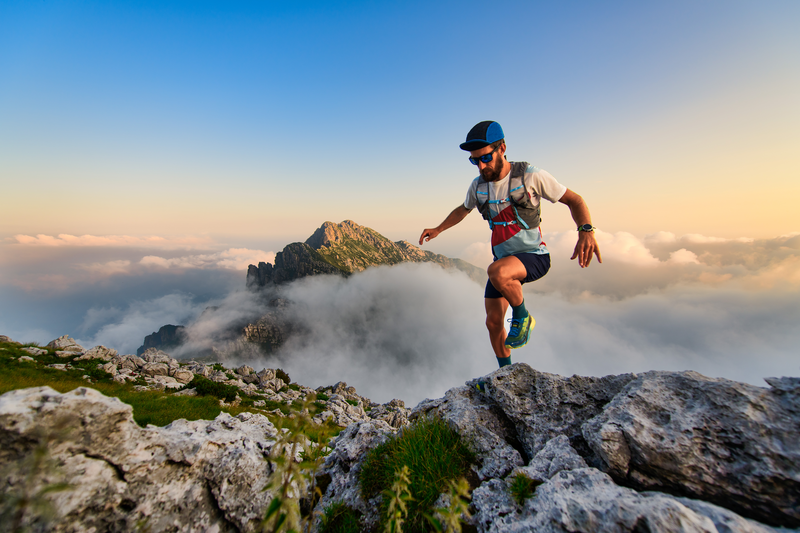
94% of researchers rate our articles as excellent or good
Learn more about the work of our research integrity team to safeguard the quality of each article we publish.
Find out more
REVIEW article
Front. Chem. , 05 July 2024
Sec. Medicinal and Pharmaceutical Chemistry
Volume 12 - 2024 | https://doi.org/10.3389/fchem.2024.1439167
Dihydroquercetin (DHQ) is a representative of flavonoids that is available on the market as a food supplement and registered as an active pharmaceutical ingredient. The structure of this compound is characterized by the presence of two chiral centers in positions 2 and 3 of the pyranone ring. Current regulatory documentation on DHQ lacks quantitative analysis of the stereoisomers of this flavanonol. This poses potential risks for consumers of DHQ-based dietary supplements and developers of new drugs. This review was conducted to systematize data on the pharmacology of DHQ stereoisomers and the possible methods of controlling them in promising chiral drugs. We found that relying on literature data of polarimetry for the identification of DHQ stereoisomers is currently impossible due to these heterogeneities. NMR spectroscopy allows to distinguishing between trans- and cis-DHQ using chemical shifts values. Only HPLC is currently characterized by sufficient enantioselectivity. Regarding pharmacology, the most active stereoisomer of DHQ should be identified, if the substituents in chiral centers both take part in binding with the biological target. The significant impact of stereochemical structure on the pharmacokinetics of DHQ isomers was reported. The question about these toxicity of these compounds remains open. The results of the conducted review of scientific literature indicate the necessity of revising the pharmacology of DHQ taking into account its stereoisomerism.
Throughout the history of pharmaceutical science, herbal materials have been the focus of investigators (Bokov and Samylina, 2015; Bokov et al., 2020; Fitzgerald et al., 2020; Giannenas et al., 2020). Nowadays, natural compounds continue to be promising objects for drug development (Du et al., 2020; Shikov et al., 2021; Savelyeva et al., 2022; Rehman et al., 2023; Yang et al., 2023). Newman and Cragg (2020) reported that from 1981 to 2019 there was 1881 new remedies, which were approved for medical application, and the 22.9% of them are of natural origin. Phytotherapy plays an important role in the treatment of cardiovascular (Kirichenko et al., 2020; Archakova et al., 2022; Karahan et al., 2022), immune (Babich et al., 2020; Ligacheva et al., 2022; Abdugafurova et al., 2024), cancer (Medvedev et al., 2020; Kitaeva et al., 2022; Jia-Xing et al., 2023), and infectious diseases (Gorlenko et al., 2020; Luzhanin et al., 2022; Zrig, 2022). According to the WHO, herbal medications are the main choice in many regions of the world (Aware et al., 2022).
The number of natural compounds cannot be counted. In 1978, the Chemical Abstract Services contained information about 4,039,907 structures (Maugh, 1978), and now it is more than 279 million. In pharmaceutical science, natural compounds are classified based on their chemical structure to systematize their biological effects, extraction approaches, and chemical control.
Flavonoids are a group of natural polyphenols that widely occur in different parts of plants. They are derivatives of 1,3-diphenyl propane. The aglycones of flavonoids are characterized by acidic chemical centers, high solubility in alcohols and hot water, and high free radical scavenging capacity (Ilyasov et al., 2018; Ilyasov et al., 2020; Elapov et al., 2021; Agatonovic-Kustrin et al., 2022; Poluyanov et al., 2023). Traditionally, researchers associate the pharmacological effects of flavonoids with their antioxidant activity (Shilova et al., 2017; Shevelev et al., 2020; Mordvinov et al., 2021). However, currently, there is an increase in data that argues a contrary view: Flavonoids selectively bind to biological targets (Shubina et al., 2023; Taldaev et al., 2022B). With this knowledge in mind, the importance of flavonoids’ stereochemistry and the impact of different stereoisomers on human health is recognized.
Dihydroquercetin (DHQ), also known as taxifolin, is a representative of flavonoids, specifically, flavanonols. The systematic IUPAC name of this compound is 2,3-dihydro-3,5,7-trihydroxy-2-(3,4-dihydroxyphenyl)-4H-1-benzopyran-4-one. The structure of this flavonoid is characterized by the presence of two chiral centers in positions 2 and 3 of the pyranone ring (Figure 1). This fact results in four possible stereoisomers of DHQ: a pair of enantiomers—(2R,3R)-DHQ and (2S,3S)-DHQ—are trans-DHQ, and another pair—(2R,3S)-DHQ and (2S,3R)-DHQ—are cis-DHQ. The pairs of trans- and cis-isomers are diastereomers of each other.
Diquertin® is the first pharmaceutical product based on DHQ that has been registered in Russia. It was approved as an antioxidant in the treatment of acute pneumonia, chronic obstructive pulmonary disease, asthma, coronary heart disease, and supraventricular arrhythmia (Kolhir et al., 1998; Plotnikov et al., 2003). The reference sample of DHQ was developed to standardize the active pharmaceutical ingredient (API) of Diquertin®. Using chiroptical analytical methods, it was discovered that this sample consists of (2R,3R)-DHQ (Selivanova et al., 1999).
Currently, DHQ is registered as an API in the Russian Federation and the Republic of Kazakhstan. In the European Union, it is on the market as a food supplement. Various preclinical and clinical studies are ongoing in Russia, China, and Japan (Shinozaki et al., 2023; Taldaev et al., 2023; Choi et al., 2024).
Larix wood is a natural source for DHQ. Russia has a rich source of this raw material. Since 1970, 31 technologies related to the extraction of DHQ from natural sources have been patented in Russia (Table 1). The majority of these technologies involve the use of water and high temperatures, with 80.6% and 51.6% of the technologies describing them, respectively. In some cases, the resulting extract was purified by HPLC (SU 351847 A1, RU 2114631 C1, RU 2317093 C1, RU 2349 31 C1, RU 2359666 C2, and RU 2 435 766 C1). To increase the extraction yield, raw materials were mechanoactivated with a solid base (RU 2307834 C1 and RU 2 386 624 C2). In 2020, a patent for the extraction of DHQ with supercritical carbon dioxide was granted (RU 2 747 696 C1). Additionally, in 12.9% of cases, highly active acidic and basic agents are required. All these conditions can lead to isomerization of the flavonoid molecule (Kiehlmann and Li, 1995). Recent studies have confirmed these findings. For example, it was discovered that up to 3.7% of the molecules in commercially available DHQ samples have a cis-configuration (Terekhov et al., 2024A). Furthermore, spray drying was associated with a significant increase in the amount of the cis-isomer, reaching 9.5% (Taldaev et al., 2022A).
However, current regulatory documentation on DHQ lacks indicators for the quantitative analysis of the stereoisomers of this flavanonol. Nevertheless, the Guidance document, published by FDA in 1992, prescribes to identify compounds with chiral centers and to know the quantitative isomeric composition of the material used in pharmacy (US Food and Drug Administration, 1992). The absence of proper control in this area poses potential risks for both consumers of DHQ-based dietary supplements and developers of new drugs. This literature review aimed to organize data on the pharmacology of DHQ stereoisomers and possible methods of controlling them in promising chiral drugs based on it.
The literature data deposited in MEDLINE were used to build a bibliometric network based on keywords co-occurring with the terms “taxifolin” and “dihydroquercetin” in articles (Figure 2A).
Figure 2. Bibliometric network of terms “taxifolin” and “dihydroquercetin”: (A) general view of network; (B) co-occurrence terms for “stereoisomerism”. Created by VOSviewer (Van Eck and Waltman, 2010; Van Eck and Waltman, 2011; Waltman and van Eck, 2013).
The size of bubbles corresponds to the frequency of term mentions. The pseudocolor scale from blue to yellow reflects the novelty of articles from 2001 or earlier to the year 2024. What stands out in this figure is a pronounced trend in the area of DHQ investigation from the traditional analysis of plant extracts using standard chromatographic and spectral approaches to omics research (Mei et al., 2019; Mao et al., 2022). Additionally, these data confirm the increasing interest of investigators in the selective interaction of DHQ with different biological targets, such as nuclear factor erythroid 2-related factor 2 (NRF-2) (Turovskaya et al., 2019) and nuclear factor κ-light-chain-enhancer of activated B cells (NF-κB) (Akinmoladun et al., 2022). The opportunities for using DHQ in the therapy of socially significant diseases (Alzheimer’s disease and COVID-19) are increasingly in focus of research (Zhu et al., 2022; Hattori et al., 2023; Saxena et al., 2024). Furthermore, new computational methods of analysis have been implemented in research practice (Terekhov et al., 2019; Rudrapal et al., 2022; Ahmad et al., 2024).
Figure 2B shows a decrease in interest in the stereochemistry of DHQ over the last 10 years. In the latest articles, this topic is discussed in the context of the individual presence of DHQ enantiomers in plant extracts (Vega-Villa et al., 2009; Gaggeri et al., 2012). It is notable that in these studies, HPLC was used to separate the individual DHQ substances. Additionally, in some cases, chiroptical analytical methods, such as circular dichroism spectroscopy, were used to identify the configuration of the flavanonol molecule.
The next sections will focus on the major stereoselective analytical approaches used to control the configuration of DHQ molecules.
Polarimetric analysis is the primary routine tool in the pharmacist’s arsenal for assessing the stereochemical composition of APIs.
Over the 76-year period of DHQ study, an impressive amount of data on the ability of solutions of different stereoisomers of this flavanonol to rotate the polarization plane of monochromatic light has been accumulated (Table 2).
According to the literature data, alcohols and less frequently acetone are mainly used as solvents for the analyzed DHQ samples. The scientific community’s commitment to using these solvents is explained by DHQ’s solubility. Higher concentration is associated with better values of trueness, precision, and robustness. Limited solubility in combination with a small value of the specific rotation angle leads to a significant error in the results, especially in the absence of automated measurement. It is important to note that data obtained for samples in different solvents are not comparable due to the medium’s effect on the value of the rotation angle of the polarization plane of light.
Methanol is most often used as a solvent. However, the available data are characterized by pronounced heterogeneity. Thus, for (2R,3R)-DHQ, the specific rotation values vary from + 4.1° to + 35.6°, with a mean value of + 20.8° ± 6.9°. No correlation was found between temperature and the measured value (r2 = 0.0299). Based on the symmetry of the funnel plot, we can say that there are no publication errors or data defaults on the part of researchers (Figure 3). Obviously, the accuracy of the measurement increases with increasing concentration. However, the proportion of drop-out points remains high, so it is necessary to refine the available data by repeating measurements in different laboratories.
Figure 3. The funnel plot of relationship between (2R,3R)-DHQ concentrations in methanol solutions and values of specific ration angle (p = 0.01).
The specific rotation angle value for (2R,3R)-DHQ in ethanol ranges from +12.0° to +21.1° (mean value + 16.6° ± 6.4°) and in acetone from +17.3° to +24.6° (mean value + 21.1° ± 3.7°).
Data on the optical activity of other stereoisomers of DHQ is scarce. For solutions of (2S,3S)-DHQ in methanol, values of the specific rotation angle equal to −15.0° and −16.2° are given, and in acetone −20.6°. For the methanol solution of (2R,3S)-DHQ, a single value of −20.0° has been described. From the data in Table 2, it can be stated that in acetone, the modulus of the specific rotation angle value of the stereoisomers of DHQ is higher than in alcohols. It should also be emphasized that the authors do not always provide a full description of the methods for obtaining the optical activity data, which makes it difficult to reproduce the experiment and confirm the results.
Several explanations for the observed heterogeneity in the polarimetry data are possible. One reason is the imperfection of the analysis technique and the human factor. In addition, it may be a result of the presence of accompanying optically active impurities, including other stereoisomers of DHQ.
Therefore, reliance on literature data of polarimetry for the identification of stereoisomers of DHQ is currently impossible. Repeated studies are required in this area, considering the transition to a new technological level and automated analytical equipment.
In contrast to polarimetry, nuclear magnetic resonance (NMR) spectroscopy is rarely mentioned in pharmacopeial monographs on APIs and remains mainly a tool for researchers. Data on the spectral characteristics of DHQ stereoisomers has accumulated (Table 3).
This method of analysis does not allow for the identification of enantiomers but can differentiate between diastereomers. There is considerably more information on NMR spectra for trans-DHQ than for the cis-configuration of this flavanonol. Deuterated methanol, and less frequently acetone and dimethyl sulfoxide, are predominantly used for NMR spectroscopy, which can be attributed to the solubility of DHQ.
The trans- and cis-isomers can be identified by the chemical shift (δ) values for the signals of hydrogen atoms at positions 2 and 3 of the pyranone ring and by the spin-spin coupling constants (J) between them. The NMR spectrum of trans-DHQ is characterized by signals with chemical shift values of 4.96 ± 0.05 ppm and 4.54 ± 0.05 ppm belonging to hydrogen atoms at positions 2 and 3, respectively. For the cis-isomer, these parameters were 5.32 ± 0.06 ppm and 4.21 ± 0.04 ppm, respectively.
The spin-spin coupling constants for the DHQ molecules existing in trans- and cis-configurations were 11.5 ± 0.2 Hz and 2.8 ± 0.2 Hz, respectively. These data can be used to calculate the dihedral angles (φ) between hydrogen atoms in positions 2 and 3 for DHQ diastereomers using the Haasnoot-de Leeuw-Altona equation:
where Δχi is the electronegativity differences of the substituents, ξ is the sign parameter takes the value + 1 or −1, depending on the orientation of the substituent, P1, P2, P3, P4, P5, and P6 are empirically derived parameters, which in our case equal to 13.88, −0.81, 0, 0.56, −2.32, and 17.90, respectively (Haasnoot et al., 1980; Coxon, 2009). So, the molecule of trans-DHQ is characterized by dihedral angle of 179.0 ± 8.4° between these atoms, while in cis-molecule this structural parameter is equal to 68.0 ± 1.5°. The Newmans projections of these conformation are presented in Figure 4. The calculation and visualization were performed in MestReJ [Navarro-Vázquez et al.].
Figure 4. The Newmans projections of DHQ conformation, calculated by NMR data: (A) trans-DHQ; (B) cis-DHQ. Created by MestReJ (Navarro-Vázquez et al., 2004).
These characteristics allow us to reliably distinguish between the two diastereomers. However, authors of publications often omit details of the methods of analysis, making it difficult to reproduce the results. Enriching the profiles of NMR spectra of DHQ stereoisomers with data from 2D spectroscopy, particularly heteronuclear single-quantum correlation spectroscopy (HSQC) and spectroscopy with the Overhauser effect (NOESY), will help define the structural characteristics of the molecules of the DHQ diastereomers more accurately.
Chromatography allows for the identification and control of the quantity of both the main component and accompanying impurities, making it a more universal analytical method. Semi-preparative chromatography is used for the purification and accumulation of a specific compound. However, information on the chromatography of stereoisomers of DHQ is more modest (Table 4) than in the case of the methods discussed above.
Reversed-phase chromatography was used in all detected methods. In general, the elution conditions are usual for DHQ: acid-modified mobile phase with a major proportion of water and acetonitrile or methanol as an organic solvent.
Due to differences in the physicochemical properties of the diastereomers, trans- and cis-DHQ can be detected and analysed by high-performance liquid chromatography (HPLC). The separation of DHQ diastereomers using silica gel with grafted biphenyl radicals as a stationary phase has been described. The number of theoretical plates (NTP) for trans- and cis-DHQ was 20,351 and 26,285, respectively, and the peak-to-peak resolution (Rs) was 5.3. These data indicates a high efficacy of diastereomers separation, which can be a result of π,π-stacking between the molecules of stationary phase and the flavanonol. The total elution time for a single sample takes 15 min. Thus, the methodology designed for the identification of diastereomers of DHQ appears to be adapted to the needs of the pharmaceutical industry.
The separation of a mixture of enantiomers requires the creation of chiral conditions in a mobile or stationary phase. Tris-(4-methylbenzoate)-cellulose was used as a chiral stationary phase in two articles. Despite good separation efficacy of all four stereoisomers, their retention times (RT) remained impressive and required up to 126 min of waiting time, which is unappropriated for routine analysis. Nevertheless, this method is used for preparative separation of DHQ enantiomers.
The observed data indicate good intermediate results in the chromatographic separation of DHQ stereoisomers. However, further research is required to develop techniques to identify DHQ enantiomers more reliably and rapidly.
A number of articles devoted to exploring the biological effects of DHQ are published annually. However, these studies are conducted with no relation to the stereochemical structure of DHQ. In the current review, we tried to accumulate the data that were obtained with respect to the stereoisomerism of this flavonoid and provide an opportunity to compare the affinities of isomers to different biological targets. In the case of in vivo or in vitro experiments, the configuration of DHQ molecules should be confirmed by any analytical method, e.g., polarimetry. We found only 3 studies that met these criteria (Table 5).
This table is quite revealing in several ways. First, the study of differences in pharmacological properties of DHQ stereoisomers is in the early stages: The majority of data were obtained in silico and there are no experimental results in vivo. Even if authors took into account the stereoisomerism of this flavonoid, the most biology tests performed were conducted with a reduced collection of DHQ enantiomers. So, Oi et al. (2012) designed the research of anti-cancer properties of DHQ in translation form, including the stages in silico, in vitro, ex vivo, and in vivo. However, the pure (85%) (2R,3R)-DHQ was used during the in vitro test, and the in vivo experiment was performed with a mixture of (2R,3R)- and (2S,3R)-isomers, which characterized 90% purity. In another case, Sato et al. (2013) synthesized the racemate of trans-DHQ, using the modified Roschek et al. (2009) method, and separated the individual enantiomers. Although the purity of the generated DHQ samples was not provided, the ability to aggregate the 42-residue amyloid β proteins (Aβ42) was assessed for both trans-enantiomers. We suppose that the main reason for the observed gap in this research area is the difficulties in obtaining pure samples of DHQ isomers in amounts that would be enough to conduct the in vivo study. With that knowledge in mind, the in silico analysis looks preferable for the screening of pharmacology activity. However, these should be approved in moist biology experiments (Taldaev et al., 2022B).
What is interesting about the data in this table is that depending on the biological target structure, the difference in DHQ isomers’ affinities may be quite high or absolutely absent. For example, in the case of epidermal growth factor receptor (EGFR) and phosphoinositide 3-kinase (PI3-K), the molecule of DHQ builds in ATP-binding sites by phenolic groups in positions 3′, 4′, and 5. As the pyrocatechinic substitute can freely rotate about σ-bound and the hydroxyl group in position 3 did not take part in supramolecular interaction, the configuration of chiral centers does not affect the affinity of DHQ to these proteins. The similar explanation can be provided in the case of Aβ42. Using computational analysis, Ginex et al. (2018) showed that the covalent binding of the oxidized pyrocatechinic ring with Lys16 plays the primary role in aggregation of this biological target. The inhibition of the main protease (Mpro) of Severe Acute Respiratory Syndrome CoronaVirus 2 (SARS-CoV-2) demonstrates another situation. Fischer et al. (2020) reported that the DHQ molecule interacts with Mpro by phenolic groups in positions 3′ and 7 forming H-bonds with Gln192 and Cys44, respectively. However, the (2S,3S)-configuration provides an opportunity to generate an additional bound between the alcohol group in position 3 and Glu166 resulting in better affinity of (2S,3S)-DHQ to Mpro. These data confirmed that configuration of chiral centers in flavanonol molecule affects on the efficacy of its interaction with protein. This knowlege should be taken into accounte during the development of new chiral drugs.
Due to the lack of stereoselective analytical methods for the quantitative control of DHQ isomers, the data on pharmacokinetics is poor. The only article on this topic in rats was published in 2009 by Vega-Villa et al. (2009), where the HPLC approach was used. Later, these data were discussed more broadly (Vega-Villa et al., 2011).
The significant impact of stereochemical structure on the pharmacokinetics of DHQ isomers was discussed. The elimination half-life (t1/2) of (2R,3R)-DHQ was 14.63 ± 2.18 h, and it was at least 1.6 times lower than the value of this parameter for any other enantiomer of this flavonoid. The reason for this result is not explained. Probably, the amount of (2R,3R)-DHQ was much higher than the other three isomers, the binding by blood proteins was not full, and a significant part of this enantiomer was excreted.
Comparing with cis-DHQ, the renal and hepatic clearances of trans-DHQ were 22 times lower and 18 times higher, respectively. The 2R-configuration was associated with a greater elimination rate. Another interesting observation was the accumulation of DHQ isomers in the flesh and skin. The percentage of cis-diastereomers was a greater order than for trans-isomers. Furthermore, the 2S-configuration was associated with higher accumulation in body tissues.
This study has several limitations. The chromatographic parameters on initial racemic mixture of DHQ were not presented. The AUC data reported in both articles are contradictory. As mentioned by Vega-Villa et al. (2011), the pharmacokinetics of DHQ was not adequately researched, as the sampling period was not enough to assess exposure. Additionally, the biochemistry of rats and humans differs significantly, so it is not appropriate to transfer data from one species to another. Taken together, these limitations indicate the necessity of further research on the pharmacokinetics of DHQ stereoisomers.
The present review was undertaken to clarify the current state of DHQ stereoisomerism in pharmaceutical science. In the course of the work, it was found that due to the heterogeneity of data on the optical activity of flavanonol molecules, it is difficult to rely on the results of polarimetry when conducting research and developing regulatory documentation. The information on NMR spectroscopy of DHQ diastereomers is more reliable; however, this method does not provide an opportunity to identify individual stereoisomers and has not been implemented in routine pharmaceutical analysis. Of all the methods considered, only HPLC is currently characterized by sufficient enantioselectivity. The wide variety of identified biological effects of DHQ in the absence of information about the substance and evidence of its stereoisomeric composition does not give confidence in the effectiveness and safety. The results of the conducted review of scientific literature indicate the necessity of revision in the pharmacology of DHQ taking into account its stereoisomerism.
RT: Conceptualization, Funding acquisition, Project administration, Visualization, Writing–original draft. AS: Data curation, Investigation, Writing–original draft. DP: Data curation, Methodology, Writing–review and editing. MK: Investigation, Writing–review and editing. AT: Formal Analysis, Methodology, Software, Validation, Visualization, Writing–review and editing. LY: Resources, Software, Writing–review and editing. SZ: Formal Analysis, Resources, Writing–review and editing. AZ: Formal Analysis, Project administration, Writing–review and editing. IS: Conceptualization, Supervision, Writing–review and editing.
The author(s) declare that financial support was received for the research, authorship, and/or publication of this article. This work was supported by a grant from the Russian Science Foundation, No. 23-75-01130 (chemical aspects), and by the Ministry of Science and Higher Education of the Russian Federation within the framework of state support for the creation and development of World-Class Research Centers, “Digital biodesign and personalized healthcare”, №075-15-2022-305 (pharmacological aspects).
The authors declare that the research was conducted in the absence of any commercial or financial relationships that could be construed as a potential conflict of interest.
All claims expressed in this article are solely those of the authors and do not necessarily represent those of their affiliated organizations, or those of the publisher, the editors and the reviewers. Any product that may be evaluated in this article, or claim that may be made by its manufacturer, is not guaranteed or endorsed by the publisher.
Abdugafurova, D. G., Oripova, M.Zh., Amanlikova, D. A., Mahmudov, L. U., Koraboeva, B. B., Kuzieva, Z. N., et al. (2024). Study of the immunomodulatory effect of polysaccharides isolated from seeds of turnip BRASSICA RAPA. Pharm. Chem. J. 57, 1552–1556. doi:10.1007/s11094-024-03048-7
Agatonovic-Kustrin, S., Gegechkori, V., Kustrin, E., and Morton, D. W. (2022). The effect of lactic acid fermentation on extraction of phenolics and flavonoids from sage leaves. Appl. Sci. 12, 9959. doi:10.3390/app12199959
Ahmad, V., Alotibi, I., Alghamdi, A. A., Ahmad, A., Jamal, Q. M. S., and Srivastava, S. (2024). Computational approaches to evaluate the acetylcholinesterase binding interaction with taxifolin for the management of alzheimer’s disease. Mol. Basel Switz. 29, 674. doi:10.3390/molecules29030674
Akinmoladun, A. C., Famusiwa, C. D., Josiah, S. S., Lawal, A. O., Olaleye, M. T., and Akindahunsi, A. A. (2022). Dihydroquercetin improves rotenone-induced parkinsonism by regulating NF-κB-Mediated inflammation pathway in rats. J. Biochem. Mol. Toxicol. 36, e23022. doi:10.1002/jbt.23022
Archakova, O. A., Bagaeva, N. S., Komarov, T. N., Rogov, A. V., Shchelgacheva, D. S., Suvorova, A. V., et al. (2022). Pharmacokinetics study of the long-acting antiarrhythmic drug of lappaconitine hydrobromide (Allaforte®, JSC pharmcenter VILAR, Russia). Drug Dev. Regist. 11, 140–147. doi:10.33380/2305-2066-2022-11-1-140-147
Aware, C., Patil, D., Suryawanshi, S., Mali, P., Rane, M., Gurav, R., et al. (2022). Natural bioactive products as promising therapeutics: a review of natural product-based drug development. South Afr. J. Bot. 151, 512–528. doi:10.1016/j.sajb.2022.05.028
Babich, O., Sukhikh, S., Prosekov, A., Asyakina, L., and Ivanova, S. (2020). Medicinal plants to strengthen immunity during a pandemic. Pharmaceuticals 13, 313. doi:10.3390/ph13100313
Baderschneider, B., and Winterhalter, P. (2001). Isolation and characterization of novel benzoates, cinnamates, flavonoids, and lignans from riesling wine and screening for antioxidant activity. J. Agric. Food Chem. 49, 2788–2798. doi:10.1021/jf010396d
Bokov, D. O., Barkalova, V. E., Suslikova, M. A., Sokhin, D. M., Kakhramanova, S. D., Rendyuk, T. D., et al. (2020). Lathraea squamaria L. (orobanchaceae): a review of its botany, phytochemistry, traditional uses and pharmacology. Pharmacogn. J. 12, 667–673. doi:10.5530/pj.2020.12.98
Bokov, D. O., and Samylina, I. A. (2015). Applications of snowdrop medicinal plant material in homeopathic pharmacy: a brief historical background and a systematic review of homeopathic medicines standardization. Sechenov Med. J. 2, 76–83.
Braune, A., Gütschow, M., Engst, W., and Blaut, M. (2001). Degradation of quercetin and luteolin by eubacterium ramulus. Appl. Environ. Microbiol. 67, 5558–5567. doi:10.1128/AEM.67.12.5558-5567.2001
Choi, M. C. Y., Law, T. H. P., Chen, S., Cheung, W. S. K., Yim, C., Ng, O. K. S., et al. (2024). Case report: taxifolin for neurosurgery-associated early-onset cerebral amyloid angiopathy. Front. Neurol. 15, 1360705. doi:10.3389/fneur.2024.1360705
Coxon, B. (2009). Developments in the Karplus equation as they relate to the NMR coupling constants of carbohydrates. Adv. Carbohydr. Chem. Biochem. 62, 17–82. doi:10.1016/S0065-2318(09)00003-1
Du, L., Zhang, R., Yang, H., Tang, S., Hou, Z., Jing, J., et al. (2020). Synthesis, characteristics and medical applications of plant nanomaterials. Planta 252, 108. doi:10.1007/s00425-020-03509-9
Elapov, A. A., Kuznetsov, N. N., and Marakhova, A. I. (2021). The use of ultrasound in the extraction of biologically active compounds from plant raw materials, used or promising for use in medicine (review). Drug Dev. Regist. 10, 96–116. doi:10.33380/2305-2066-2021-10-4-96-116
Elsinghorst, P. W., Cavlar, T., Müller, A., Braune, A., Blaut, M., and Gütschow, M. (2011). The thermal and enzymatic taxifolin-alphitonin rearrangement. J. Nat. Prod. 74, 2243–2249. doi:10.1021/np200639s
Fedorova, T. E., Fedorov, S. V., and Babkin, V. A. (2019). Phenolic compounds of picea obovata ledeb. Bark. Russ. J. Bioorg. Chem. 45, 927–932. doi:10.1134/S1068162019070045
Fischer, A., Sellner, M., Neranjan, S., Smieško, M., and Lill, M. A. (2020). Potential inhibitors for novel coronavirus protease identified by virtual screening of 606 million compounds. Int. J. Mol. Sci. 21, 3626. doi:10.3390/ijms21103626
Fitzgerald, M., Heinrich, M., and Booker, A. (2020). Medicinal plant analysis: a historical and regional discussion of emergent complex techniques. Front. Pharmacol. 10, 1480. doi:10.3389/fphar.2019.01480
Gaggeri, R., Rossi, D., Christodoulou, M. S., Passarella, D., Leoni, F., Azzolina, O., et al. (2012). Chiral flavanones from amygdalus lycioides spach: structural elucidation and identification of TNFalpha inhibitors by bioactivity-guided fractionation. Mol. Basel Switz. 17, 1665–1674. doi:10.3390/molecules17021665
Giannenas, I., Sidiropoulou, E., Bonos, E., Christaki, E., and Florou-Paneri, P. (2020). “Chapter 1 - the history of herbs, medicinal and aromatic plants, and their extracts: past, current situation and future perspectives,” in Feed additives. Editors P. Florou-Paneri, E. Christaki, and I. Giannenas (London: Academic Press), 1–18.
Ginex, T., Trius, M., and Luque, F. J. (2018). Computational study of the aza-michael addition of the flavonoid (+)-Taxifolin in the inhibition of β-amyloid fibril aggregation. Chem. Weinh. Bergstr. Ger. 24, 5813–5824. doi:10.1002/chem.201706072
Gorlenko, C. L., Kiselev, H. Y., Budanova, E. V., Zamyatnin, A. A., and Ikryannikova, L. N. (2020). Plant secondary metabolites in the battle of drugs and drug-resistant bacteria: new heroes or worse clones of antibiotics? Antibiotics 9, 170. doi:10.3390/antibiotics9040170
Haasnoot, C. A. G., de Leeuw, F. A. A. M., and Altona, C. (1980). The relationship between proton-proton NMR coupling constants and substituent electronegativities—I. Tetrahedron 36, 2783–2792. doi:10.1016/0040-4020(80)80155-4
Hattori, Y., Saito, S., Nakaoku, Y., Ogata, S., Hattori, M., Nakatsuji, M., et al. (2023). Taxifolin for cognitive preservation in patients with mild cognitive impairment or mild dementia. J. Alzheimers Dis. 93, 743–754. doi:10.3233/JAD-221293
Ilyasov, I., Beloborodov, V., Antonov, D., Dubrovskaya, A., Terekhov, R., Zhevlakova, A., et al. (2020). Flavonoids with glutathione antioxidant synergy: influence of free radicals inflow. Antioxidants 9, 695. doi:10.3390/antiox9080695
Ilyasov, I. R., Beloborodov, V. L., and Selivanova, I. A. (2018). Three ABTS•+ radical cation-based approaches for the evaluation of antioxidant activity: fast- and slow-reacting antioxidant behavior. Chem. Pap. 72, 1917–1925. doi:10.1007/s11696-018-0415-9
Imai, T., Inoue, S., Ohdaira, N., Matsushita, Y., Suzuki, R., Sakurai, M., et al. (2008). Heartwood extractives from the amazonian trees dipteryx odorata, hymenaea courbaril, and astronium lecointei and their antioxidant activities. J. Wood Sci. 54, 470–475. doi:10.1007/s10086-008-0975-3
Inada, A., Murata, H., Somekawa, M., and Nakanishi, T. (1992). Phytochemical studies of seeds of medicinal plants. II. A new dihydroflavonol glycoside and a new 3-methyl-1-butanol glycoside from seeds of platycodon grandiflorum A. DE candolle. Chem. Pharm. Bull. (Tokyo) 40, 3081–3083. doi:10.1248/cpb.40.3081
Jiang, W.-J., Ishiuchi, K., Furukawa, M., Takamiya, T., Kitanaka, S., and Iijima, H. (2015). Stereospecific inhibition of nitric oxide production in macrophage cells by flavanonols: synthesis and the structure-activity relationship. Bioorg. Med. Chem. 23, 6922–6929. doi:10.1016/j.bmc.2015.09.042
Jia-Xing, W., Chao-Yi, L., Wei-Ya, C., Yi-Jun, C., Chun-Yu, L., Fei-Fei, Y., et al. (2023). The pulmonary biopharmaceutics and anti-inflammatory effects after intratracheal and intravenous administration of Re-Du-Ning injection. Biomed. Pharmacother. 160, 114335. doi:10.1016/j.biopha.2023.114335
Karahan, F., Avşar, C., Turkmen, M., Gezici, S., and Ilcim, A. (2022). Comparative study on phytochemical profiles, antiproliferative, antimicrobial and antioxidant activities of adonis species from Turkey. Pharm. Chem. J. 56, 667–678. doi:10.1007/s11094-022-02693-0
Kasai, R., Hirono, S., Chou, W.-H., Tanaka, O., and Chen, F.-H. (1988). Sweet dihydroflavonol rhamnoside from leaves of engelhardtia chrysolepis, a Chinese folk medicine, hung-qi. Chem. Pharm. Bull. (Tokyo) 36, 4167–4170. doi:10.1248/cpb.36.4167
Kiehlmann, E., and Li, E. P. M. (1995). Isomerization of dihydroquercetin. J. Nat. Prod. 58, 450–455. doi:10.1021/np50117a018
Kirichenko, T. V., Sukhorukov, V. N., Markin, A. M., Nikiforov, N. G., Liu, P.-Y., Sobenin, I. A., et al. (2020). Medicinal plants as a potential and successful treatment option in the context of atherosclerosis. Front. Pharmacol. 11, 403. doi:10.3389/fphar.2020.00403
Kitaeva, M. P., Aksenov, A. A., Fedotcheva, T. A., Semeikin, A. V., and Shimanovsky, N. L. (2022). Cytotoxic activity of extracts from the intact plant and cell cultures of podophyllum peltatum against cervical cancer cells. Pharm. Chem. J. 56, 361–365. doi:10.1007/s11094-022-02652-9
Kolhir, V. K., Bykov, V. A., Teselkin, Yu.O., Babenkova, I. V., Tjukavkina, N. A., Rulenko, I. A., et al. (1998). Use of a new antioxidant diquertin as an adjuvant in the therapy of patients with acute pneumonia. Phytother. Res. 12, 606–608. doi:10.1002/(SICI)1099-1573(199812)12:8<606::AID-PTR367>3.0.CO;2-U
Kuroyanagi, M., Yamamoto, Y., Fukushima, S., Ueno, A., Noro, T., and Miyase, T. (1982). Chemical studies on the constituents of polygonum nodosum. Chem. Pharm. Bull. (Tokyo) 30, 1602–1608. doi:10.1248/cpb.30.1602
Kuspradini, H., Mitsunaga, T., and Ohashi, H. (2009). Antimicrobial activity against Streptococcus sobrinus and glucosyltransferase inhibitory activity of taxifolin and some fl avanonol rhamnosides from kempas (koompassia malaccensis) extracts. J. Wood Sci. 55, 308–313. doi:10.1007/s10086-009-1026-4
Lee, E. H., Kim, H. J., Song, Y. S., Jin, C., Lee, K.-T., Cho, J., et al. (2003). Constituents of the stems and fruits of opuntia ficus-indica var. Saboten. Arch. Pharm. Res. 26, 1018–1023. doi:10.1007/BF02994752
Ligacheva, A. A., Gulina, E. I., Shabanova, I. V., Trofimova, E. S., Krivoshchekov, S. V., Gurkin, N. V., et al. (2022). Effect of water-soluble polysaccharides plant extraction of the saussurea genus on the activity of mice peritoneal macrophage NO-synthase. Drug Dev. Regist. 11, 59–64. doi:10.33380/2305-2066-2022-11-2-59-64
Lundgren, L. N., and Theander, O. (1988). Cis- and trans-dihydroquercetin glucosides from needles of pinus sylvestris. Phytochemistry 27, 829–832. doi:10.1016/0031-9422(88)84101-3
Luzhanin, V. G., Whaley, A. K., Ponkratova, A. O., Novikova, V. V., and Bezverkhniaia, E. A. (2022). Antimicrobial activity of polyphenolic compounds. Drug Dev. Regist. 11, 65–72. doi:10.33380/2305-2066-2022-11-2-65-72
Mao, C., Li, L., Yang, T., Gui, M., Li, X., Zhang, F., et al. (2022). Transcriptomics integrated with widely targeted metabolomics reveals the cold resistance mechanism in hevea brasiliensis. Front. Plant Sci. 13, 1092411. doi:10.3389/fpls.2022.1092411
Maugh, T. H. (1978). Chemicals: how many are there? Science 199, 162. doi:10.1126/science.199.4325.162
Medvedev, Y. V., Kolganova, M. A., Sas, O. A., Komarov, T. N., Fisher, E. N., Shohin, I. E., et al. (2020). Immunogenicity assessment of pegfilgrastim in patients with breast cancer. Drug Dev. Regist. 9, 140–144. doi:10.33380/2305-2066-2020-9-2-140-144
Mei, X., Zhou, C., Zhang, W., Rothenberg, D. O., Wan, S., and Zhang, L. (2019). Comprehensive analysis of putative dihydroflavonol 4-reductase gene family in tea plant. PloS One 14, e0227225. doi:10.1371/journal.pone.0227225
Mohammed, H. A., Almahmoud, S. A., El-Ghaly, E.-S. M., Khan, F. A., Emwas, A.-H., Jaremko, M., et al. (2022). Comparative anticancer potentials of taxifolin and quercetin methylated derivatives against HCT-116 cell lines: effects of O-methylation on taxifolin and quercetin as preliminary natural leads. ACS Omega 7, 46629–46639. doi:10.1021/acsomega.2c05565
Mordvinov, V. A., Ponomarev, D. V., Pakharukov, Y. V., and Pakharukova, M. Y. (2021). Anthelmintic activity of antioxidants: in vitro effects on the liver fluke opisthorchis felineus. Pathogens 10, 284. doi:10.3390/pathogens10030284
Navarro-Vázquez, A., Cobas, J. C., Sardina, F. J., Casanueva, J., and Díez, E. (2004). A graphical tool for the prediction of vicinal Proton−Proton 3JHH coupling constants. J. Chem. Inf. Comput. Sci. 44, 1680–1685. doi:10.1021/ci049913t
Newman, D. J., and Cragg, G. M. (2020). Natural products as sources of new drugs over the nearly four decades from 01/1981 to 09/2019. J. Nat. Prod. 83, 770–803. doi:10.1021/acs.jnatprod.9b01285
Nifant’ev, E. E., Koroteev, M. P., Kaziev, G. Z., Uminskii, A. A., Grachev, A. A., Men’shov, V. M., et al. (2006). On the problem of identification of the dihydroquercetin flavonoid. Russ. J. Gen. Chem. 76, 161–163. doi:10.1134/S1070363206010324
Nonaka, G.-I., Goto, Y., Kinjo, J.-E., Nohara, T., and Nishioka, I. (1987). Tannins and Related Compounds. LII. Studies on the Constituents of the Leaves of Thujopsis Dolabrata SIEB. et ZUCC. Chem. Pharm. Bull. (Tokyo) 35, 1105–1108. doi:10.1248/cpb.35.1105
Ohmura, W., Ohara, S., Hashida, K., Aoyama, M., and Doi, S. (2002). Hydrothermolysis of flavonoids in relation to steaming of Japanese larch wood. Holzforschung 56, 493–497. doi:10.1515/HF.2002.076
Oi, N., Chen, H., Ok Kim, M., Lubet, R. A., Bode, A. M., and Dong, Z. (2012). Taxifolin suppresses UV-induced skin carcinogenesis by targeting EGFR and PI3K. Cancer Prev. Res. phila. Pa 5, 1103–1114. doi:10.1158/1940-6207.CAPR-11-0397
Plotnikov, M. B., Aliev, O. I., Maslov, M. J., Vasiliev, A. S., and Tjukavkina, N. A. (2003). Correction of haemorheological disturbances in myocardial infarction by diquertin and ascorbic acid. Phytother. Res. 17, 86–88. doi:10.1002/ptr.1082
Poluyanov, A. M., Sokolova, A. Y., Koynova, A.-D., Kulikova, S. D., Malashenko, E. A., and Bobkova, N. V. (2023). Identification and quantitative determination of flavonoids by HPLC-UV method in the raw materials of some representatives of the genus rumex of three vegetation time. Drug Dev. Regist. 12, 134–142. doi:10.33380/2305-2066-2023-12-3-134-142
Rehman, H., Ali, W., Javed, R., Khan, N. Z., Aasim, M., Khan, T., et al. (2023). In vitro evaluation of antioxidant, antimicrobial, cytotoxic, anti-inflammatory, anti-aging, enzyme inhibition potential, and HPLC analysis of traditionally used plant delphinium uncinatum wall. Pharm. Chem. J. 57, 1056–1069. doi:10.1007/s11094-023-02984-0
Roschek, B., Fink, R. C., McMichael, M. D., Li, D., and Alberte, R. S. (2009). Elderberry flavonoids bind to and prevent H1N1 infection in vitro. Phytochemistry 70, 1255–1261. doi:10.1016/j.phytochem.2009.06.003
Rudrapal, M., Issahaku, A. R., Agoni, C., Bendale, A. R., Nagar, A., Soliman, M. E. S., et al. (2022). In silico screening of phytopolyphenolics for the identification of bioactive compounds as novel protease inhibitors effective against SARS-CoV-2. J. Biomol. Struct. Dyn. 40, 10437–10453. doi:10.1080/07391102.2021.1944909
Sakurai, A., and Okumura, Y. (1983). Chemical studies on the mistletoe. V. The structure of taxillusin, a new flavonoid glycoside isolated from taxillus kaempferi. Bull. Chem. Soc. Jpn. 56, 542–544. doi:10.1246/bcsj.56.542
Sato, M., Murakami, K., Uno, M., Ikubo, H., Nakagawa, Y., Katayama, S., et al. (2013). Structure–activity relationship for (+)-Taxifolin isolated from silymarin as an inhibitor of amyloid β aggregation. Biosci. Biotechnol. Biochem. 77, 1100–1103. doi:10.1271/bbb.120925
Savelyeva, E. E., Bulgakova, N. A., Lapkina, E. Z., Chernaya, V. V., and Kurbatsky, V. I. (2022). Antimicrobial activity of water-alcoholic extracts of representatives of the genus potentilla L. Drug Dev. Regist. 11, 20–27. doi:10.33380/2305-2066-2022-11-4-20-27
Saxena, B., Parmar, P., Chauhan, H., Singh, P., Datusalia, A. K., Vyas, V. K., et al. (2024). Neuroprotective effect of taxifolin against aluminum chloride-induced dementia and pathological alterations in the brain of rats: possible involvement of toll-like receptor 4. Toxicol. Mech. Methods 34, 703–716. doi:10.1080/15376516.2024.2329653
Selivanova, I. A., Tyukavkina, N. A., Kolesnik, Yu.A., Nesterov, V. N., Kuleshova, L. N., Khutoryanskii, V. A., et al. (1999). Study of the crystalline structure of dihydroquercetin. Pharm. Chem. J. 33, 222–224. doi:10.1007/BF02509946
Shevelev, A. B., La Porta, N., Isakova, E. P., Martens, S., Biryukova, Y. K., Belous, A. S., et al. (2020). In vivo antimicrobial and wound-healing activity of resveratrol, dihydroquercetin, and dihydromyricetin against Staphylococcus aureus, Pseudomonas aeruginosa, and Candida albicans. Pathogens 9, 296. doi:10.3390/pathogens9040296
Shikov, A. N., Narkevich, I. A., Akamova, A. V., Nemyatykh, O. D., Flisyuk, E. V., Luzhanin, V. G., et al. (2021). Medical species used in Russia for the management of diabetes and related disorders. Front. Pharmacol. 12, 697411. doi:10.3389/fphar.2021.697411
Shilova, I. V., Suslov, N. I., Otmakhov, V. I., Zibareva, L. N., Samylina, I. A., Mazin, E. V., et al. (2017). Chemical and pharmacological study of herbal preparations that improve cognitive-mnestic functions. Pharm. Chem. J. 50, 654–658. doi:10.1007/s11094-017-1508-4
Shinozaki, F., Kamei, A., Shimada, K., Matsuura, H., Shibata, T., Ikeuchi, M., et al. (2023). Ingestion of taxifolin-rich foods affects brain activity, mental fatigue, and the whole blood transcriptome in healthy young adults: a randomized, double-blind, placebo-controlled, crossover study. Food Funct. 14, 3600–3612. doi:10.1039/d2fo03151e
Shubina, V. S., Kobyakova, M. I., and Shatalin, Yu.V. (2023). The effect of taxifolin, a conjugate of taxifolin with glyoxylic acid, and naringenin on the functional activity of neutrophils. Biophysics 68, 772–778. doi:10.1134/S0006350923050275
Taldaev, A., Savina, A. D., Olicheva, V. V., Ivanov, S. V., Terekhov, R. P., Ilyasov, I. R., et al. (2023). Protective properties of spheroidal taxifolin form in streptozotocin-induced diabetic rats. Int. J. Mol. Sci. 24, 11962. doi:10.3390/ijms241511962
Taldaev, A., Terekhov, R., Nikitin, I., Zhevlakova, A., and Selivanova, I. (2022a). Insights into the pharmacological effects of flavonoids: the systematic review of computer modeling. Int. J. Mol. Sci. 23, 6023. doi:10.3390/ijms23116023
Taldaev, A., Terekhov, R. P., Selivanova, I. A., Pankov, D. I., Anurova, M. N., Markovina, I. Y., et al. (2022b). Modification of taxifolin properties by spray drying. Sci. Pharm. 90, 67. doi:10.3390/scipharm90040067
Terekhov, R. P., Melnikov, E. S., Nikitin, I. D., Tokareva, M. A., Rodina, T. A., Savina, A. D., et al. (2024a). Diastereomers of spheroidal form and commercially available taxifolin samples. Sci. Pharm. 92, 5. doi:10.3390/scipharm92010005
Terekhov, R. P., Selivanova, I. A., Zhevlakova, A. K., Porozov, Y. B., and Dzuban, A. V. (2019). Analysis of dihydroquercetin physical modification via in vitro and in silico methods. Biomeditsinskaia Khimiia 65, 152–158. doi:10.18097/PBMC20196502152
Terekhov, R. P., Taldaev, A., Bocharov, E. V., Pankov, D. I., Savina, A. D., and Selivanova, I. A. (2024b). Analysis of cis-isomer-enriched dihydroquercetin sample by 1D and 2D NMR spectroscopy. Drug Dev. Regist. doi:10.33380/2305-2066-2024-13-2-1751
Trofimova, N. N., Stolpovskaya, E. V., Babkin, V. A., Fedorov, S. V., Kalabin, G. A., Goryainov, S. V., et al. (2015). The structure and electrochemical properties of metal complexes with dihydroquercetin. Russ. J. Bioorg. Chem. 41, 745–752. doi:10.1134/S1068162015070146
Turnbull, J. J., Nakajima, J.-I., Welford, R. W. D., Yamazaki, M., Saito, K., and Schofield, C. J. (2004). Mechanistic studies on three 2-oxoglutarate-dependent oxygenases of flavonoid biosynthesis: anthocyanidin synthase, flavonol synthase, and flavanone 3beta-hydroxylase. J. Biol. Chem. 279, 1206–1216. doi:10.1074/jbc.m309228200
Turovskaya, M. V., Gaidin, S. G., Mal’tseva, V. N., Zinchenko, V. P., and Turovsky, E. A. (2019). Taxifolin protects neurons against ischemic injury in vitro via the activation of antioxidant systems and signal transduction pathways of GABAergic neurons. Mol. Cell. Neurosci. 96, 10–24. doi:10.1016/j.mcn.2019.01.005
US Food and Drug Administration (1992). Development of new stereoisomeric drugs. Available at: https://www.fda.gov/regulatory-information/search-fda-guidance-documents/development-new-stereoisomeric-drugs (Accessed June 07, 2024).
Van Eck, N. J., and Waltman, L. (2010). Software survey: VOSviewer, a computer program for bibliometric mapping. Scientometrics 84, 523–538. doi:10.1007/s11192-009-0146-3
Van Eck, N. J., and Waltman, L. (2011). Text mining and visualization using VOSviewer. ISSI Newsl. 7, 50–54.
Vega-Villa, K. R., Remsberg, C. M., Ohgami, Y., Yáñez, J. A., Takemoto, J. K., Andrews, P. K., et al. (2009). Stereospecific high-performance liquid chromatography of taxifolin, applications in pharmacokinetics, and determination in tu fu ling (rhizoma smilacis glabrae) and apple (malus x domestica). Biomed. Chromatogr. 23, 638–646. doi:10.1002/bmc.1165
Vega-Villa, K. R., Remsberg, C. M., Takemoto, J. K., Ohgami, Y., Yáñez, J. A., Andrews, P. K., et al. (2011). Stereospecific pharmacokinetics of racemic homoeriodictyol, isosakuranetin, and taxifolin in rats and their disposition in fruit. Chirality 23, 339–348. doi:10.1002/chir.20926
Waltman, L., and van Eck, N. J. (2013). A smart local moving algorithm for large-scale modularity-based community detection. Eur. Phys. J. B 86, 471. doi:10.1140/epjb/e2013-40829-0
Wang, X., Zhou, H., and Zeng, S. (2012). Identification and assay of 3’-O-methyltaxifolin by UPLC-MS in rat plasma. J. Chromatogr. B Anal. Technol. Biomed. Life. Sci. 911, 34–42. doi:10.1016/j.jchromb.2012.09.006
Yang, F., Wen, H., Ma, S., Chang, Q., Pan, R., Liu, X., et al. (2023). Icaritin promotes myelination by simultaneously enhancing the proliferation and differentiation of oligodendrocyte precursor cells. Molecules 28, 5837. doi:10.3390/molecules28155837
Yoon, K. D., Lee, J.-Y., Kim, T. Y., Kang, H., Ha, K.-S., Ham, T.-H., et al. (2020). In vitro and in vivo anti-hyperglycemic activities of taxifolin and its derivatives isolated from pigmented rice (oryzae sativa L. Cv. Superhongmi). J. Agric. Food Chem. 68, 742–750. doi:10.1021/acs.jafc.9b04962
Yoshida, T., Zhe, X. J., and Okuda, T. (1989). Taxifolin apioside and davuriciin M1, a hydrolysable tannin from rosa davurica. Phytochemistry 28, 2177–2181. doi:10.1016/S0031-9422(00)97939-1
Zhu, Y., Scholle, F., Kisthardt, S. C., and Xie, D.-Y. (2022). Flavonols and dihydroflavonols inhibit the main protease activity of SARS-CoV-2 and the replication of human coronavirus 229E. Virology 571, 21–33. doi:10.1016/j.virol.2022.04.005
Keywords: dihydroquercetin, flavonoid, stereoisomerism, optical activity, NMR spectroscopy, HPLC, pharmacodynamics, pharmacokinetics
Citation: Terekhov RP, Savina AD, Pankov DI, Korochkina MD, Taldaev A, Yakubovich LM, Zavadskiy SP, Zhevlakova AK and Selivanova IA (2024) Insights into the stereoisomerism of dihydroquercetin: analytical and pharmacological aspects. Front. Chem. 12:1439167. doi: 10.3389/fchem.2024.1439167
Received: 27 May 2024; Accepted: 11 June 2024;
Published: 05 July 2024.
Edited by:
Ahmed A. Al-Karmalawy, Ahram Canadian University, EgyptReviewed by:
Maria Laura Uhrig, University of Buenos Aires, ArgentinaCopyright © 2024 Terekhov, Savina, Pankov, Korochkina, Taldaev, Yakubovich, Zavadskiy, Zhevlakova and Selivanova. This is an open-access article distributed under the terms of the Creative Commons Attribution License (CC BY). The use, distribution or reproduction in other forums is permitted, provided the original author(s) and the copyright owner(s) are credited and that the original publication in this journal is cited, in accordance with accepted academic practice. No use, distribution or reproduction is permitted which does not comply with these terms.
*Correspondence: Roman P. Terekhov, dGVyZWtob3Zfcl9wQHN0YWZmLnNlY2hlbm92LnJ1
Disclaimer: All claims expressed in this article are solely those of the authors and do not necessarily represent those of their affiliated organizations, or those of the publisher, the editors and the reviewers. Any product that may be evaluated in this article or claim that may be made by its manufacturer is not guaranteed or endorsed by the publisher.
Research integrity at Frontiers
Learn more about the work of our research integrity team to safeguard the quality of each article we publish.