- 1SINOPEC (Dalian) Research Institute of Petroleum and Petrochemicals, Dalian, China
- 2Dalian University of Technology, Chemical Engineering Institute, Dalian, China
The M/SSZ-39 catalysts (M = In, Co, Cu, Fe) with different metal species and metal loadings were synthesized using the wet impregnation method on a small-pore SSZ-39 molecular sieve. X-ray diffraction (XRD), transmission electron microscopy (TEM), nitrogen adsorption-dehydrogenation and hydrogen temperature program reduction (H2-TPR) were employed to characterize the effects of various metal components and metal loadings on the performance of CH4 selective catalytic reduction of NO reaction (CH4-SCR). The characterization results showed that the In/SSZ-39 catalyst exhibited significantly higher catalytic activity compared to the Cu-, Co-, and Fe/SSZ-39 catalysts, suggesting that indium (In) is a more suitable active ingredient for the CH4-SCR reaction. The xIn/SSZ-39 (x = 1, 2, 3, x represents the In loadings of 1.0 wt%, 2.0 wt% and 3.0 wt%) catalysts, with different In loadings, all present excellent CH4-SCR performance. By varying the In loadings, the type of In species present in the catalyst can be regulated, thus enhancing DeNOx activity and CH4 selectivity in the CH4-SCR reaction. At a low temperature of 400 °C and a low CH4/NO feed ratio (CH4/NO = 1), the 3In/SSZ-39 catalyst, featuring highly active InOx clusters, achieves the best low-temperature CH4-SCR performance, with a high NO conversion rate of up to 90% and a CH4 selectivity of up to 74.2%.
1 Introduction
Nitrogen oxides (NOx) are major pollutants that not only directly jeopardize human health but also trigger a series of environmental issues such as acid rain, photochemical smog, ozone hole, etc (Wang et al., 2017). Thus, addressing the pressing requirements for NOx emission reduction (DeNOx) is crucial for the sustainable development of human society. One of the primary sources of NOx emissions is industrial flue gas from thermal power plants, cement plants, and petrochemical facilities. The main method for reducing NO emissions is ammonia selective catalytic reduction (NH3-SCR), which uses V2O5-WO3 (MoO3)/TiO2 as the catalyst (Han et al., 2019; Wang et al., 2021). However, vanadium catalysts are biological toxicity, and using NH3 as a reducing agent can lead to pipeline corrosion, obstructions, ammonia leaks and other secondary pollution issues (Martin et al., 2006; Mrad et al., 2015). Selective catalytic reduction of NO with methane (CH4-SCR) is a promising new DeNOx technique. This method uses CH4 as a reductant instead of NH3, enabling the synergistic purification of NOx and greenhouse gas CH4, thus avoiding many issues associated with NH3 (Campa et al., 2021; Wen et al., 2021).
As the primary component of natural gas, CH4 is abundant and readily available. However, CH4 is the most stable hydrocarbon molecule, which makes it less effective for selective reduction of NOx at low temperatures and more prone to undergo a non-selective catalytic combustion at high temperatures. Therefore, the CH4-SCR technology is still meeting many challenges. Among them, developing effective CH4-SCR catalysts is critically important. Currently, the two most developed catalytic systems are metal oxides and molecular sieves. Compared to metal oxide catalysts, molecular sieve catalysts have gained more attention due to their rich pore structure, adjustable acidity, and metal-ion exchange ability (Jin et al., 2009).
Catalysts containing Pd, Ag, Ni, In and Co supported by molecular sieve have been investigated in CH4-SCR reaction (Lee et al., 2003; Shi et al., 2004; Chupin et al., 2006; Kubacka et al., 2006; Campa et al., 2015; Sazama et al., 2016). Noble metal-based molecular sieve catalysts can activate CH4 at lower temperatures and display a broad reaction temperature window (350°C–500° C), but they are expensive and the CH4 selectivity decreases rapidly as the temperature rises. Thus, high CH4/NO feed ratios are generally required to achieve high NO conversion in CH4-SCR reaction catalyzed by the noble metal-based molecular sieve catalysts (Chen et al., 2011; Costilla et al., 2011). Non-precious metal-based molecular sieve catalysts typically require higher reaction temperatures (>500 °C) for good CH4-SCR performance. However, they also face issues of CH4 susceptibility to non-selective catalytic combustion at high temperatures (Chen et al., 2011; Huang et al., 2018). Therefore, the key challenge is to design metal-based molecular sieve catalysts that can moderately activate CH4 at low temperatures, promoting CH4-SCR reactions and achieving high NOx conversion rates.
To develop effective CH4-SCR catalysts with good low-temperature activity and high CH4 selectivity. In this study, a series of M/SSZ-39 catalysts with different metal loadings (M = In, Co, Cu, Fe) were prepared using a simple wet impregnation method on a SSZ-39 molecular sieves. The physicochemical properties of the catalysts were systematically investigated, and their CH4-SCR performance was evaluated to assess the impact of various metal and metal loadings. The catalytic evaluation results showed that In/SSZ-39 catalysts performed significantly better performance than Co-, Cu-, and Fe/SSZ-39 catalysts, with enhanced low-temperature DeNOx activity as In loading increased. Characterization results demonstrated that In species were highly dispersed without nanoparticle formation on the xIn/SSZ-39 catalysts prepared with different loadings (x = 1, 2, 3, x represents the In loadings of 1.0 wt%, 2.0 wt% and 3.0 wt%).
2 Experimental section
2.1 Materials and instruments
SSZ-39 molecular sieve with a Si/Al ratio of 11 was purchased from Dalian Zhongcaptor Molecular Sieve Factory; Indium nitrate tetrahydrate (In(NO3)3 4H2O), analytically pure, was obtained from Aladdin Reagent; Analytically pure Cobalt nitrate hexahydrate (Co(NO3)2 6H2O), Copper nitrate trihydrate (Cu(NO3)2 3H2O) and Iron nitrate hydrate nine (Fe(NO3)3 9H2O) were purchased from Tianjin Damao Reagent Factory.
Powder X-ray diffraction (XRD) patterns were recorded on a X′Pert PRO-type diffractometer (Panacor) with a nickel-filtered Cu Kα X-ray source at a scanning rate of 5°/min over the range of 5°–50°. N2 adsorption-desorption isotherms were recorded at -196° C on a ASAP-2020-type physical adsorptive instrument. The sample (0.05–0.1 g) was degassed in a vacuum at 150 °C for 4 h prior to measurement. The Brunauer-Emmauer-Teller (BET) method was used to calculate the specific surface area of catalyst. The scanning electron microscopy (SEM) images were taken on a FEI-Verios 460L. Transmission electron microscopic (TEM) images were taken on a Tecnai G220 S-twin instrument (FEI Company) with an acceleration voltage of 200 kV. High-angle annular dark field-scanning transmission electron microscopy (HAADF-STEM) was carried out using a JEM-ARM200F instrument. Energy-dispersive X-ray spectroscopic (EDS) images were collected using a JED-2300T instrument. The H2 temperature-programmed reduction (H2-TPR) test was conducted on a AutoChem 2920-type chemisorptive instrument. The feeding gas of 10% H2 + 90% Ar was fed into the reactor with 60 mL/min, and the H2 intensity profiles were record from 50°C to 800° C at a heating rate of 10° C/min.
2.2 Preparation of catalysts
The In/SSZ-39 catalysts were prepared using the wet impregnation method with SSZ-39 molecular sieves as the carrier and In(NO3)3 4H2O as the indium source. Firstly, a suitable amount of In(NO3)3· 4H2O was fully dissolved in deionized water. Then the SSZ-39 molecular sieve was added, and the mixture was agitated for 4 h at room temperature until the mixture slowly evaporated at 80° C. The obtained viscous sample was put into a blast drying oven at 120° C for 12 h. Subsequently, the sample was calcined at 550° C for 4 h with an increase rate of 5° C/min in a muffle furnace. The obtained catalysts were labeled as xIn/SSZ-39, where x represents the theoretical In loadings of 1.0 wt%, 2.0 wt%, and 3.0 wt%, respectively.
The xCo/SSZ-39, xCu/SSZ-39 and xFe/SSZ-39 catalysts were prepared using the same method as that of the xIn/SSZ-39 catalysts, except that In(NO3)3 4H2O was replaced by Co(NO3)2 6H2O, Cu(NO3)2 3H2O, and Fe(NO3)3 9H2O, respectively, and x represents the theoretical metal loading 3.0 wt%.
2.3 Evaluation of the catalyst activities
Catalytic activity tests were conducted in a fixed-bed reactor at atmosphere pressure. A total of 0.75 mL catalyst with a particle size of 40–60 mesh was filled in a fixed-bed reactor with an inner diameter of 8 mm. The feed gas consisted of 0.1 vol% NO, 0.1 vol% CH4, 6 vol% O2 and balance N2, with a GHSV of 8,000 h−1. Before the measurement, the catalyst was pretreated at 400° C for 30 min under N2 atmosphere to remove any adsorbed impurity gases and moisture on the catalyst. During tests, the steady-state outlet compositions (NO, NO2, N2O and CH4 concentrations) at different reaction temperature (400, 450, 500°C and 550°C) were measured by an on-line infrared flue gas analyzer (MKS Instruments, Inc., 6030). The NO conversion rate, CH4 conversion rate and CH4 selectivity were calculated using Eqs 1–3, respectively, as shown below:
where
3 Results and discussion
3.1 Characterization results of catalysts
Figure 1 presents the XRD spectra of M/SSZ-39 catalysts. A series of distinctive diffraction peaks at 2θ = 9°–11° and 2θ = 15°–18° are present in all samples, which are attributed to the typical AEI topology of SSZ-39 molecular sieves (Li et al., 2019; Du et al., 2023). The XRD spectra of M/SSZ-39 catalysts with different metals and different metal loadings do not change significantly, indicating that the loading of metals through the wet impregnation procedure does not affect the AEI topology of SSZ-39 molecular sieve. Figure 1B shows a localized enlarged XRD spectrum of Figure 1A, where no diffraction peaks of metal oxides are observed in all catalysts. This indicates that metal species exist as highly dispersed metal oxide clusters or ionic metal sites on SSZ-39 molecular sieve at higher metal loading. This phenomenon is attributed to the large specific surface area and regular cage structure of the small-pore SSZ-39 molecular sieve, which provides a lot of space and specific surface for dispersing metal species (Ren et al., 2011; Lim et al., 2020; Xu et al., 2022).
The textual properties of xIn/SSZ-39 catalysts were characterized by N2 adsorption-desorption isotherms, as shown in Figure 2. All samples display type I adsorption isotherm features, typical adsorption isotherm characteristics of microporous materials (Kruk et al., 2001). This is consistent with the uniform microporous nature of small-pore SSZ-39 molecular sieve. Table 1 shows the calculated textual properties of xIn/SSZ-39. With increasing In loading, all catalystsʹ specific surface areas only slightly decreased. Notably, the 1In/SSZ-39 and 2In/SSZ-39 catalysts exhibit nearly identical microporous specific surface area, exterior specific surface area, and microporous pore volume. This demonstrates that the In species are able to maintain a high degree of dispersion and do not obstruct the microporous pores of SSZ-39 when the loading of In is controlled to be no more than 2 wt%. When the In loading is increased to 3 wt%, the microporous specific surface area of 3In/SSZ-39 catalyst begins to decrease slightly, while its external specific surface area became significantly larger. This may be attributed to the formation of highly dispersed InOx clusters on the external surface of SSZ-39, which increases the external specific surface area of 3In/SSZ-39 catalyst (no InOx nanoparticles were observed in the TEM images). The specific surface area of 3Co/SSZ-39, 3Cu/SSZ-39, and 3Fe/SSZ-39 catalysts are similar to that of In/SSZ-39 catalyst. It indicates that these metal species (Co, Cu and Fe) are also highly dispersed on SSZ-39.
The redox properties of catalyst are crucial for CH4-SCR activity, therefore, H2-TPR characterization was carried out to investigate the reducibility of metal oxide species in catalysts, as shown in Figure 3. In Figure 3A, it can be observed that the H2-TPR spectra of all In/SSZ-39 catalysts display broad reduction signals between 260° C and 480° C, which are attributed to the reduction of InOx species to In+ sites (Yoo et al., 2015; Zhao et al., 2023). Moreover, the primary reduction peaks of three catalysts are almost located in the same positions, centered at 260°C–480° C, without shifting to lower or higher temperature regions. This demonstrates that the forms of the major InOx species in catalysts are essentially the same. The H2 consumption gradually increased as In loading increased, corresponding to the increased area of the major reduction peaks in xIn/SSZ-39 catalysts. Remarkably, a notable reduction peak centered at a lower temperature of only ∼232 °C appeared in the H2-TPR profile of 3In/SSZ-39 catalyst, suggesting the better reducibility of highly dispersed InOx species. This phenomenon can be attributed to the formation of highly dispersed InOx clusters on the external surface of SSZ-39 resulting from the increased In loading, as corroborated by the N2 physical adsorption-desorption test. This fraction of the InOx species exhibits better reducibility as it is not constrained by the pores or cavities of SSZ-39, which is essential for the moderate activation of CH4 and contributes significantly to the enhancement of low-temperature CH4-SCR performance (Yoo et al., 2015; Zhao et al., 2023).
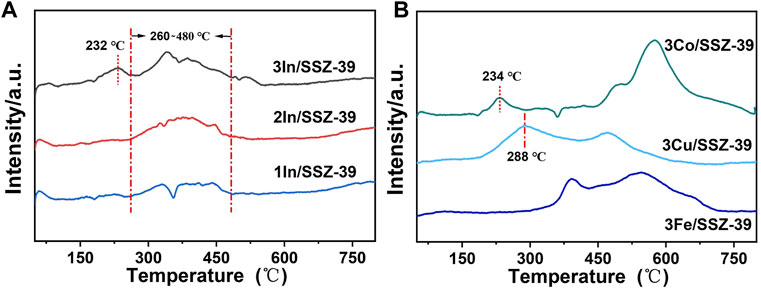
Figure 3. H2-TPR profiles of (A) xIn/SSZ-39 catalysts and (B) 3Co/SSZ-39, 3Cu/SSZ-39 and 3Fe/SSZ-39 catalysts.
In Figure 3B, the 3Co/SSZ-39, 3Cu/SSZ-39 and 3Fe/SSZ-39 catalysts display a significantly different H2-TPR spectrum compared to the 3In/SSZ-39 catalyst. A primary reduction peak is located at a lower temperature of 288° C in 3Cu/SSZ-39 catalyst which is much lower than that of 3Co/SSZ-39, 3Fe/SSZ-39 and 3In/SSZ-39 catalysts, suggesting the good reducibility of major CuOx species. This is conducive to activating CH4 at low-temperatures. However, the major CuOx species, with good reducibility, also facilitates the non-selective catalytic combustion reaction, may leading to very low N2 selectivity of 3Cu/SSZ-39 catalyst. In the H2-TPR spectrum of 3Fe/SSZ-39 catalyst, there are not any reduction peaks located at lower temperatures can be observed, and the major reduction peaks are almost located at higher temperatures between 375°C and 700° C. The results suggest that FeOx species are not conducive to the activation of CH4 at low temperatures. For 3Co/SSZ-39 catalysts, a small reduction peak centered at 234 °C appeared in the H2-TPR profile similar to that of 3In/SSZ-39. But, the major reduction peak located at higher temperatures between 450°C and 700° C which are much higher than that (260°C–480° C) of 3In/SSZ-39 catalysts. In the H2-TPR spectrum of 3In/SSZ-39 catalyst, a small reduction peak located at lower temperature of 232° C and a major reduction peak located at medium temperature of 342° C are observed, indicating the moderate reducibility of highly dispersed InOx species. Compared to the 3Co/SSZ-39, 3Cu/SSZ-39 and 3Fe/SSZ-39 catalysts, the InOx species in 3In/SSZ-39 catalyst, with moderate reducibility, may promote the moderate activation of CH4 and enhance the CH4-SCR activity at low temperatures.
Figures 4A,B depict the SEM images of 3In/SSZ-39 catalyst, revealing typical zeolite particles with a regular and smooth hexahedral structure, and no nanoparticles are observed on the surface of the smooth hexahedral structure. Further, TEM and EDS characterizations were conducted to examine the dispersion form of InOx species on SSZ-39 molecular sieve, as shown in Figure 4C, 4days and Figure 5. The basic hexahedral structure of SSZ-39 remains intact, consistent with the SEM results. Figure 4D presents the high-resolution TEM image of 3In/SSZ-39 catalyst, where no distinct InOx nanoparticles were observed, aligning with the XRD results. The combination of N2 physical adsorption-desorption tests and H2-TPR characterizations strongly indicates that the InOx species are highly dispersed on the SSZ-39 molecular sieve, possibly existing in the form of InOx clusters or In+ sites, and do not aggregate to form nanoparticles. This hypothesis is further supported by the EDS characterization, which shows that no InOx nanoparticles are generated since the In elements are extremely uniformly dispersed in SSZ-39 molecular sieves (Figure 5).
3.2 CH4-SCR performance of catalysts
The CH4-SCR performance of M/SSZ-39 catalysts (40–60 mesh) was tested in a fixed-bed reactor at 400°C–550° C, using a feed gas composed of 0.1 vol% NO, 0.1 vol% CH4, 6 vol% O2 and the balance N2 with a GHSV of 8,000 h−1. Initially, the effects of different metal components on the performance of catalysts for CH4-SCR were investigated. Figure 6A displays the temperature-dependent NO conversion curves of 3In/SSZ-39, 3Co/SSZ-39, 3Cu/SSZ-39, and 3Fe/SSZ-39 catalysts. It is evident that the activities of catalysts follow the order: In > Co > Fe > Cu when the reaction temperature is below 530° C. Notably, the 3In/SSZ-39 catalyst exhibits significant performance compared to the 3Co, 3Cu, and 3Fe/SSZ-39 catalysts, underscoring the significance of In as an active component in catalyzing the selective catalytic reduction of NOx by CH4 (Zhao et al., 2023). As indicated by the H2-TPR results, compared to the 3Co/SSZ-39, 3Cu/SSZ-39 and 3Fe/SSZ-39 catalysts, the highly dispersed InOx species in 3In/SSZ-39 catalyst, with moderate reducibility, can promote the moderate activation of CH4 and enhance the SCR process at low temperatures. Therefore, the 3In/SSZ-39 catalyst exhibits excellent CH4-SCR activity and good CH4 selectivity, achieving a high NO conversion rate over 90% at lower temperatures (400°C–450° C) and a lower CH4/NO feed ratios (CH4/NO = 1/1).
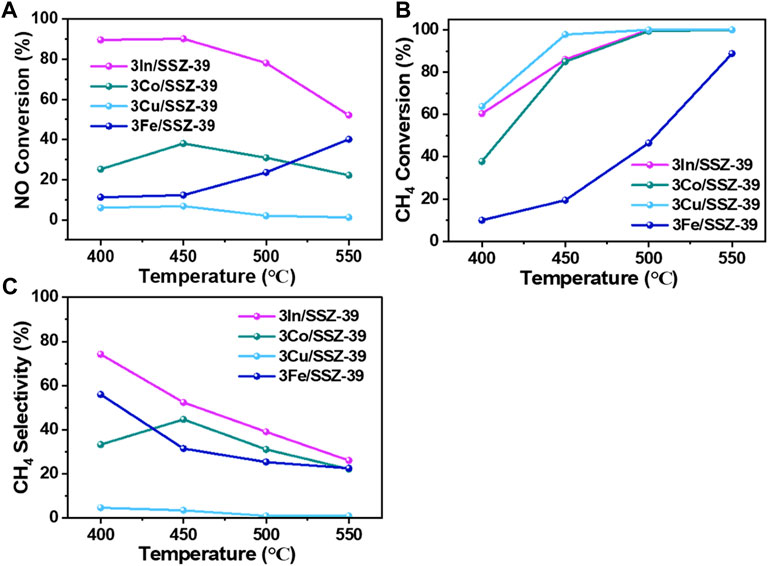
Figure 6. NO conversion rates (A), CH4 conversion rates (B) and CH4 selectivities (C) as a function of temperatures in CH4-SCR over 3In/SSZ-39, 3Co/SSZ-39, 3Cu/SSZ-39 and 3Fe/SSZ-39 catalysts (NO/CH4 = 1).
The NO conversion rate of 3In/SSZ-39 catalyst remains almost unchanged at 90% (Figure 6A) as the reaction temperature is increased from 400°C to 450 °C. However, as shown in Figure 6B, the CH4 conversion rate increased from 60% to 86%. This suggests that 26% of the converted CH4 undergoes non-selective catalytic combustion rather than a selective reduction of NOx, demonstrating that the 3In/SSZ-39 catalyst achieves the highest CH4 selectivity and NO conversion rate at 400 °C, as depicted in Figure 6C. In contrast, compared to NO conversion rates, the CH4 conversion rates of the 3Co/SSZ-39 and 3Cu/SSZ-39 catalysts are significantly higher, suggesting that the Co and Cu components are more prone to catalyzing the CH4 combustion reaction, leading to lower CH4 selectivity, as illustrated in Figure 6C. As for the 3Fe/SSZ-39 catalyst, both lower NO and CH4 conversion rates are achieved, indicating that the Fe component is less effective in catalyzing CH4 catalytic combustion and CH4-SCR reaction. In conclusion, the types of metal components can be tuned to control the competition between CH4 non-selective catalytic combustion and CH4-SCR reactions.
For the xIn/SSZ-39 catalysts with superior CH4-SCR performance, the impact of various In loadings on the catalytic performance was further investigated, as shown in Figure 7. It is evident that both the NO and CH4 conversion rates of catalysts increase with temperature when the in loading is less than 2 wt%, corresponding to their high CH4 selectivity, as illustrated in Figure 7C. Among these, the 2In/SSZ-39 catalyst exhibits a 60% NO conversion rate and a 34% CH4 conversion rate at 400° C, indicating that the ratio of converted NO to CH4 molecules is close to 2, which is the theoretical NO/CH4 ratio when a reaction proceeds in accordance with the stoichiometric ratio. This demonstrates that the 2In/SSZ-39 catalyst can achieve a very high CH4 selectivity (up to 88.5%) at 400° C, as shown in Figure 7C. For the 3In/SSZ-39 catalyst, it is NO conversion rate decreases rapidly as the temperature raised from 450°C to 550° C, indicating a rapid decrease in CH4 selectivity as well. This can be attributed to the presence of highly dispersed InOx clusters on the external surface of SSZ-39 (as indicated by H2-TPR results). These clusters exhibit the best reducibility (low-temperature reduction peak of only ∼232° C), which promote the moderate activation of CH4 and enhance the CH4-SCR activity at low temperatures. However, at higher temperatures, these highly active InOx clusters can lead to over-activation of CH4, which increases the likelihood of converted CH4 undergoing a non-selective catalytic combustion reaction and thus reducing the CH4 selectivity.
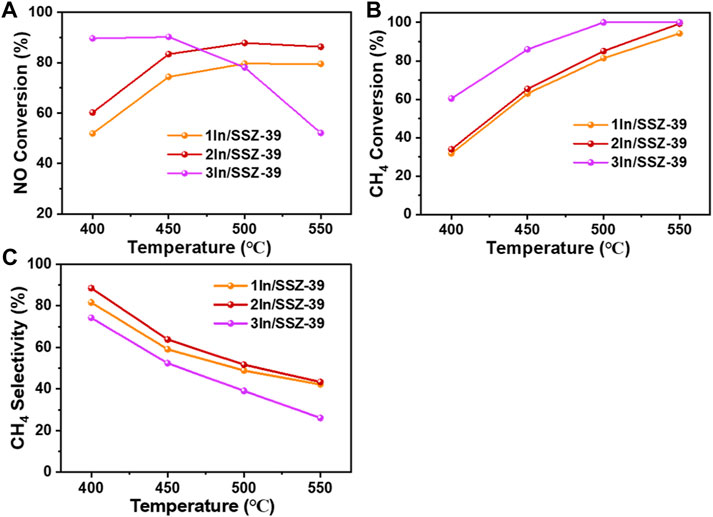
Figure 7. NO conversion rates (A) CH4 conversion rates (B) and CH4 selectivities (C) as a function of temperatures in CH4-SCR over 1In/SSZ-39, 2In/SSZ-39 and 3In/SSZ-39 catalysts (NO/CH4 = 1).
4 Conclusion
In this paper, a series of M/SSZ-39 catalysts (M = In, Co, Cu, Fe) were prepared using the wet impregnation method on a small-pore SSZ-39 molecular sieve. The effects of different metal loadings and metal loadings on the performance of CH4-SCR were investigated. The characterization results revealed that the performance of In/SSZ-39 catalysts are significantly higher than that of the Cu-, Co-, and Fe/SSZ-39 catalysts, indicating that indium (In) is a more suitable active ingredient for the CH4-SCR reaction. The low-temperature CH4-SCR activity of catalysts is notably enhanced with increasing In loadings. Among them, the 3In/SSZ-39 catalyst exhibits a high NO conversion rate of up to 90% and a CH4 selectivity of up to 74.2% at a reaction temperature of 400 °C and a low CH4/NO feed ratio (CH4/NO = 1). TEM and EDS characterization results showed that the In species were highly dispersed on the SSZ-39 molecular sieves, greatly promoting the moderate activation of CH4 and enhancing the CH4-SCR activity at low temperatures. These findings demonstrate a potentially generalizable approach toward the design and synthesis of high-efficiency CH4-SCR catalyst.
Data availability statement
The original contributions presented in the study are included in the article/Supplementary Material, further inquiries can be directed to the corresponding authors.
Author contributions
SA: Data curation, Investigation, Writing–original draft, Writing–review and editing. PW: Formal Analysis, Writing–review and editing. KW: Formal Analysis, Writing–review and editing. XW: Writing–review and editing. BL: Writing–review and editing. XG: Funding acquisition, Project administration, Writing–review and editing.
Funding
The author(s) declare that financial support was received for the research, authorship, and/or publication of this article. This study was financially supported by the National Natural Science Foundation of China (No. 22202029, 22372022, 22372169); the Technology Development Contract of Sinopec (Grant No. 324021, KL324009).
Conflict of interest
The authors declare that the research was conducted in the absence of any commercial or financial relationships that could be construed as a potential conflict of interest.
Publisher’s note
All claims expressed in this article are solely those of the authors and do not necessarily represent those of their affiliated organizations, or those of the publisher, the editors and the reviewers. Any product that may be evaluated in this article, or claim that may be made by its manufacturer, is not guaranteed or endorsed by the publisher.
References
Campa, M. C., Doyle, A. M., and Fierro, G. (2021). Pietrogiacomi D., Simultaneous abatement of NO and N2O with CH4 over modified Al2O3 supported Pt, Pd, Rh. Catal. Today. 384-386, 76–87. doi:10.1016/j.cattod.2021.06.020
Campa, M. C., Pietrogiacomi, D., and Occhiuzzi, M. (2015). The simultaneous selective catalytic reduction of N2O and NOx with CH4 on Co- and Ni-exchanged mordenite. Appl. Catal. B Environ. 168−169, 293–302. doi:10.1016/j.apcatb.2014.12.040
Chen, S. W., Yan, X. L., Wang, Y., Chen, J. Q., Pan, D. H., Ma, J. H., et al. (2011). Effect of SO2 on Co sites for NO-SCR by CH4 over Co-Beta. Catal. Today. 175 (1), 12–17. doi:10.1016/j.cattod.2011.05.024
Chen, X. M., Zhu, A. M., Au, C., and Shi, C. (2011). Enhanced low-temperature activity of Ag-promoted Co-ZSM-5 for the CH4-SCR of NO. Catal. Lett. 14, 207–212. doi:10.1007/s10562-010-0435-8
Chupin, C., van Veen, A. C., Konduru, M., Després, J., and Mirodatos, C. (2006). Identity and location of active species for NO reduction by CH4 over Co-ZSM-5. J. Catal. 241 (1), 103–114. doi:10.1016/j.jcat.2006.04.025
Costilla, I. O., Sanchez, M. D., Volpe, M. A., and Gigola, C. E. (2011). Ce effect on the selective catalytic reduction of NO with CH4 on Pd-mordenite in the presence of O2 and H2O. Catal. Today. 172 (1), 84–89. doi:10.1016/j.cattod.2011.03.025
Du, J., Han, S., Huang, C., Shan, Y., Zhang, Y., Shan, W., et al. (2023). Comparison of precursors for the synthesis of Cu-SSZ-39 zeolite catalysts for NH3-SCR reaction. Appl. Catal. B Environ. 338, 123072. doi:10.1016/j.apcatb.2023.123072
Han, L. P., Cai, S. X., Gao, M., Hasegawa, J., Wang, P., Zhang, J., et al. (2019). Selective catalytic reduction of NOx with NH3 by using novel catalysts: state of the art and future prospects. Chem. Rev. 119 (19), 10916–10976.
Huang, F. J., Hu, W., Chen, J. J., Wu, Y., Qu, P. F., Yuan, S., et al. (2018). Insight into enhancement of NO reduction with methane by multifunctional catalysis over a mixture of Ce/HZSM-5 and CoO<i>x</i> in excess of oxygen. Ind. Eng. Chem. Res. 57 (40), 13312–13317. doi:10.1021/acs.iecr.8b00773
Jin, G. H., Li, J. H., Yang, D., and Hao, J. M. (2009). Progress of selective catalytic reduction of NOx with methane over zeolite-based catalysts. Chem. Ind. Eng. Prog. 28 (3), 504–510.
Kruk, M., and Jaroniec, M. (2001). Gas adsorption characterization of ordered organic-inorganic nanocomposite materials. Chem. Mater. 13 (10), 3169–3183. doi:10.1021/cm0101069
Kubacka, A., Janas, J., and Sulikowski, B. (2006). In/Co-Ferrierite: a highly active catalyst for the CH4-SCR no process under presence of steam. Appl. Catal. B Environ. 69 (1), 43–48. doi:10.1016/j.apcatb.2006.05.014
Lee, T. J., Nam, I. S., Ham, S. W., Baek, Y. S., and Shin, K. H. (2003). Effect of Pd on the water tolerance of Co-ferrierite catalyst for NO reduction by CH4. Appl. Catal. B Environ. 41 (1), 115–127. doi:10.1016/s0926-3373(02)00197-2
Li, G., Jiao, F., Miao, D., Wang, Y., Pan, X., Yokoi, T., et al. (2019). Selective conversion of syngas to propane over ZnCrO -SSZ-39 OX-ZEO catalysts. J. Energy Chem. 36, 141–147. doi:10.1016/j.jechem.2019.07.006
Lim, J. B., Shin, J., Ahn, N. H., Heo, I., and Hong, S. B. (2020). Selective catalytic reduction of NO with CH4 over cobalt-exchanged cage based, small-pore zeolites with different framework structures. Appl. Catal. B Environ. 267, 118710. doi:10.1016/j.apcatb.2020.118710
Martin, J., Avila, P., Suarez, S., Yates, M., Martinrojo, A., Barthelemy, C., et al. (2006). Influence of support acid pretreatment on the behaviour of CoOx/γ-alumina monolithic catalysts in the CH4-SCR reaction. Appl. Catal. B Environ. 67 (3-4), 270–278. doi:10.1016/j.apcatb.2006.05.010
Mrad, R., Aissat, A., Cousin, R., Courcot, D., and Siffert, S. (2015). Catalysts for NOx selective catalytic reduction by hydrocarbons (HC-SCR). Appl. Catal. A Gen. 504, 542–548. doi:10.1016/j.apcata.2014.10.021
Ren, L., Zhu, L., Yang, C., Chen, Y., Sun, Q., Zhang, H., et al. (2011). Designed copper-amine complex as an efficient template for one-pot synthesis of Cu-SSZ-13 zeolite with excellent activity for selective catalytic reduction of NOx by NH3. Chem. Commun. 47 (35), 9789–9791. doi:10.1039/c1cc12469b
Sazama, P., Pilar, R., Mokrzycki, L., Vondrova, A., Kaucky, D., Plsek, J., et al. (2016). Remarkably enhanced density and specific activity of active sites in Al-rich Cu-Fe-and Co-beta zeolites for selective catalytic reduction of NOx. Appl. Catal. B Environ. 189, 65–74. doi:10.1016/j.apcatb.2016.02.020
Shi, C., Cheng, M. J., Qu, Z. P., and Bao, X. H. (2004). Investigation on the catalytic roles of silver species in the selective catalytic reduction of NO with methane. Appl. Catal. B-Environ. 51, 171–181. doi:10.1016/j.apcatb.2003.12.003
Wang, C., Wang, J., Wang, J., Yu, T., Shen, M., Wang, W., et al. (2017). The effect of sulfate species on the activity of NH3-SCR over Cu/SAPO-34. Appl. Catal. B Environ. 204, 239–249. doi:10.1016/j.apcatb.2016.11.033
Wang, X. F., Zhao, Z., Xu, Y., and Li, Q. B. (2021). Promoting effect of Ti addition on three-dimensionally ordered macroporous Mn-Ce catalysts for NH3-SCR reaction: enhanced N2 selectivity and remarkable water resistance. Appl. Surf. Sci. 569, 151047. doi:10.1016/j.apsusc.2021.151047
Wen, N. N., Lin, R., Su, Y. X., Deng, W. Y., Zhou, H., and Zhao, B. T. (2021). SCR of NO with CH4 over Fe/Ga2O3-Al2O3 and the mechanism. J. Environ. Chem. Eng. 9 (1), 105014. doi:10.1016/j.jece.2020.105014
Xu, Y., Wang, X. F., Qin, M. Y., and Li, Q. B. (2022). Selective catalytic reduction of NOx with CH4 over zeolite catalysts: research progress, challenges and perspectives. J. Environ. Chem. Eng. 10 (2), 107270. doi:10.1016/j.jece.2022.107270
Yoo, J. S., Khan, T. S., Abild-Pedersen, F., Nørskov, J. K., and Studt, F. (2015). On the role of the surface oxygen species during A-H (A =C, N, O) bond activation: a density functional theory study. Chem. Commun. 51, 2621–2624. doi:10.1039/c4cc08658a
Keywords: CH4-SCR, In/SSZ-39, highly dispersed InOx species, low temperature activation of CH4, high CH4 selectivity
Citation: An S, Wang P, Wang K, Wang X, Li B and Guo X (2024) Efficient In/SSZ-39 catalysts for the selective catalytic reduction of NO with CH4. Front. Chem. 12:1439581. doi: 10.3389/fchem.2024.1439581
Received: 28 May 2024; Accepted: 10 July 2024;
Published: 26 July 2024.
Edited by:
Dedong He, Kunming University of Science and Technology, ChinaReviewed by:
Beibei Gao, Zhengzhou University, ChinaIvaylo Tankov, Assen Zlatarov University, Bulgaria
Junjie Li, Chinese Academy of Sciences (CAS), China
Copyright © 2024 An, Wang, Wang, Wang, Li and Guo. This is an open-access article distributed under the terms of the Creative Commons Attribution License (CC BY). The use, distribution or reproduction in other forums is permitted, provided the original author(s) and the copyright owner(s) are credited and that the original publication in this journal is cited, in accordance with accepted academic practice. No use, distribution or reproduction is permitted which does not comply with these terms.
*Correspondence: Sufeng An, YW5zdWZlbmcuZnNoeUBzaW5vcGVjLmNvbQ==; Xinwen Guo, Z3VveHdAZGx1dC5lZHUuY24=