- Engineering Research Center of Protection and Utilization of Plant Resources, College of Bioscience and Biotechnology, Shenyang Agricultural University, Shenyang, Liaoning, China
The Chinese medicinal plant Thesium chinense Turcz. is the only plant used in the manufacture of Bairui Granules. However, to date, there has been very little research into the cytotoxic activity of active substances derived from Bairui Granules. Using chemical separation and spectroscopic methods, phenolic compounds 1–5 were identified as methyl-p-hydroxycinnamate, vanillin, kaempferol, isorhamnetin-3-O-glucoside, and astragalin, respectively. UPLC-MS/MS analyses revealed that compounds 1–5 were present at concentrations of 0.006 ± 0.002, 1.63 ± 0.87, 3.65 ± 0.83, 26.97 ± 11.41, and 27.67 ± 2.91 μg/g, respectively in Bairui Granules. Compounds 1, 2, and 4 were detected here for the first time in Bairui Granules. Using co-culture experiments, isorhamnetin-3-O-glucoside (4) was found to be beneficial to the proliferation Chinese hamster ovary (CHO) cells (6.46% ± 0.86% to 38.45% ± 9.04%), natural killer cells from human umbilical cord blood (UCB NK cells) (25.68% ± 0.02% to 70.81% ± 0.26%), and mesenchymal stem cells from human umbilical cord blood (UCB MSC cells) (1.66% ± 0.05% to 27.64% ± 0.51%) when the concentration was similar to that found in Bairui granules. Moreover, vanillin (2) was conducive to UCB NK cells proliferation (28.21% ± 0.44%) at a concentration of 64 μg/mL, while maintaining cell viability. UCB NK cell proliferation was promoted at rates of 41.03% ± 0.48% to 67.22% ± 0.68% when astragalin (5) was present at low concentrations (8 and 16 μg/mL). Methyl-p-hydroxycinnamate (1) and vanillin (2) at different concentrations both had an inhibitory effect on the proliferation of natural killer cells from human peripheral blood (PB NK cells), but the inhibitory concentration ranges of these compounds were not equivalent to the concentration ranges of the compounds in Bairui Granules. These results provide a foundation for the safe use of T. chinense preparations.
1 Introduction
Thesium chinense Turcz. Is a perennial herbaceous plant belonging to the Satalaceae family, and is widely distributed in eastern Asia (Shao et al., 2019). In China, Thesium chinense is used as a traditional Chinese medicine with many years of history, and described recorded in several Chinese ethnomedical monographs (Li G. H. et al., 2021). As early as the Song Dynasty, the Ben Cao Tu Jing described the usage and required dosage of T. chinense for clearing heat and detoxifying, tonifying the kidneys, and as an astringent essence (Liu et al., 2023). In 1950, other uses of T. chinense, including the treatment of head sores and cervical lymphadenitis, were recorded in the Guo Yao Ti Yao (Li G. H. et al., 2021). The decoction of the whole plant is used to treat mastopathy, headache resulting from pneumonia, stomach pain, and tuberculosis of cervical lymph nodes in Anhui and Fujian Provinces (Li G. H. et al., 2021; Parveen et al., 2007). Furthermore, T. chinense has also been made into modern pharmaceutical preparations, including Bairui Granules and Bairui Tablets, which are mainly used to treat tracheitis, rhinitis and colds. The Bairui Granules sales more than 400 million yuan in the last year. T. chinense has therefore played an important role as a Chinese herbal medicine at least since the Song Dynasty.
Because T. chinense has such widespread traditional uses, the active chemical substances in the plant have received widespread attention. T. chinense has been found to contain abundant secondary metabolites, including flavonoids, organic acids, alkaloids, and terpenoids (Liu et al., 2018; Lombard et al., 2020). The various biological activities of these chemical substances have been studied in in vitro and in vivo experiments (Li G. H. et al., 2021). The flavonoids are now considered to be the main functional substances in T. chinense (Cao et al., 2021), and total flavonoids and kaempferol are now taken to be the quality control standards for traditional medicinal preparations of T. chinense in China. These flavonoids not only exhibit anti-inflammatory and antioxidant activities, and inhibit the proliferation of cancer cells, but have also been found to play a certain role in protecting the liver and nerves (Khan et al., 2020; Soromou et al., 2012; Zhen et al., 2017). The phenolic compounds isolated from T. chinense also exhibited certain anti-inflammatory effects (Liu et al., 2023). The positive therapeutic effects of T. chinense are therefore likely to be closely related to the chemical substances it contains. However, there is little research on the active substances present in Bairui Granules, which are prepared with T. chinense as their sole herbal ingredient.
The human body contains many types of immune cells and stem cells, including natural killer cells (NK cells) and mesenchymal stem cells (MSC cells) (Musina et al., 2005; Strowig et al., 2010). NK cells have the ability to clear leukemia and tumor cells, and MSC cells have strong hematopoietic support function and are able to differentiate into various different types of human cells as required (Steward and Kelly, 2014; Vyas et al., 2023). Chinese hamster ovary cells (CHO cells) are the most widely applied mammalian recombinant protein cell line in immunology (Róisín et al., 2020). However, it is not yet known whether the chemical substances present in T. chinense have an impact on human immune cells or stem cells. In this study, we focused on flavonoids and phenolic compounds present in Bairui Granules and investigated whether these have an impact on mammalian expression vectors, immune cells, and stem cells, further providing reference for the correct and safe dosage in preparations of T. chinense.
2 Materials and methods
2.1 Plant materials and test cell lines
Whole dried T. chinense plants were purchased from the Hebei Baoding Anguo Medicinal Material Wholesale Market, and were identified by Professor Bo Qu. Chinese hamster ovary cells (CHO cells), natural killer cells from human umbilical cord blood (UCB NK cells), natural killer cells from human peripheral blood (PB NK cells), and mesenchymal stem cells from human umbilical cord blood (UCB MSC cells) were provided by the Cell Preparation Center of Qinhuangdao Weiming Health City Development Co., Ltd. CHO cells were cultured in RPMI-1640 medium (Hyclone) containing 10% fetal bovine serum (Gibco). NK cells and MSC cells were cultured with the corresponding cell culture kits. The NK and MSC cell culture kits were purchased from the Beijing Tongli Haoyuan Biotechnology Co., Ltd. FITC-conjugated anti-human CD3 antibody and APC-conjugated anti-human CD56 antibody were purchased from Meitianni Biotechnology Co., Ltd.
2.2 Isolation and identification of phenolic compounds from Thesium chinense
The entire dried T. chinense plants (15.0 Kg) were subjected to a methanol extraction. The resulting extract was then concentrated under a vacuum and was treated with an equal volume of ethyl acetate (1:1, v/v). The crude ethyl acetate fraction (204.0 g) was purified on a silica gel column using eluents (dichloromethane/methanol, 1:0−0:1), and resulting in five fractions (Fr.-1−Fr.-5). Fr.-2 (29.0 g) was passed through an MCI gel column chromatography column (methanol/water, 70:30−100:0) to get four parts (Fr.-2.1−Fr.-2.4). Fr-2.1 (19.4 g) was separated on a silica gel column with petroleum ether/acetone elution (15:1, v/v) to obtain six fractions (Fr.-2.1.1−Fr.-2.1.6). Fr.-2.1.5 (4.5 g) and Fr.-2.1.6 (6.5 g) were passed separately through a Sephadex LH-20 column to get four parts (Fr.-2.1.5.1−Fr.-2.1.5.4) and five parts (Fr.-2.1.6.1−Fr.-2.1.6.5), respectively. Fr.-2.1.5.2 (320.0 mg) was purified using semi-preparative HPLC with DAD detector (methanol/water, 45:55) to yield compound 1 (50.7 mg). Fr.-2.1.5.3 (452.2 mg) was purified using semi-preparative HPLC with DAD detector (methanol/water, 25:75) to yield compound 2 (90.4 mg). Fr.-2.1.5.4 (783.0 mg) was purified using semi-preparative HPLC with DAD detector (methanol/0.2% acid water, 58:42) to yield compound 3 (110.4 mg), and Fr.-2.1.6.3 (2.4 g) was purified using semi-preparative HPLC with DAD detector (methanol/0.2% acid water, 41:59) to yield compounds 4 (156.0 mg) and 5 (175.3 mg).
2.3 Qualitative and quantitative analysis of compounds one to five in Bairui Granules
Samples of Bairui Granules (Anhui Jiuhua Huayuan Pharmaceutical Co., Ltd.) were prepared for detection of the compounds. About 0.2 g sample was subjected three times in succession to a methanol extraction in an ultrasonic bath, each time for 45 min. The obtained methanol extract was then concentrated under vacuum until dry. Then, the dissolved liquid (500 μL chromatographic methanol) was filtered through 0.22 μm filter, and the prepared samples were finally analyzed on the UPLC-MS/MS (Shimadzu LCMS-8050). Briefly, 10 μL samples were injected into Shim-pack GIST C18 column (2 μm, 100 × 2.1 mm, 0.2 mL/min), and the column temperature was maintained at 40 °C. A (0.1% acid water) and B (acetonitrile) were selected as mobile phases, and the elution procedure was as follows: 0–12 min, from 5% B to 95% B; 12–13 min, 95% B; 13–14 min, from 95% B to 5% B; 14–17 min, 5% B. The ESI procedure was as follows: dry gas flow rate 10 L/min, heating block temperature 450 °C; interface temperature 300 °C; heating gas flow rate 10 L/min; atomizing gas flow rate 3 L/min; (Yang et al., 2022). Compounds one to five were monitored using multiple reaction monitoring (MRM) mode, and their related parameters are given in Supplementary Table S1. The calibration curves for compounds one to five are shown in Supplementary Table S2.
2.4 In vitro cytotoxicity assay
The compounds to be assayed were dissolved in DMSO (0.5% of the total volume). Four cell suspensions (CHO cells, UCB NK cells, PB NK cells, and UCB MSC cells) were prepared in advance. Each of the compounds was diluted to the final concentrations: 8, 16, 32, 64, 128, and 256 μg/mL, and were added to a 24-well plate, in which compound 4 prepared for CHO cells, compounds 2, 4, and 5 prepared for UCB NK cells, compounds 1, 2, and 4 prepared for PB NK and UCB MSC cells. Each well included cell suspension, cell culture medium and compound solution, at the ratio of 100:99:1. 500 μL of cell culture medium/DMSO (99:1, v/v) was added to 500 μL of cell suspension as a control (Li et al., 2022). The number of cells in each well was approximately 1.0E+06, and each treatment included three biological replicates. The co-cultured cell suspensions were then cultured in a carbon dioxide incubator (5% CO2, 37°C, saturated humidity) for 48 h. After the cultivation step was complete, 10 μL of co-cultured cell suspension was added to 0.4% trypan blue (10 μL) and a uniform mixture was prepared. Subsequently, 10 μL of this mixture was then injected into each cavity of the slide and maintained for 30 s. Finally, the cell morphology was observed using an inverted microscope (Nikon) and cells were counted using a cell counter (Thermo).
2.5 Detection of PB NK cell surface markers
PB NK cells were cultured for 48 h according to the method described above. The co-cultured cell suspension (about 1.0E+06) was moved to a EP tube (1.5 mL). The samples were centrifuged at 1,500 rpm for 3 min, an then the supernatant was removed and discarded. The remaining cells were re-suspended using 1,000 μL of phase buffer saline (PBS) and then centrifuged at 1,500 rpm for 3 min. The supernatant was then removed and discarded. This process was repeated twice. Finally, the obtained cells were re-suspended in 1,000 μL of PBS to obtain a new cell suspension. 100 μL of the new cell suspension was injected into each of three EP tubes, with one tube containing 5 μL of FITC-conjugated anti-human CD3 antibody, one containing 5 μL of APC-conjugated anti-human CD56 antibody, and with one tube serving as a blank control (5 μL PBS). Evenly mixed samples were incubated at 4 °C in the dark for 10 min. After incubation, each tube of cells was re-suspended using the above method, resulting in a new cell suspension with a final volume of 500 μL. Finally, the samples were analyzed using flow cytometry (MiltenyiBiotecB. V. and Co. KG).
2.6 Statistical analyses
Data were analyzed using PASW Statistics 19 and GraphPad. Prism 9. If the two groups of data conformed to a normal distribution, independent samples t-tests were used to investigate comparisons between two sets of data, and comparisons among three or more groups were made using one-way ANOVAs with Tukey’s tests. Differences were considered to be statistically significant if p < 0.05. The values given represent the mean ± standard deviation (SD).
3 Results
3.1 Structural identification of phenolic compounds one to five from Thesium chinense
Chemical separation and spectroscopic methods allowed the identification of compounds 1–5 as methyl-p-hydroxycinnamate (Kwon and Kim, 2003), vanillin (Pironti et al., 2021), kaempferol (Singh et al., 2008), isorhamnetin-3-O-glucoside (Fico et al., 2007), and astragalin (Wei et al., 2011), respectively (Supplementary Tables S3–S4; Figure 1A). Compounds 1 and 2 are phenolics, and compounds three to five are flavonoids. In this study compounds 2 and 4 have been isolated for the first time from T. chinense.
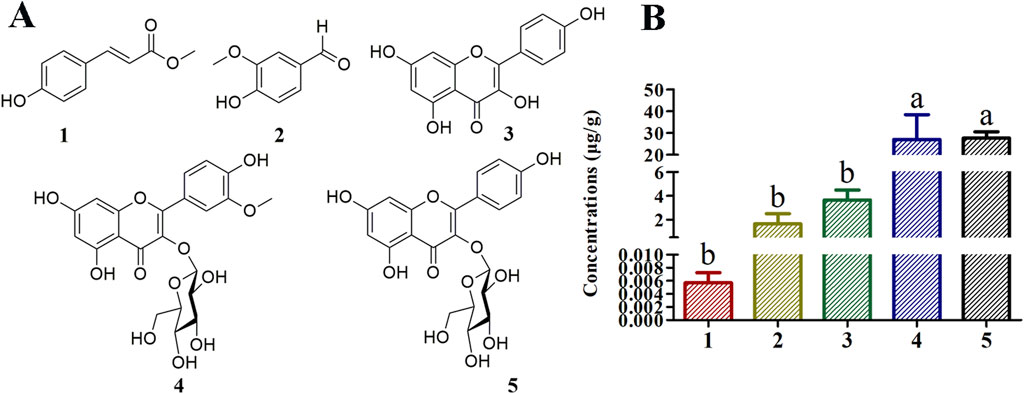
Figure 1. Structures of compounds isolated from Thesium chinense and quantitative analysis of their concentrations in Bairui Granules. (A) Chemical structures of flavonoid and phenolic compounds 1–5; (B) Concentrations of compounds one to five in Bairui Granules. Mean differences were compared using one-way ANOVA with Tukey’s test. The different small letters (A, B) represent significant differences at the p < 0.05 level. The results shown represent the means ± standard deviation.
3.2 Qualitative and quantitative analyses of compounds one to five in Bairui Granules
To determine concentrations of compounds one to five present in Bairui Granules, an extract of Bairui Granules was assessed using an UPLC-MS/MS. Prepared samples were all analyzed using an external standard. The retention times of compounds one to five were found to be 7.864, 6.215, 8.112, 6.772, and 6.068 min (Figure 2), respectively, and compounds one to five were present in Bairui Granules at concentrations of 0.006 ± 0.002, 1.63 ± 0.87, 3.65 ± 0.83, 26.97 ± 11.41, and 27.67 ± 2.91 μg/g, respectively (Figure 1B, 2A–E). These results suggested that the concentrations of the flavonoid compounds three to five in Bairui Granules were higher than those of the phenolic compounds 1 and 2. This study represents that compounds 1, 2, and 4 have been detected for the first time in Bairui Granules.
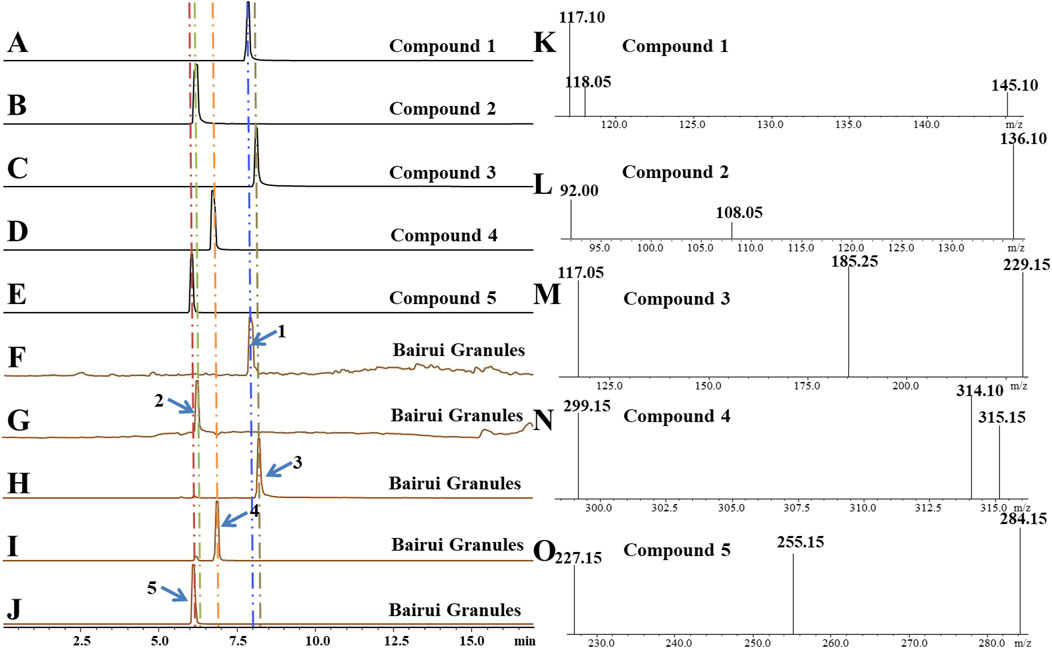
Figure 2. Qualitative analysis of in Bairui Granules using UPLC-MS/MS with an MRM-model. (A-E) Chromatogram of compounds 1–5; (F-J) Chromatograms of the Bairui Granules extracts; (K-O) Mass spectra of compounds one to five.
3.3 Effect of compound 4 on the proliferation of CHO cells
In order to determine the effect of compound 4 on the proliferation of CHO cells, different concentrations of compound 4 were co-cultured with CHO cells for 48 h. Examination with an inverted microscope revealed that after the CHO cells were treated with compound 4 at different concentrations, the cells remained round and bright, which was consistent with the morphology of the control group (Supplementary Figure S1). Treatment with compound 4 at concentrations of 8, 16, 32, 64, 128, and 256 μg/mL led to increases in CHO cell proliferation, with the promotion rates being 6.46 ± 0.86, 3.44 ± 0.43, 12.91 ± 0.43, 22.09 ± 4.16, 34.43 ± 7.89, and 38.45% ± 9.04%, respectively (Figure 3A). Treatment with compound 4 at concentrations of 8, 16, and 64 μg/mL had no obvious effect on CHO cells viability compared with the control. However, treatment with compound 4 at concentrations of 128 and 256 μg/mL significantly promoted the viability of CHO cells (p < 0.01) (Figure 3B). The above results indicated that at the concentrations present in Bairui Granules, compound 4 was beneficial to the proliferation of CHO cells.
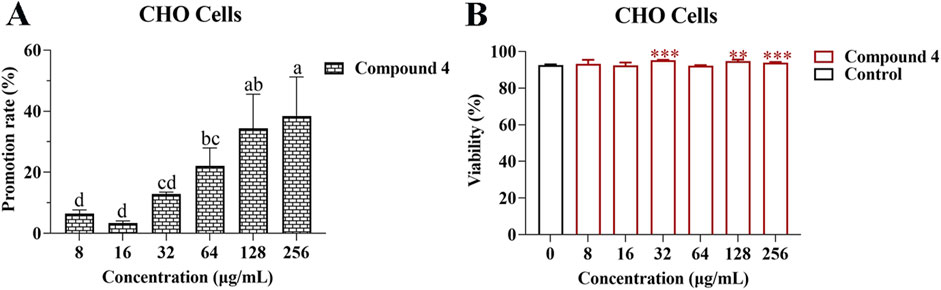
Figure 3. Effects of compound 4 on the proliferation and viability of CHO cells. (A) Promotion rate of compound 4 at different concentrations on the proliferation of CHO cells; (B) Effect of compound 4 at different concentrations on the viability of CHO cells. Mean differences were compared using one-way ANOVA with Tukey’s test. The different small letters (A, B) represent significant differences at the p < 0.05 level. Double or triple asterisks (** or ***) indicate significant differences between the control group and other treatments. ** Indicates p < 0.01. ***p < 0.001.
3.4 Effects of compounds 2, 4, and 5 on the proliferation of UCB NK cells
In order to investigate the effects of compounds 2, 4, and 5 on the proliferation of UCB NK cells, compounds 2, 4, and 5 at different concentrations were separately co-cultured with UCB NK cells for 48 h. UCB NK cell morphology was not affected under any concentrations of compounds 2, 4, or 5 (Supplementary Figures S2–S4).
When the concentrations of compound 2 were 16, 64, and 128 μg/mL, the rate of promotion of UCB NK cell proliferation remained within a certain range (25.09% ± 0.56% to 28.21% ± 0.44%). However, at a concentration of 64 μg/mL, compound 2 could significantly promote the viability of UCB NK cells compared with the control, while at a concentration of 128 μg/mL, compound 2 exhibited a significant inhibitory effect.
At concentrations of 32, 64, 128, and 256 μg/mL, compound 4 was able to promote the proliferation of UCB NK cells, with promotion rates ranging from 25.68% ± 0.02% to 70.81% ± 0.26%. However, at the same concentrations, compound 4 exhibited an inhibitory effect on the viability of UCB NK cells.
When compound 5 was present at low concentrations (8 and 16 μg/mL), the promotion rate of UCB NK cell proliferation ranged from 41.03% ± 0.48% to 67.22% ± 0.68%, but the compound exhibited an inhibitory effect on viability of the cells at these concentrations. At a concentration of 256 μg/mL, compound 5 promoted cell proliferation, but had no effect on cell viability (Figures 4A, B). These results showed that the phenolic compound 2 was conducive to the proliferation of UCB NK cells while maintaining normal cell morphology and viability at a concentration of 64 μg/mL.
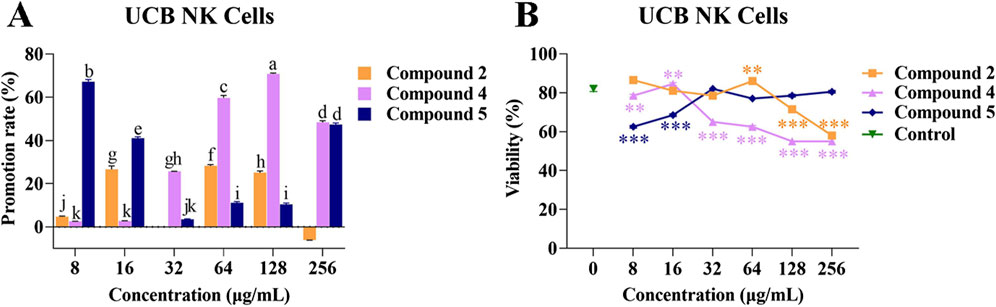
Figure 4. Effects of compounds 2, 4, and 5 on the proliferation and viability of UCB NK cells. (A) Promotion rate of compounds 2, 4, and 5 at different concentrations on the proliferation of UCB NK cells; (B) The effects of compounds 2, 4, and 5 at different concentrations on the viability of UCB NK cells. Mean differences were compared using one-way ANOVA with Tukey’s test. The different small letters (a, b, c, d, e, f, g, h, i, j, and k) represent significant differences at the p < 0.05 level. Double or triple asterisks (** or ***) indicate significant differences between the control group and other treatments. ** Indicates p < 0.01. ***p < 0.001.
3.5 The effects of compounds 1, 2, and 4 on the proliferation of PB NK cells
Compounds 1, 2, and 4 at different concentrations were co-cultured with PB NK cells to determine their effects on cell proliferation. Following co-culture for 48 h with compounds 1, 2, and 4 at different concentrations, no effect on PB NK cell morphology was observed (Supplementary Figures S5–S7). However, when the concentration of compound 1 was increased, the inhibition rate of PB NK cells proliferation also gradually increased, and the cell viability gradually decreased.
The inhibitory rate of compound 2 on the proliferation of PB NK cells (2.70% ± 0.22% to 26.80% ± 0.68%) was comparable to that of compound 4 (6.08% ± 0.04% to 23.96% ± 0.80%). Compounds 2 and 4 at other concentrations had no effect on viability of PB NK cells compared with the control, although at a concentration of 256 μg/mL, compound 4 had an inhibitory effect on cell viability (Figures 5A, B). The above results suggested that compound 1 has a higher inhibitory effect on the proliferation of PB NK cells than compounds 2 and 4.
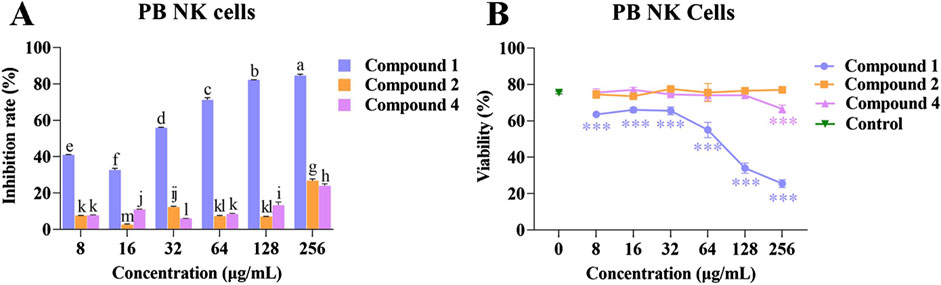
Figure 5. Effects of compounds 1, 2, and 4 on the proliferation and viability of PB NK cells. (A) Inhibition rate of compounds 1, 2, and 4 at different concentrations on the proliferation of PB NK cells; (B) The effects of compounds 1, 2, and 4 at different concentrations on the viability of PB NK cells. Mean differences were compared using one-way ANOVA with Tukey’s test. The different small letters (a, b, c, d, e, f, g, h, i, j, k, l, and m) represent significant differences at the p < 0.05 level. Triple asterisks (***) indicate significant differences between the control group and other treatments. ***p < 0.001.
3.6 The effects of compounds 1, 2, and 4 on the proliferation of UCB MSC cells
Compounds 1, 2, and 4 at different concentrations were co-cultured with UCB MSC cells to investigate their effects on cell proliferation. Following co-culture for 48 h with compounds 1, 2, and 4 at different concentrations, the UCB MSC cells were spindle shaped, which is consistent with normal MSC cell morphology (Supplementary Figures S8–S10). The promotion rates of compounds 1 and 4 at different concentrations on UCB MSC cell proliferation range from 1.64% ± 0.19% to 11.28% ± 0.16% and from 1.66% ± 0.05% to 27.64% ± 0.51%, respectively.
Compound 2 inhibited UCB MSC cell proliferation at concentrations of 16, 32, 128, and 256 μg/mL, but had a promoting effect at a concentration of 8 μg/mL (Figure 6A). Compared with the control, compound 1 had a significant inhibitory effect on UCB MSC cell viability at concentrations of 16, 32, 128, and 256 μg/mL (p < 0.05), while compounds 2 and 4 had no significant effect on cell viability (Figure 6B). The above results indicate that compound 4 is able to promote UCB MSC cells proliferation without affecting cell viability.
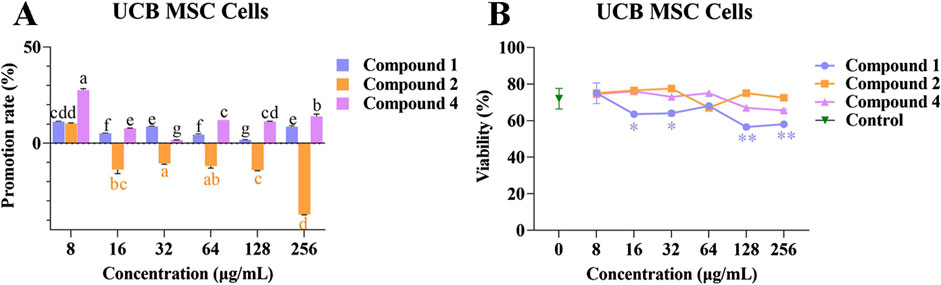
Figure 6. The effects of compounds 1, 2, and 4 on the proliferation and viability of UCB MSC cells. (A) Effect of compounds 1, 2, and 4 at different concentrations on the proliferation of UCB MSC cells; (B) The effects of compounds 1, 2, and 4 at different concentrations on the viability of UCB MSC cells. Mean differences were compared using one-way ANOVA with Tukey’s test. The different small letters (a, b, c, d, e, f, and g) represent significant differences at the p < 0.05 level. Single or double asterisks (* or **) indicate significant differences between the control group and other treatments. *p < 0.05. ** Indicates p < 0.01.
3.7 The effects of compounds 1, 2, and 4 on the expression of PB NK cell markers
We next wanted to investigate the effects of low concentrations of compounds 1, 2, and 4 on the expression of NK cell markers. Low concentrations of compounds 1, 2, and 4 were co-cultured separately with PB NK cells. In human pathology, the proportion of CD3−CD56+ is commonly used as a proxy for NK cells in the diagnosis of certain diseases. The proportion of NK cells with a CD3−CD56+ cell phenotype when cultured in the presence of compound 1 at a concentration of 32 μg/mL was 54.46% (Figure 7A). Similarly, after 48 h of co-culture of PB NK cells with compound 2 at concentrations of 8 and 16 μg/mL, the proportion of cells with the CD3−CD56+ phenotype were 69.80% and 69.40%, respectively, while the proportions of cells co-cultured with this phenotype following co-culture with compound 4 under the same conditions were 70.94% and 70.32%, respectively (Figures 7B–E). These results suggest that compound 1 had an inhibitory effect on PB NK cell differentiation compared to the control (72.10%) at a concentration of 32 μg/mL.
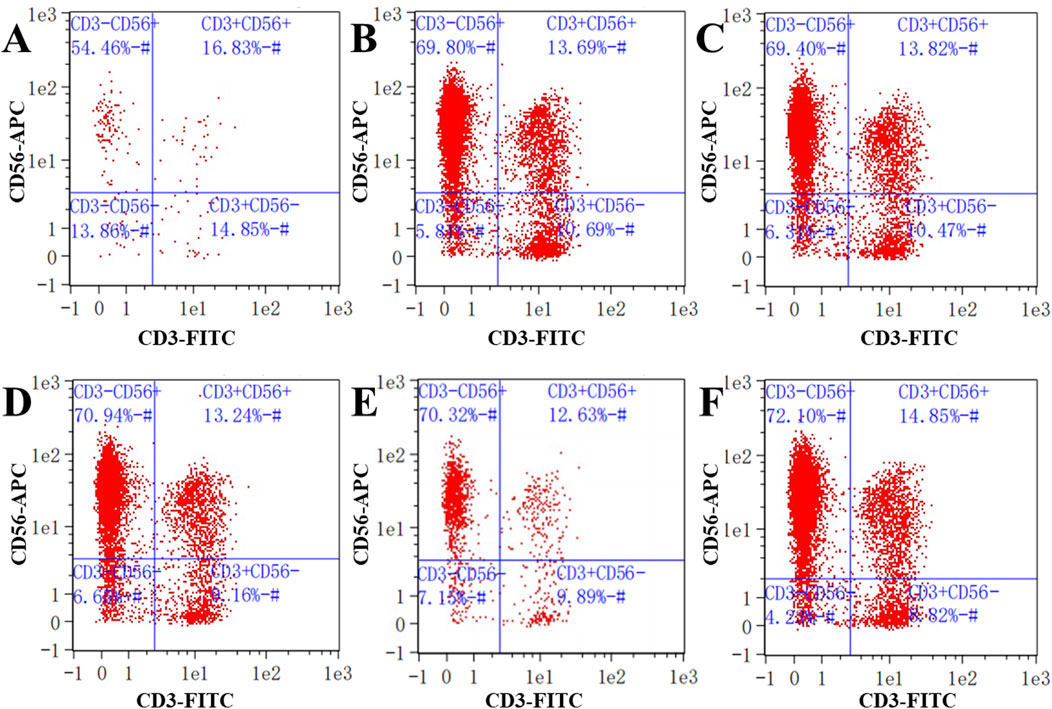
Figure 7. Detection of NK cell surface markers. (A) Expression of NK cell biomarkers in cells co-cultured with compound 1 at a concentration of 32 μg/mL; (B, C) Expression of NK cell biomarkers in cells co-cultured with compound 2 at concentrations of 8 and 16 μg/mL; (D, E) Expression of NK cell biomarkers in cells co-cultured with compound 4 at concentrations of 8 and 16 μg/mL; (F) Expression of NK cell biomarkers in the control. CD3−represents CD3 negative; CD56+ represents CD56 positive.
4 Discussion
4.1 Bairui granules contain a high diversity of phenolic compounds
T. chinense is an important traditional Chinese medicine. When applied as a crude drug, T. chinense is able to significantly reduce ear swelling caused by xylene in mice (Yuan et al., 2006). Moreover, a concentration of the methanol extract of T. chinense is able to significantly reduce the edema in mouse paws induced by carrageenan, and reduces xylene-induced ear edema in mice (Parveen et al., 2007). The chemical substances present in this plant have been widely studied and identified. At present, most of the compounds isolated from T. chinense have been flavonoids and phenylpropanoids (Li G. H. et al., 2021; Liu et al., 2023). In this study, we isolated and identified two phenolic compounds and three flavonoids from T. chinense, with vanillin (2) and isorhamnetin-3-O-glucoside (4) isolated from this plant for the first time here. The concentrations of these compounds in Bairui Granules were further determined using UPLC-MS/MS.
4.2 Phenolic compounds exhibit diverse biological activities
Flavonoids in T. chinense have abundant biological activity. Previous research on the activities of kaempferol (3) and astragalin (5) mainly focused on their anti-inflammatory and analgesic properties (Ramanan et al., 2016; Sudarshan and Aidhen, 2016). Kaempferol (3) inhibited the writhing reaction following abdominal contraction tests induced by a certain concentration of acetic acid in mice (Parveen et al., 2007). Certain concentrations of astragalin (5) were able to inhibit the infiltration of inflammatory cells, reduce breast congestion and edema and inhibit the release of inflammatory cytokines in a mastitis mouse model (Li et al., 2013). Moreover, astragalin (5) was also able to block the NF-κB signaling pathway to combat colitis (Han et al., 2020). Diverse biological activities have also been observed in methyl-p-hydroxycinnamate (1), vanillin (2), and isorhamnetin-3-O-glucoside (4). Methyl-p-hydroxycinnamate (1) was found to inhibit inflammation of RAW 264.7 macrophages under LSP induced stimulation (Vo et al., 2014). Vanillin (2) and isorhamnetin-3-O-glucoside (4) both had anticancer, anti-inflammatory, and antibacterial effects. Furthermore, vanillin (2) also has a protective effect on nerves and blood (Arya et al., 2021; Du et al., 2014). From this, it can be seen that research into T. chinense mainly focuses on how the component chemical substances exert their therapeutic effects. However, Bairui Granules are a medical preparation, whose only herbal ingredient is T. chinense, and to date there has been little research into the effects of the active substances on mammalian expression vectors, human immune cells, and stem cells.
4.3 Phenolics are the most biologically active substances in Bairui Granules
Flavonoids and phenolic compounds have certain effects on mammalian expression vectors, human immune cells, and stem cells (Burkard et al., 2017; Ozdal et al., 2018). Flavonoids from Astragalus complanatus R.Br. ex Bunge. seeds significantly promoted the proliferation of NK cells (Han et al., 2015), and the flavonoid quercetin has a close relationship with the differentiation of MSC cells (Yu et al., 2010). In addition, phenolic compounds are able to increase NK cell activity significantly and can also serve as antioxidants to protect MSC cells (Kilani Jaziri et al., 2016; Li H. S. et al., 2021). In this study, the methyl-p-hydroxycinnamate (1), vanillin (2), and isorhamnetin-3-O-glucoside (4) present in Bairui Granules were qualitatively and quantitatively studied for the first time. Importantly, isorhamnetin-3-O-glucoside (4) was able to promote the proliferation of CHO cells, UCB NK cells, and UCB MSC cells when its concentration was close to that found in the Bairui Granules. Meanwhile, methyl-p-hydroxycinnamate (1) was conducive to the proliferation of UCB MSC cells, and vanillin (2) and astragalin (5) at different concentrations all promoted the proliferation of UCB NK cells. These results indicated that methyl-p-hydroxycinnamate (1), vanillin (2), isorhamnetin-3-O-glucoside (4), and astragalin (5) were potentially the active substances in Bairui Granules. However, not all flavonoids and phenolic compounds promote the activity of immune cells (Perche et al., 2014). Our results also demonstrated that methyl-p-hydroxycinnamate (1), vanillin (2), and isorhamnetin-3-O-glucoside (4) exhibited inhibitory effects on PB NK cells. The cytotoxicity tests of the phenolic compounds present in Bairui Granules could thus provide a scientific basis for the medicinal value of T. chinense.
5 Conclusion
Five phenolics were identified in Bairui Granules that obtained from the medicinal plant T. chinense. Moreover, methyl-p-hydroxycinnamate (1), vanillin (2), and isorhamnetin-3-O-glucoside (4) were found for the first time in Bairui Granules. These metabolites had a certain effect on mammalian expression vectors, human immune cells, and stem cells. The biological activity of those secondary metabolites from T. chinense justify the medicinal use of this plant.
Data availability statement
The data presented in the study are deposited in the figshare repository, accession number 10.6084/m9.figshare.27894771.
Author contributions
SZ: Conceptualization, Formal Analysis, Investigation, Validation, Writing–original draft. HC: Conceptualization, Data curation, Formal Analysis, Investigation, Methodology, Software, Supervision, Validation, Visualization, Writing–original draft. JH: Data curation, Funding acquisition, Investigation, Methodology, Project administration, Resources, Supervision, Validation, Visualization, Writing–original draft, Writing–review and editing. SL: Conceptualization, Data curation, Formal Analysis, Funding acquisition, Investigation, Methodology, Project administration, Resources, Software, Supervision, Validation, Visualization, Writing–original draft, Writing–review and editing.
Funding
The author(s) declare that financial support was received for the research, authorship, and/or publication of this article. This research was funded by the National Natural Science Foundation of China (no. 32370416).
Acknowledgments
Our sincere thanks to Prof. Bo Qu for helping in the identification of the plant.
Conflict of interest
The authors declare that the research was conducted in the absence of any commercial or financial relationships that could be construed as a potential conflict of interest.
Generative AI statement
The author(s) declare that no Generative AI was used in the creation of this manuscript.
Publisher’s note
All claims expressed in this article are solely those of the authors and do not necessarily represent those of their affiliated organizations, or those of the publisher, the editors and the reviewers. Any product that may be evaluated in this article, or claim that may be made by its manufacturer, is not guaranteed or endorsed by the publisher.
Supplementary material
The Supplementary Material for this article can be found online at: https://www.frontiersin.org/articles/10.3389/fchem.2024.1506792/full#supplementary-material
References
Arya, S. S., Rookes, J. E., Cahill, D. M., and Lenka, S. K. (2021). Vanillin: a review on the therapeutic prospects of a popular flavouring molecule. Adv. Tradit. Med. 21, 1–17. doi:10.1007/s13596-020-00531-w
Burkard, M., Leischner, C., Lauer, U. M., Busch, C., Venturelli, S., and Frank, J. (2017). Dietary flavonoids and modulation of natural killer cells: implications in malignant and viral diseases. J. Nutr. Biochem. 46, 1–12. doi:10.1016/j.jnutbio.2017.01.006
Cao, J. F., Zhang, Y., Chen, P. Y., Wu, C., and Deng, Y. (2021). Analysis of transcriptome sequencing and related genes of flavonoid biosynthesis from Thesium chinense Turcz. Med. Plant Res. 11, 1–11. doi:10.5376/mpr.2021.11.0004
Du, L. Y., Zhao, M., Xu, J., Qian, D. W., Jiang, S., Shang, E. X., et al. (2014). Analysis of the metabolites of isorhamnetin 3-O-glucoside produced by human intestinal flora in vitro by applying ultraperformance liquid chromatography/quadrupole time-of-flight mass spectrometry. J. Agric. Food Chem. 62, 2489–2495. doi:10.1021/jf405261a
Fico, G., Rodondi, G., Flamini, G., Passarella, D., and Tome´, F. (2007). Comparative phytochemical and morphological analyses of three Italian Primula species. Phytochemistry 68, 1683–1691. doi:10.1016/j.phytochem.2007.04.019
Han, R., Wu, W. Q., Wu, X. P., and Liu, C. Y. (2015). Effect of total flavonoids from the seeds of Astragali complanati on natural killer cell function. J. Ethnopharmacol. 173, 157–165. doi:10.1016/j.jep.2015.07.017
Han, Y. M., Koh, J., Kim, J. H., Lee, J., Im, J. P., and Kim, J. S. (2020). Astragalin inhibits nuclear factor-κb signaling in human colonic epithelial cells and attenuates experimental colitis in mice. Gut Liver 15, 100–108. doi:10.5009/gnl19268
Khan, H., Tundis, R., Ullah, H., Aschner, M., Belwal, T., Mirzaei, H., et al. (2020). Flavonoids targeting NRF2 in neurodegenerative disorders. Food Chem. Toxicol. 146, 111817. doi:10.1016/j.fct.2020.111817
Kilani Jaziri, S., Mokdad Bzeouich, I., Krifa, M., Nasr, N., Ghedira, K., and Chekir-Ghedira, L. (2016). Immunomodulatory and cellular anti-oxidant activities of caffeic, ferulic, and p-coumaric phenolic acids: a structure-activity relationship study. Drug Chem. Toxicol. 40, 416–424. doi:10.1080/01480545.2016.1252919
Kwon, Y. S., and Kim, C. M. (2003). Antioxidant constituents from the stem of Sorghum bicolor. Arch. Pharm. Res. 26, 535–539. doi:10.1007/BF02976877
Li, F., Liang, D., Yang, Z., Wang, T., Wang, W., Song, X., et al. (2013). Astragalin suppresses inflammatory responses via down-regulation of NF-kappa B signaling pathway in lipopolysaccharide-induced mastitis in a murine model. Int. Immunopharm. 17, 478–482. doi:10.1016/j.intimp2013.07.010
Li, G. H., Fang, K. L., Yang, K., Cheng, X. P., Wang, X. N., Shen, T., et al. (2021a). Thesium chinense Turcz.: an ethnomedical, phytochemical and pharmacological review. J. Ethnopharmacol. 273, 113950. doi:10.1016/j.jep.2021.113950
Li, H. D., Kang, Z. L., Hua, J., Feng, Y. L., and Luo, S. H. (2022). Root exudate sesquiterpenoids from the invasive weed Ambrosia trifida regulate rhizospheric Proteobacteria. Sci. Total Environ. 834, 155263. doi:10.1016/j.scitotenv.2022.155263
Li, H. S., Zhu, H., Ge, T., Wang, Z. F., and Zhang, C. (2021b). Mesenchymal stem cell-based therapy for diabetes mellitus: enhancement strategies and future perspectives. Stem Cell Rev. Rep. 17, 1552–1569. doi:10.1007/s12015-021-10139-5
Liu, C., Li, X. T., Cheng, R. R., Han, Z. Z., Yang, L., Song, Z. C., et al. (2018). Anti-oral common pathogenic bacterial active acetylenic acids from Thesium chinense Turcz. J. Nat. Med-Tokyo 72, 433–438. doi:10.1007/s11418-018-1180-3
Liu, Z. Z., Ma, J. C., Deng, P., Ren, F. C., and Li, N. (2023). Chemical constituents of Thesium chinense Turcz. and their in vitro antioxidant, anti-Inflammatory and cytotoxic activities. Molecules 28, 2685. doi:10.3390/molecules28062685
Lombard, N., van Wyk, B. E., and le Roux, M. M. (2020). A review of the ethnobotany, contemporary uses, chemistry and pharmacology of the genus Thesium (Santalaceae). J. Ethnopharmacol. 256, 112745. doi:10.1016/j.jep.2020.112745
Musina, R. A., Bekchanova, E. S., and Sukhikh, G. T. (2005). Comparison of mesenchymal stem cells obtained from different human tissues. Bull. Exp. Biol. Med. 139, 504–509. doi:10.1007/s10517-005-0331-1
Ozdal, T., Sari-Kaplan, G., Mutlu-Altundag, E., Boyacioglu, E., and Capanoglu, E. (2018). Evaluation of Turkish propolis for its chemical composition, antioxidant capacity, anti-proliferative effect on several human breast cancer cell lines and proliferative effect on fibroblasts and mouse mesenchymal stem cell line. J. Apic. Res. 57, 627–638. doi:10.1080/00218839.2018.1494888
Parveen, Z., Deng, Y. L., Saeed, M. K., Dai, R. J., Ahamad, W., and Yu, Y. H. (2007). Antiinflammatory and analgesic activities of Thesium chinense Turcz extracts and its major flavonoids, kaempferol and kaempferol-3-O-glucoside. J. Pharm. Soc. Jpn. 127, 1275–1279. doi:10.1248/yakushi.127.1275
Perche, O., Vergnaud-Gauduchon, J., Morand, C., Dubray, C., Mazur, A., and Vasson, M. P. (2014). Orange juice and its major polyphenol hesperidin consumption do not induce immunomodulation in healthy well-nourished humans. Clin. Nutr. 33, 130–135. doi:10.1016/j.clnu.2013.03.012
Pironti, C., Ricciardi, M., Motta, O., Camin, F., Bontempo, L., and Proto, A. (2021). Application of 13C quantitative NMR spectroscopy to isotopic analyses for vanillin authentication source. Foods 10, 2635. doi:10.3390/foods10112635
Ramanan, M., Sinha, S., Sudarshan, K., Aidhen, I. S., and Doble, M. (2016). Inhibition of the enzymes in the leukotriene and prostaglandin pathways in inflammation by 3-aryl isocoumarins. Eur. J. Med. Chem. 124, 428–434. doi:10.1016/j.ejmech.2016.08.066
Róisín, O. f., Bergin, A., Flampouri, E., Mota, L. M., Butler, M., Quigley, A., et al. (2020). Mammalian cell culture for production of recombinant proteins: a review of the critical steps in their biomanufacturing. Biotechnol. Adv. 43, 107552. doi:10.1016/j.biotechadv.2020.107552
Shao, L. J., Sun, Y., Liang, J., Li, M. Q., and Li, X. L. (2019). Decolorization affects the structural characteristics and antioxidant activity of polysaccharides from Thesium chinense Turcz.: comparison of activated carbon and hydrogen peroxide decolorization. Int. J. Biol. Macromol. 155, 1084–1091. doi:10.1016/j.ijbiomac.2019.11.074
Singh, R., Singh, B., Singh, S., Kumar, N., Kumar, S., and Arora, S. (2008). Anti-free radical activities of kaempferol isolated from Acacia nilotica (L.) Willd. Ex. Del. Toxicol. Vitro 22, 1965–1970. doi:10.1016/j.tiv.2008.08.007
Soromou, L. W., Chen, N., Jiang, L., Huo, M., Wei, M., Chu, X., et al. (2012). Astragalin attenuates lipopolysaccharideinduced inflammatory responses by down-regulating NF-kappa B signaling pathway. Biochem. Biophys. Res. Commun. 419, 256–261. doi:10.1016/j.bbrc.2012.02.005
Steward, A. J., and Kelly, D. J. (2014). Mechanical regulation of mesenchymal stem cell differentiation. J. Anat. 765, 717–731. doi:10.1111/joa.12243
Strowig, T., Chijioke, O., Carrega, P., Arrey, F., Meixlsperger, S., Rämer, P. C., et al. (2010). Human NK cells of mice with reconstituted human immune system components require preactivation to acquire functional competence. Blood 116, 4158–4167. doi:10.1182/blood-2010-02-270678
Sudarshan, K., and Aidhen, I. S. (2016). Convenient synthesis of 3-glycosylated isocoumarins. Eur. J. Org. Chem. 2017, 34–38. doi:10.1002/ejoc.201601264
Vo, V. A., Lee, J. W., Shin, S. Y., Kwon, J. H., Lee, H. J., Kim, S. S., et al. (2014). Methyl p-hdroxycinnamate suppresses lipopolysaccharide-induced inflammatory responses through Akt phosphorylation in RAW264.7 cells. Biomol. Ther. 22, 10–16. doi:10.4062/biomolther.2013.095
Vyas, M., Requesens, M., Nguyen, T. H., Peigney, D., Azin, M., and Demehri, S. (2023). Natural killer cells suppress cancer metastasis by eliminating circulating cancer cells. Front. Immunol. 13, 1098445. doi:10.3389/fimmu.2022.1098445
Wei, Y., Xie, Q. Q., Fisher, D., and Sutherland, I. A. (2011). Separation of patuletin-3-O-glucoside, astragalin, quercetin, kaempferol and isorhamnetin from Flaveria bidentis (L.) Kuntze by elution-pump-out high-performance counter-current chromatography. J. Chromatogr. A 1218, 6206–6211. doi:10.1016/j.chroma.2011.01.058
Yang, W., Li, H. D., Liu, J. Y., Shao, H., Hua, J., and Luo, S. (2022). Degraded metabolites of phlorizin promote germination of Valsa mali var. Mali in its host Malus spp. J. Agric. Food Chem. 70, 149–156. doi:10.1021/acs.jafc.1c06206
Yu, C. S., Lai, K. C., Yang, J. S., Chiang, J. H., Lu, C. C., Wu, C., et al. (2010). Quercetin inhibited murine leukemia WEHI-3 cells in vivo and promoted immune response. Phytother. Res. 24, 163–168. doi:10.1002/ptr.2841
Yuan, Y., Long, Z., Xu, X., Wang, L., and Ying, M. (2006). Comparison of wild and cultured Thesium chinense turcz. on bacteriostasis and anti-inflammation. Chin. J. Pharm. 13, 219–222. doi:10.1016/S0379-4172(06)60071-1
Keywords: Thesium chinense Turcz., phenolic compounds, Bairui granules, active substances, cytotoxic activity
Citation: Zhang S, Chen H, Hua J and Luo S (2024) Cytotoxic activity of phenolic compounds in Bairui Granules obtained from the Chinese medicinal plant Thesium chinense. Front. Chem. 12:1506792. doi: 10.3389/fchem.2024.1506792
Received: 06 October 2024; Accepted: 19 November 2024;
Published: 02 December 2024.
Edited by:
Essa M. Saied, Humboldt University of Berlin, GermanyReviewed by:
Kasireddy Sudarshan, Purdue University, United StatesMaría Del Rayo Camacho Corona, Autonomous University of Nuevo León, Mexico
Ela Hoti, University of Medicine, Tirana, Albania
Pankaj Sharma, Rutgers University, Newark, United States
Sourav Ghorai, Enanta Pharmaceuticals, Inc., United States
Copyright © 2024 Zhang, Chen, Hua and Luo. This is an open-access article distributed under the terms of the Creative Commons Attribution License (CC BY). The use, distribution or reproduction in other forums is permitted, provided the original author(s) and the copyright owner(s) are credited and that the original publication in this journal is cited, in accordance with accepted academic practice. No use, distribution or reproduction is permitted which does not comply with these terms.
*Correspondence: Juan Hua, aHVhanVhbkBzeWF1LmVkdS5jbg==; Shihong Luo, bHVvc2hpaG9uZ0BzeWF1LmVkdS5jbg==
†ORCID: Shihong Luo, orcid.org/0000-0003-3500-3466