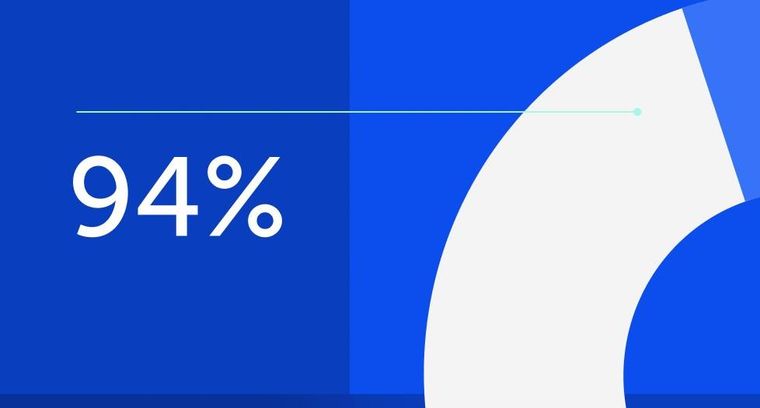
94% of researchers rate our articles as excellent or good
Learn more about the work of our research integrity team to safeguard the quality of each article we publish.
Find out more
MINI REVIEW article
Front. Chem., 19 February 2025
Sec. Analytical Chemistry
Volume 13 - 2025 | https://doi.org/10.3389/fchem.2025.1536209
This article is part of the Research TopicRecent Advances in Pharmaceutical Analysis: Applications and New Challenges for the Quality of MedicinesView all 12 articles
One way to combat the black pharmaceutical market is to exchange experience and knowledge among the laboratories involved in this fight. A beneficial approach is compiling application examples that demonstrate the development and growing potential of the two analytical techniques that are undoubtedly useful in investigating pharmacologically active ingredients found in products dangerous to consumers health and life. Attenuated total reflectance Fourier transform infrared spectroscopy and X-ray powder diffraction are nondestructive techniques substantial for examining evidence seized by the police, demanding minimal preparation of the sample. Importantly, they are among the few that do not negatively impact the environment because they do not require the production or disposal of chemical reagents or solvents, aligning with the principles of green chemistry. Both techniques provide consistent, reproducible results, essential for legal and scientific validity.
Although law enforcement agencies and analysts worldwide have been fighting against drug falsification for years, the market for falsified medications and the illegal use of pharmacologically active ingredients continues to thrive. Many of these products are sold without prescriptions on unregulated platforms, including websites, markets, and sex shops, often bypassing product control by being labeled, for example, as “not for human consumption” or “for research use only”. This opens up opportunities for misuse, as these drugs may contain incorrect or harmful ingredients or exist in various polymorphic forms, posing health risks. In many cases, they do not contain any active pharmaceutical ingredients (API) and consist only of excipients such as lactose, starch, sucrose, or mannitol. Falsified products are typically produced in poorly equipped laboratories, resulting in the presence of residual solvents and impurities (Sansone et al., 2021). Despite investigations and arrests, new manufacturing sites and distribution networks continue to emerge. Rapid identification of the composition of these products is crucial for law enforcement to intervene quickly and prevent the spread of these dangerous substances.
The structural similarities, physicochemical properties, and multicomponent matrices of these products pose substantial analytical challenges. Consequently, various techniques have been employed to determine the composition of falsified products. Common methods include chromatography, spectroscopy, titrimetry, electrochemistry, electrophoresis, nuclear magnetic resonance (NMR), and liquid chromatography combined with mass spectrometry (LC-MS) (Rao et al., 2023; Rebiere et al., 2017). High-performance liquid chromatography and ultraviolet-visible spectrophotometry are popular for their speed and precision. Titrimetric methods, such as potentiometric titration, and electrochemical techniques, such as voltammetry, are also widely used. NMR spectroscopy provides detailed molecular information (Keizers et al., 2019), while LC-MS is preferred for comprehensive drug assays and analysis of impurities and degradation products. Nondestructive methods, such as attenuated total reflectance-Fourier transform infrared spectroscopy (ATR-FTIR) and X-ray powder diffraction (XRPD), allow the identification of components that are discarded by other techniques during dissolution or filtration processes, which is an important aspect, especially in the case of excipients. This mini-review aims to display potential of both nondestructive techniques in the field of forensic science. While they share similarities, crucial differences make them complementary, enabling a comprehensive analysis of samples (Table 1).
Table 1. Similarities and differences between ATR-FTIR and XRPD in the analysis of suspected samples.
IR spectroscopy involves changes in molecular energy due to absorbed IR radiation, causing vibrational and rotational motions. Absorbed radiation increases molecular energy, resulting in a spectrum with signals or bands. The position, intensity, and shape of these absorption bands are key characteristics. These bands correspond to the functional groups in the compound, as specific groups absorb IR radiation at defined frequencies, known as group frequencies, leading to characteristic vibrations. ATR is an IR measurement mode in which a sample is applied to a high-refractive-index crystal. Key phenomena include the reflection of the electromagnetic wave at the boundary between two media and its penetration into the lower optical density medium (European Pharmacopoeia, 2021). This technique allows for direct measurement of solid and liquid samples in less than a minute.
IR spectroscopy can analyze both mixtures and individual substances. The spectrum of a mixture is distinctive and confirms product authenticity, making it useful for examining suspected falsified products. Another purpose is to monitor changes within mixtures, such as confirming synthesis progress, decomposition, or surface adsorption. IR spectroscopy can identify different polymorphic forms of the same substance. The ATR mode is applicable for analyzing thick samples with strong absorption, as well as multilayered surface coatings and coatings on solid bodies. This technique relies on durable and chemically resistant crystals suitable for powders, solids, and even liquid samples by applying a single droplet (e.g., viscous liquids, biological materials, and aqueous solutions) (Song et al., 2020).
The most frequently falsified group of drugs are those used to treat erectile dysfunction (Figure 1) (Interpol, 2023). These data are reflected in the popularity of ATR-FTIR applications for testing this group of products. In a London-based study, three purported herbal supplements for men’s sexual performance were purchased from an e-commerce platform (Ho et al., 2022). Although these products were advertised as containing only herbal ingredients, sildenafil citrate was also detected in each. This was confirmed using several techniques, including ATR-FTIR. Measurements performed using this technique confirmed the presence of bands characteristic of sildenafil citrate, corresponding to N-H stretching, N-H bending, S=O symmetrical and asymmetric stretching, C–N stretching. Two of the products were unregistered generic tablets and one was a falsified medical product.
Figure 1. Distribution of drugs into pharmacological groups of products collected during Interpol’s global Pangea XVI 2023 campaign [3].
R.S. Ortiz et al. demonstrated the application of this technique using a diamond crystal for the rapid identification of falsified medicinal products containing phosphodiesterase type 5 inhibitors (Ortiz et al., 2013). The results revealed similarities between the mixtures used to prepare various counterfeit products. Similar findings were reported in a study of the same products on samples collected by law enforcement agencies in Poland (Piorunska-Sedlak and Stypulkowska, 2021). ATR-FTIR can also be used as an auxiliary technique accompanying the main research method. Products obtained from questionable online pharmacies in the Czech Republic were examined using Raman spectroscopy and mapping, but the results of identifying the qualitative composition were additionally confirmed by measurements in infrared spectroscopy (Spalovska et al., 2021).
In falsified drugs, active ingredients often originate from illegal sources. These ingredients may differ in efficacy from the original if they are in a different salt form or may pose additional risks if they contain impurities. Even a negative result of a test confirming the identity of an API without the possibility of identification due to database limitations may constitute valuable information. P.H.J. Keizers et al. after excluding the presence of sildenafil citrate by ATR-FTIR and confirming the presence of sildenafil by LC-MS decided to take the next step. They used several additional advanced techniques, including high-resolution tandem mass spectrometry, Raman microscopy, melting point analysis, and NMR spectroscopy, to demonstrate that sildenafil in the form of a mesylate, instead of citrate, can be found as an active ingredient in the illegal market (Keizers et al., 2016).
ATR-FTIR data can be further processed using numerous computational and chemometric methods. By applying singular value decomposition to calculate the wavenumber importance index, Brito et al. proposed a novel method for wavenumber selection (Brito et al., 2020). This method aimed to classify authentic and falsified drugs containing sildenafil and tadalafil citrate. A combination of these types of proceedings was also presented by other researchers (Mittal et al., 2021). Examination and analysis of results from over 400 antibiotic samples led to the development of a classification method aimed at detecting and identifying specific solid dosage forms of antibiotics. The combination of data recorded using the attenuated total reflection technique with the partial least squares–discriminant analysis method can be a useful tool for distinguishing between less and more sophisticated falsified Durateston® products (Brazilian product containing an anabolic steroid) in a study of 96 samples (Neves et al., 2017).
IR spectroscopy can be used not only for qualitative analysis but also for quantitative analysis. A well-designed method can differentiate between substandard and falsified products. Researchers in the United Kingdom developed such a method using preparations containing paracetamol as an example (Lawson et al., 2018).
Not only synthetic drugs but also herbal medicines are subject to falsification. An Indonesian study presented an approach for the quantification and classification of herbal medicine products adulterated with synthetic drugs (prednisone and metamizole) by combining ATR-FTIR with multivariate calibration and discriminant analysis (Fatmarahmi et al., 2022).
The analysis of falsified drugs does not have to focus solely on the form of the active ingredients. Drug falsification also involves packaging, which can be used to assess the authenticity. M.R. Bin Salim et al. utilized this approach to study the blister packaging materials of paracetamol samples (Bin Salim et al., 2021). In another packaging study, a technique was used for the chemical analysis of vial label paper, flip-off caps, and cello tape on vial labels and stoppers (Degardin al., 2019). Additional techniques, such as Raman spectroscopy and microscopy, optical microscopy, and X-ray fluorescence spectroscopy, have also been employed. The combination of these tools enabled the examination of 31 falsified vials and revealed unexpected connections between counterfeits, which may be useful for supporting ongoing investigations.
IR spectroscopic identification methods are applied not only to study medicinal products but also to analyze other substances that affect the human body, including new psychoactive substances (NPS), narcotic, and psychotropic substances.
In Poland, IR spectral analysis has been applied for the rapid differentiation of structural isomers among NPS (Piorunska-Sedlak and Stypulkowska, 2020). The combination of ATR-FTIR technique and computational methods has broad applications in analyzing various other substances. J. Hughes et al. described method for the rapid identification and quantification during police operations and seizures (Hughes et al., 2013). A thoroughly developed and validated method can be used as an inexpensive, fast, and mobile alternative to expensive and labor-intensive processes. Darie et al. analyzed spectra to classify various drugs of abuse, including hallucinogens, cannabinoids, and opioids, using machine learning models, such as support vector machines and eXtreme Gradient Boosting (Darie et al., 2023). An interesting application of spectral data involves the identification and selection of compounds that can be used as surrogates for opioids in the development and training of spectroscopic sensors. Compounds such as heroin, fentanyl, and carfentanil have been studied to enhance the safety of drug enforcement officer training (Daniels et al., 2022). Chemometric models also enable the use of spectroscopic data to determine the origin of drugs based on impurity profiles, characteristic of specific synthetic pathways. McKeown et al. described the application of the orthogonal partial least squares-discriminant analysis model to the fentanyl precursors N-phenethyl-4-piperidone and 4-anilino-N-phenethylpiperidine (McKeown et al., 2023). The combination of experimental and computational approaches in the analysis of atomic locality for selected vibrational models and well-known compounds, such as variously substituted piperidine, facilitates the detection of novel derivatives in illicit drug-related samples and supports the identification of fentanyl and its analogs, including salts, in environmental samples (Shan et al., 2022). Due to the sensitivity of this technique to polymorphisms, it is often used in studies of APIs. Combined with Raman spectroscopy and computational methods, this technique has proven useful for identifying different polymorphic forms of pyrazinamide in mixtures (Zhou et al., 2024).
A different case was reported by J. Coelho Neto et al. They examined colorful tablets sold as ecstasy (MDMA) (3,4-methylenedioxymethamphetamine) and found no traces of the declared MDMA (Neto et al., 2018). However, they detected spectral bands characteristic of sildenafil. The tablets were repainted genuine Brazilian products for the treatment of erectile dysfunction.
Another application was shown in quantitative analysis. Ramsay et al. described the role of ATR-FTIR as part of a multi-technology approach used to determine fentanyl in complex mixtures containing caffeine and fentanyl in illicit opioids (Ramsay et al., 2021).
The examples mentioned show that ATR-FTIR and Raman spectroscopy are often used together. They are complementary techniques in modern vibrational spectroscopy, as they are based on different phenomena: IR absorption and Raman scattering. Raman spectroscopy detects vibrational modes that involve changes in molecular polarizability, making it effective for analyzing non-polar bonds, while IR spectroscopy identifies modes associated with changes in dipole moments, making it sensitive to polar functional groups. Therefore, together they provide a more comprehensive molecular characterization of pharmaceutical compounds, excipients, and falsified drugs by capturing different vibrational features governed by distinct selection rules (Muro et al., 2015).
Each crystalline substance is characterized by a distinct crystal system composed of regularly repeating structural elements, such as atoms, ions, and molecules. These elements form lattice planes, whose arrangement can act as a diffraction grating for radiation. The diffraction of radiation, in this case, X-rays, arises from interactions with the electron clouds of atoms. Depending on the specific arrangement of the atoms, the incident radiation can be either enhanced or diminished in the crystal lattice. The amplification condition requires that the path difference between two diffracted X-ray waves must be an integral multiple of the wavelength, a principle described by Bragg’s law (European Pharmacopoeia, 2022). When this requirement is fulfilled, strongly reflected X-rays are produced at specific angles and recorded as a function of the angle to produce diffraction patterns. The positions and intensities of the peaks can be analyzed because they are characteristic of different crystalline substances.
XRPD is a highly effective analytical technique with a long history of use. For years, it has been used to analyze and characterize crystalline structures, providing information on both active pharmaceutical ingredients and excipients. Other advantageous application of the method is detection of changes in polymorphic structure of the substance, as indicated by numerous research papers (Thakral et al., 2018; Spiliopoulou et al., 2020; Rodríguez et al., 2021). This is crucial because different polymorphic forms can have different properties that affect the bioavailability, stability, and manufacturing of API (Ainurofiq et al., 2020). Differences in polymorphic forms can be clear indicators of falsified products because legitimate drugs are manufactured under strict controls to maintain consistency in their crystalline forms. Moreover, this technique can detect variations in excipients and coatings, thereby distinguishing between genuine and counterfeit medicines. As a nondestructive analytical tool, it supports the pharmaceutical industry in quality control, regulatory compliance, and the development of novel therapeutic agents, thereby enhancing overall drug discovery and development.
XRPD offers substantial development potential for the detection and prevention of drug falsification, which is an area of growing concern in pharmaceutical regulations and consumer safety. This method provides a highly accurate means of verifying the authenticity of various medications, especially when the reference diffraction pattern of the original product is available.
XRPD is a technique used to analyze drugs for erectile dysfunction. Such investigations were evaluated in Poland, covering the topic of falsification of the well-known medication Viagra® containing sildenafil citrate. Substantial differences in diffraction patterns have been observed between authentic and falsified products (Piorunska-Sedlak and Stypulkowska, 2021; Maurin et al., 2007). Moreover, E Deconinck et al. employed chemometric models to distinguish between tadalafil and sildenafil citrate samples from different manufacturers, which can help in determining the API sources (Deconinck et al., 2022; 2023). The aforementioned London-based study also provided valuable insights into the physical form of the active ingredient, its phase transitions, and the potential impact on product performance through the use of simultaneous differential scanning calorimetry and XRPD techniques (Ho et al., 2022). Similar investigations have been carried out on other medications, for example, on the anti-ulcer medication omeprazole (Rebiere et al., 2022), and also to differentiate between genuine and counterfeit medicines claiming to contain acetylsalicylic acid and ascorbic acid (Jendrzejewska et al., 2018). These examples illustrate the broad applicability of this technique in pharmaceutical authentication.
To increase revenue, falsified drug producers frequently use cheaper alternatives for both active pharmaceutical ingredients and excipients. These alternatives may be of inferior quality, ineffective, or even hazardous, posing serious health risks to consumers. Research on drugs containing paracetamol has shown that XRPD can effectively reveal quality inconsistencies between different pharmaceutical products with the same active ingredients and distinguish polymorphic forms of paracetamol in different drug formulations (Oloyede et al., 2023; Jendrzejewska et al., 2020). This is particularly important because legitimate pharmaceuticals are produced under tightly controlled conditions to ensure that the crystalline form of the active ingredient remains consistent across all batches. Any deviation in these forms may suggest that the product is falsified or substandard. The ability of XRPD to identify these subtle yet critical differences makes it a valuable tool in fighting drug falsification.
The application of XRPD extends beyond the identification of falsified drugs. It can be applied not only to final products but also during the pre-approval drug studies. Stability studies are pivotal in the pharmaceutical industry for estimating the long-term behavior of substances. Environmental conditions such as temperature (Kumar and Jha, 2017), light exposure, and humidity (Ng et al., 2022) can cause changes in the crystalline structure of APIs and excipients, thereby affecting the stability, efficacy, and safety of medicines. Phase transitions, amorphization, and recrystallization are phenomena that may occur (Jojart-Laczkovich et al., 2016). Understanding these changes is essential for establishing proper storage conditions and identifying degradation products or impurities, which can optimize formulations. Amorphous drugs often exhibit higher solubility and dissolution rates than their crystalline counterparts (Kapoor et al., 2023).
Therefore, studies using XRPD to monitor changes in medicines are very popular, leading to many applications, such as in the field of co-amorphous formulations. M. Ruponen et al. analyzed furosemide with arginine and P-glycoprotein inhibitor drugs (Ruponen et al., 2021), while other scientists evaluated the stability of amorphous olanzapine and its co-amorphous form with saccharin (da Costa et al., 2022). There was also a Danish research conducted on co-amorphous systems consisting of naproxen, arginine, and lysine (Wostry et al., 2020). An investigation into diabetes treatment in this area showed that mixing poorly soluble nateglinide with metformin hydrochloride enhanced the dissolution rate of nateglinide. The amorphization and stabilization of this form in the mixture were confirmed, leading to increased drug release and the potential for improved therapy in patients with diabetes (Wairkar and Gaud, 2016). Other studies focused on developing inclusion complexes of meloxicam with β-cyclodextrin-based nanosponges that should enhance solubility, stability, and prolong drug release. The crystalline form of meloxicam converts into an amorphous state through complexation with nanosponges, which is a new approach in terms of drug release (Shende et al., 2015). Similar studies were conducted in Poland on inclusion complexes of ketoprofen (Betlejewska-Kielak et al., 2021) and flurbiprofen (Betlejewska-Kielak et al., 2023) with β-cyclodextrin, proving the formation of a complex.
Determining which crystalline form of the compound occurs in a given drug is crucial. In this regard, XRPD technique experiments were carried out with segesterone acetate, a drug used as a contraceptive (Aragon et al., 2021), flavonoids such as taxifolin (Terekhov et al., 2020), and antiepileptic drug levetiracetam (Xu et al., 2015). In a previously mentioned study of different polymorphic forms of pyrazinamide, XRPD was used for identification (Zhou et al., 2024).
Another interesting topic that has attracted increasing attention is the production and distribution of NPS. These substances are typically sold online and marketed as fertilizers, bath salts (Altun and Cok, 2020; Riley et al., 2020), and research chemicals (Hohmann et al., 2014; Thornton et al., 2019). XRPD can be a useful tool for identifying NPS not only in the form of pure substances but also in street mixtures containing both organic and inorganic impurities (Jurasek et al., 2019).
Attenuated total reflectance- Fourier transform infrared spectroscopy and X-ray powder diffraction are nondestructive analytical techniques that allow direct measurement of samples with minimal preparation, thus preserving their original composition. Both techniques are noninvasive, simple, and reproducible, enabling the identification of both the main and auxiliary components. The combination of the techniques enables the rapid and accurate identification of contaminants or falsified substances, including salts, co-crystals, mixtures, and various polymorphic forms (Table 2), although both techniques require access to databases or reference materials. Therefore, they are well suited for rapid screening tests and identification of known compounds registered in databases. Negative results of database searches direct further research towards the use of other techniques for identifying unknown substances or detecting low concentrations of compounds, such as LC-MS or NMR. Whereas ATR-FTIR has long been established for its speed and simplicity, the importance of XRPD has grown due to advances in miniaturization and faster analysis. They play relevant roles in confirming the identity and authenticity of products, highlighting their importance in ensuring product safety and quality across regulatory and industrial settings.
Table 2. Applications of ATR-FTIR and XRPD techniques in the study of products and compounds with pharmacological activity.
AM: Conceptualization, Formal Analysis, Investigation, Visualization, Writing–original draft. KP: Writing–review and editing, Conceptualization, Formal Analysis, Investigation, Visualization, Writing–original draft. JM: Writing–review and editing. AB: Funding acquisition, Supervision, Writing–review and editing.
The author(s) declare that financial support was received for the research, authorship, and/or publication of this article. The publication was co-financed by the state budget under the program of the Minister of Education, and Science called “Science for the Society II”, project number NdS-II/SN/0270/2023/01, amount of funding 10269 PLN; total value of the project 1956000 PLN.
The authors declare that the research was conducted in the absence of any commercial or financial relationships that could be construed as a potential conflict of interest.
The author(s) declare that no Generative AI was used in the creation of this manuscript.
All claims expressed in this article are solely those of the authors and do not necessarily represent those of their affiliated organizations, or those of the publisher, the editors and the reviewers. Any product that may be evaluated in this article, or claim that may be made by its manufacturer, is not guaranteed or endorsed by the publisher.
Ainurofiq, A., Dinda, K. E., Pangestika, M. W., Himawati, U., Wardhani, W. D., and Sipahutar, Y. T. (2020). The effect of polymorphism on active pharmaceutical ingredients: a review. Int. J. Res. Pharm. Sci. 11 (2), 1621–1630. doi:10.26452/ijrps.v11i2.2044
Altun, B., and Cok, I. (2020). Psychoactive bath salts and neurotoxicity risk. Turk. J. Pharm. Sci. 17 (2), 235–241. doi:10.4274/tjps.galenos.2018.40820
Aragon, F. F. H., Haeck, C. M., Morais, P. C., and Variano, B. (2021). Polymorphism characterization of segesterone acetate: a comprehensive study using XRPD, FT-IR and Raman spectroscopy. Int. J. Pharm. 596, 120234. doi:10.1016/j.ijpharm.2021.120234
Betlejewska-Kielak, K., Bednarek, E., Budzianowski, A., Michalska, K., and Maurin, J. K. (2021). Comprehensive characterisation of the ketoprofen-β-cyclodextrin inclusion complex using X-ray techniques and NMR spectroscopy. Molecules 26 (13), 4089. doi:10.3390/molecules26134089
Betlejewska-Kielak, K., Bednarek, E., Budzianowski, A., Michalska, K., and Maurin, J. K. (2023). Comprehensive characterisation of the flurbiprofen/β-cyclodextrin inclusion complex using X-ray techniques and NMR spectroscopy. J. Mol. Struct. 1285, 135450. doi:10.1016/j.molstruc.2023.135450
Bin Salim, M. R., Widodo, R. T., and Noordin, M. I. (2021). Fast detection of counterfeit paracetamol by the analysis of blister plastic materials using fourier transform infrared spectroscopy (FTIR) and differential scanning calorimetry (DSC). Glob. Res. J. Chem. 5 (1), 53–59.
Brito, J. B. G., Bucco, G. B., John, D. K., Ferrão, M. F., Ortiz, R. S., Mariotti, K. C., et al. (2020). Wavenumber selection based on Singular Value Decomposition for sample classification. Forensic Sci. Int. 309, 110191. doi:10.1016/j.forsciint.2020.110191
Chauhan, A., and Chauhan, P. (2014). Powder XRD technique and its applications in science and technology. J. Anal. Bioanal. Tech. 5. doi:10.4172/2155-9872.1000212
da Costa, N. F., Daniels, R., Fernandes, A. I., and Pinto, J. F. (2022). Downstream processing of amorphous and Co-amorphous olanzapine powder blends. Pharmaceutics 14 (8), 1535. doi:10.3390/pharmaceutics14081535
Daniels, G. C., Whitener, K. E., Smith, Ch. D., Giordano, B. C., and Collins, G. E. (2022). Assessment of opioid surrogates for spectroscopic testing (Part III). Forensic Chem. 30, 100443. doi:10.1016/j.forc.2022.100443
Darie, I.-F., Anton, S. R., and Praisler, M. (2023). Machine learning systems detecting illicit drugs based on their ATR-FTIR spectra. Inventions 8 56, 56. doi:10.3390/inventions8020056
Deconinck, E., Courselle, P., Raimondo, M., Grange, Y., Rebière, H., Mihailova, A., et al. (2022). GEONs API fingerprint project: selection of analytical techniques for clustering of sildenafil citrate API samples. Talanta 239, 123123. doi:10.1016/j.talanta.2021.123123
Deconinck, E., Raimondo, M., Borioni, A., Grange, Y., Rebière, H., Mihailova, A., et al. (2023). Clustering of tadalafil API samples according to their manufacturer in the context of API falsification detection. J. Pharm. Sci. 112 (11), 2834–2842. doi:10.1016/j.xphs.2023.05.015
Degardin, K., Jamet, M., Guillemain, A., and Mohn, T. (2019). Authentication of pharmaceutical vials. Talanta 198, 487–500. doi:10.1016/j.talanta.2019.01.121
European Pharmacopoeia (2021). Absorption spectrophotometry, infrared. Available at: https://pheur.edqm.eu/app/11-4/content/11-4/20224E.htm?highlight=on&terms=20224 (Accessed December 30, 2024).
European Pharmacopoeia (2022). Characterisation of crystalline solids by XRPD. Available at: https://pheur.edqm.eu/app/11-4/content/default/20933E.htm (Accessed November 22, 2024).
Fatmarahmi, D. C., Susidarti, R. A., Swasono, R. T., and Rohman, A. (2022). Application of FTIR-ATR spectroscopy in combination with multivariate analysis to analyze synthetic drugs adulterant in ternary mixtures of herbal medicine products. Indones. J. Pharm. 33 (1), 63–71. doi:10.22146/ijp.2609
Ho, H. M. K., Xiong, Z., Wong, H. Y., and Buanz, A. (2022). The era of fake medicines: investigating counterfeit medicinal products for erectile dysfunction disguised asherbal supplements. Int. J. Pharm. 617, 121592. doi:10.1016/j.ijpharm.2022.121592
Hohmann, N., Mikus, G., and Czock, D. (2014). Effects and risks associated with novel psychoactive substances: mislabeling and sale as bath salts, spice, and research chemicals. Dtsch. Ärzteblatt Int. 111 (9), 139–147. doi:10.3238/arztebl.2014.0139
Hughes, J. M., Ayoko, G., Collett, S., and Golding, G. (2013). Rapid quantification of methamphetamine: using attenuated total reflectance fourier transform infrared spectroscopy (ATR-FTIR) and chemometrics. PLOS ONE 8 (7), e69609. doi:10.1371/journal.pone.0069609
INTERPOL (2023). Global illicit medicines targeted by INTERPOL operation. Available at: https://www.interpol.int/News-and-Events/News/2023/Global-illicit-medicines-targeted-by-INTERPOL-operation (Accessed October 29, 2024).
Jendrzejewska, I., Goryczka, T., Pietrasik, E., Klimontko, J., and Jampilek, J. (2020). X-Ray and thermal analysis of selected drugs containing acetaminophen. Molecules 25 (24), 5909. doi:10.3390/molecules25245909
Jendrzejewska, I., Zajdel, P., Pietrasik, E., Barsova, Z., and Goryczka, T. (2018). Application of X-ray powder diffraction and differential scanning calorimetry for identification of counterfeit drugs. Monatsh Chem. 149, 977–985. doi:10.1007/s00706-018-2193-z
Jojart-Laczkovich, O., Katona, G., Aigner, Z., and Szabo-Revesz, P. (2016). Investigation of recrystallization of amorphous trehalose through hot-humidity stage X-ray powder diffraction. Eur. J. Pharm. Sci. 95, 145–151. doi:10.1016/j.ejps.2016.08.003
Jurasek, B., Bartunek, V., Huber, S., and Kuchar, M. (2019). X-Ray powder diffraction - a non-destructive and versatile approach for the identification of new psychoactive substances. Talanta 195, 414–418. doi:10.1016/j.talanta.2018.11.063
Kapoor, D. U., Singh, S., Sharma, P., and Prajapati, B. G. (2023). Amorphization of low soluble drug with amino acids to improve its therapeutic efficacy: a state-of-art-review. AAPS PharmSciTech 24 (8), 253. doi:10.1208/s12249-023-02709-2
Keizers, P. H. J., Bakker, F., Ferreira, J., Wackers, P. F. K., van Kollenburg, D., van der Aa, E., et al. (2019). Benchtop NMR spectroscopy in the analysis of substandard and falsified medicines as well as illegal drugs. J. Pharm. Biomed. 178, 112939. doi:10.1016/j.jpba.2019.112939
Keizers, P. H. J., Wiegard, A., Bastiaan, J., and Venhuis, B. J. (2016). The quality of sildenafil active substance of illegal source. J. Pharm. Biomed. 131, 133–139. doi:10.1016/j.jpba.2016.08.027
Kumar, N., and Jha, A. (2017). Temperature excursion management: a novel approach of quality system in pharmaceutical industry. Saudi Pharm. J. 25 (2), 176–183. doi:10.1016/j.jsps.2016.07.001
Lawson, G., Ogwu, J., and Tanna, S. (2018). Quantitative screening of the pharmaceutical ingredient for the rapid identification of substandard and falsified medicines using reflectance infrared spectroscopy. PLOS ONE 13 (8), e0202059. doi:10.1371/journal.pone.0202059
Maurin, J. K., Plucinski, F., Mazurek, A. P., and Fijalek, Z. (2007). The usefulness of simple X-ray powder diffraction analysis for counterfeit control—the Viagra® example. J. Pharm. Biomed. 43 (4), 1514–1518. doi:10.1016/j.jpba.2006.10.033
McKeown, H. E., Rook, T. J., Pearson, J. R., and Jones, O. A. H. (2023). Investigations into fentanyl precursors method classification by handheld Fourier transform infrared and Raman spectroscopy combined with multivariate statistical analysis. Forensic Chem. 33, 100476. doi:10.1016/j.forc.2023.100476
Mittal, M., Sharma, K., and Rathore, A. (2021). Checking counterfeiting of pharmaceutical products by attenuated total reflection mid-infrared spectroscopy. Spectroc. Acta Pt. A-Molec. Biomolec. Spectr. 255, 119710. doi:10.1016/j.saa.2021.119710
Muro, C. K., Doty, K. C., Bueno, J., Halámková, L., and Lednev, I. K. (2015). Vibrational spectroscopy: recent developments to revolutionize forensic science. Anal. Chem. 87 (1), 306–327. doi:10.1021/ac504068a
Neto, J. C., Faraco, R. F. P., Alves, C. F., Castro, S. M. M., and Machado, Y. (2018). Genuine sildenafil tablets sold in Brazil disguised as MDMA. Forensic Sci. Int. 283, e8–e12. doi:10.1016/j.forsciint.2017.12.006
Neves, D. B., Talhavini, M., Braga, J. W., Zacca, J. J., and Caldas, E. D. (2017). Detection of counterfeit Durateston using Fourier transform infrared spectroscopy and partial least squares: discriminant analysis. J. Braz. Chem. Soc. 28, 1288–1296. doi:10.21577/0103-5053.20160293
Ng, L. H., Ling, J. K. U., and Hadinoto, K. (2022). Formulation strategies to improve the stability and handling of oral solid dosage forms of highly hygroscopic pharmaceuticals and nutraceuticals. Pharmaceutics 14 (10), 2015. doi:10.3390/pharmaceutics14102015
Oloyede, O. O., Alabi, Z. O., Akinyemi, A. O., Oyelere, S. F., Oluseye, A. B., and Owoyemi, B. C. D. (2023). Comparative evaluation of acetaminophen form (I) in commercialized paracetamol brands. Sci. Afr. 19, e01537. doi:10.1016/j.sciaf.2022.e01537
Ortiz, R. S., de Cássia Mariotti, K., Fank, B., Limberger, R. P., Anzanello, M. J., and Mayorga, P. (2013). Counterfeit Cialis and Viagra fingerprinting by ATR-FTIR spectroscopy with chemometry: can the same pharmaceutical powder mixture be used to falsify two medicines? Forensic Sci. Int. 226 (1–3), 282–289. doi:10.1016/j.forsciint.2013.01.043
Piorunska-Sedlak, K., and Stypulkowska, K. (2020). Strategy for identification of new psychoactive substances in illicit samples using attenuated total reflectance infrared spectroscopy. Forensic Sci. Int. 312, 110262. doi:10.1016/j.forsciint.2020.110262
Piorunska-Sedlak, K., and Stypulkowska, K. (2021). Selectivity of identification of compounds from the group of phosphodiesterase-5 inhibitors (PDE-5i) in falsified products from the Polish market using attenuated total reflectance Fourier transform infrared spectroscopy and X-ray powder diffraction. Sci. and Justice 61 (6), 714–722. doi:10.1016/j.scijus.2021.08.008
Ramsay, M., Gozdzialski, L., Larnder, A., Wallace, B., and Hore, D. (2021). Fentanyl quantification using portable infrared absorption spectroscopy. A framework for community drug checking. Vib. Spectrosc. 114, 103243. doi:10.1016/j.vibspec.2021.103243
Rao, A. S., Dileep, B., and Madhusudhan, B. (2023). A review on “pharmaceutical analysis in the modern era: advanced analytical methods”. J. Nov. Res. Devel. 8 (10), a876–a881.
Rebiere, H., Grange, Y., Deconinck, E., Courselle, P., Acevska, J., Brezovska, K., et al. (2022). European fingerprint study on omeprazole drug substances using a multi analytical approach and chemometrics as a tool for the discrimination of manufacturing sources. J. Pharm. Biomed. 208, 114444. doi:10.1016/j.jpba.2021.114444
Rebiere, H., Guinot, P., Chauvey, D., and Brenier, Ch. (2017). Fighting falsified medicines: the analytical approach. J. Pharm. Biomed. 142, 286–306. doi:10.1016/j.jpba.2017.05.010
Riley, A. L., Nelson, K. H., To, P., Lopez-Arnau, R., Xu, P., Wang, D., et al. (2020). Abuse potential and toxicity of the synthetic cathinones (i.e., “Bath salts”). Neurosci. Biobehav. Rev. 110, 150–173. doi:10.1016/j.neubiorev.2018.07.015
Rodríguez, I., Gautam, R., and Tinoco, A. D. (2021). Using X-ray diffraction techniques for biomimetic drug development, formulation, and polymorphic characterization. Biomimetics 6 (1), 1. doi:10.3390/biomimetics6010001
Ruponen, M., Kettunen, K., Santiago Pires, M., and Laitinen, R. (2021). Co-amorphous formulations of furosemide with arginine and P-glycoprotein inhibitor drugs. Pharmaceutics 13 (2), 171. doi:10.3390/pharmaceutics13020171
Sansone, A., Cuzin, B., and Jannini, E. A. (2021). Facing counterfeit medications in sexual medicine. A systematic scoping review on social strategies and technological solutions. Sex. Med. 9 (6), 100437. doi:10.1016/j.esxm.2021.100437
Shan, X., Lee, L., Clewes, R. J., Howle, Ch. R., Sambrook, M. R., and Clary, D. C. (2022). Computational analyses of the vibrational spectra of fentanyl, carfentanil and remifentanil. Spectroc. Acta Pt. A-Molec. Biomolec. Spectr. 270, 120763. doi:10.1016/j.saa.2021.120763
Shende, P. K., Gaud, R. S., Bakal, R., and Patil, D. (2015). Effect of inclusion complexation of meloxicam with β-cyclodextrin- and β-cyclodextrin-based nanosponges on solubility, in vitro release and stability studies. Colloids Surf. B Biointerfaces 136, 105–110. doi:10.1016/j.colsurfb.2015.09.002
Song, Y., Cong, Y., Wang, B., and Zhang, N. (2020). Applications of Fourier transform infrared spectroscopy to pharmaceutical preparations. Expert Opin. Drug Deliv. 17 (4), 551–571. doi:10.1080/17425247.2020.1737671
Spalovska, D., Pekarek, T., Kuchar, M., and Setnicka, V. (2021). Comparison of genuine, generic and counterfeit Cialis tablets using vibrational spectroscopy and statistical methods. J. Pharm. Biomed. 206, 114383. doi:10.1016/j.jpba.2021.114383
Spiliopoulou, M., Valmas, A., Triandafillidis, D.-P., Kosinas, C., Fitch, A., Karavassili, F., et al. (2020). Applications of X-ray powder diffraction in protein crystallography and drug screening. Crystals 10 (2), 54. doi:10.3390/cryst10020054
Terekhov, R. P., Selivanova, I. A., Tyukavkina, N. A., Ilyasov, I. R., Zhevlakova, A. K., Dzuban, A. V., et al. (2020). Assembling the puzzle of taxifolin polymorphism. Molecules 25 (22), 5437. doi:10.3390/molecules25225437
Thakral, N. K., Zanon, R. L., Kelly, R. C., and Thakral, S. (2018). Applications of powder X-ray diffraction in small molecule pharmaceuticals: achievements and aspirations. J. Pharm. Sci. 107 (12), 2969–2982. doi:10.1016/j.xphs.2018.08.010
Thornton, S. L., Darracq, M. A., Gugelmann, H. M., and Armenian, P. (2019). Surface internet marketplace presence and availability of NPS sold as research chemicals: a snapshot study. Toxicol. Commun. 3 (1), 67–74. doi:10.1080/24734306.2019.1648067
Wairkar, S., and Gaud, R. (2016). Co-amorphous combination of nateglinide-metformin hydrochloride for dissolution enhancement. AAPS PharmSciTech 17, 673–681. doi:10.1208/s12249-015-0371-4
Wostry, M., Plappert, H., and Grohganz, H. (2020). Preparation of Co-amorphous systems by freeze-drying. Pharmaceutics 12 (10), 941. doi:10.3390/pharmaceutics12100941
Xu, K., Xiong, X., Guo, L., Wang, L., Li, S., Tang, P., et al. (2015). An investigation into the polymorphism and crystallization of levetiracetam and the stability of its solid form. J.Pharm. Sci. 104 (12), 4123–4131. doi:10.1002/jps.24628
Keywords: falsified medicinal products, illegal substances, API, analytical chemistry, FTIR, ATR, XRPD
Citation: Mocarska A, Piorunska K, Maurin JK and Blazewicz A (2025) The usefulness of infrared spectroscopy and X-ray powder diffraction in the analysis of falsified, illegal, and medicinal products. Front. Chem. 13:1536209. doi: 10.3389/fchem.2025.1536209
Received: 28 November 2024; Accepted: 03 February 2025;
Published: 19 February 2025.
Edited by:
Anna Borioni, National Institute of Health (ISS), ItalyReviewed by:
Mariangela Raimondo, National Institute of Health (ISS), ItalyCopyright © 2025 Mocarska, Piorunska, Maurin and Blazewicz. This is an open-access article distributed under the terms of the Creative Commons Attribution License (CC BY). The use, distribution or reproduction in other forums is permitted, provided the original author(s) and the copyright owner(s) are credited and that the original publication in this journal is cited, in accordance with accepted academic practice. No use, distribution or reproduction is permitted which does not comply with these terms.
*Correspondence: Karolina Piorunska, ay5waW9ydW5za2FAbmlsLmdvdi5wbA==
Disclaimer: All claims expressed in this article are solely those of the authors and do not necessarily represent those of their affiliated organizations, or those of the publisher, the editors and the reviewers. Any product that may be evaluated in this article or claim that may be made by its manufacturer is not guaranteed or endorsed by the publisher.
Research integrity at Frontiers
Learn more about the work of our research integrity team to safeguard the quality of each article we publish.