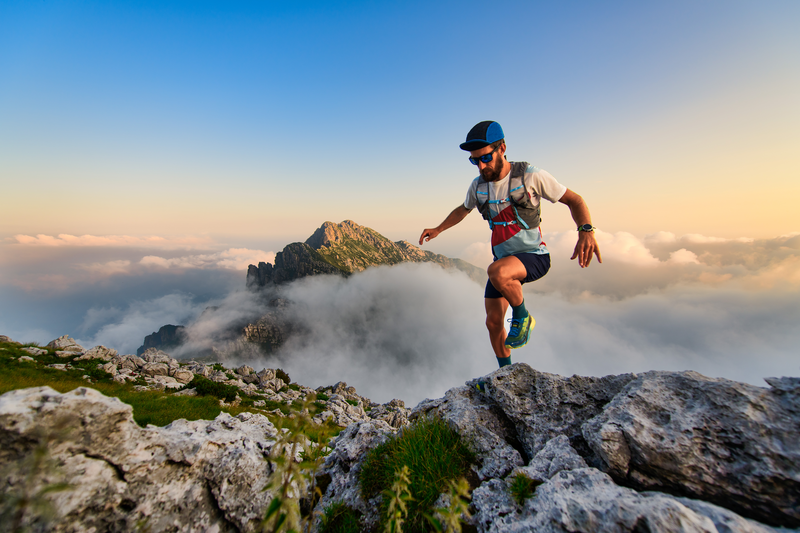
94% of researchers rate our articles as excellent or good
Learn more about the work of our research integrity team to safeguard the quality of each article we publish.
Find out more
ORIGINAL RESEARCH article
Front. Chem. , 25 November 2024
Sec. Molecular Liquids
Volume 12 - 2024 | https://doi.org/10.3389/fchem.2024.1508396
This article is part of the Research Topic Intermolecular Interaction Studies in Binary and Higher Order Liquid Mixtures, Ionic Liquids and Deep Eutectic Solvents (DES) Based Systems View all 5 articles
Active Pharmaceutical Ingredients-Ionic liquids (API-ILs) can increase drug solubility and bioavailability of solid drugs without changing the structure of drug molecules. In the present work, nonivamide (pelargonic acid vanillylamide, PAVA) was used as the active drug and choline (Ch) and citric acid (CA) were selected as the ions to prepare PAVA–based ionic liquid ([Ch][PAVA] and [PAVA]3 [CA]), respectively. The characterization and physical properties of [Ch][PAVA] and [PAVA]3 [CA], such as FT–IR spectra, 1H NMR spectra, thermal stability and hydrophilicity, were investigated. And the irritant effects of the PAVA–based ionic liquids were measured by the animal irritation experiment. [Ch][PAVA] has higher decomposition temperatures, better hydrophilicity and comparable irritant effects. The results show that the PAVA–based ILs synthesized in this study may potentially serve as a promising novel riot control agent.
Nonivamide (PAVA; CAS registry number: 2444–46–4; Figure 1), known as synthetic capsaicin, is a capsaicinoid that exhibits various biological and pharmacological effects and has a wide range of applications in medicine (Constant et al., 1996; Rottke et al., 2014; Heck et al., 2017), health supplements (Rosa et al., 2009), pesticides (Kong et al., 2013), especially riot control agents (Reilly et al., 2001; Patocka and Kuca, 2011; Satpute et al., 2018; Mörén et al., 2024). PAVA exerts strong irritant effects on the eyes and upper respiratory tract. Upon contact with the human body, it immediately causes lacrimation, involuntary eye closure, coughing, sneezing, and chest pain, resulting in temporary incapacitation. Thus, the novel irritant has been widely used as an effective and safe incapacitating agent against violence by law enforcement agencies and police departments in many countries around the world (Reilly et al., 2001; Bettencourt da Silva et al., 2014; Wang et al., 2017; Antonio et al., 2019; Zhai and Cui, 2021).
Capsaicin–based incapacitating agents are typically deployed in two forms, namely liquid aerosol or ultrafine solid powder (Reilly et al., 2001; Luo and Wang, 2011; Bettencourt da Silva et al., 2014; Zhai and Cui, 2021). However, PAVA has extremely low hydrophilicity and can be considered practically insoluble in water (Lei et al., 2010; Yan et al., 2012; Cao et al., 2014), and the way for its application in tar gas liquid is adding considerable amounts of organic solubilizers and surfactants to boost the solubility of PAVA (Reilly et al., 2001; Bettencourt da Silva et al., 2014; Wang et al., 2017). The resultant has several drawbacks such as high production cost, low degree of environmental friendliness, and other environmental consequences that are difficult to address. Therefore, enhancing the hydrophilicity of capsaicinoids is a key technical hurdle that must be overcome for the preparation of environment–friendly, high–concentration, water–based formulations of PAVA.
Ionic liquids (ILs) are “designer liquids” composed entirely of organic cations and organic/inorganic anions (Zhang et al., 2010; Hu and Peng, 2014; Sosnowska et al., 2014; Mir et al., 2024). ILs are classified as green chemical solvents owing to characteristics such as flame retardancy, non–volatility, good chemical and thermal stability, and environmental friendliness (Sosnowska et al., 2014; Qu et al., 2022). In recent years, API-ILs have been regarded as powerful yet safe tools that can assist in overcoming the issues of low solubility and poor bioavailability in insoluble drugs (Ford et al., 2020; Lu et al., 2021; Gao and Shu, 2023; Shamshina and Rogers, 2023; Zhuo et al., 2024).
In this study, highly water–soluble choline cations ([Ch]+) were introduced into the IL molecules of PAVA to improve the hydrophilicity of PAVA via electrostatic and hydrogen bond interactions. Choline cations were selected as the ideal cations due to their superb hydrophilicity, permeability, and biocompatibility. Moreover, choline is generally recognized as safe (GRAS) by the United States Food and Drug Administration (USFDA (Sosnowska et al., 2014)). In addition, IL synthesis was also performed with citric acid (CA) and PAVA to compare the effects on the hydrophilicity of PAVA. Compared with PAVA, the solubility of [Ch][PAVA] was significantly increased. Furthermore, diluted solutions of the synthesized ILs exhibited irritant effects comparable to those of PAVA and capsaicin. Therefore, the PAVA–based ILs synthesized in this study may potentially serve as a promising novel irritant material.
The following reagents were used: PAVA, >98% purity (Shanghai yuanye Bio-Technology Co., Ltd., China); ethanol, analytical reagent (AR) grade (Beijing Chemical Works, China); choline hydroxide (50 wt% aqueous solution) and CA (Shanghai Macklin Biochemical Co., Ltd., China); distilled water (Hangzhou Wahaha Group Co., Ltd., China); Capsaicin, containing 60% capsaicin and 35% dihydrocapsaicin as active ingredients (Tianjin Heowns Biochemical Co., Ltd., China).
Dunkin Hartley guinea pigs (50%/50% male and female) weighing 200–300 g were purchased from Huafukang Beijing Bioscience Co., Ltd (Beijing, China). The guinea pigs were provided with an appropriate growth environment with adequate water and food. All animal experimentation was performed hewing to the Guidelines for the Care and Use of Experimental Animals, and was authorized by the State Key Laboratory of NBC Protection for Civilians under permission No. LAE-2023–03–002.
Synthesis of the [Ch][PAVA] and [PAVA]3 [CA] were performed as previously reported (Lu et al., 2021). PAVA powder (29.34 g, 0.1 mol) was dissolved in anhydrous ethanol (30 g) at about 30°C. Under conditions of vigorous stirring 1,000 rpm and 30°C, an equivalent number of moles of choline hydroxide (50 wt% aqueous solution) or an one-third number of moles of CA (20 wt% aqueous solution) was added to the PAVA–ethanol system and reacted under continuous stirring at room temperature for 24 h. Subsequently, ethanol and water were removed from the solution by rotary evaporation for 3 h at 65°C, and a brownish–yellow viscous IL ([Ch][PAVA] or [PAVA]3 [CA]) was obtained.
Fourier transform infrared (FT–IR) spectra were recorded on a Mettler Toledo Nicolet 6700 FT–IR spectrometer with a wavelength range of 400–4,000 cm−1.
1H nuclear magnetic resonance (NMR) spectra were collected in Bruker 600 MHz NMR spectrometer, with DMSO–d6 and D2O separately used as solvents.
Elemental analysis were measured using a elementar analyzer UNICUBE, with measurements performed using O2 atmosphere at 1,000°C for elements CHSN and He atmosphere for element O.
Thermogravimetric analysis (TGA) spectra were measured using a Mettler Toledo thermogravimetric analyzer/differential scanning calorimeter (TGA/DSC) 2, with measurements performed using a heating rate of 10°C min−1 and in an air atmosphere.
Contact angles were measured using a Dataphysics OCA20 contact angle system. Each sample was first preheated in a 70°C water bath for the enhancement of fluidity, and the preheated sample was subsequently added dropwise onto a microscopic slide and subjected to contact angle measurement after cooling to room temperature.
The degree of intensity of the irritant reactions of guinea pigs to different samples of identical concentration was measured to determine the strength of irritant effects. Experimental animals were placed in a viewable container. Identical volumes of each sample solution were separately sprayed onto the face of each animal, and the total number of face–scratching episodes over a fixed duration was recorded to observe the irritant responses of the animals (Szolcsányi and JancsóGábor, 1975). To compare the irritant effects of different irritant agents, 10% ethanol solutions of [Ch][PAVA], [PAVA]3 [CA], PAVA, and natural capsaicin (caps) were formulated for a comparative experiment performed on 16 guinea pigs (equal numbers of males and females) randomly divided into four groups.
In the present study, the [Ch][PAVA] IL was synthesized by simple mixing of equivalent numbers of moles of PAVA and choline hydroxide in ethanol solvent. Figure 2 shows the pathway of [Ch][PAVA] synthesis.
The IR spectra (Figure 3) show the presence of the characteristic peak of the NH group of secondary amides in PAVA at 3,441 cm−1 and fingerprint peaks at 1,531 cm−1 and 752 cm−1. However, all three peaks were not observed in the IR spectrum of [Ch][PAVA] and [PAVA]3 [CA]. This is attributed to the fact that the NH group of secondary amides in PAVA is highly susceptible to replacement reactions and thus readily reacted during the IL synthesis process (see Figure 2). The sharp peak belonging to the phenolic hydroxyl group in PAVA at 3,283 cm−1 became wider in [Ch][PAVA] and [PAVA]3 [CA], possibly due to the formation of hydrogen bonds between phenolic hydroxyl groups and cations. Peak locations in the fingerprint region of [Ch][PAVA] were shifted compared with those of PAVA, which may be caused by the substantial influence of cation-anion interactions on functional groups.
Based on the comparison of the 1H NMR spectra of PAVA and [Ch][PAVA] (Figure 4), it can be clearly seen that the chemical shifts of 8.80 and 8.15, which respectively represent the phenolic hydroxyl (–OH) and amide (–NH–) groups in PAVA, were absent in the NMR spectrum of [Ch][PAVA]. This may be due to the reaction of–OH and–NH–or the activation of H on the functional groups, leading to disappearance from the spectrum. Additionally, we observed a certain bias in the shifts of other functional groups on PAVA, which may be attributed to the effects caused by cation and anion formation. In the NMR spectrum of [PAVA]3 [CA], in addition to the disappearance of the shifts of–OH, –NH–, and the carboxyl group (–COOH), there were less changes in the chemical shift of the other functional groups than the [Ch][PAVA]. A possible explanation is the presence of weaker interactions within [PAVA]3 [CA] than the [Ch][PAVA], which exerted smaller effects on the functional groups.
For [Ch][PAVA], 1H-NMR peaks 19 and 2 respectively represent N-CH2 and ArCH3 in [Ch]+ and [PAVA]‒ and the area proportion of them is 2:3, which show that the proportion of cation to anion is 1:1. For [PAVA]3 [CA], 1H-NMR peaks 2 and 24 respectively represent Ar-OCH3 and C-CH2 in [PAVA]+ and [CA]3‒ and the area proportion of them is 3:0.67, which show that the proportion of cation to anion is 3:1.
The elemental analysis data of [Ch][PAVA] and [PAVA]3 [CA], including N, C, H, and O, is shown in Table 1. It can be seen that the measured data of element content for each ionic liquid is basically consistent with the theoretical values. Therefore, elemental analysis characterization can further demonstrate the proportions of cation:anion for prepared [Ch][PAVA] and [PAVA]3 [CA].
The TGA graphs indicate that [Ch][PAVA] and [PAVA]3 [CA] had relatively higher thermal stability, exhibiting decomposition temperatures (Td) of 181.98°C and 206.05°C, respectively (Figure 5). Compared with pure PAVA, the weights of [Ch][PAVA] and [PAVA]3 [CA] slightly decreased when the temperature increased from 100°C to 150°C because of water evaporation. This is mainly explained by the fact that salt–based ILs readily absorb moisture from the air. Below 600°C, both [Ch][PAVA] and [PAVA]3 [CA] exhibited two–step thermal decomposition within the temperature ranges of 150°C–250°C and 250°C–500°C. The first thermal decomposition step may be attributed to the generation of PAVA from the decomposition of the PAVA–based IL. This led to mass reductions of 26% and 20%, respectively, which were comparable to the mass fractions of choline and CA in the ILs. The second thermal decomposition step involved the decomposition of PAVA. Changes in the decomposition temperatures of PAVA revealed that PAVA decomposition temperatures after IL formation were 326.01°C and 336.94°C, respectively, which were both higher than that of single–compound PAVA (290.68°C). Therefore, it can be inferred that ILs of PAVA will exhibit better anti–thermal decomposition performance than single–compound PAVA during heat dissipation processes such as explosions.
Contact angles were measured using water as the test liquid to determine the degree of hydrophilicity of the compounds. As shown in Figure 6, a large contact angle of approximately 65.5° was reached at 0 s for pure PAVA, which was mainly due to its strong hydrophobicity. PAVA has a relatively high molecular weight and cohesive energy and possesses a structure containing both hydrophobic octyl side chains and phenolic and amide groups that provide multiple sites for oxygen bond reactions. The unique backbone and functional groups of PAVA, combined with the complex inter–and intra–molecular interactions along with the spatial arrangement and configuration that they induce, contribute to the poor solubility of PAVA, with extremely low solubilities in water and organic solvents containing nonpolar or weakly polar simple molecules. However, the introduction of the strongly hydrophilic choline structure to form the [Ch][PAVA] IL resulted in a significantly smaller contact angle under identical conditions. The water contact angle of [Ch][PAVA] was approximately 33.1° at 0 s and reached 8° at 5 s, which was markedly lower than those of [PAVA]3 [CA] (approximately 71.8° at 0 s) and PAVA (approximately 65.5° at 0 s). Further analysis revealed that the water contact angle of [Ch][PAVA] decreased linearly in a time–dependent manner, with the water droplet completely penetrating the sample within 10 s (Figures 6, 7). These results demonstrate the high affinity of [Ch][PAVA] for water.
Figure 6. Digital image showing contact angles of water droplets on PAVA, [PAVA]3 [CA], and [Ch][PAVA].
[Ch][PAVA] contains a quaternary ammonium cation structure, which has certain ionization and strong polarity. It can interact with polar solvents such as water, enhance intermolecular electrostatic forces, and form stronger hydrogen bonds. Therefore, [Ch][PAVA] containing a quaternary ammonium cation structure has significantly higher solubility in polar solvent water than [PAVA]. The water solubility of [PAVA]3 [CA] is worse than that of PAVA which maybe due to the hydrophilic groups of [PAVA]3 [CA] less than PAVA and CA and the hydrophilic groups of PAVA and CA formed hydrogen bonds or other interactions with each other. And this is maybe why CA used as a material for hydrophobic modification.
The calculation results (Table 2) indicate that preparation of ILs using PAVA increased the water solubility of PAVA to a certain extent. It can be deduced that the distribution of PAVA in the aqueous phase was enhanced through electrostatic and hydrogen bond interactions after [Ch][PAVA] formation, which contributed to the increased hydrophilicity. The structure of [Ch][PAVA] shows the formation of cation–anion pairs between PAVA and quaternary ammonium salts, which enabled the continuous existence of [PAVA]‒. However, the solubility of [PAVA]3 [CA] is worse than PAVA, maybe resulting in a weakly hydrophilic and space effect of CA.
Figure 8 shows that pure PAVA powder and [PAVA]3 [CA] were insoluble in water and formed significant sedimentation and stratification. However, [Ch][PAVA] was uniformly dispersed in water (Figure 8C) without separation, forming a transparent, stable solution. This result is highly consistent with the results of the contact angle experiment and that may be attributed to the strong hydrophilicity of [Ch]+.
We also explored the solubility of [Ch][PAVA] with PAVA concentrations decreasing from 50wt% to 0.01wt%, and the results are shown in Figure 9. It can be seen that the 0.02wt% aqueous solution of PAVA remains clear and transparent, indicating that [Ch][PAVA] is much more soluble than PAVA powder in water. At the same time, the dissolution of [Ch][PAVA] in water showed good solubility at both low and high concentrations. This interesting phenomenon may be the result of competition interaction between [PAVA]‒ and water and it can be explained set on excess enthalpy. The reported experimental data state that the thermodynamic quantities of mixing could provide valuable insight into molecular interactions and microscopic structures in such mixtures (Ficke et al., 2010; Podgoršek et al., 2016; Ma et al., 2018). And some (ionic liquids + water) mixtures show an S-shaped curve dependence of ΔmixH with composition with positive enthalpies of mixing for compositions poor in ionic liquids. When the concentration of [Ch][PAVA] is high, the interaction between [Ch]+ and [PAVA]− is strong and cannot be completely destroyed, the H2O molecules mix well with IL with strong interactions (van der Waals, H-bonds, electrostatic, etc.), and H2O is embedded inside and at the periphery of the IL without any H2O clusters formed and it likes water soluble in [Ch][PAVA]. With a further addition of H2O, the IL clusters start to dissociate into individual ions or ion-pairs and the dissociated ions are gradually hydrated. When the concentration of [Ch][PAVA] is extremely low, the IL structures are completely dissociated as ions. Depending on the size of the IL-ions, some of them are fully hydrated, while some are partially hydrated and [Ch]+ are surrounded by water molecules, greatly weakening the interaction of [Ch]+ and [PAVA]‒, which resulting in poor solubility of ionic liquids in the system.
Figure 9. Dissolution of of [Ch] [PAVA] with different PAVA concentrations. (A) Newly prepared solution. (B) Solution after 72 h.
As shown in Figure 10, [PAVA], [Ch][PAVA], [PAVA]3 [CA], and capsaicin all have strong irritant responses in the animals, which included violent face–scratching, squinting, sneezing, and tachypnea. The experiment observed two time periods of 1 min and 5 min, and the number of face scratching episodes observed in the animals were statistically analyzed. Within 1 min, with PAVA sample as the control group, there was a significant difference between the [Ch][PAVA] sample (P < 0.05) and the [PAVA]3 [CA] sample (P < 0.05), while there was no significant difference in the capsaicin sample (P > 0.05). Within 5 min, there was a significant difference between the [PAVA]3 [CA] sample (P< 0.05), while there was no significant difference between the [Ch][PAVA] sample (P > 0.05) and the capsaicin sample (P > 0.05). Compared with the PAVA and capsaicin samples at identical concentrations, the [Ch][PAVA] and [PAVA]3 [CA] samples exhibited irritant effects that were comparable or even stronger.
In this study, we proposed a method for the introduction of readily water–soluble ions into PAVA to form ILs. Two different types of ions, namely [Ch]+ and [CA]3‒, were introduced to compare the effects of different ions on PAVA properties. The [Ch][PAVA] IL exhibited the best dissolution performance among the various test compounds. Our results indicated that the introduction of highly water–soluble choline cations into the PAVA IL improved the hydrophilicity of PAVA. Upon the formation of an IL, the distribution of PAVA in the aqueous phase could be improved through the presence of electrostatic and hydrogen bond interactions, thereby enhancing its hydrophilicity. When diluted solutions of the prepared ILs were used in an animal irritation experiment, the synthesized ILs exhibited irritant effects comparable to those of PAVA and natural capsaicin when using identical concentrations. Therefore, the PAVA–based ILs synthesized in this study may potentially serve as a promising novel riot control agent.
The original contributions presented in the study are included in the article, further inquiries can be directed to the corresponding author.
The animal study was approved by State Key Laboratory of NBC Protection for Civilians. The study was conducted in accordance with the local legislation and institutional requirements.
WM: Conceptualization, Data curation, Formal Analysis, Investigation, Methodology, Writing–original draft, Writing–review and editing. HW: Conceptualization, Data curation, Formal Analysis, Investigation, Methodology, Writing–review and editing. ZW: Data curation, Formal Analysis, Writing–review and editing. DC: Investigation, Writing–review and editing. LY: Data curation, Formal Analysis, Writing–review and editing. LQ: Investigation, Writing–review and editing. ZM: Investigation, Writing–review and editing.
The author(s) declare that no financial support was received for the research, authorship, and/or publication of this article.
The authors declare that the research was conducted in the absence of any commercial or financial relationships that could be construed as a potential conflict of interest.
The author(s) declare that no Generative AI was used in the creation of this manuscript.
All claims expressed in this article are solely those of the authors and do not necessarily represent those of their affiliated organizations, or those of the publisher, the editors and the reviewers. Any product that may be evaluated in this article, or claim that may be made by its manufacturer, is not guaranteed or endorsed by the publisher.
Antonio, A. S., Fonseca, G. D., Mendes, Á. J. B., Wiedemann, L. S. M., and Veiga-Junior, V. F. (2019). Perspectives and applications of natural products as civilian defense devices. J. Braz. Chem. Soc. 30, 1115–1129. doi:10.21577/0103-5053.20190028
Bettencourt da Silva, R. J. N., Silveira, D. M., Camões, M. F. G. F. C., Borges, C. M. F., and Salgueiro, P. A. S. (2014). Validation, uncertainty, and quality control of qualitative analysis of tear gas weapons by gas chromatography-mass spectrometry. Anal. Lett. 47, 250–267. doi:10.1080/00032719.2013.832274
Cao, Y., Yang, Q., Xing, H., Bao, Z., Su, B., Yang, Y., et al. (2014). Determination and correlation of solubility of nonivamide in different solvents. Chin. J. Chem. Eng. 22, 1141–1144. doi:10.1016/j.cjche.2013.05.001
Constant, H. L., Cordell, G. A., and West, D. P. (1996). Nonivamide, a constituent of capsicum oleoresin. J. Nat. Prod. 59, 425–426. doi:10.1021/np9600816
Ficke, L. E., Novak, R. R., and Brennecke, J. F. (2010). Thermodynamic and thermophysical properties of ionic liquid + water systems. J. Chem. Eng. Data 55, 4946–4950. doi:10.1021/je100522z
Ford, L., Tay, E., Nguyen, T. H., Williams, H. D., Porter, C. J. H., Scammells, P. J., et al. (2020). API ionic liquids: probing the effect of counterion structure on physical form and lipid solubility. RSC Adv. 10, 12788–12799. doi:10.1039/d0ra00386g
Gao, Y., and Shu, Y. (2023). Antibacterial performance of tetracycline-active drugs based on ionic liquids. J. Mol. Liq. 386, 122450. doi:10.1016/j.molliq.2023.122450
Heck, R., Luki, M., Savi, D., Daniels, R., and Lunter, D. (2017). Ex vivo skin permeation and penetration of nonivamide from and in vivo skin tolerability of film-forming formulations containing porous silica. Eur. J. Pharm. Sci. 106, 34–40. doi:10.1016/j.ejps.2017.05.045
Hu, Y., and Peng, X. (2014). “Effect of the structures of ionic liquids on their physical Chemical,” in Structure and interactions of ionic liquids.
Kong, X., Chen, G., Zheng, L., Gao, Y., and Wang, J. (2013). Preparation of nonivamide and its insecticidal activities. Plant Dis. Pests 4, 29–31.
Lei, C., Chang, Z. D., and Liu, H. Z. (2010). Measurement and correlation of solubility of capsaicin in different solvents. Chin. J. Process Eng. 10, 738–742.
Lu, B., Yi, M., Hu, S., Wu, D., Zhu, Z., Wu, C., et al. (2021). Taurine-based ionic liquids for transdermal protein delivery and enhanced anticancer activity. ACS Sustain. Chem. Eng. 9, 5991–6000. doi:10.1021/acssuschemeng.1c01064
Luo, Y., and Wang, Y. (2011). Wounding effects of capsaicin,a new irritant riot control agent:an overview. J. Int. Pharm. Res. 38, 432–437. doi:10.13220/j.cnki.jipr.2011.06.005
Ma, C., Laaksonen, A., Liu, C., Lu, X., and Ji, X. (2018). The peculiar effect of water on ionic liquids and deep eutectic solvents. Chem. Soc. Rev. 47, 8685–8720. doi:10.1039/c8cs00325d
Mir, B., Bakhtawar, , Naz, S., Uroos, M., Ali, B., and Sharif, F. (2024). Pharmaceutical applications of lidocaine-based ionic liquids – a remarkable innovation in drug delivery. J. Mol. Liq. 397, 124052. doi:10.1016/j.molliq.2024.124052
Mörén, L., Östin, A., Larsson, A., Forsberg, J., Wiktelius, D., and Lindén, P. (2024). Rapid screening of riot control agents using DART-TD-HRMS. Forensic Toxicol. 42, 152–162. doi:10.1007/s11419-024-00681-5
Patocka, J., and Kuca, K. (2011). Pelargonic acid vanilyllamide (PAVA): riot control agent. Mil. Med. Sci. Lett. 80, 72–79. doi:10.31482/mmsl.2011.011
Podgoršek, A., Jacquemin, J., Pádua, A. A. H., and Costa Gomes, M. F. (2016). Mixing enthalpy for binary mixtures containing ionic liquids. Chem. Rev. 116, 6075–6106. doi:10.1021/acs.chemrev.5b00379
Qu, Y., Liu, Y., Zhang, H., Dai, Y., Zhu, Z., Ma, Y., et al. (2022). Application of Imidazolium-based polyionic liquids to separate the 1,3,5-Trioxane-Water/Ethanol-Water system based on experimental verification and molecular mechanism analysis. J. Mol. Liq. 348, 118079. doi:10.1016/j.molliq.2021.118079
Reilly, C. A., Crouch, D. J., Yost, G. S., and Fatah, A. A. (2001). Determination of capsaicin, dihydrocapsaicin, and nonivamide in self-defense weapons by liquid chromatography–mass spectrometry and liquid chromatography–tandem mass spectrometry. J. Chromatogr. A 912, 259–267. doi:10.1016/s0021-9673(01)00574-x
Rosa, A., Appendino, G., Melis, M. P., Deiana, M., Atzeri, A., Alessandra, I., et al. (2009). Protective effect and relation structure-activity of nonivamide and iododerivatives in several models of lipid oxidation. Chem. Biol. Interact. 180, 183–192. doi:10.1016/j.cbi.2009.01.002
Rottke, M., Lunter, D. J., and Daniels, R. (2014). In vitro studies on release and skin permeation of nonivamide from novel oil-in-oil-emulsions. Eur. J. Pharm. Biopharm. 86, 260–266. doi:10.1016/j.ejpb.2013.09.018
Satpute, R. M., Kushwaha, P. K., Nagar, D. P., and Rao, P. V. L. (2018). Comparative safety evaluation of riot control agents of synthetic and natural origin. Inhal. Toxicol. 30, 89–97. doi:10.1080/08958378.2018.1451575
Shamshina, J. L., and Rogers, R. D. (2023). Ionic liquids: new forms of active pharmaceutical ingredients with unique, tunable properties. Chem. Rev. 123, 11894–11953. doi:10.1021/acs.chemrev.3c00384
Sosnowska, A., Barycki, M., Zaborowska, M., Rybinska, A., and Puzyn, T. (2014). Towards designing environmentally safe ionic liquids: the influence of the cation structure. Green Chem. 16, 4749–4757. doi:10.1039/c4gc00526k
Szolcsányi, J., and JancsóGábor, A. (1975). Sensory effects of capsaicin congeners I. Relationship between chemical structure and pain-producing potency of pungent agents. Arzneimittelforschung 25, 1877–1881.
Wang, H., Liang, D., Guo, X., Zhao, S., and Cao, X. (2017). Study on the preparation and characterization of PAVA irritant explosive. Initiat. Pyrotech., 37–40. doi:10.3969/j.issn.1003-1480.2017.04.010
Yan, H., Wang, Z., and Wang, J. (2012). Correlation of solubility and prediction of the mixing properties of capsaicin in different pure solvents. Ind. Eng. Chem. Res. 51, 2808–2813. doi:10.1021/ie202917x
Zhai, H., and Cui, X. (2021). Thermal behavior test and characterization of a capsicum oleoresin (OC) composite material used for burning tear bomb. J. Phys. Conf. Ser. 1965, 012058. doi:10.1088/1742-6596/1965/1/012058
Zhang, S., Qi, X., Ma, X., Lu, L., and Deng, Y. (2010). Hydroxyl ionic liquids: the differentiating effect of hydroxyl on polarity due to ionic hydrogen bonds between hydroxyl and anions. J. Phys. Chem. B 114, 3912–3920. doi:10.1021/jp911430t
Keywords: nonivamide, riot control agent, ionic liquid, solubility, irritant effects
Citation: Ma W, Wang H, Wang Z, Chen D, Yuan L, Qin L and Mei Z (2024) Synthesis, characterization and irritant effects of nonivamide irritant riot control agent based on ionic liquids. Front. Chem. 12:1508396. doi: 10.3389/fchem.2024.1508396
Received: 09 October 2024; Accepted: 14 November 2024;
Published: 25 November 2024.
Edited by:
Slobodan Gadžurić, University of Novi Sad, SerbiaReviewed by:
Hossein Haghani, Shiraz University of Technology, IranCopyright © 2024 Ma, Wang, Wang, Chen, Yuan, Qin and Mei. This is an open-access article distributed under the terms of the Creative Commons Attribution License (CC BY). The use, distribution or reproduction in other forums is permitted, provided the original author(s) and the copyright owner(s) are credited and that the original publication in this journal is cited, in accordance with accepted academic practice. No use, distribution or reproduction is permitted which does not comply with these terms.
*Correspondence: Hongying Wang, d2xhYjIwMjRAMTI2LmNvbQ==
Disclaimer: All claims expressed in this article are solely those of the authors and do not necessarily represent those of their affiliated organizations, or those of the publisher, the editors and the reviewers. Any product that may be evaluated in this article or claim that may be made by its manufacturer is not guaranteed or endorsed by the publisher.
Research integrity at Frontiers
Learn more about the work of our research integrity team to safeguard the quality of each article we publish.