- 1School of Chemistry and Chemical Engineering, Guangzhou University, Guangzhou, China
- 2School of Life Sciences, Sun Yat-sen University, Guangzhou, China
Single-atom nanozymes (SAzymes) represent a cutting-edge advancement in nanomaterials, merging the high catalytic efficiency of natural enzymes with the benefits of atomic economy. Traditionally, natural enzymes exhibit high specificity and efficiency, but their stability are limited by environmental conditions and production costs. Here we show that SAzymes, with their large specific surface area and high atomic utilization, achieve superior catalytic activity. However, their high dispersibility poses stability challenges. Our review focuses on recent structural and preparative advancements aimed at enhancing the catalytic specificity and stability of SAzymes. Compared to previous nanozymes, SAzymes demonstrate significantly improved performance in biomedical applications, particularly in tumor medicine. This progress positions SAzymes as a promising tool for future cancer treatment strategies, integrating the robustness of inorganic materials with the specificity of biological systems. The development and application of SAzymes could revolutionize the field of biocatalysis, offering a stable, cost-effective alternative to natural enzymes.
1 Introduction
The specific structure of enzymes determines their high catalytic efficiency and catalytic specificity. Most natural enzymes, which are proteins produced by living organisms (Jiang and Liang, 2021), have activity that is highly dependent on specific environmental conditions such as pH and temperature, making them susceptible to deactivation. In addition, natural enzymes have high production costs, low yields, and are difficult to apply on a large scale in industrial production due to their limited lifespan and difficulty in recycling. To overcome these limitations, scientists have been exploring alternative solutions, hoping to find an artificial enzyme with high catalytic efficiency, strong stability, low cost, and simple preparation process. Nanozymes have therefore been proposed. Nanozymes encompass a variety of types, ranging from the metal oxide nanozymes initially discovered by Gao et al. (2007), to later developments in metal organic frameworks (Li et al., 2020), and more recently, to polypeptide protein aggregates. Unlike natural enzymes, which derive their activity from protein folding, nanozymes are characterized by their assembly into nanostructures, leading to distinct mechanisms of activity. Erkang Wang defined nanozymes as enzymes that still exhibit high catalytic activity over a wide temperature range and pH range (Nanozymes, 2018). Its essence is a nanomaterial, and the nanomaterial effect enables it to exhibit the efficient catalytic activity of natural enzymes.
Despite the advantages of nanozymes, their catalytic activity often falls short of natural enzymes due to a low density of active centers (Sun et al., 2022; Xiao et al., 2023). In recent years, Single-atom catalysts (SACs) was prepared, a type of catalyst uniformly disperses single atoms on the carrier with a certain distance. This certain distance endow SACs with high catalytic activity (Figure 1). In addition, as research on SACs deepens, the concept of single-atom nanozymes (SAzymes) emerged accordingly (Xie et al., 2023). The metal centers of these SAzymes are highly dispersed (Cai et al., 2023), and the catalytic sites are uniformly distributed as single atoms, thus greatly improving the atomic utilization efficiency of the active center (Peng et al., 2024). Its catalytic activity is 10–100 times that of traditional nanozymes, almost reaching the level of natural enzymes (Pei et al., 2020). Designing active sites with atomic-level distribution on the carrier can maximize atomic regulation, thereby bridging the gap in efficiency, selectivity, and catalytic performance between natural enzymes and SAzymes (Xu et al., 2021). Due to the high catalytic activity of SAzymes, they can effectively respond to the acidic and hypoxic microenvironment of tumors. By utilizing abundant substrates, such as hydrogen peroxide (H2O2), SAzymes generate reactive oxygen species (ROS) that induce apoptosis in cancer cells and deplete the reducing agents or nutrients necessary for their survival. This process ultimately facilitates effective antitumor therapies (Guo et al., 2024). Additionally, the hypoxic microenvironment not only hinders the antitumor activity of related immune cells but also fosters the proliferation and metastasis of tumor cells. The modulation of the tumor hypoxic microenvironment by SAzymes can enhance the oxygen content at tumor sites (Jiang et al., 2024).
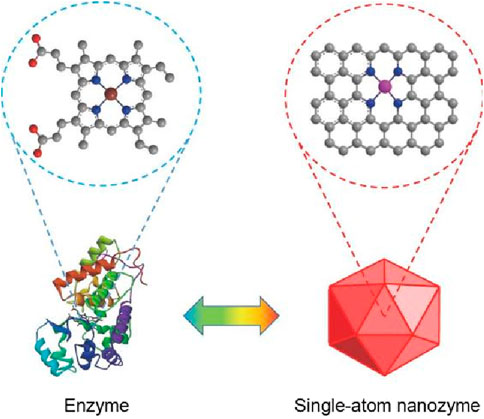
Figure 1. Similar catalytic activity between enzymes and single-atom nanozymes (Jiang and Liang, 2021).
This article summarizes the structural characteristics and preparation methods of SAzymes in recent years and introduces the latest progress of SAzymes in tumor medicine such as biosensing and anti-tumor. SAzymes precisely construct and regulate the active center at the atomic level (Shen et al., 2024), and their small size at the micro nano level endows them with high specific surface area and high atomic utilization, thus possessing efficient catalytic activity comparable to proteases. By carefully regulating the active center of SAzymes to resemble that of natural enzymes, their catalytic activity can be increased (Xie et al., 2020; Chen et al., 2023a; Wang et al., 2023a; Yang et al., 2023). However, this atomic scale also poses great challenges to the structural regulation of SAzymes (Bhalothia et al., 2024). Meanwhile, SAzymes lack a certain degree of specificity. To address this issue, organic frameworks are generally used to regulate the local coordination structure of the central atom.
SAzymes have been widely used in the field of biomedicine, especially in the field of oncology; The research mainly focuses on its catalytic performance, and there is relatively little research on whether it will bring side effects; In addition, nowadays, what widely used are non-precious metals such as, Fe, Ni, this limits the types of materials available, and future researches need to focus on the integration and application of the Materials Genome Initiative (Figure 2).
2 The foundation of metal organic framework type single-atom nanozymes
In the field of catalysis, first principles are usually used to design SAzymes from a theoretical perspective. At present, most SAzymes belong to metal organic framework type SAzymes. Isolated metal atoms tend to aggregate, so SAzymes are generally difficult to exhibit the desired effect. The solution is to disperse SAzymes in some frameworks to increase the interaction between individual metal atoms and substrates, in order to solve the problem of metal single atom clusters (Chen et al., 2021; Bhalothia et al., 2024). Carbon based materials have a large surface area and strong interactions with metal centers, making them the first choice for constructing SAzymes (Wan et al., 2023). This organic framework can also interact with metal central ions to affect the local coordination structure of active sites, thereby altering its catalytic performance (Xie et al., 2022). In order to improve the dispersion of atoms, hydrocarbons with a network structure are generally chosen, which act like cages separating the centers of metal atoms. Commonly used compounds with hydrocarbon network structures include zeolitic imidazolic acid skeleton-8 (ZIF-8), carbon dots (CDs), and ZIF-67. In addition, the higher dispersion of single atoms increases their specific surface area, thus increasing their catalytic activity (Wang and Zhou, 2021). They have been proven to function like natural enzymes (Zhang et al., 2022; Zhu et al., 2023a). The common preparation methods for SAzymes currently include vapor deposition method, one-pot solvothermal method, pyrolysis method (Hua et al., 2024).
2.1 The application of first principles calculations in the design of single-atom nanozymes
First principles calculations, especially those based on density functional theory (DFT), have become one of the core tools in modern catalytic science. These calculation methods are based on basic physical laws and do not rely on any empirical parameters, understanding and predicting the structure and reaction mechanism of catalysts from a microscopic level. By accurately calculating the electronic structure, density functional theory has helped researchers reveal the properties of catalyst active sites, which are the most critical parts of catalytic reactions.
In the computational research of catalysts, the determination of active sites is crucial. Evaluation methods include several key calculations and analyses. Calculating adsorption energy is used to determine the stability of molecules on the catalyst surface. Density of States (DOS, can be used to evaluate orbital components) analysis is then employed to study the distribution and energy level structure of electronic states, which includes calculating the d-band center. Bader charge analysis (can be used to qualitatively calculate valence) provides an accurate calculation of charge distribution, helping to understand the relationship between charge migration and catalytic activity. Crystal Orbital Hamiltonian Population (COHP) analysis is used to identify interactions between molecular orbitals. Zero-point energy correction (ZPE, makes the evaluation of reaction energy more accurate) is applied to evaluate the impact of quantum mechanical effects on catalytic activity.
Bolong Xu et al. (2022) used DOS analysis and found that compared to FeN4 SAzyme, the DOS of FeN5 SAzyme is significantly higher near the Fermi level, and several new hybrid electronic states appear, indicating a stronger interaction between FeN5 SAzyme and H2O2. Wang et al. (2022b) used adsorption energy, Bader charge, COHP analysis and DOS analysis to illustrate a better catalytic performance of MnSA-N3-C compared to MnSA-N4-C. The exploration of computational methods has never stopped. Due to the fact that the d-band center theory is often more suitable for metal atoms in clusters with close distances. Their energy levels overlap, so strong hybridization can be observed in a wide energy range on DOS. However, for SAzymes, metal atoms are far apart, and energy levels split under the action of crystal fields, corresponding to different frontier orbitals. For example, for Au atomic catalysts, there have been numerous studies discussing their catalytic activity (Camellone and Fabris, 2009; Zhou et al., 2018), but no consensus has been reached. However, Fu et al. (2020) used the frontier orbital method to analyze the orbital overlap between Au atoms and substrates, and successfully obtained an accurate dominant configuration of Au atom catalysts in the absence of a contradiction between d-band center theory and adsorption energy. According to the actual situation, the catalytic mechanism of SAzymes can be more comprehensively elucidated by combining multiple theoretical calculation methods. In addition to calculations in conventional environments, simulating catalytic reaction conditions in more complex environments (such as temperature, pressure, and chemical environment) makes catalytic systems more efficient. Zou et al. (2020) considered the influence of pressure on adsorption energy in their study of N2 reduction, designed more specific reaction pathways, and provided more specific activation energy values, which is of great significance for catalyst selection.
Once the application of first principles methods in catalysis is established, it is essential to design experimental methods to identify the necessary transition states. The introduction of the Materials Genome Initiative can be seen as an innovative solution to the current limited development in the field of SAzymes. By systematically modeling potential catalyst skeletons and replacing their central metal atoms, this strategy can generate a wide variety of different catalyst structures. On this basis, utilizing adsorption module in advanced materials science tools such as pymatgen and ase can efficiently generate multiple adsorption site models (Ong et al., 2013; Larsen et al., 2017). Subsequently, minimizing the energy of these models can not only find the ground state structure of the system, but also conduct further adsorption energy analysis. This method effectively combines computational chemistry and experimental chemistry. The allocation of experimental resources can be optimized with large-scale pre-screening before experimental operations.
Following the preliminary evaluation of active sites, first principles calculation methods such as Bader charge analysis, COHP, and DOS analysis can be employed to meticulously compare the catalytic performance of different SAzymes with the same reaction substrate. Bader charge analysis is mainly used to quantitatively understand the degree of charge transfer between metal atoms and reaction substrates; COHP analysis provides a method for evaluating the strength of chemical bonds between metal atoms and reaction substrates. By analyzing the contributions of each energy level in the energy band, we can understand the covalent and ionic properties of chemical bonds, thereby predicting the tendency of chemical bond breakage and formation in chemical reactions. DOS analysis focuses on the hybridization degree of orbitals between metal atoms and reaction substrates, especially in transition metal catalysts, where the p-d hybridization phenomenon between their d-orbitals and the p-orbitals of reaction substrates is particularly important. For catalysts containing transition metals with d-orbitals, magnetic exchange mechanisms [including direct exchange (Van Vleck, 1953), super exchange (Anderson, 1959) and double exchange (De Gennes, 1960)] are also dynamically affect the progress of catalytic reactions. These mechanisms affect electrons’ control towards the reaction pathway by altering the spin arrangement of electrons. This complex interaction is crucial in catalytic science as it can determine the stability and activity of catalysts in different reaction environments (Figure 3).
2.2 Structure and properties
The essence of SAzymes is nanomaterial. The activity and specificity of nanomaterials is different because of their different structure, so the effectiveness of their application in tumor treatment is also different. Reasonably designing the types of central atoms and ligands can effectively regulate DOS and d-orbital states of central atoms, optimize the adsorption free energy of substrate and thus efficiently promote its conversion (Wan et al., 2023).
After selecting the central atom, the common method is to change the ligand. This alters the charge distribution and coordination environment, enabling precise regulation of the active sites of SAzymes by modifying the local coordination structure (Figure 4A). This can better simulate the active sites and spatial configurations of natural enzymes and improve their catalytic activity. The more similar the structure of this complex is to that of a natural enzyme, the higher its catalytic activity. By examining the free energy diagram of SAzymes, the catalytic activity of the catalyst can be roughly determined (Chen et al., 2023a) (Figure 4B).
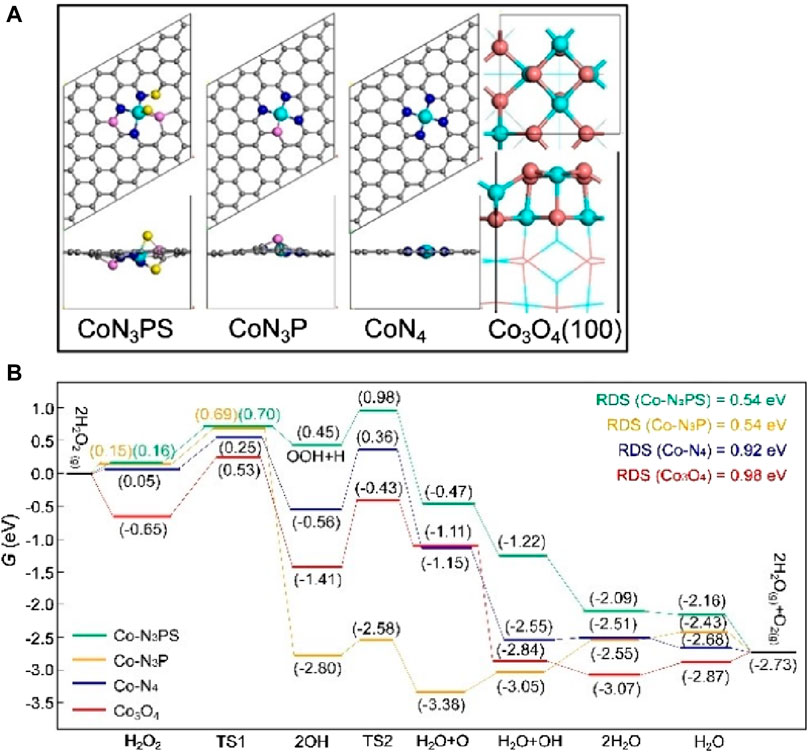
Figure 4. (Chen et al., 2023a) (A) The top and side view structures of Co-N3PS, Co-N3P, CoN4 and Co3O4. (B) Under neutral conditions, free energy diagrams of possible catalytic processes for Co-N3PS, Co-N3P, CoN4 and Co3O4 Color code: Co (green), C (gray), N (blue), P (pink), S (yellow), O (orange).
CDs are a type of carbon based material with ultra small size and abundant surface functional groups, which have significant advantages in riveting and exposing active metal sites. Therefore, the rich oxygen-containing groups on carbon dots can be utilized to regulate the local coordination structure by interacting with metal centers (Zhang et al., 2023). Yang et al. (2023) utilized CDs as organic frameworks to adjust the active centers of SAzymes, resulting in a morphology similar to that of peroxidase (POD). Due to the coordination between CDs and Fe3+, atomically dispersed Fe (II) can be stabilized by oxygen atoms, forming Fe-O3N2. The active site structure of iron SAzymes (Fe-O3N2) is similar to that of (S) -2-hydroxypropyl and 1-phosphonate (S-HPP) epoxidase (HppE), a typical natural non heme iron based peroxidase (Figure 5C). This iron SAzymes have relatively saturated single iron atom sites, so its POD-like activity is 750 units/mg, much higher than the traditional SAzymes of Fe-N4 and Fe-N3P with planar structures (316 units/mg), and natural Horseradish Peroxidase, (HRP) (504 units/mg).
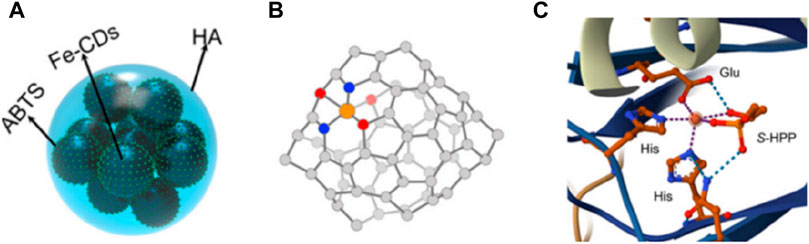
Figure 5. (A) The model of Fe-CDs/ABTS@HA. (B) The catalytic active center of Fe-CDs/ABTS@HA. (C) The catalytic active center of HppE.
The interaction between sulfur atoms and metal centers also makes it possible to regulate local coordination structures. In 2024, Wendong Liu et al. (2024) utilized the doping of S atoms to disrupt the symmetric charge distribution around the Fe single atom, and designed Fe-S/N-C SAzymes (coordination structure is Fe-N3S1) with asymmetric charge distribution. Bader charge analysis shows that the charge of iron atoms in the asymmetric coordination structure Fe-N3S1 is lower than that in the symmetric coordination structure Fe-N4. This enhanced electron localization can optimize the adsorption energy of oxygen-containing substances such as H2O2, endowing Fe-S/N-C SAzymes with strong POD-like activity (Xu et al., 2023). Based on measurements and calculations using the Arrhenius equation, the activation energies (Ea) for H2O2 activation catalyzed by Fe-S/N-C and Fe-N-C are 10.31 kJ/mol and 18.12 kJ/mol, respectively. According to the electron spin resonance (ESR) spectrum, Fe-S/N-C exhibits a higher intensity (f OH dimethylpyridine nitrate oxide) DMPO signal, confirming its superior POD-like activity. The rate constant of Fe-S/N-C consuming glutathione (GSH) is 0.46 min−1, which is higher than the rate constant of Fe-N-C consuming GSH (0.04 min−1), indicating that Fe-S/N-C has higher GSH-like activity. In 2024, Xu et al. (2024) designed a copper based SAzyme (Cu-N/S-C SAzymes) using sulfur engineering technology, which introduces S atoms into the catalyst to redistribute the electrons of copper atoms, presenting an asymmetric distribution. The introduction of S atoms enhances the electron transfer between the active center Cu-N1S2 and H2O2, and also promotes the adsorption and activation process of H2O2, resulting in better generation of OH. Cu-N/S-C SAzymes exhibiting higher catalase-like(CAT-like) activity compared to Cu-N-C SAzymes with Cu-N3 sites, the affinity of Cu-N/S-C SAzymes for hydrogen peroxide substrate increased by 13.8 times, and the catalytic efficiency increased by 65.2 times. Meanwhile, Cu-N/S-C SAzymes have a high photothermal conversion efficiency (31.7%) and good photothermal cycling stability, which makes them potential as photothermal agents in tumor treatment.
Changing the type of carrier gas can alter the valence state of the central atom, thereby altering its local coordination environment. In 2022, Wang et al. (2022a) utilizes the abundant and adjustable defects on the surface of TiO2 to fine tune the local coordination environment of the active site (Co atom). The XPS results showed that cobalt based SAzymes (Co/A-TiO2 SAzymes) calcined in air had a lower Co2+/Co3+ ratio than cobalt based single atom nanozymes (Co/N-TiO2 SAzymes) calcined in nitrogen. Theoretical studies have shown that the lower the charge of Co, the higher the activity of catalyzing the decomposition of H2O2 into O2; The Michaelis Menten kinetic experiment results indicate that at pH 6.5, the Michaelis Menten constant (Km) of Co/A TiO2 catalyzing H2O2 is 1.95 mM, and the maximum initial velocity (Vmax) is 1.22 × 10−6 M s−1, which is superior to most single atom nanozymes reported in the past (their Vmax value is one quantity higher than previous systems). Therefore, Co/A-TiO2 SAzyme calcined in air has higher catalytic activity.
In order to enhance the practicality of single atom nanozymes, coatings can be added on the surface of SAzymes to give them some certain functions. In order to improve the colloidal stability and prolong the blood circulation time of CDs doped iron SAzymes (Fe-CDs), Qingyuan Yang et al. (2023) encapsulated ABTS on their surface and coated it with a layer of HA to give them a typical quasi spherical shape (Figures 5A, B). In 2024, Liu et al. (2024) used macrophage membranes to encapsulate Fe-S/N-C SAzymes in order to endow them with targeting ability. Vascular cell adhesion protein 1(VCAM-1) expressed on the surface of cancer cells, can bind to α4 and β1 integrins overexpressed on the surface of macrophages, thereby achieving effective tumor targeting. Long et al. (2024) utilized the high dispersibility of CDs in water or buffer solutions to prepare an iron SAzyme with good water solubility and easy target sensing in water.
2.3 Preparation methods
2.3.1 Vapor deposition method
In the process of vapor deposition method, the reactants are usually heated at high temperatures, diluted with rare gas or N2 to dilute the vapor phase composition, and deposited in a single atom dispersed form on the substrate surface Figure 6. Xu et al. (2024) used silicon dioxide to protect the pyrolysis of ZIF-8 under nitrogen atmosphere at 1,000°C, obtaining a carbon carrier. After adsorbing copper ions onto carbon carriers, Xu placed them at the lower airflow outlet of the tube furnace, placed sodium sulfate powder at the upper airflow outlet of the tube furnace. After pyrolyzing, Cu-N/S-C SAzyme whose active center is Cu-N1S2 can be obtained (Figure 7). Qin et al. (2022) used atomic deposition technology (ALD), a modified chemical vapor deposition technique to mix 45 mL DMF, 135 mL IPA, and 4.5 mL C16H36O4Ti for a reaction. After two high-temperature treatments, TiO2 support was obtained. Introducing ultra-high purity N2 to a self-designed ALD reactor, they prepared xFeOx/TiO2 nanozyme. Using the ALD ultra-thin modification strategy, xFeOx/TiO2 nanozymes were used as carriers to load FeOx. As the number of TiO2 ALD cycles increased, highly dispersed iron-based SAzymes limited to porous TiO2 nanospheres were obtained. After calcination and reduction treatment, TiO2 can be transformed from an amorphous form to a crystalline form, resulting in iron-based SAzymes with twice the activity.
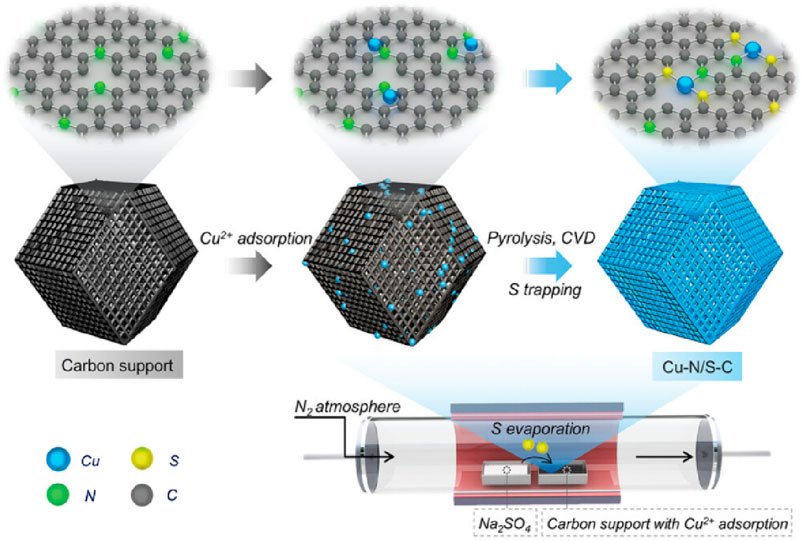
Figure 7. (Xu et al., 2024) The preparation of Cu-N/S-C.
2.3.2 One-pot solvothermal method
According to the low solubility of salts in organic solvents, one-pot solvothermal method can be used to reduce the collision probability between particles, resulting in highly dispersed SAzymes. Chen et al. (2023b) utilizes the condensation reaction between formamide molecules during the solution heating process (condensation of primary amines and active carbonyl compounds makes it easy to synthesize Schiff bases), coordination of Schiff bases and Fe ions, and simultaneous occurrence of carbonization to design a Schiff bases-derived Fe-NC-x SAzymes. They adopted one-pot solvothermal method, using different concentrations of iron ions and formamide as raw materials. After high-temperature treatment, they were centrifuged, washed, and dried to obtain Fe-NC-x SAzymes (x reflects the relative content of iron ions and formamide). This synthesis strategy has a certain universality, which can be extended to prepare SAzymes with different metal atomic centers, such as Cu Mn, Co, Zn, and Ni. Bo Wang et al. (2022b) synthesized manganese nitrogen co-doped carbon materials (f-MnNC) containing monodisperse Mn-N4 sites using MnCl2 and formamide as precursors through one-pot solvothermal method at 180°C for 12 h. This manganese single-atom nanozyme has efficient peroxidase-like activity. Lin et al. (2020) add 0.2 g of citric acid and a certain amount of sodium chlorophyllin copper salt (CuCh) to 4.0 mL of deionized water, and add 1 mL of 0.6 mg/mL polyoxyethanolamine to it. After mixing evenly, add 4.0 mL of absolute ethanol. After heating the mixed solution at 200°C for 10 h, cooling, filtering, and dialysis, Cu-N-C SAzymes can be obtained. Zhu et al. (2023) prepared TiO2 nanotubes in a 1.0 wt% hydrofluoric acid aqueous solution under a working voltage of 20 V for 30 min. After removing the remaining electrolyte in deionized water, it annealed at 450°C for 2 h and labeled with TNT. Then, Fe-NC nanozymes were prepared on the surfaces of Ti and TNT, using 100 mL of formamide solution containing 10 mM FeCl3 as the solvent.
2.3.3 Pyrolysis method
The pyrolysis method mainly includes two key steps. Fixing metal ions in organic frameworks and evaporating some atoms at high temperatures to anchor isolated metal centers within the substrates (Figure 8). Sun et al. (2022) prepared precursors, bimetallic Zn/Co metal organic frameworks (ZIF-67 doped with zinc) (Cao et al., 2020), using Zn(NO3)2·6H2O, Co(NO3)2·6H2O and 2-MI. Subsequently, it was pyrolyzed in an argon atmosphere and cooled to obtain Co-N-C SAzymes, a material with Co single atoms uniformly dispersed on nitrogen doped porous carbon. In 2022, Bolong Xu et al. (2022) employed a two-step pyrolysis method mediated by melamine, utilizing zeolite imidazole framework-8 (ZIF-8) as a precursor and coating its surface with SiO2. Initially, pyrolysis was conducted at a temperature of 1,000°C to obtain a monodisperse single carbon carrier, followed by the deposition of Fe3+ onto its surface. Subsequently, it was mixed with melamine, a nitrogen-rich precursor. Ultimately, an iron-based SAzyme with a five-coordinated (FeN5) structure was prepared via pyrolysis at 900°C.
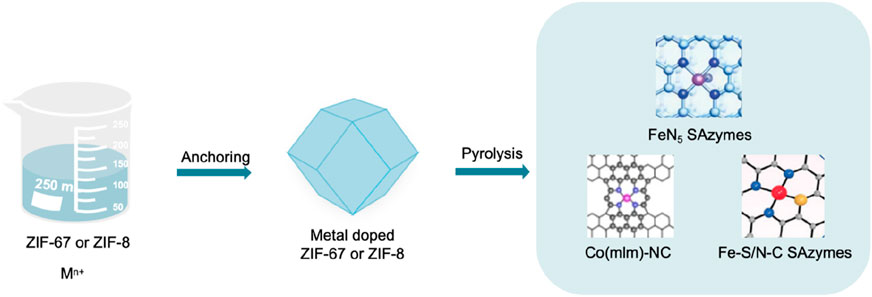
Figure 8. Key steps of the pyrolysis method and the structure of SAzymes prepared by pyrolysis (Xie et al., 2020; Xu et al., 2022; Liu et al., 2024).
In 2023, Yang et al. (2023) partially carbonized the precursor Fe (III)–tannic acid (TA), stabilizing and dispersing individual Fe(II) by the phenolic hydroxyl groups of Fe (III)–TA and using the dehydration network as a three-dimensional carbon framework to form monodisperse quasi spherical nanoparticles (Fe-CDs/ABTS@HA). In 2024, Wang et al. (2022a) uniformly dispersed Fe doped zeolite imidazolium salt framework-8 (Hemin/ZIF-8) in methanol solution, and then underwent pyrolysis at 920°C when it was partially carbonized and evolved into an asymmetric Fe-N3S1 portion (represented as Fe-S/N-C). The single atom catalyst with this Fe-N3S1 active center is Fe-S/N-C SAzymes. Xie et al. (2020) first added Co(acac)3(acac, acetylpropionat), Zn(NO3)2·6H2O and 2-methylimidazole (mlm) in a methanol solution to encapsulate Co(acac)3 in ZIF-8 micropores. Then, it was subjected to a solvothermal treatment at 140°C for 4 h, which can be converted into Co(mlm)4 through ligand exchange. Pyrolysis can directly convert it into a SAzyme named Co(mlm)-NC, with CoNx sites atomically dispersed in porous carbon.
3 Applications of single-atom nanozymes in oncology
In oncology, SAzymes are primarily used to mimic natural enzymes for detecting the concentration of biomolecules, thereby detecting the presence of tumor cells Figure 9. They also simulate oxidoreductases to promote the transformation of toxic substances in the body, thus achieving cancer treatment purposes.
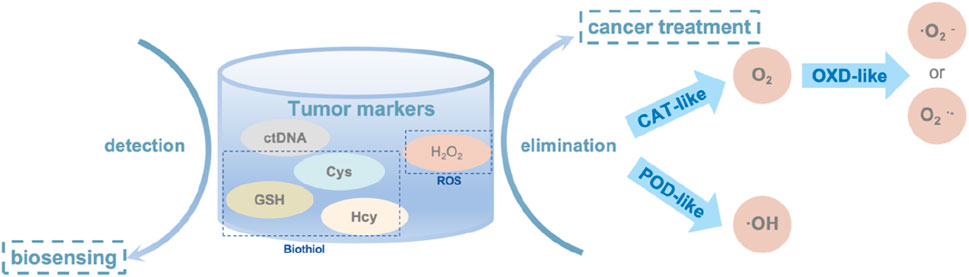
Figure 9. The main tumor markers and their applications in oncology, the elimination mechanism of H2O2 in oncology.
3.1 Biosensing
Biosensor technology based on SAzymes has found extensive applications in disease diagnosis due to its high active site density and atomic utilization efficiency, which results in faster reaction rates, higher sensitivity, and accuracy (Chen et al., 2023c). Currently, SAzymes are primarily used in cancer screening (Liang et al., 2023). Canceration cells typically produces various cancer markers, and employing SAzymes as biosensors enables more efficient and rapid detection of these markers, allowing for earlier intervention and treatment of cancer patients. The main cancer markers currently detected include biothiols and circulating tumor DNA (ctDNA) (Wang et al., 2024).
3.1.1 Detection of biothiol
Biothiols primarily refer to GSH and cysteine (Cys). Biothiols are abnormally expressed in cancer cells, resulting in higher concentrations in cancer cells compared to normal cells. Therefore, they can be used as tumor markers for cancer screening in clinical practice. Sun et al. (2022) have developed a Co-N-C SAzyme based on the acid-base pairing theory for detecting biothiol levels. This SAzyme exhibits strong coordination between the Co atoms and the S atoms of biothiols, enabling specific binding. Using a spectrophotometer, a working curve can be plotted to determine the concentration of solutions containing cancer markers (biothiols). Sun conducted five repeated measurements and found no significant differences in the absorption rates of GSH/Cys, indicating that the Co-N-C SAzyme has good reproducibility as a biothiol sensor. When other amino acids, with concentrations ten times lower than those of GSH or Cys, were introduced, the detected absorbance was significantly lower than that of GSH or Cys, demonstrating the specificity of the Co-N-C SAzyme. The detection limits for GSH and Cys were 0.07 µM and 0.06 µM, respectively, indicating the high sensitivity of the SAzyme. Therefore, SAzyme have promising applications in the field of biosensing.
3.1.2 Detection of ctDNA
When somatic cells in a healthy human body undergo apoptosis or necrosis, DNA can be released and enter the bloodstream. This type of DNA is called cell free DNA (cfDNA). For cancer patients, the DNA released during the canceration of their cells is called ctDNA (Sakaeda and Naito, 2021). Cancer screening can be conducted by collecting body fluids containing ctDNA (Rossi, 2023). CtDNA carries characteristic changes of tumor cells, such as mutations and methylation (Wen et al., 2022), and can therefore serve as a next-generation tumor marker.
However, ctDNA is relatively unstable in body fluids, with a half-life of approximately 16 min to 2.5 h (Chen et al., 2020). Therefore, finding an efficient method to detect ctDNA levels is particularly important. Long et al. (2024) utilized CDs to prepare a water-soluble iron SAzyme (SA Fe-CDs). The doping of CDs endowed SA Fe-CDs with abundant functional groups, enabling the binding of single strands and the output of large amounts of DNA products, thus improving the sensitivity and specificity of the detection. Using SA Fe-CDs as signal carriers, they developed an electrochemical biosensor that specifically attaches to electrodes. This stable sandwich structure can generate consistent electrochemical signals and further catalytically amplify the signal, resulting in a low detection limit of 1.26 aM. Long set up seven control groups, demonstrating that the biosensor has high reproducibility. The signal output was observed continuously for 28 days, and after 28 days, the signal remained at 89.97% of its original value, indicating high stability. The experiments introducing other types of DNA fragments proved that SA Fe-CDs have high selectivity.
3.2 Cancer treatment
Reactive oxygen species (ROS) are byproducts of mitochondrial respiration in organisms, including oxygen-containing radicals as well as peroxides easily to form radicals such as ⋅O2− and H2O2. ROS are often overexpressed in tumor cells, and the excessive production of ROS can significantly harm cellular components. This includes damaging cell membranes, nucleic acids, and proteins, leading to severe pathological processes such as cancer, diabetes, and sepsis. Overexpression of ROS can also promote the formation of tumor cells. Based on this understanding, a method for cancer therapy involves converting ROS to non-toxic products like O2 (Chen et al., 2023b) or toxic radicals (Xu et al., 2022) to target tumor cells. However, the application of natural enzymes in clinical treatment is limited due to their challenging extraction, high cost, low stability, and susceptibility to inactivation. Therefore, SAzymes, which are cost-effective and highly stable, can be used as substitutes.
3.2.1 Converting ROS to non-toxic O2
Chen et al. (2023b) discovered that Fe-NC-x SAzymes possess a rich π-conjugated structure, which endows the N-C matrix with high electron affinity. Consequently, the N-C matrix can interact with O2− through π-π stacking, converting it into non-toxic H2O2 and O2.
The optimal temperature for Fe-NC-x SAzymes is approximately 40°C, which aligns with the surface temperature of the human body, indicating that this SAzyme can achieve maximum activity when applied in cancer treatment within the human body. Chen conducted stability tests under harsh acidic and alkaline conditions, as well as storage stability tests, demonstrating that its stability is significantly higher than that of natural enzymes. Furthermore, Fe-NC-x SAzymes exhibit good blood compatibility, low cytotoxicity, and specificity in eliminating ROS. Fe-NC SAzymes can decompose H2O2 to protect cells from oxidative stress. After treatment, the viability of oxidatively stressed cells increased from 1.4% to 80.3%, showing the excellent cell-protective performance of Fe-NC-x SAzymes. The Fe2-SAzyme designed by Zhang et al. (2024) features dual single iron sites, which act as molecular tweezers, effectively capturing H2O2 through hydrogen-bond-induced end-bridge adsorption and decomposing it into O2 (Figure 10).
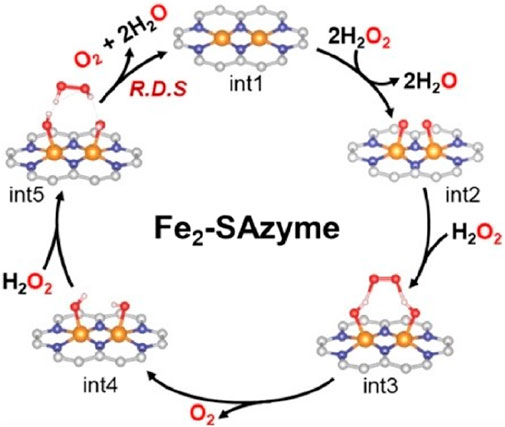
Figure 10. (Zhang et al., 2024) The process of Fe2-SAzyme adsorbing H2O2 and decomposing it into O2.
3.2.2 Converting ROS to toxic radicals
In recent years, efforts have been made to design catalysts that can specifically bind to ROS (such as H2O2) produced by the human body Table 1. These catalysts can convert adsorbed H2O2 into radicals, thereby selectively killing tumor cells named as chemodynamic therapy (CDT). In the acidic tumor microenvironment (TME), H2O2 is often overexpressed. Efficiently converting it into OH (Yang et al., 2023; Liu et al., 2024; Xu et al., 2024), O2− (Cai et al., 2022) or O2− (Wang et al., 2022a) that selectively kill tumor cells without causing off-target toxicity to normal tissues and organs holds promise for clinical anti-tumor therapy. It is known that transition metals such as Fe, Cu, and Co have suitable adsorption energies for H2O2. Therefore, current research focuses on designing various ligands to guide the charge redistribution of the central atom to facilitate electron transfer with H2O2 at the microscopic level.
Metal centers such as Fe and Cu exhibit advantages in demonstrating peroxidase (POD)-like enzyme activity. Researchers utilize various ligands to regulate their spatial structure, making them more efficient in adsorbing H2O2 and converting it into OH. In 2022, Xu et al. (2022) (Figure 11) designed an FeN5 SAzyme with a five-coordinate structure that can effectively adsorb H2O2. Biological tests indicated that it has a low hemolysis rate and minimal damage to the major organs of mice, suggesting good in vivo biocompatibility. Furthermore, it exhibited high tumor inhibition rates, with 78.0% inhibition via intravenous injection and 91.9% inhibition via intratumoral injection, indicating its potential as an efficient anti-tumor agent. In 2023, Yang et al. (2023) designed a non-heme iron SAzyme (Fe-CDs/ABTS@HA), featuring a distorted and non-planar Fe-O3N2 active site. This twisted five-coordination geometry allows the single iron site to become more saturated, keeping the adsorption energy within an optimal range. This enables better adsorption and catalytic conversion of ROS into OH and more efficient desorption of the produced H2O. Vivo and vitro studies have demonstrated that Fe-CDs/ABTS@HA can induce tumor necrosis in mice. Under laser irradiation, the cell apoptosis rate and tumor growth inhibition rate reached 96.6% and 58.4%, respectively. Hematoxylin and eosin (H&E) staining of tumor tissues (Figure 12A) revealed more pronounced necrosis and pyknosis with Fe-CDs/ABTS@HA under laser irradiation. Additionally, Fe-CDs/ABTS@HA exhibited low toxicity to other mouse tissues, indicating high tissue compatibility. In 2023, Liu et al. (2024) utilized sulfur atom doping to disrupt the symmetrical charge distribution around the Fe metal center, designing iron-based SAzymes (Fe-S/N-C SAzymes) with an asymmetric charge distribution. By coating the Fe-S/N-C SAzymes with macrophage membranes, they achieved M@Fe-S/N-C with a smoother surface. The Fe sites in this catalyst exhibit enhanced adsorption capacity and electron transfer ability, leading to improved catalytic activity. Consequently, it can more effectively adsorb H2O2 and convert it into OH. H&E staining of tumor tissues (Figure 12B) revealed significant nuclear shrinkage and fragmentation in the M@Fe-S/N-C group, indicating that M@Fe-S/N-C effectively disrupted the tumor. Treatment of mice with M@Fe-S/N-C did not affect their body weight, demonstrating high biocompatibility. Similarly, in 2024, Xu et al. (2024) employed sulfur engineering technology to design a copper-based SAzyme (Cu-N/S-C SAzymes), incorporating sulfur atoms into the catalyst. This incorporation resulted in the redistribution of electrons around the copper atoms, leading to an asymmetric distribution. The introduction of sulfur atoms enhanced the electron transfer process between the Cu-N1S2 active center and H2O2, facilitating the adsorption and activation of H2O2, thereby more effectively generating OH. Under near-infrared (NIR) light irradiation, Cu-N/S-C SAzymes achieved a tumor inhibition rate of 85.9%. H&E staining of tumor issues after phototherapy (Figure 12C) revealed a significant decrease in the proportion of purple-stained nuclei, indicating high tumor inhibition rates. Biochemical analysis of the blood from other tissues demonstrated high biosafety, confirming its potential as an efficient and low-toxicity tumor therapeutic agent.
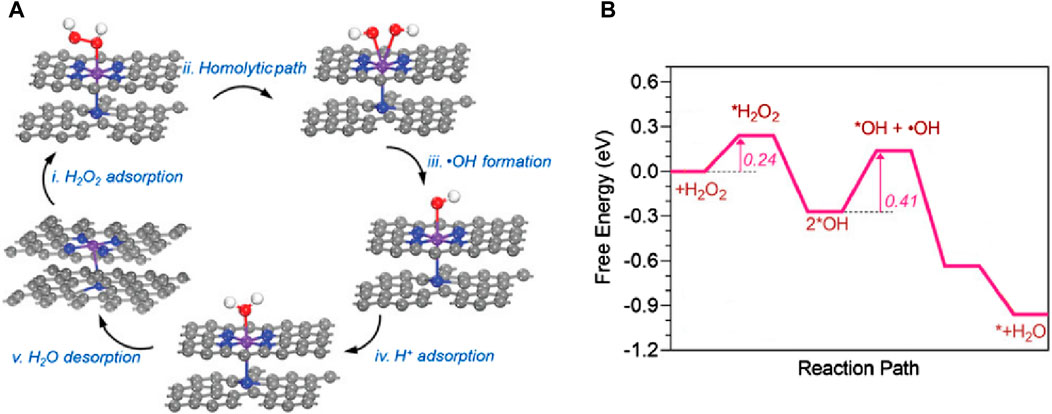
Figure 11. (Xu et al., 2022) (A) The catalytic mechanism of peroxidase-like reactions on FeN5 (B) The corresponding free energy of FeN5 peroxidase-like reactions.
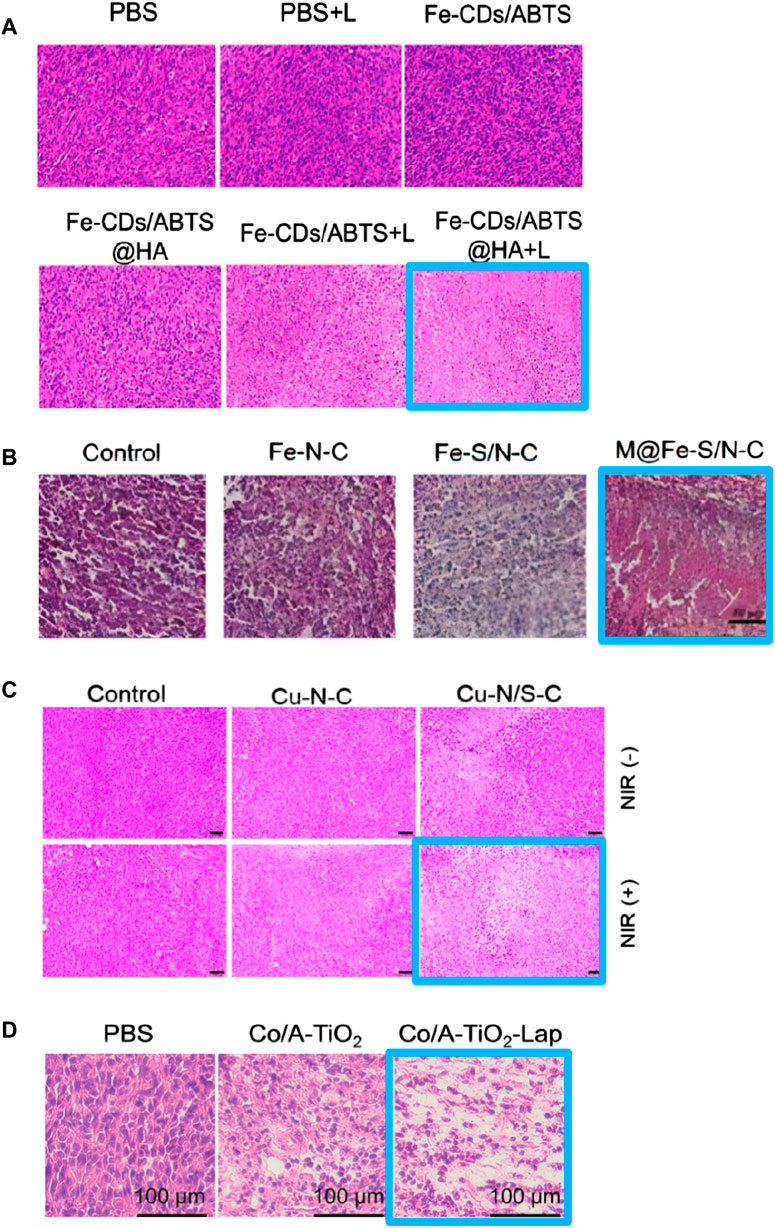
Figure 12. (A) (Yang et al., 2023) (B) (Liu et al., 2024) (C) (Xu et al., 2024) (D) (Wang et al., 2023a) H&E staining of tumor tissues after treatment with different single-atom nanozymes.
The Co metal center exhibits advantages in demonstrating oxidase (OXD)-like enzyme activity. Researchers utilize various ligands to regulate its spatial structure, enhancing its ability to adsorb H2O2 and convert it into O2− or O2−.
Based on the high catalytic efficiency exhibited by cobalt in various catalytic reactions and the efficient exposure of active sites on porous carbon with a large surface, Cai et al. (2022) constructed Co-SAs@NC SAzymes with cobalt highly dispersed on nitrogen-doped porous carbon. The Co sites can effectively adsorb H2O2 and convert it into O2, thereby alleviating hypoxia in cancerous tissue (Datta et al., 2021). Furthermore, these Co sites can continuously catalyze the conversion of O2 into highly cytotoxic O2−, effectively killing tumor cells. Vivo studies showed that the injection of Co-SAs@NC alone achieved a tumor inhibition efficiency of 66% while the tumor inhibition efficiency increased to 92% when combined with doxorubicin (DOX).
In 2022, Wang et al. (2022a) used TiO2 as a reducible support to prepare Co/A-TiO2 SAzymes with low Co2+/Co3+ ratios by modifying the local coordination environment of Co metal centers through strong electronic metal-support interactions between atomic metals and the surrounding support (Yang et al., 2020). Theoretical studies indicate that the lower the charge on atomic Co, the higher its activity in catalyzing the decomposition of H2O2 into O2. Consequently, Co/A-TiO2 SAzymes exhibit high tumor treatment efficacy. Co/A-TiO2 SAzymes can selectively convert H2O2 into O2, potentially alleviating hypoxia in tumor regions, and promote the generation of ROS. These ROS can further act as substrates in OXD-like enzymatic reactions, generating O2− which are more toxic to tumor cells than OH. The catalytic performance of Co/A-TiO2 SAzymes was also validated by ROS indicator staining of tumor cells. H&E staining of tumor tissues (Figure 12D) revealed that the combination of Co/A-TiO2 SAzymes with β-Lapachone (Lap), which accelerates ROS generation by promoting lactate accumulation, resulted in severe necrosis in tumor tissues, demonstrating the high tumor inhibition rate of this SAzyme.
3.2.3 Multi-enzymatic cascade reactions
In biological systems, biocatalytic cascade reactions occur within organelles, which are separated by cell membranes to prevent interference between reactions, thereby enhancing production efficiency. This strategy has garnered significant attention in the burgeoning field of systems chemistry. Unlike synergistic catalysis, where multiple catalysts operate simultaneously in the same step or reaction (Zhu et al., 2022), biocatalytic cascades involve a sequential, step-by-step catalytic process. Metal-organic frameworks (MOFs) are employed to create distinct spatial compartments that facilitate multi-enzyme cascade reactions. This methodology is particularly applied in tumor therapy to enhance therapeutic outcomes using SAzymes (Man et al., 2022). For instance, Cai et al. (2022) utilized N-doped porous carbon within MOFs to synthesize Co-SAs@NC. This composite acts as a catalase (CAT) mimic to convert hydrogen peroxide (H2O2) into oxygen (O2), and as an oxidase (OXD) mimic to transform O2 into highly cytotoxic superoxide (O2−) radicals.
4 Conclusion
The emergence of SAzymes has changed people’s long-term understanding of the application of inorganic materials in the biomedical field, making them aware that inorganic nanomaterials may also play an important role in this field. Traditional enzymes are essentially organic macromolecules such as proteins or RNA, which are often not heat-resistant and have a narrow range of pH values. Therefore, they have the characteristics of being difficult to extract, preserve, and easily inactivated in practical applications; The SAzyme, as an inorganic material, compensates for this drawback.
Compared to the complex and intricate three-dimensional spatial structure of natural enzymes, the active center of SAzymes is only constructed by a single atom, lacking strict geometric and electronic structure constraints, resulting in lower substrate specificity and selectivity. In order to improve the catalytic specificity of SAzymes and make them more widely used in the field of biocatalysis, the current main methods are to change the coordination situation of the central atom (Xie et al., 2020; Wang et al., 2023a), change the charge distribution state of the central atom (Chen et al., 2023b; Liu et al., 2024), design defects (Wang et al., 2023a), or regulate their morphology characteristics (Yang et al., 2023) to endow them higher substrate selection and adsorption ability, thereby improving their catalytic specificity.
SAzymes utilize single atoms as active centers, thus avoiding the problem of molecular-level biomolecule inactivation. These single-atom catalysts have high atomic dispersion and large specific surface area, which theoretically endows them with superior catalytic activity. Nevertheless, SAzymes still face some limitations, and we propose several promising solutions to address these challenges:
(1) At present, research on SAzymes is mostly focused on the field of biomedicine, especially in areas such as anti-tumor, antibacterial, and antioxidant properties. However, it remains to be debated whether SAzymes are harmful to the human body as they are prepared from materials such as metals (Wang et al., 2015). Recently, most studies have been conducted on its physiological stability and biosafety (but based on whether it causes harm to other tissues and organs). However, there is still relatively little research on whether it will bring side effects and whether it has long-term safety. Therefore, although SAzymes exhibit efficient catalytic performance and good stability, their current applicability to the human body remains to be studied and cannot be directly applied in clinical treatment.
(2) The existing SAzyme materials are mainly based on non-precious metal elements (such as Co, Cu, Fe, etc.), while the exploration of some precious metals and rare metal elements with excellent performance in the field of chemical catalysis is still relatively lagging behind. Therefore, expanding the types of SAzymes, especially the inclusion of precious metals and rare metal elements, is expected to endow SAzymes with more excellent catalytic activity and novel functions, thereby promoting the better application prospects of SAzymes in the field of oncology.
(3) There is a lack of a unified database to evaluate the different possible active intermediates and corresponding activation energies of SAzymes with different metal bases catalyzing a unified substrate. Therefore, it is difficult to select a certain kind of SAzyme having certain metal center with excellent catalytic performance, much less regulate the coordination environment. Therefore, a Materials Genome Initiative is urgently needed in the field of SAzymes.
(4) SAzymes have undergone the evolution of type selection (metal oxide type, metal organic type or polypeptide protein aggregate type) to the regulation of the surrounding environment of the central atom, and then to the current combination of other common cancer treatment methods (combined with photothermal therapy or other anti-tumor drugs). This synergistic effect in combination with other common cancer treatments contributes to the achievement of better tumor outcomes with SAzymes. However, the side effects of the beam on the human body (e.g., the burning effect on the human body) should be considered when applying photothermal therapy, and the drug interaction should be considered when using multiple antineoplastic drugs.
Author contributions
HL: Writing–original draft. YX: Writing–review and editing, Software, Investigation. XW: Writing–review and editing, Writing–original draft, Resources, Investigation.
Funding
The author(s) declare that no financial support was received for the research, authorship, and/or publication of this article.
Conflict of interest
The authors declare that the research was conducted in the absence of any commercial or financial relationships that could be construed as a potential conflict of interest.
Publisher’s note
All claims expressed in this article are solely those of the authors and do not necessarily represent those of their affiliated organizations, or those of the publisher, the editors and the reviewers. Any product that may be evaluated in this article, or claim that may be made by its manufacturer, is not guaranteed or endorsed by the publisher.
References
Anderson, P. W. (1959). New approach to the theory of superexchange interactions. Phys. Rev. 115 (1), 2–13. doi:10.1103/physrev.115.2
Bhalothia, D., Beniwal, A., Kumar Saravanan, P., Chen, P., and Chen, T. (2024). Bridging the gap between single atoms, atomic clusters and nanoparticles in electrocatalysis: hierarchical structured heterogeneous catalysts. ChemElectroChem 11, e202400034. doi:10.1002/celc.202400034
Cai, S., Liu, J., Ding, J., Fu, Z., Li, H., Xiong, Y., et al. (2022). Tumor-microenvironment-responsive cascade reactions by a cobalt-single-atom nanozyme for synergistic nanocatalytic chemotherapy. Angew. Chem. Int. Ed. Engl. 134 (48), e202204502. doi:10.1002/anie.202204502
Cai, S., Zhang, W., and Yang, R. (2023). Emerging single-atom nanozymes for catalytic biomedical uses. Nano Res. 16 (12), 13056–13076. doi:10.1007/s12274-023-5864-y
Camellone, M. F., and Fabris, S. (2009). Reaction mechanisms for the CO oxidation on Au/CeO2 catalysts: activity of substitutional Au3+/Au+ cations and deactivation of supported Au+ adatoms. J. Am. Chem. Soc. 131 (30), 10473–10483. doi:10.1021/ja902109k
Cao, F., Zhang, L., You, Y., Zheng, L., Ren, J., and Qu, X. (2020). An enzyme-mimicking single-atom catalyst as an efficient multiple reactive oxygen and nitrogen species scavenger for sepsis management. Angew. Chem. 132 (13), 5146–5153. doi:10.1002/ange.201912182
Chen, S., Lu, W., Xu, R., Tan, J., and Liu, X. (2023b). Pyrolysis-free and universal synthesis of metal-NC single-atom nanozymes with dual catalytic sites for cytoprotection. Carbon 201, 439–448. doi:10.1016/j.carbon.2022.09.034
Chen, X., Shu, W., Zhao, L., and Wan, J. (2023c). Advanced mass spectrometric and spectroscopic methods coupled with machine learning for in vitro diagnosis. View 4 (1), 20220038. doi:10.1002/viw.20220038
Chen, X., Wang, L., and Lou, J. (2020). Nanotechnology strategies for the analysis of circulating tumor DNA: a review. Med. Sci. Monit. 26, e921040. doi:10.12659/msm.921040
Chen, Y., Jiang, B., Hao, H., Li, H., Qiu, C., Liang, X., et al. (2023a). Atomic-level regulation of cobalt single-atom nanozymes: engineering high-efficiency catalase mimics. Angew. Chem. Int. Ed. 62 (19), e202301879. doi:10.1002/anie.202301879
Chen, Y., Wang, P., Hao, H., Hong, J., Li, H., Ji, S., et al. (2021). Thermal atomization of platinum nanoparticles into single atoms: an effective strategy for engineering high-performance nanozymes. J. Am. Chem. Soc. 143 (44), 18643–18651. doi:10.1021/jacs.1c08581
Datta, A., West, C., O'connor, J. P., Choudhury, A., and Hoskin, P. (2021). Impact of hypoxia on cervical cancer outcomes. Int. J. Gynecol. Cancer 31 (11), 1459–1470. doi:10.1136/ijgc-2021-002806
De Gennes, P.-G. (1960). Effects of double exchange in magnetic crystals. Phys. Rev. 118 (1), 141–154. doi:10.1103/physrev.118.141
Fu, Z., Yang, B., and Wu, R. (2020). Understanding the activity of single-atom catalysis from frontier orbitals. Phys. Rev. Lett. 125 (15), 156001. doi:10.1103/physrevlett.125.156001
Gao, L., Zhuang, J., Nie, L., Zhang, J., Zhang, Y., Gu, N., et al. (2007). Intrinsic peroxidase-like activity of ferromagnetic nanoparticles. Nat. Nanotechnol. 2, 577–583. doi:10.1038/nnano.2007.260
Guo, Z., Hong, J., Song, N., and Liang, M. (2024). Single-atom nanozymes: from precisely engineering to extensive applications. Accounts Mater. Res. 5 (3), 347–357. doi:10.1021/accountsmr.3c00250
Hua, S., Dong, X., Peng, Q., Zhang, K., Zhang, X., and Yang, J. (2024). Single-atom nanozymes shines diagnostics of gastrointestinal diseases. J. Nanobiotechnology 22 (1), 286. doi:10.1186/s12951-024-02569-3
Jiang, B., and Liang, M. (2021). Advances in single-atom nanozymes research. Chin. J. Chem. 39 (1), 174–180. doi:10.1002/cjoc.202000383
Jiang, J., Cui, X., Huang, Y., Yan, D., Wang, B., Yang, Z., et al. (2024). Advances and prospects in integrated nano-oncology. Nano Biomed. Eng. 16 (2), 152–187. doi:10.26599/nbe.2024.9290060
Larsen, A. H., Mortensen, J. J., Blomqvist, J., Castelli, I. E., Christensen, R., Dułak, M., et al. (2017). The atomic simulation environment—a Python library for working with atoms. J. Phys. Condens. Matter 29 (27), 273002. doi:10.1088/1361-648x/aa680e
Li, F., Li, S., Guo, X., Dong, Y., Yao, C., Liu, Y., et al. (2020). Chiral carbon dots mimicking topoisomerase I to mediate the topological rearrangement of supercoiled DNA enantioselectively. Angew. Chem. Int. Ed. Engl. 59 (27), 11087–11092. doi:10.1002/anie.202002904
Liang, D., Wang, Y., and Qian, K. (2023). Nanozymes: applications in clinical biomarker detection. Interdiscip. Med. 1 (4), e20230020. doi:10.1002/inmd.20230020
Lin, Z., Zheng, L., Yao, W., Liu, S., Bu, Y., Zeng, Q., et al. (2020). A facile route for constructing Cu–N–C peroxidase mimics. J. Mater. Chem. B 8 (37), 8599–8606. doi:10.1039/d0tb01494j
Liu, W., Chen, Q., Wu, J., Zhang, F., Han, L., Liu, J., et al. (2024). Asymmetric coordination of iron single-atom nanozymes with efficient self-cascade catalysis for ferroptosis therapy. Adv. Funct. Mater. 34 (14), 2312308. doi:10.1002/adfm.202312308
Long, Y., Zhao, J., Ma, W., Pei, W., Hou, J., et al. (2024). Fe single-atom carbon dots nanozyme collaborated with nucleic acid exonuclease III-driven DNA walker cascade amplification strategy for circulating tumor DNA detection. Anal. Chem. 96 (12), 4774–4782. doi:10.1021/acs.analchem.3c04202
Man, T., Xu, C., Liu, X.-Y., Li, D., Tsung, C. K., Pei, H., et al. (2022). Hierarchically encapsulating enzymes with multi-shelled metal-organic frameworks for tandem biocatalytic reactions. Nat. Commun. 13 (1), 305. doi:10.1038/s41467-022-27983-9
Nanozymes, Y. X. (2018). A new generation of artificial mimic enzymes. Adv. Biochem. Biophysics 45 (02), 101–104. doi:10.16476/j.pibb.2018.0041
Ong, S. P., Richards, W. D., Jain, A., Hautier, G., Kocher, M., Cholia, S., et al. (2013). Python Materials Genomics (pymatgen): a robust, open-source python library for materials analysis. Comput. Mater. Sci. 68, 314–319. doi:10.1016/j.commatsci.2012.10.028
Pei, J., Zhao, R., Mu, X., Wang, J., Liu, C., and Zhang, X. D. (2020). Single-atom nanozymes for biological applications. Biomaterials Sci. 8 (23), 6428–6441. doi:10.1039/d0bm01447h
Peng, C., Pang, R., Li, J., and Wang, E. (2024). Current advances on the single-atom nanozyme and its bioapplications. Adv. Mater. 36 (10), 2211724. doi:10.1002/adma.202211724
Qin, F., Zhang, J., Zhou, Z., Xu, H., Cui, L., Lv, Z., et al. (2022). TiO2 nanoflowers decorated with FeOx nanocluster and single atoms by atomic layer deposition for peroxidase-mimicking nanozymes. ACS Appl. Nano Mater. 5 (9), 13090–13099. doi:10.1021/acsanm.2c02875
Rossi, D. (2023). Circulating Tumor DNA (Liquid biopsy). Hematol. Oncol. 41 (S2), 37. doi:10.1002/hon.3163_8
Sakaeda, S., and Naito, Y. (2021). Circulating tumor DNA in oncology. Processes 9 (12), 2198. doi:10.3390/pr9122198
Shen, J., Chen, J., Qian, Y., Wang, X., Wang, D., Pan, H., et al. (2024). Atomic engineering of single-atom nanozymes for biomedical applications. Adv. Mater. 36, 2313406. doi:10.1002/adma.202313406
Sun, L., Yan, Y., Chen, S., Zhou, Z., Tao, W., Li, C., et al. (2022). Co–N–C single-atom nanozymes with oxidase-like activity for highly sensitive detection of biothiols. Anal. Bioanal. Chem. 414, 1857–1865. doi:10.1007/s00216-021-03816-4
Van Vleck, J. H. (1953). Models of exchange coupling in ferromagnetic media. Rev. Mod. Phys. 25 (1), 220–227. doi:10.1103/revmodphys.25.220
Wan, K., Chu, T., Li, B., Ming, P., and Zhang, C. (2023). Rational design of atomically dispersed metal site electrocatalysts for oxygen reduction reaction. Adv. Sci. 10 (11), 2203391. doi:10.1002/advs.202203391
Wang, B., Zhang, X., Kang, G., et al. (2022b). Colorimetric detection of alkaline phosphatase activity by manganese single-atom nanoenzymes. Anal. Chem. 50 (01), 54–63.
Wang, D., Wang, J., Gao, X. J., Ding, H., Yang, M., He, Z., et al. (2023a). Employing noble metal–porphyrins to engineer robust and highly active single-atom nanozymes for targeted catalytic therapy in nasopharyngeal carcinoma. Adv. Mater. 36 (7), e2310033. doi:10.1002/adma.202310033
Wang, F., and Zhou, Z. (2021). Advances in the synthesis, characterization and biological application of metallo-organic framework-derived single-atom nanozymes. J. Shanghai Normal Univ. Nat. Sci. Ed. 50 (06), 745–753.
Wang, H., Wang, Y., Lu, L., Ma, Q., Feng, R., Xu, S., et al. (2022a). Reducing valence states of Co active sites in a single-atom nanozyme for boosted tumor therapy. Adv. Funct. Mater. 32 (28), 2200331. doi:10.1002/adfm.202200331
Wang, R., Yang, S., Wang, M., Zhou, Y., Li, X., Chen, W., et al. (2024). A sustainable approach to universal metabolic cancer diagnosis. Nat. Sustain. 7, 602–615. doi:10.1038/s41893-024-01323-9
Wang, Y., Cho, A., Jia, G., Cui, X., Shin, J., Nam, I., et al. (2023b). Tuning local coordination environments of manganese single-atom nanozymes with multi-enzyme properties for selective colorimetric biosensing. Angew. Chem. 135 (15), e202300119. doi:10.1002/anie.202300119
Wang, Z., Xia, T., and Liu, S. (2015). Mechanisms of nanosilver-induced toxicological effects: more attention should be paid to its sublethal effects. Nanoscale 7 (17), 7470–7481. doi:10.1039/c5nr01133g
Wen, X., Pu, H., Liu, Q., Guo, Z., and Luo, D. (2022). Circulating tumor DNA—a novel biomarker of tumor progression and its favorable detection techniques. Cancers 14 (24), 6025. doi:10.3390/cancers14246025
Xiao, X., Hu, X., Liu, Q., Zhang, Y., Zhang, G. J., and Chen, S. (2023). Single-atom nanozymes as promising catalysts for biosensing and biomedical applications. Inorg. Chem. Front. 10 (15), 4289–4312. doi:10.1039/d3qi00430a
Xie, S., Liu, L., Lu, Y., Wang, C., Cao, S., Diao, W., et al. (2022). Pt atomic single-layer catalyst embedded in defect-enriched ceria for efficient CO oxidation. J. Am. Chem. Soc. 144 (46), 21255–21266. doi:10.1021/jacs.2c08902
Xie, X., He, C., Li, B., He, Y., Cullen, D. A., Wegener, E. C., et al. (2020). Performance enhancement and degradation mechanism identification of a single-atom Co–N–C catalyst for proton exchange membrane fuel cells. Nat. Catal. 3 (12), 1044–1054. doi:10.1038/s41929-020-00546-1
Xie, X., Zhang, Y., Li, D., Fan, Y., Huang, B., and Yang, X. (2023). Recent advances in common transition metal-based single-atom nanozymes and their applications in pollutant detection and degradation. Part. and Part. Syst. Charact. 40 (10), 2300039. doi:10.1002/ppsc.202300039
Xu, B., Li, S., Han, A., Zhou, Y., Sun, M., Yang, H., et al. (2024). Engineering atomically dispersed Cu–N1S2 sites via chemical vapor deposition to boost enzyme-like activity for efficient tumor therapy. Adv. Mater. 36 (13), 2312024. doi:10.1002/adma.202312024
Xu, B., Li, S., Zheng, L., Liu, Y., Han, A., Zhang, J., et al. (2022). A bioinspired five-coordinated single-atom iron nanozyme for tumor catalytic therapy. Adv. Mater. 34 (15), 2107088. doi:10.1002/adma.202107088
Xu, J., Zhang, S., Liu, H., Yuan, Y., Meng, Y., et al. (2023). Breaking local charge symmetry of iron single atoms for efficient electrocatalytic nitrate reduction to ammonia. Angew. Chem. Int. Ed. 62 (39), e202308044. doi:10.1002/anie.202308044
Xu, Y., Yin, H., and Xiao, F. (2021). Burgeoning single atoms as new types of nanozymes and electrocatalysts for sensing, biomedicine and energy conversion. J. Phys. Mater. 4 (4), 044013. doi:10.1088/2515-7639/ac1ab7
Yang, J., Li, W., Wang, D., and Li, Y. (2020). Electronic metal–support interaction of single-atom catalysts and applications in electrocatalysis. Adv. Mater. 32 (49), 2003300. doi:10.1002/adma.202003300
Yang, Q., Liu, J., Cai, W., Liang, X., Zhuang, Z., Liao, T., et al. (2023). Non-heme iron single-atom nanozymes as peroxidase mimics for tumor catalytic therapy. Nano Lett. 23 (18), 8585–8592. doi:10.1021/acs.nanolett.3c02406
Zhang, H., Wang, P., Zhang, J., Sun, Q., He, Q., He, X., et al. (2024). Boosting the catalase-like activity of SAzymes via facile tuning of the distances between neighboring atoms in single-iron sites. Angew. Chem. 136 (9), e202316779. doi:10.1002/anie.202316779
Zhang, L., Dong, Q., Hao, Y., Wang, Z., Dong, W., Liu, Y., et al. (2023). Drug-primed self-assembly of platinum-single-atom nanozyme to regulate cellular redox homeostasis against cancer. Adv. Sci. 10 (30), 2302703. doi:10.1002/advs.202302703
Zhang, S., Li, Y., Sun, S., Liu, L., Mu, X., Liu, S., et al. (2022). Single-atom nanozymes catalytically surpassing naturally occurring enzymes as sustained stitching for brain trauma. Nat. Commun. 13 (1), 4744. doi:10.1038/s41467-022-32411-z
Zhou, X., Shen, Q., Yuan, K., Yang, W., Chen, Q., Geng, Z., et al. (2018). Unraveling charge state of supported Au single-atoms during CO oxidation. J. Am. Chem. Soc. 140 (2), 554–557. doi:10.1021/jacs.7b10394
Zhu, D., Chen, H., Huang, C., Wang, X., Jiang, W., et al. (2022). H2O2 self-producing single-atom nanozyme hydrogels as light-controlled oxidative stress amplifier for enhanced synergistic therapy by transforming “cold” tumors. Adv. Funct. Mater. 32 (16), 2110268. doi:10.1002/adfm.202110268
Zhu, H., Zhang, H., Chen, S., Guan, S., Lu, W., et al. (2023b). Fe-NC nanozymes-loaded TiO2 nanotube arrays endow titanium implants with excellent antioxidant capacity for inflammation inhibition and soft tissue integration. Compos. Part B Eng. 267, 111054. doi:10.1016/j.compositesb.2023.111054
Zhu, Y., Liao, Y., Zou, J., Cheng, J., Pan, Y., Lin, L., et al. (2023a). Engineering single-atom nanozymes for catalytic biomedical applications. Small 19 (30), 2300750. doi:10.1002/smll.202300750
Keywords: single-atom nanozymes, nanomaterials, biomedical, cancer treatment, first-principle
Citation: Liang H, Xian Y and Wang X (2024) Preparation and application of single-atom nanozymes in oncology: a review. Front. Chem. 12:1442689. doi: 10.3389/fchem.2024.1442689
Received: 02 June 2024; Accepted: 01 August 2024;
Published: 12 August 2024.
Edited by:
Weiwei Zhang, Beijing Technology and Business University, ChinaReviewed by:
Kun Qian, Shanghai Jiao Tong University, ChinaCharles Oluwaseun Adetunji, Edo University, Nigeria
Kelong Fan, Chinese Academy of Sciences (CAS), China
Copyright © 2024 Liang, Xian and Wang. This is an open-access article distributed under the terms of the Creative Commons Attribution License (CC BY). The use, distribution or reproduction in other forums is permitted, provided the original author(s) and the copyright owner(s) are credited and that the original publication in this journal is cited, in accordance with accepted academic practice. No use, distribution or reproduction is permitted which does not comply with these terms.
*Correspondence: Huiyuan Liang, 1354369036@qq.com