- 1Information Materials and Intelligent Sensing Laboratory of Anhui Province, Institutes of Physical Science and Information Technology, Anhui University, Hefei, China
- 2Leibniz International Joint Research Center of Materials Sciences of Anhui Province, Anhui University, Hefei, China
- 3Laboratory of Dielectric Functional Materials, School of Materials Science and Engineering, Anhui University, Hefei, China
Solid-state cooling, represented by the electrocaloric effect (ECE) in (anti)ferroelectric materials, has emerged as an alternative green refrigeration technology by virtue of its high efficiency and miniaturization and is expected to substitute conventional vapor-compression. Significant progress has been made in developing high-performance EC materials since its revival. However, anomalous EC behaviors are frequently observed, including asymmetric and negative EC profiles, and the physical mechanism behind this is still under debate. Its rationalization is of great importance since full utilization of anomalous EC behaviors could enhance EC strength and/or cooling capacity. This mini-review gives a brief overview of research advances in EC anomalies in (anti)ferroelectrics with the hope of provoking thought on the design of reconstructed refrigeration cycles and superior EC materials for application in solid-state cooling devices.
1 Introduction
Conventional vapor compression technology has always occupied a dominant status in device cooling. However, it is being gradually phased out due to its global-warming potential and the difficulty of scaling it down to cool miniaturized chips (Shi et al., 2019; Valant, 2012). As a typical solid-state cooling method, ferroelectric cooling based on the electrocaloric effect (ECE) stands out because of its merits of high efficiency, easy integration, and miniaturization. ECE is defined as an entropy change with electric-field (E) stimuli in polar dielectrics; superior EC performances are largely explored in relaxors and antiferroelectrics (AFEs) contributed by relaxor–ferroelectric (FE) and AFE–FE phase transitions, respectively (Qian et al., 2023; Lu and Zhang, 2009). Admittedly, compared to sole ferroelectric domain reorientations, phase transitions with higher entropy change are expected to enable the design of excellent EC materials; for example, a giant temperature change ΔT ∼ 12 K is found in AFE PbZr0.95Ti0.05O3 thin films and relaxor copolymers P(VDF-TrFE-CFE) (Mischenko et al., 2006; Neese et al., 2008). Under normal circumstances, exothermic and endothermic peaks appear at nearly equal height as E is applied and released (positive ECE), and an inverse situation is defined for negative ECE (NECE) (Fan et al., 2022; Wu et al., 2022). Similarly, remarkable differences in height for exothermic and endothermic peaks are featured as asymmetrical EC profiles (Figure 1A). A question that currently concerns the ferroelectric cooling community is what is the physical mechanism behind for anomalous electrocaloric behaviors (AECE) in (anti)ferroelectric ceramics. Unfortunately, AEC behaviors have not been seriously considered before, and an understanding of it is essential not only for fundamental research but also for enhancing EC strength and/or cooling capacity. This is the focus in this mini-review. To characterize ECE, direct methods (e.g., temperature reading and heat flow measurement) and indirect methods with Maxwell relations are usually adopted (Molin et al., 2017; Birks et al., 2017; Lu et al., 2019). Here, direct-measured AECE and NECE properties in bulk ceramics are discussed.
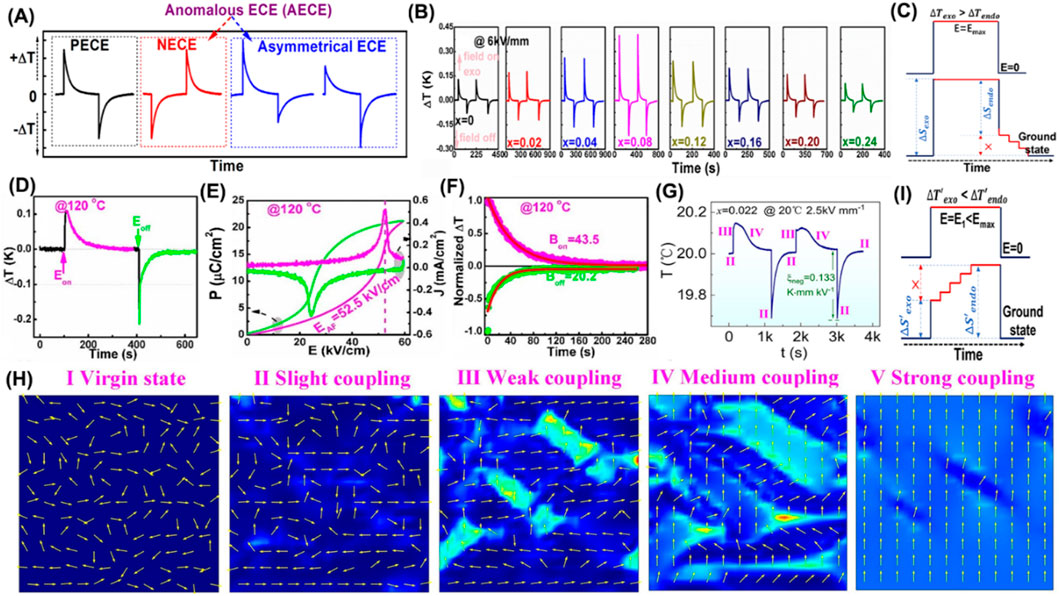
Figure 1. (A) Schematic profiles for positive ECE (PECE), negative ECE (NECE), and asymmetrical ECE. (B) EC profiles for poled Bi0.5Na0.5TiO3-BaTiO3-(Sr0.7Bi0.2□0.1)TiO3 (x = 0–0.24) ceramics; reproduced with permission from Li et al. (2017). (C) Schematic graph for
2 Anomalous electrocaloric behaviors in (anti)ferroelectric ceramics
2.1 Asymmetrical EC profiles
Usually, exothermic and endothermic peaks appearing as E applied and released in normal EC materials and entropy/temperature change in dual processes (denoted
1)
2)
2.2 Negative ECE in AFE
An insight into NECE is significant since a reorganized refrigeration cycle with the synergy of PECE and NECE is expected to improve the cooling efficiency. A giant NECE (ΔT ∼ −5 K) has been discovered in AFE La-doped Pb(Zr,Ti)O3 thin films, triggering research interest in NECE (Geng et al., 2015). The NECE is explained as an entropy increase by canting the dipoles under a moderate E. This mechanism could only account for NECE in a portion of AFEs, and instead it may be completely absent in other AFEs from low-to-high E sweep. Therefore, the underlying mechanism concerning NECE is still controversial. Novak et al. (2018) have determined that the EC property rests with temperature-dependent latent heat response and AFE coupling strength in Pb0.99Nb0.02 [(Zr0.58Sn0.43)0.92Ti0.08]0.98O3 (PNZST) ceramic (Figures 2A, B). A competitive role in AFE and FE strength, and latent heat finally produces an EC curve with an uncoordinated positive and negative EC evolution near separative FE–AFE and AFE–paraelectric (PE) phase transition regions, and NECE even disappears at high E across the AFE–PE phase boundary (Novak et al., 2018). Interestingly, a synergistic boosting of positive and negative ΔT is found in archetypal PbZrO3 AFE as E increases across AFE–FE and FE–PE phase transition (Figure 2C) instead of NECE extinction in PNZST ceramic. Vales-Castro et al. (2021) proposed an updated mechanism in which large NECE is based on endothermic AFE–FE switching instead of a main contribution from the dipole canting of the antiparallel lattice (Vales-Castro, et al., 2021). The similar EC behaviors are also found in B-site complex perovskite PbMg0.5W0.5O3 AFE, and a symmetric giant positive and negative ΔT appears at near room temperature (Li et al., 2021; Huang, et al., 2024).
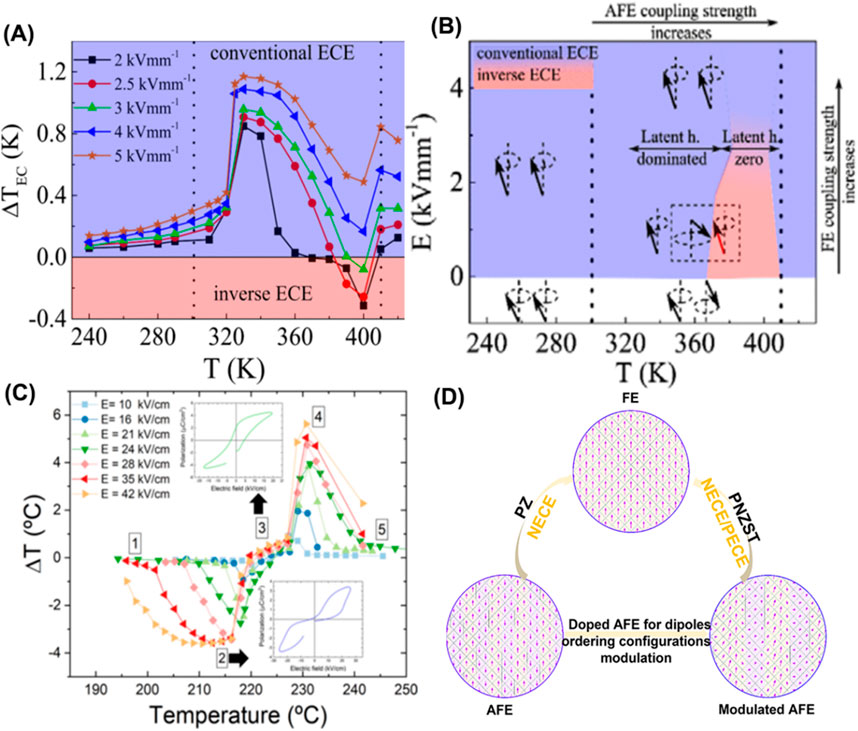
Figure 2. (A) PNZST ceramic features a crossover from positive to negative EC response with temperature and E. (B) Schematic representation of a possible mechanism responsible for EC behavior in PNZST; reproduced with permission from Novak et al. (2018). (C) ΔT of PbZrO3; the inset represents the P-E loops for different phases; reproduced with permission from Vales-Castro et al. (2021). (D) Dipoles ordering configurations in controlling EC behaviors in prototypical and modulated AFEs; adapted from Liu et al. (2020).
Experimental evidence demonstrates that the pristine (rigid) and doped (soft) AFEs present distinct EC behavior. The stringent antiparallel arrangement of adjacent electric dipoles is established in prototypical PbZrO3 AFE, and the entropy change contributor solely stems from pure AFE–FE and PE–FE switching in AFE and PE regions. Therefore, a latent-heat-mediated ECE comes from the endothermic AFE–FE (ΔT = −3.5 K) and exothermic PE–FE phase transition (ΔT = + 5.6 K). Notably, both positive and negative ΔT grow as long as E increases before breakdown. However, it is entirely different in chemical modified PNZST ceramics. The dopants change the rigid AFE order and evolve into an intermediate ferrielectric (FiE) state with a flexible configuration and imbalanced polarization. Such an FiE with a competitive AFE and FE order diversely impacts EC properties with external stimuli of temperature and E. Generally, as temperature increases, 1) FE order switching facilitates (ΔT > 0), 2) and AFE coupling strength enhances and leads to an endothermic AFE–FE phase transition (ΔT < 0); 3) with a possible emergence of AFE/FE nanoclusters (ΔT > 0). Therefore, EC behavior undergoes a complex evolution with a superposition between AFE/FE coupling strength and latent heat contributions in FiE. This is also confirmed in a Pb(Yb0.5Nb0.5)O3-Pb(Mg1/3Nb2/3)O3 (PYMN) FiE sample with erratic EC behavior, of which a hop–hop character, asymmetric EC response, and NECE is simultaneously found as temperature evolves (Li et al., 2023). In addition, instantaneous endothermal behavior is observed in a Pb0.97-xBaxLa0.02Zr0.95Ti0.05O3 (x = 0.04) sample, further illustrating a complex thermal response in AFEs (Li et al., 2020). A remarkable EC difference between pure PZ and PNZST/PYMN FiE is that negative ΔT for the latter will be offset under high E, strongly indicating the competitive role of AFE and FE phases in controlling EC performances. Therefore, the above two mechanisms proposed by Novak et al. (2018) and Vales-Castro et al. (2021) are not mutually incompatible but complement each other. Dipoles ordering configurations in AFEs thus play a decisive role in EC properties and should be analyzed case-by-case (Figure 2D).
2.3 Artificially engineered NEC behaviors
Except for AFEs, NECE can also be artificially engineered in FEs and relaxors. 1) The anisotropic (001)- and (011)-oriented 0.7Pb(Mg1/3Nb2/3)O3-0.3PbTiO3 (PMN-30PT) single crystal displays NECE under appropriate E and temperature; it originates from a monoclinic to tetragonal/orthogonal phase transition under noncollinear E (Figure 3A) (Li et al., 2020). Benefitting from the synergy of PECE and NECE in a (001)-oriented PMN-30PT single crystal at high (15 kV/cm) and low E (5 kV/cm), a significant 1.5× enhancement in cooling capacity is obtained by merging dual endothermic peaks (Figure 3B) (Li et al., 2017). Notably, this approach is easy to implement by simply adjusting interval time instead of a PE-to-AFE phase transition induced by the former EC cycle in AFEs (Li et al., 2020). 2) NECE can also be established by designing polar defects, such as in Ba0.9Sr0.1Hf0.1Ti0.9O3 ceramic. The pre-poled sample presents a ferro-restorable polarization feature capable of enhancing ΔT by up to 54% (Figure 3C). Moreover, both PECE and NECE emerge via a two-field step at Ed and Emax and enable a novel refrigeration cycle (Figures 3D, E). Therefore, the defect–dipole strategy is an elegant way to tailor EC performance in ferroelectrics (Li et al., 2021). Monte Carlo simulations also underscore the influence of defect dipoles on ECE in acceptor-doped BaTiO3 and reveal that in the case of antiparallel defect dipoles, the ECE can be positive or negative depending on the dipole density (Ma et al., 2016). 3) A hybrid normal ferroelectric/relaxor ferroelectric polymer blend is designed to obtain large cooling with an exclusion of a heating effect (Figures 3F, G); such a cooling response facilitates on-chip hotspot cooling. It is notable that this exotic EC response cannot occur in a sole neat copolymer and underlines the critical role of the relaxor end-member. This special EC response originates from the mesoscale dipolar interactions between ferroelectric/relaxor components, where dipole ordering in the poled relaxor polymer can be depolarized and stabilized with random distribution under a moderate inverse E, as simulated by a phase field (Figures 3H, I; Qian et al., 2016). The above artificial exotic EC behaviors may open many new application scenarios.
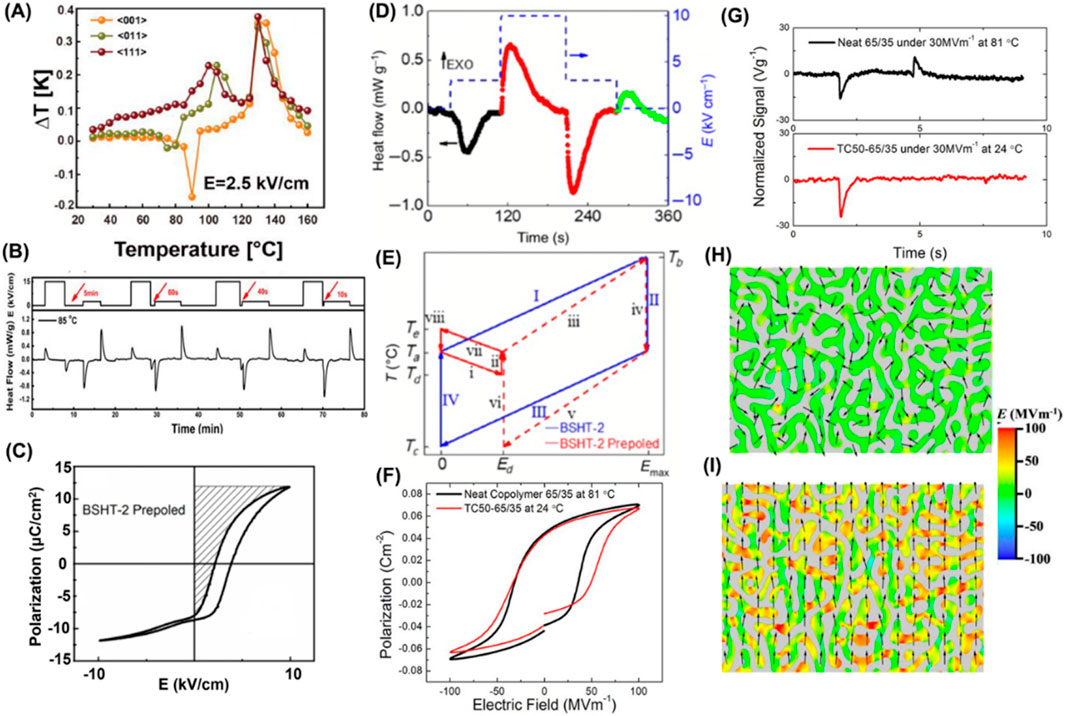
Figure 3. (A) Comparison of temperature-dependent ΔT for <001>-, <011>-, and <111>-oriented PMN-30PT single crystals; reproduced with permission from Li et al. (2020). (B) Alternate E1 = 15 and E2 = 2.5 kV/cm with varying interval time from 5 min to 10 s; reproduced with permission from Li et al. (2017). (C) Shifted P–E loop for pre-poled BSHT-2. (D) EC signal for poled BSHT-2. (E) Idealized refrigeration cycle utilizing both NECE and PECE based on a pre-poled BSHT-2 sample compared with conventional cycle; reproduced with permission from Li et al. (2021). (F, G) Comparison of P–E loops and heat-flux signals between TC50-65/35 blends and neat copolymer P(VDF-TrFE) 65/35 mol%. (H, I) Phase field simulation of dipolar direction distribution for TC50-65/35 blends in poled and de-poled states; reproduced with permission from Qian et al. (2016).
3 Concluding remarks
The electrocaloric effect is now a research frontier in solid-state cooling technology. The anomalous EC behaviors in (anti)ferroelectrics provide an alternative way of enhancing EC performance by realizing low-field high ΔT and engineering brand new refrigeration cycles. This mini-review provides an overview of research progress in asymmetrical EC profiles, negative EC, and engineered EC behaviors in bulk ceramics. However, the underlying physical mechanisms for these phenomena are still pending and deserve deeper exploration.
1) Although
2) It is necessary when using a direct method to rigorously claim negative EC performance in (anti)ferroelectrics since the leakage and unsaturated polarization in P–E loops may interfere with calculation procedures using Maxwell’s relation (Lu et al., 2021). Large PEC and NEC values are obtained in pure PZ ceramic across high AFE–FE and FE–PE transition temperature. As the dopant is incorporated into PZ to move the transition toward room temperature, the modulated AFE state in PNZST results in competitive AFE and FE states (and/or AFE/FE nanoclusters), and EC is either positive or negative depending on E and temperature. This in fact impairs the absolute EC value, and how to fulfill a separated AFE/FE EC response in modulated AFEs is important. This will realize a better coupling of PECE and NECE to boost cooling capacity. The artificial EC behaviors also deserve attention since they provide an alternative approach to achieving NECE in FE and relaxors and are expected to open new application scenarios.
3) Intrinsically, EC behaviors are optimized in near-phase transition regions, such as FE–FE, AFE/FE–PE, and FE–relaxor. Additionally, enhancing breakdown strength in (anti)ferroelectrics is an extrinsic factor to enhanced ECE, such as thick-film ceramics (Wang et al., 2022). Recent novel avenues are supposed to improve the ECE. i) By utilizing temperature and electric field compensation mechanisms, the laminated BNT-based compositions with discrete TFR are engineered (Lin et al., 2024). ii) Regulating the Schottky barrier at the grain boundary network in Ba0.8Zr0.2TiO3 ceramics and 2.4× enhancement of ΔT is achieved in annealed samples with a lower Schottky barrier (Xiao et al., 2024). Unfortunately, the design of large NEC materials seems to be elusive at present, and the method of improving PECE cannot be directly transferred to NECE. NECE may be even offset by PECE with increasing E, such as in soft PNZST AFE and <001>-PMN-PT single crystals; therefore, a critical E should be selected (Novak et al., 2018; Liao et al., 2024). It is thus promising though complex to artificially design high PECE and NECE in the future.
4) Theoretical models such as phase-field simulations and Monte Carlo (MC) simulations should be continuously optimized to predict and reveal series of high-performance EC materials, thus deepening recognition of the related physical mechanism (Hou et al., 2024; Fan et al., 2022; Xu et al., 2024). In addition to widely explored inorganic oxide counterparts, newly discovered molecular AFE and organometallic perovskite exhibit intriguing EC behaviors and reveal their great potential for solid-state refrigeration (Xu et al., 2021; Han et al., 2024). In summary, we hope that this mini-review serves as a catalyst for further development of high-performance EC materials and related physical mechanisms in the ferroelectric cooling community, laying a solid foundation for future practical applications.
Author contributions
FL: writing–original draft and writing–review and editing. CW: writing–review and editing. LS: writing–review and editing.
Funding
The authors declare that financial support was received for the research, authorship, and/or publication of this article. This work was supported by the National Natural Science Foundation of China (Nos 12104001 and 12174001).
Conflict of interest
The authors declare that the research was conducted in the absence of any commercial or financial relationships that could be construed as a potential conflict of interest.
Publisher’s note
All claims expressed in this article are solely those of the authors and do not necessarily represent those of their affiliated organizations, or those of the publisher, the editors, and the reviewers. Any product that may be evaluated in this article, or claim that may be made by its manufacturer, is not guaranteed or endorsed by the publisher.
References
Birks, E., Dunce, M., Peräntie, J., Hagberg, J., and Sternberg, A. (2017). Direct and indirect determination of electrocaloric effect in Na0.5Bi0.5TiO3. J. Appl. Phys. 121, 224102. doi:10.1063/1.4985067
Fan, N. B., Íñiguez, J., Bellaiche, L., and Xu, B. (2022). Origin of negative electrocaloric effect in Pnma-type antiferroelectric perovskites. Phys. Rev. B 106, 224107. doi:10.1103/PhysRevB.106.224107
Geng, W. P., Liu, Y., Meng, X. J., Bellaiche, L., Scott, J. F., Dkhil, B., et al. (2015). Giant negative electrocaloric effect in antiferroelectric La-doped Pb(ZrTi)O3 thin films near room temperature. Adv. Mater. 27, 3165–3169. doi:10.1002/adma.201501100
Guo, H. Z., Ma, C., Liu, X. M., and Tan, X. L. (2013). Electrical poling below coercive field for large piezoelectricity. Appl. Phys. Lett. 102, 092902. doi:10.1063/1.4794866
Han, S. G., Bie, J., Fa, W., Chen, S., Tang, L. W., Guo, W. Q., et al. (2024). Field-induced antiferroelectric-ferroelectric transformation in organometallic perovskite displaying giant negative electrocaloric effect. J. Am. Chem. Soc. 146, 8298–8307. doi:10.1021/jacs.3c13422
Hou, X., Bin, C. W., Zheng, S. Z., Gao, Z. G., Chen, P., Wang, J., et al. (2024). Room temperature electrocaloric effect in PTO/STO superlattice induced by topological domain transition. Acta Mater 277, 120152. doi:10.1016/j.actamat.2024.120152
Huang, Y. Y., Zhang, L. Y., Ge, P. J., Yang, Y. L., Jing, R. Y., Shur, V., et al. (2024). Boosting room-temperature electrocaloric performance in B-site complex antiferroelectrics: a synergistic design approach. Acta Mater 277, 120177. doi:10.1016/j.actamat.2024.120177
Li, F., Chen, G., Liu, X., Zhai, J., Shen, B., Zeng, H. R., et al. (2017). Phase-composition and temperature dependence of electrocaloric effect in lead-free Bi0.5Na0.5TiO3-BaTiO3-(Sr0.7Bi0.2□0.1)TiO3 ceramics. J. Eur. Ceram. Soc. 37, 4732–4740. doi:10.1016/j.jeurceramsoc.2017.06.033
Li, F., Liu, W., Lou, X. J., Zhai, J. W., and Wang, C. C. (2022). Isothermal phase transition across phase boundary in (Pb0.95Ba0.05)ZrO3 ceramics. Appl. Phys. Lett. 120, 023902. doi:10.1063/5.0075892
Li, F., Long, M. S., Lou, X. J., Wang, C. C., and Shan, L. (2023). Order-degree-modulated ferroic response and an unconventional electrocaloric effect in B-site complex systems: a case study in Pb[(Yb1/2Nb1/2)0.84(Mg1/3Nb2/3)0.16]O3 ceramic. J. Phys. D. Appl. Phys. 56, 405501. doi:10.1088/1361-6463/ace1fe
Li, G. H., Shi, C., Zhu, K., Ge, G. L., Yan, F., Lin, J. F., et al. (2022). Achieving synergistic electromechanical and electrocaloric responses by local structural evolution in lead-free BNT-based relaxor ferroelectrics. Chem. Eng. J. 431, 133386. doi:10.1016/j.cej.2021.133386
Li, J. J., Li, J. T., Wu, H. H., Qin, S. Q., Su, X. P., Wang, Y., et al. (2020). Giant electrocaloric effect and ultrahigh refrigeration efficiency in antiferroelectric ceramics by morphotropic phase boundary design. ACS Appl. Mater. Interfaces 12, 45005–45014. doi:10.1021/acsami.0c13734
Li, J. J., Su, X. P., Wu, H. H., Li, J. T., Qin, S. Q., Yin, R. W., et al. (2022). Electric hysteresis and validity of indirect electrocaloric characterization in antiferroelectric ceramics. Scr. Mater. 216, 114763. doi:10.1016/j.scriptamat.2022.114763
Li, J. J., Wu, H. H., Li, J. T., Su, X. P., Yin, R. W., Qin, S. Q., et al. (2021). Room-temperature symmetric giant positive and negative electrocaloric effect in PbMg0.5W0.5O3 antiferroelectric ceramic. Adv. Funct. Mater. 33, 2101176. doi:10.1002/adfm.202101176
Li, J. N., Lv, J., Zhang, D. W., Zhang, L. X., Hao, X. H., Wu, M., et al. (2021). Doping-induced polar defects improve the electrocaloric performance of Ba0.9Sr0.1Hf0.1Ti0.9O3. Phys. Rev. Appl. 16, 014033. doi:10.1103/PhysRevApplied.16.014033
Li, J. T., Qin, S. Q., Bai, Y., Li, J. J., and Qiao, L. J. (2017). Flexible control of positive and negative electrocaloric effects under multiple fields for a giant improvement of cooling capacity. Appl. Phys. Lett. 111, 093901. doi:10.1063/1.4997068
Li, J. T., Yin, R. W., Su, X. P., Wu, H. H., Li, J. J., Qin, S. Q., et al. (2020). Complex phase transitions and associated electrocaloric effects in different oriented PMN-30PT single crystals under multi-fields of electric field and temperature. Acta Mater 182, 250–256. doi:10.1016/j.actamat.2019.11.017
Liao, L. C., Shan, D. L., Lei, C. H., Pan, K., Li, J. Y., and Liu, Y. Y. (2024). Revealing the mechanisms of electrocaloric effects by simultaneously direct measuring local electrocaloric and electrostrain under ambient conditions. Acta Mater 278, 120264. doi:10.1016/j.actamat.2024.120264
Lin, W. K., Li, G. H., Qian, J., Ge, G. L., Wang, S. M., Lin, J. F., et al. (2024). Broadening the operating temperature span of the electrocaloric effect in lead-free ceramics via creating multi-stage phase transitions. J. Mater. Chem. A 12, 16438–16446. doi:10.1039/d4ta02319f
Liu, H., Zhou, Z. Y., Qiu, Y., Gao, B. T., Sun, S. D., Lin, K., et al. (2020). An intriguing intermediate state as a bridge between antiferroelectric and ferroelectric perovskites. Mater. Horiz. 7, 1912–1918. doi:10.1039/D0MH00253D
Lu, B., Yao, Y. B., Jian, X. D., Tao, T., Liang, B., Zhang, Q. M., et al. (2019). Enhancement of the electrocaloric effect over a wide temperature range in PLZT Ceramics by doping with Gd3+ and Sn4+ ions. J. Eur. Ceram. Soc. 39, 1093–1102. doi:10.1016/j.jeurceramsoc.2018.11.042
Lu, S. G., Lin, X. W., Li, J., Li, D. D., Yao, Y. B., Tao, T., et al. (2021). Enhanced electrocaloric strengths at room temperature in (SrxBa1-x)(Sn0.05Ti0.95)O3 lead-free ceramics. J. Alloys Compd. 871, 159519. doi:10.1016/j.jallcom.2021.159519
Lu, S. G., and Zhang, Q. M. (2009). Electrocaloric materials for solid-state refrigeration. Adv. Mater. 21, 1983–1987. doi:10.1002/adma.200802902
Ma, Y. B., Grünebohm, A., Meyer, K. C., Albe, K., and Xu, B. X. (2016). Positive and negative electrocaloric effect in BaTiO3 in the presence of defect dipoles. Phys. Rev. B 94, 094113. doi:10.1103/PhysRevB.94.094113
Mischenko, A. S., Zhang, Q., Scott, J. F., Whatmore, R. W., and Mathur, N. D. (2006). Giant electrocaloric effect in thin-film PbZr0.95Ti0.05O3. Science 311, 1270–1271. doi:10.1126/science.1123811
Molin, C., Peräntie, J., Goupil, F. L., Weyland, F., Sanlialp, M., Stingelin, N., et al. (2017). Comparison of direct electrocaloric characterization methods exemplified by 0.92Pb(Mg1/3Nb2/3)O3-0.08PbTiO3 multilayer ceramics. J. Am. Ceram. Soc. 100, 2885–2892. doi:10.1111/jace.14805
Neese, B., Chu, B. J., Lu, S. G., Wang, Y., Furman, E., and Zhang, Q. M. (2008). Large electrocaloric effect in ferroelectric polymers near room temperature. Science 321, 821–823. doi:10.1126/science.1159655
Novak, N., Weyland, F., Patel, S., Guo, H. Z., Tan, X. L., Rödel, J., et al. (2018). Interplay of conventional with inverse electrocaloric response in (Pb,Nb)(Zr,Sn,Ti)O3 antiferroelectric materials. Phys. Rev. B 97, 094113. doi:10.1103/PhysRevB.97.094113
Qian, X. S., Chen, X., Zhu, L., and Zhang, Q. M. (2023). Fluoropolymer ferroelectrics: Multifunctional platform for polar-structured energy conversion. Science 380, eadg0902. doi:10.1126/science.adg0902
Qian, X. S., Yang, T. N., Zhang, T., Chen, L. Q., and Zhang, Q. M. (2016). Anomalous negative electrocaloric effect in a relaxor/normal ferroelectric polymer blend with controlled nano- and meso-dipolar couplings. Appl. Phys. Lett. 108, 142902. doi:10.1063/1.4944776
Shi, J. Y., Han, D. L., Li, Z. C., Yang, L., Lu, S. G., Zhong, Z. F., et al. (2019). Electrocaloric cooling materials and devices for zero-global-warming-potential, high-efficiency refrigeration. Joule 3, 1200–1225. doi:10.1016/j.joule.2019.03.021
Su, X. P., Li, J. J., Hou, Y. X., Yin, R. W., Li, J. T., Qin, S. Q., et al. (2023). Large electrocaloric effect over a wide temperature span in lead-free bismuth sodium titanate-based relaxor ferroelectrics. J. Materiomics 9, 289–298. doi:10.1016/j.jmat.2022.10.005
Valant, M. (2012). Electrocaloric materials for future solid-state refrigeration technologies. Prog. Mater. Sci. 57, 980–1009. doi:10.1016/j.pmatsci.2012.02.001
Vales-Castro, P., Faye, R., Vellvehi, M., Nouchokgwe, Y., Perpiñà, X., Caicedo, J. M., et al. (2021). Origin of large negative electrocaloric effect in antiferroelectric PbZrO3. Phys. Rev. B 103, 054112. doi:10.1103/PhysRevB.103.054112
Wang, S. B., Zhao, P. F., Jian, X. D., Yao, Y. B., Tao, T., Liang, B., et al. (2022). Large energy storage density and electrocaloric strength of Pb0.97La0.02(Zr0.46-xSn0.54Tix)O3 antiferroelectric thick film ceramics. Scr. Mater. 210, 114426. doi:10.1016/j.scriptamat.2021.114426
Wen, L. J., Yin, J., Wu, X. J., Wei, X. W., Liu, W. B., Yang, D. Y., et al. (2023). Comprehending the underlying mechanism behind directly/indirectly obtained large electrocaloric response in Bi0.5Na0.5TiO3-based relaxor ferroelectrics. Acta Mater 255, 119090. doi:10.1016/j.actamat.2023.119090
Wu, M., Xiao, Y. N., Li, H. Q., Liu, Y. B., Gao, J. H., Zhong, L. S., et al. (2022). Negative electrocaloric effects in antiferroelectric materials: a review. J. Inorg. Mater. 37, 376–386. doi:10.15541/jim20210420
Xiao, W. R., Zhang, C., Gong, X. T., Qiu, S. Y., Wang, J. Y., Zhang, H. B., et al. (2024). Enhancement of electrocaloric effect in ferroelectric polycrystalline ceramics through grain boundary barrier engineering. Adv. Funct. Mater. 2405241. doi:10.1002/adfm.202405241
Xu, H. J., Guo, W. Q., Wang, J. Q., Ma, Y., Han, S. G., Liu, Y., et al. (2021). A metal-free molecular antiferroelectric material showing high phase transition temperatures and large electrocaloric effects. J. Am. Chem. Soc. 143, 14379–14385. doi:10.1021/jacs.1c07521
Xu, K., Shi, X. M., Shao, C. C., Dong, S. Z., and Huang, H. B. (2024). Design of polar boundaries enhancing negative electrocaloric performance by antiferroelectric phase-field simulations. npj Comput. Mater. 10, 150. doi:10.1038/s41524-024-01334-2
Keywords: electrocaloric effect, anomalous ECE, relaxors, antiferroelectrics, solid-state cooling
Citation: Li F, Wang C and Shan L (2024) Anomalous electrocaloric behaviors in (anti)ferroelectrics: a mini-review. Front. Chem. 12:1476273. doi: 10.3389/fchem.2024.1476273
Received: 05 August 2024; Accepted: 25 September 2024;
Published: 23 October 2024.
Edited by:
Ming Wu, Xi’an Jiaotong University, ChinaReviewed by:
Sheng-Guo Lu, Guangdong University of Technology, ChinaCopyright © 2024 Li, Wang and Shan. This is an open-access article distributed under the terms of the Creative Commons Attribution License (CC BY). The use, distribution or reproduction in other forums is permitted, provided the original author(s) and the copyright owner(s) are credited and that the original publication in this journal is cited, in accordance with accepted academic practice. No use, distribution or reproduction is permitted which does not comply with these terms.
*Correspondence: Feng Li, fengli@ahu.edu.cn