qi, C., Lin, J., Fu, L.-H., and Huang, P. (2018). Calcium-based biomaterials for diagnosis, treatment, and theranostics. Chem. Soc. Rev. 47, 357–403. doi:10.1039/c6cs00746e
Wang, Y., Fu, X., Zhu, Y., Lin, M., Cai, R., Zhu, Y., et al. (2024). An intratumor bacteria-targeted DNA nanocarrier for multifaceted tumor microenvironment intervention. Mater. Today Bio 27, 101144. doi:10.1016/j.mtbio.2024.101144
Wong, A. S. T., Che, C.-M., and Leung, K.-W. (2015). Recent advances in ginseng as cancer therapeutics: a functional and mechanistic overview. Nat. Product. Rep. 32 (2), 256–272. doi:10.1039/c4np00080c
Xie, Z., Cheng, X., Mao, J., Zhu, Y., Li, L., and Mei, Z. (2024). Extracellular vesicles enhance the in vivo antitumor effects of millettia species-derived compounds in chronic myelogenous leukemia therapy. Front. Chem. 12, 1425318. doi:10.3389/fchem.2024.1425318
Yu, X., An, L., Wang, Y., Zhao, H., and Gao, C. (2003). Neuroprotective effect of Alpinia oxyphylla Miq. fruits against glutamate-induced apoptosis in cortical neurons. Toxicol. Lett. 144 (2), 205–212. doi:10.1016/s0378-4274(03)00219-4
Zhang, M., Cui, C., Lin, Y., and Cai, J. (2021). Ameliorating effect on glycolipid metabolism and chemical profile of Millettia speciosa champ. extract. J. Ethnopharmacol. 279, 114360. doi:10.1016/j.jep.2021.114360
Zhang, Q., Zheng, Y., Hu, X., Hu, X., Lv, W., Lv, D., et al. (2018). Ethnopharmacological uses, phytochemistry, biological activities, and therapeutic applications of Alpinia oxyphylla Miquel: a review. J. Ethnopharmacol. 224, 149–168. doi:10.1016/j.jep.2018.05.002
Zhang, Y., Qiu, L., Li, H., Cai, W., Liu, E., Zhang, H., et al. (2024). Biomimetically engineered plant-derived exosomes-like nanovesicles for rheumatoid arthritis therapy. Chin. Chem. Lett., 110658. doi:10.1016/j.cclet.2024.110658
Zhao, J., Duan, Z., Ma, X., Liu, Y., and Fan, D. (2021). Recent advances in systemic and local delivery of ginsenosides using nanoparticles and nanofibers. Chin. J. Chem. Eng. 30, 291–300. doi:10.1016/j.cjche.2020.11.012
Zhao, Y., Bian, Y., Xiao, X., Liu, B., Ding, B., Cheng, Z., et al. (2022). Tumor microenvironment-responsive Cu/CaCO3-based nanoregulator for mitochondrial homeostasis disruption-enhanced chemodynamic/sonodynamic therapy. Small 18, 2204047. doi:10.1002/smll.202204047
Keywords: ginseng, Millettia speciosa Champ., Alpinia oxyphylla Miq., calcium carbonate nanoparticles, drug delivery
Citation: Zhuang X, Ma T, Liu R, Fang X and Huang L (2025) Composite nanoparticle-based vesicles achieve enhanced delivery effects of the natural plant extract of the root, stem, and fruit. Front. Chem. 13:1552298. doi: 10.3389/fchem.2025.1552298
Received: 27 December 2024; Accepted: 10 February 2025;
Published: 17 March 2025.
Edited by:
Yang Zhu, National University of Singapore, SingaporeReviewed by:
Xue-Ming Zhou, Hainan Normal University, ChinaCopyright © 2025 Zhuang, Ma, Liu, Fang and Huang. This is an open-access article distributed under the terms of the Creative Commons Attribution License (CC BY). The use, distribution or reproduction in other forums is permitted, provided the original author(s) and the copyright owner(s) are credited and that the original publication in this journal is cited, in accordance with accepted academic practice. No use, distribution or reproduction is permitted which does not comply with these terms.
*Correspondence: Xingyue Fang, aHl5Znk0MTA5QG11aG4uZWR1LmNu; Liangjiu Huang, aHVhbmdsaWFuZ2ppdUBtdWhuLmVkdS5jbg==
Disclaimer: All claims expressed in this article are solely those of the authors and do not necessarily represent those of their affiliated organizations, or those of the publisher, the editors and the reviewers. Any product that may be evaluated in this article or claim that may be made by its manufacturer is not guaranteed or endorsed by the publisher.
Research integrity at Frontiers
Learn more about the work of our research integrity team to safeguard the quality of each article we publish.