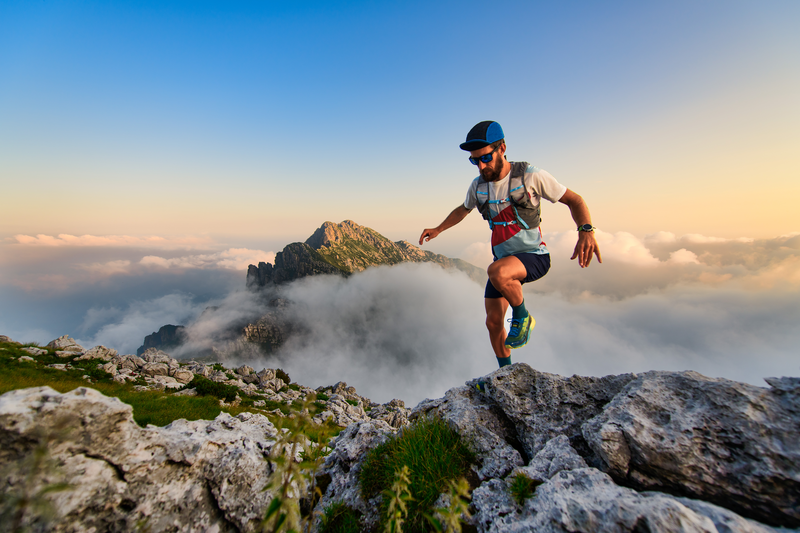
94% of researchers rate our articles as excellent or good
Learn more about the work of our research integrity team to safeguard the quality of each article we publish.
Find out more
ORIGINAL RESEARCH article
Front. Chem.
Sec. Analytical Chemistry
Volume 13 - 2025 | doi: 10.3389/fchem.2025.1549927
The final, formatted version of the article will be published soon.
You have multiple emails registered with Frontiers:
Please enter your email address:
If you already have an account, please login
You don't have a Frontiers account ? You can register here
As a kind of important inflammatory cytokines, interleukin-6 (IL-6) can mediate the entire pathological process of periodontitis and are closely associated with the degree of inflammation. Therefore, it is critical to develop convenient quantitative methods for monitoring the IL-6 amount in gingival crevicular fluid. In this study, methylene blue (MB) decorated reduced graphene oxide (rGO) are employed as signal probe and further supported the antibody enabling specifical recognition of IL-6. Due to the π-π stacking and electrostatic interactions, rGO-MB nanocomposites can be stably obtained. rGO with the characteristics of good conductivity and large surface area promotes the redox signals of MB on the glassy carbon electrode (GCE). In addition, through simple self-polymerization in situ of dopamine, the obtained polydopamine (PDA) can be not only directly used as a biological crosslinking agent for covalent immobilization of anti-IL-6 antibody, but also regarded as a protective layer to enhance the stability of rGO-MB on the GCE surface. Such designed PDA/rGO-MB/GCE based immunosensor enables the specific binding with IL-6 and produces the decreased electrochemical signal of MB, realizing the selective and sensitive quantitative measurement of IL-6. As a consequence, our fabricated PDA/rGO-MB/GCE based electrochemical immunosensor performs an excellent linear relationship with IL-6 ranging from 1 pg/mL to 100 ng/mL and the limit of detection is as low as 0.48 pg/mL. Moreover, our as-prepared sensing strategy shows accurate monitoring of IL-6 amount in gingival crevicular fluid samples.
Keywords: Methylene Blue, Reduced graphene oxide, polydopamine, Electrochemical immunosensor, IL-6
Received: 22 Dec 2024; Accepted: 03 Mar 2025.
Copyright: © 2025 Zhu, Wang and Liu. This is an open-access article distributed under the terms of the Creative Commons Attribution License (CC BY). The use, distribution or reproduction in other forums is permitted, provided the original author(s) or licensor are credited and that the original publication in this journal is cited, in accordance with accepted academic practice. No use, distribution or reproduction is permitted which does not comply with these terms.
* Correspondence:
Jiyang Liu, Zhejiang Sci-Tech University, Hangzhou, China
Disclaimer: All claims expressed in this article are solely those of the authors and do not necessarily represent those of their affiliated organizations, or those of the publisher, the editors and the reviewers. Any product that may be evaluated in this article or claim that may be made by its manufacturer is not guaranteed or endorsed by the publisher.
Research integrity at Frontiers
Learn more about the work of our research integrity team to safeguard the quality of each article we publish.