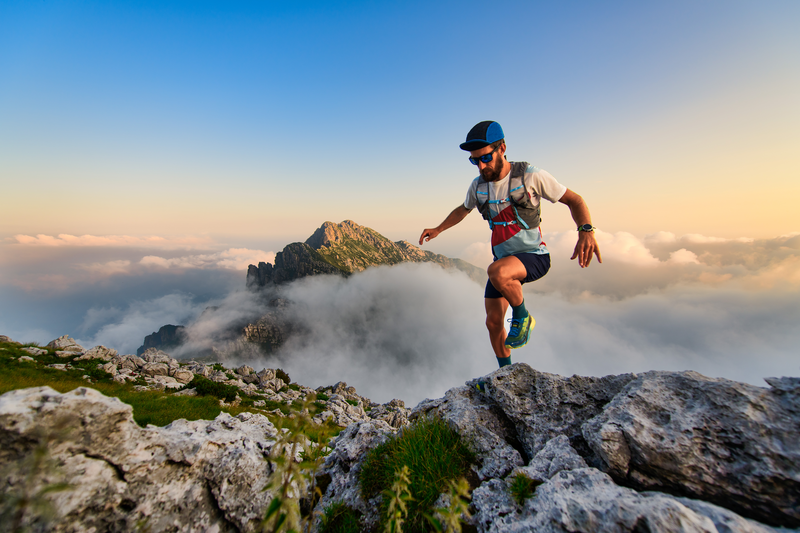
95% of researchers rate our articles as excellent or good
Learn more about the work of our research integrity team to safeguard the quality of each article we publish.
Find out more
ORIGINAL RESEARCH article
Front. Chem. , 02 August 2024
Sec. Green and Sustainable Chemistry
Volume 12 - 2024 | https://doi.org/10.3389/fchem.2024.1437277
The study aimed to extract and characterize natural fibers from Pulicaria gnaphalodes (Vent.) Boiss. plants and assess the impact of alkali treatment on the physicochemical and antioxidant properties of these fibers. Fibers were extracted from dried P. gnaphalodes aerial parts by grinding with an average yield of 18.1%. Physicochemical and FTIR analysis revealed that the hemicellulose was mostly lost during alkali treatment. Results of the X-ray diffraction and thermogravimetric analysis indicated that the crystallinity and thermal stability of P. gnaphalodes fibers were considerably increased after alkali treatment. In antioxidant activity assessment studies, raw fibers of P. gnaphalodes showed significantly higher radical scavenging and reducing power potentials compared to the alkali-treated samples, indicating that the majority of antioxidant components such as lignin and other polyphenols were lost from P. gnaphalodes fibers during alkali treatment. In conclusion, the promising antioxidant activity of raw P. gnaphalodes can be utilized in developing functional materials, particularly for cosmetic and wound healing applications.
Non-woody plant fibers are generally termed natural fibers, which are rich in cellulose and have good physicochemical properties and crystallinity (Gholampour and Ozbakkaloglu, 2020). Moreover, their affordability, environmental friendliness, and widespread availabilities make them popular materials in diverse industries including household goods, marine applications, and automotive sectors (Lokantara et al., 2020). Likewise, raw plant fibers such as sisal and ramie fibers are also found to be useful for biomedical purposes due to their biocompatibility and biological properties (Kandimalla et al., 2016; Guambo et al., 2020; Zamora-Mendoza et al., 2022). However, the use of plant fibers in their raw form has various limitations, as they possess high moisture absorption, poor durability, as well as low thermal stability and strength (Zamora-Mendoza et al., 2023). Hence, modifying surfaces of natural fibers is usually recommended to increase their crystallinity, hydrophobicity, mechanical, and thermal properties (Tavares et al., 2020).
Various physical, chemical, and biological methods have been employed to modify natural fiber surfaces (Cruz and Fangueiro, 2016). Physical treatments such as plasma, γ-ray treatments, and corona treatment reacts with surface functional groups of the fibers and modify them, thereby significantly improving the mechanical properties of natural fibers (Jagadeesh et al., 2021). Similarly, natural fibers have also been treated with various chemicals such as alkali, silane, water-repelling agents, peroxides, and permanganates to improve the crystallinity, hydrophobicity, mechanical, and thermal properties of natural fibers (Cruz and Fangueiro, 2016). Moreover, besides physical and chemical treatments, biological methods mainly using enzymes are also used to treat natural fibers (Koohestani et al., 2019). However, there is a need to understand the effect of these treatments on the surface, mechanical, and biological properties of different natural fibers.
Pulicaria gnaphalodes (Vent.) Boiss. is one of the commonly occurring Pulicaria species in Qatar, locally known as “Nufaij” (Abdel-Bari, 2012; Kasote et al., 2024). It is a perennial or subshrub that primarily grows in the temperate biome and is native to Iraq, Central Asia, the Western Himalayas, and the Arabian Peninsula (Kasote et al., 2024; POWO, 2024). However, P. gnaphalodes is reported to be a widely distributed species in the Persian region and is recognized as a medicinal plant (Hassanabadi et al., 2023). Traditionally, this plant is used as an herbal tea, including as a flavoring, antimicrobial, and anti-inflammatory agent (Kazemi et al., 2022). In recent studies, most of these traditional uses have been validated, and P. gnaphalodes has been reported to have promising antioxidant, antimicrobial, anticancer, antihypercholesterolemic, and anticonvulsant properties (Kamkar et al., 2013; Naqvi et al., 2020; Zadali et al., 2022; Pourhossein Alamdary et al., 2023). Flavonoids, terpenes (monoterpenes, sesquiterpenes, diterpenes, and triterpenes), and phenolic acids are the main reported bioactive phytochemicals in P. gnaphalodes (Kasote et al., 2024). As previously reported, Pulicaria undulata (L.) CA Mey. is known to be rich in fiber (Fahmi et al., 2019). We also found that the aerial part of P. gnaphalodes is rich in fibers. However, fibers from P. gnaphalodes have not yet been extracted and characterized, and their potential bioactivity also remains unexplored.
Herein, we extracted fibers from P. gnaphalodes for the first time and studied their physicochemical, thermal, and surface properties. Similarly, the effect of alkali treatment on the matrix and surface of P. gnaphalodes fibers was also investigated. Moreover, the antioxidant potential of both raw and alkali-treated fiber samples of P. gnaphalodes was evaluated to understand their potential wound healing and cosmetic applications.
Plants were harvested from Ash Shamal, Qatar. The aerial parts were cleaned, separated from the roots, and dried at 50°C for 72 h in a hot air oven to extract fibers. ABTS [2,2′-azinobis (3-ethylbenzothiazoline-6-sulfonic acid)] was purchased from Sigma-Aldrich, China. All other chemicals used, including potassium permanganate, potassium persulfate, sodium hydroxide, acetone, ethanol (95% pure), sulfuric acid, and ammonium oxalate, were of analytical grade.
Fibers from P. gnaphalodes were extracted by grinding the dried aerial parts in a home-use grinder. The workflow of fiber extraction from P. gnaphalodes is summarized in Figure 1. The fibers were manually cleaned before conducting physicochemical analysis and characterization studies. The fiber yield was then measured.
An aliquot of 20 mL of 0.2N NaOH (5%) was added to the Falcon tube containing around 1 g of raw fiber sample and the tube was kept in a boiling water bath for 90 min. The tube was cooled for 1 h before neutralizing the reaction mixture with a 1% (w/v) HCl solution. The fiber sample was then washed with distilled water after centrifugation at 5,000 rpm for 5 min. This washing process was repeated two to three times until the fiber sample was neutralized. The neutralized fiber sample was dried in an air oven at 105°C for 24 h and stored in Ziploc bags until further analysis (Ilangovan et al., 2018; Senthamaraikannan and Saravanakumar, 2023).
In the physical analysis, the diameters of the raw and alkali-treated fibers were measured using scanning electron microscopy (SEM). The diameter of each 3–6 single fiber was measured at 3–4 locations on each fiber, and the average value was recorded for each fiber.
Cellulose, hemicelluloses, lignin, extractives, wax, ash, and moisture content in raw and alkali-treated fibers were determined using different methods. The moisture content was initially determined using a method described by Ngoup et al. (2024). Ash content in fiber samples was determined using the ASTM E1755-01 Method (Kulandaivel et al., 2018). To estimate the wax content, 1 g ± 0.1 g of both raw and treated fiber samples (n = 3) were placed in 50 mL of hot ethanol for 3 h. After extraction, the samples were dried overnight at 105°C and reweighed. The wax content was estimated based on weight loss (Thomsen et al., 2005). The lignin content of raw and treated fibers was measured using the gravimetric method (Valls Calm, 2017). Dewaxed fiber samples (100 ± 0.1 mg, n = 3) were placed in Falcon tubes and treated with 3.0 mL of 72% sulfuric acid for 24 h. The samples were then diluted with 18 mL of water and hydrolyzed for an additional 5 h. The lignin content was determined gravimetrically after the hydrolysis process (Valls Calm, 2017). To determine the extractives content, 20 mL was added to dewaxed samples (200 ± 0.1 mg) and kept in a water bath at 56°C for 1 h. After centrifugation, the acetone extract was separated, and the acetone was allowed to evaporate. The weight of the remaining residue was then measured to calculate the percentage of extractives content based on the weight loss (Kale et al., 2018). The pectin content was estimated using the dried residues of fiber samples after extractives removal. An aliquot of 20 mL aliquot of a 0.5% solution of ammonium oxalate was added to the fiber residues and heated in a boiling water bath for 5 h. The supernatant was then separated, dried, and weighed after cooling, and the percentage of pectin was calculated based on the weight loss (Whistler et al., 1940). The remaining residue was used for the estimation of hemicellulose and cellulose after weighing. To estimate hemicellulose, 10 mL of 0.5N NaOH was added to the tube containing the fiber sample residues left after pectin isolation, and these tubes were placed in a boiling water bath for 3 h. Following centrifugation (5,000 rpm, 5 min), the residue was collected, washed with water, and then dried in the oven. The weight of this residue was measured, and the weight percentage was calculated as the hemicellulose content (Cakmak and Dekker, 2022). Finally, the cellulose content (%) was calculated using the formula “100-%wax-%extractives-%pectin-%hemicellulose-%lignin” (Alsafran et al., 2024).
FTIR analysis was conducted to identify the functional groups in the fiber samples. The raw and alkali-treated fibers were powdered, mixed with KBr, and pressed into pellets. Spectra were obtained using a Perkin Elmer Spectrometer in the range of 4,000–500 cm−1.
The PANalytical Empyrean X-ray diffractometer (Malvern Panalytical B.V., Brighton, United Kingdom; Cu Kα = 1.5404 Å) was used to estimate the crystallinity index of raw and alkali-treated fiber samples. Scans were measured at 2θ in the range of 10°–80° with an accuracy of 0.02.
Thermal stability of raw and alkali-treated fiber samples was measured using thermogravimetric analysis (PerkinElmer, Pyris 6, United States). Fiber samples were placed in an alumina crucible and then kept in the furnace with a controlled environment of nitrogen flow rate of 20 mL/min. The temperature of the chamber was increased from room temperature to 600°C at a rate of 10°C/min.
The surface topography and diameters of raw and alkali-treated fibers were analyzed using SEM. The SEM images and energy dispersive X-ray spectroscopy (EDX) data were obtained with a JCM 6000 SEM.
The antioxidant and reducing power properties of raw and alkali-treated fiber samples were evaluated using modified ABTS and potassium permanganate (KMnO4) reduction (PPR) assays described by Kasote et al. (2019). In the ABTS assay, 7.4 mM ABTS and 2.6 mM potassium persulfate solutions were prepared and mixed in equal amounts (v/v) and left to react overnight in the dark. The next day, the reagent was diluted with water (1:40, v/v) before use. Fiber samples (10 ± 0.1 mg, n = 4) were placed in 24-well plates, and 1 mL of the reagent solution was added to each well, including control wells. The plate was incubated for 5 min in the dark. Finally, fibers were removed, and absorbance was measured at 730 nm using a microplate reader. The percentage of ABTS radical scavenging activity was calculated for both raw and treated fiber samples (Kasote et al., 2019). Similarly, for the PPR assay, A 0.125 mM KMnO4 solution was prepared freshly. Then, 1 mL of this solution was added to wells containing control and fiber samples (n = 4) in a microtiter plate and incubated for 5 min. The fibers were then removed, and the absorbance of the plate was measured at 525 nm using a microplate reader. The results were calculated as % PPR activity for both raw and alkali-treated fiber samples (Kasote et al., 2019).
All assays were performed using sufficient replicates. Data were expressed as mean ± SD. Microsoft Excel was used for statistical analysis and data visualization.
In this study, raw fibers were extracted from dried P. gnaphalodes aerial part by grinding. The yield of fiber obtained was 18.1% ± 2.5%. Later, the effect of alkali treatment (5%) on the physicochemical properties of P. gnaphalodes fibers was investigated. The average diameter of raw P. gnaphalodes fibers was observed to be 4.68 ± 0.42 μm, which decreased to 4.15 ± 0.40 μm after alkali treatment (Supplementary Figure S1). This reduction is primarily attributed to the removal of impurities, wax, and extractives from the fiber surface (Pokhriyal et al., 2023). The results chemical composition analysis of raw and alkali-treated P. gnaphalodes fibers are documented in Table 1. The composition of natural fibers varies according to their botanical origins, climate, maturity, and extraction method (Chaudhary and Ahmad, 2020). The observed cellulose, hemicellulose, and lignin content of raw P. gnaphalodes fibers were similar to that of bamboo, ost, rye, and sugar fibers (Bakar et al., 2020; Karimah et al., 2021). Alkali treatment significantly removed wax, extractives, and hemicellulose from raw fiber. The observed fold loss of hemicellulose, wax, and extractives was 3.9, 4.2, and 9.6 times, respectively. The cellulose content increased by 91.8% after alkali treatment.
The results of FT-IR analysis of raw and alkali-treated P. gnaphalodes fibers are shown in Figure 2. The peak observed around 3,324 cm−1 in raw and alkali-treated P. gnaphalodes fibers was associated with the stretching vibration of OH groups and inter- and intra-molecular hydrogen bond vibrations. This peak was attributed to the presence of α-cellulose and water molecules due to the moisture content (Merlini et al., 2011; George et al., 2020). Furthermore, the peaks at 2,918 and 2,850 cm−1 correspond to the stretching vibrations of C-H bonds in alkanes found in Cellulose I or α-cellulose (Pradhan et al., 2023). The peak at 1715 cm−1 corresponds to the C=O stretching vibration in lignin and hemicellulose (Maran et al., 2022). Similarly, the C=O stretching vibration associated with the lignin and hemicellulose components was also confirmed by the peak observed at around 1,608 cm−1 (George et al., 2020; Ravindran et al., 2020). The peak at 1,242 cm−1 was associated with the C–O stretching vibration of the acetyl group in lignin and hemicellulose (Ponnu Krishnan and Selwin Rajadurai, 2017). However, this peak was almost non-visible in alkali-treated fiber samples, indicating the removal of hemicellulose and lignin from P. gnaphalodes fibers during alkali treatment, as reported in previous studies (Kamaruddin et al., 2022). Furthermore, a strong peak at 1,031 cm−1 indicated the presence of lignin (C–OH stretching) in the fiber (Senthamaraikannan and Kathiresan, 2018). As seen in the chemical composition analysis, the findings of the FTIR analysis clearly show that a significant amount of hemicellulose, lignin, and other components are removed from the surface of P. gnaphalodes fibers during alkali treatment.
The XRD patterns of the raw and alkali-treated fibers of P. gnaphalodes are shown in Figure 3. Both raw and alkali-treated fiber samples showed two intense peaks at around 2θ values at 15° and 22°, corresponding to the (110) and (200) planes, respectively. These two peaks at 2θ = 15° and 22° represent the amorphous and crystalline constituents of the fiber, respectively (Rathinavelu and Paramathma, 2023). After the alkali treatment, an increase in the intensities of both peaks was observed, indicating an increase in the crystalline fraction in the alkali-treated fibers. The Crystallinity Index (CI) is a quantitative indicator of crystallinity (Sa et al., 2017).
In general, fibers with a higher CI value may have better mechanical properties (Ravindran et al., 2020). The observed CI of the raw and alkali-treated fibers of P. gnaphalodes was 67.0% and 79.2%, respectively, indicating raw fiber had good crystallinity that enhanced considerably with alkali-treatment. This indicates that the raw fiber had good crystallinity, which significantly improved with alkali treatment.
The TGA and derivative thermogravimetric (DTG) curves of raw and alkali-treated fibers of P. gnaphalodes are shown in Figure 4. In general, studying the thermal degradation properties of natural fibers is crucial for high-temperature applications (Vijay et al., 2019). Both raw and treated samples were found to show weight loss in phases. In the phase (up to 150°C) both raw and alkali-treated fibers showed a small amount of weight loss due to the removal of moisture content in the sample (Borchani et al., 2015). At 150°C, raw and alkali-treated fibers were found to exhibit weight losses of up to 8.14% and 5.58%, respectively, due to their differential moisture content. Next phase, raw (200°C–400°C) and alkali-treated (250°C–400°C) fibers showed maximum weight loss due to the degradation of fiber components such as pectin, hemicellulose, and cellulose (Raia et al., 2021). Typically, lignocellulosic fibers degrade at around 240°C (Mahmud et al., 2021). The observed maximum fiber degradation temperatures for raw and alkali-treated fibers were 338.8°C and 351.0°C, respectively (Figure 4A). This indicates that raw P. gnaphalodes fibers have good thermal stability that can be further increased with alkali treatment. In general, alkaline treatment is also used to improve the thermal stability of natural fibers (Elfaleh et al., 2023). Lignins in plant fibers are difficult to degrade, so they begin to break down in the late phase (up to 351.0°C), leaving behind residue (Loganathan et al., 2020). At the end of the experiment, we found that the raw fibers (23.5%) left more residual mass than the alkali-treated fibers (16.5%). This could be due to their high lignin content, including wax.
Figure 5 shows the surface morphology of raw and alkali-treated fibers of P. gnaphalodes. The fiber of P. gnaphalodes is comprised of a series of parallel microfibrils. However, the surface of the raw fiber was found to be rough and had impurities and wax on it (Figure 5A). After the alkali treatment, impurities, wax, and lignin present on the surface were found to be washed out. Alkali-treated fiber had a smooth surface and clearly visible microfibrils (Figure 5B). This fact was also evidenced by the EDX spectra, which showed a loss of Si, Cl, and Mg contents after alkali treatment (Supplementary Figure S2). These observations collectively indicate that alkali-treated P. gnaphalodes fibers can be an appropriate material for making lightweight fiber-reinforced composites.
Figure 5. Scanning electron microscopy (SEM) micrographs of (A) raw and (B) alkali-treated P. gnaphalodes fibers.
The results of free radical scavenging and reducing power potentials of the raw and alkali-treated fibers of P. gnaphalodes in ABTS and PPR assays are shown in Figure 6. In both assays, the raw fibers of P. gnaphalodes showed significantly higher radical scavenging and reducing power potentials compared to the alkali-treated fibers, indicating that the majority of antioxidant components such as lignins and other polyphenols were lost from P. gnaphalodes fibers during alkali treatment. We found a nearly 7.3-fold decrease in radical scavenging activity and a 6-fold decrease in reducing power potential in ABTS and PPR assays after alkali treatment, respectively. So far, antioxidant activity has been reported in the flax, hemp and colored cotton fibers (Ma et al., 2016; Zamora-Mendoza et al., 2022; Zamora-Mendoza et al., 2023). These natural fibers with antioxidant properties can function as anti-inflammatory, wound healing, antitumor, and anti-aging agents, as well as prolonging the shelf-life of products (Pedro et al., 2022). These findings about the free radical scavenging and reducing power potentials of raw P. gnaphalodes can be utilized in developing functional materials, particularly for cosmetic and wound healing applications.
Figure 6. Antioxidant activity of the raw and the alkali-treated fibers of P. gnaphalodes in (A) ABTS and (B) PPR assays.
In the present study, raw fibers from dried P. gnaphalodes aerial parts were extracted by grinding with an average yield of 18.1%. The raw fiber of P. gnaphalodes was found to have an average diameter of 4.68 μm, mainly composed of cellulose (35.7%), lignin (24.5%), hemicellulose (22.7%), extractives (6.73%), pectin (5.64%), and wax (4.65%). Furthermore, the effect of 5% alkali treatment on the physicochemical, thermal, morphological, and antioxidant properties was studied. These findings showed that alkali treatment effectively extracted most of the hemicellulose, including lignin, pectin, wax, and extractives, and increased the cellulose content in the fibers. This was further evident from the increased thermal stability of alkali-treated fibers. In antioxidant activity assessment studies, raw fibers of P. gnaphalodes showed significantly higher radical scavenging and reducing power potentials compared to the alkali-treated fibers, indicating that the majority of antioxidant components such as lignins and other polyphenols were lost from P. gnaphalodes fibers during alkali treatment. Altogether, Overall, this study highlighted that alkali treatment enhanced mechanical and thermal characteristics, including crystallinity. However, the antioxidant activity of raw P. gnaphalodes was considerably reduced as a result of this treatment. Moreover, observed promising free radical scavenging and reducing power potentials of raw P. gnaphalodes can be utilized in developing functional materials, particularly for cosmetic and wound healing applications.
The original contributions presented in the study are included in the article/Supplementary Material, further inquiries can be directed to the corresponding author.
MA: Formal Analysis, Funding acquisition, Project administration, Resources, Supervision, Writing–review and editing. KS: Data curation, Methodology, Supervision, Visualization, Writing–review and editing. JH: Formal Analysis, Methodology, Visualization, Writing–review and editing. DK: Conceptualization, Data curation, Formal Analysis, Investigation, Methodology, Supervision, Writing–original draft.
The authors declare that financial support was received from the Agricultural Research Station (ARS), Qatar University (QU) for the research, authorship, and/or publication of this article. Open Access funding provided by the ARS, QU is greatly appreciated.
The authors would like to thank the Directors and technical staff of the Central Laboratories Unit (CLU) and Center for Advanced Materials (CAM) for their assistance with SEM, XRD, FTIR, and TGA.
The authors declare that the research was conducted in the absence of any commercial or financial relationships that could be construed as a potential conflict of interest.
All claims expressed in this article are solely those of the authors and do not necessarily represent those of their affiliated organizations, or those of the publisher, the editors and the reviewers. Any product that may be evaluated in this article, or claim that may be made by its manufacturer, is not guaranteed or endorsed by the publisher.
The Supplementary Material for this article can be found online at: https://www.frontiersin.org/articles/10.3389/fchem.2024.1437277/full#supplementary-material
Abdel-Bari, E. (2012). The flora of Qatar, the dicotyledons, volume 2 the monocotyledons. Doha, Qatar: Environmental Studies Center Qatar University, 1–2.
Alsafran, M., Sadasivuni, K. K., Haneesh, J. M., Razavi, M. M., and Kasote, D. M. (2024). Extraction and characterization of biofunctional lignocellulosic fibers from Pulicaria undulata plant and the effect of alkali treatment on their bio-physicochemical properties. Carbohydr. Polym. Technol. Appl., 100542. doi:10.1016/j.carpta.2024.100542
Bakar, N., Chin, S., Siregar, J., and Ngien, S. (2020). “A review on physical, mechanical, thermal properties and chemical composition of plant fibers,” in IOP conference series: materials science and engineering (IOP Publishing).052017.
Borchani, K. E., Carrot, C., and Jaziri, M. (2015). Untreated and alkali treated fibers from Alfa stem: effect of alkali treatment on structural, morphological and thermal features. Cellulose 22, 1577–1589. doi:10.1007/s10570-015-0583-5
Cakmak, H., and Dekker, M. (2022). Optimization of cellulosic fiber extraction from parsley stalks and utilization as filler in composite biobased films. Foods 11, 3932. doi:10.3390/foods11233932
Chaudhary, V., and Ahmad, F. (2020). A review on plant fiber reinforced thermoset polymers for structural and frictional composites. Polym. Test. 91, 106792. doi:10.1016/j.polymertesting.2020.106792
Cruz, J., and Fangueiro, R. (2016). Surface modification of natural fibers: a review. Procedia Eng. 155, 285–288. doi:10.1016/j.proeng.2016.08.030
Elfaleh, I., Abbassi, F., Habibi, M., Ahmad, F., Guedri, M., Nasri, M., et al. (2023). A comprehensive review of natural fibers and their composites: an eco-friendly alternative to conventional materials. Results Eng. 19, 101271. doi:10.1016/j.rineng.2023.101271
Fahmi, A. A., Abdur-Rahman, M., Naser, A. F. A., Hamed, M. A., Abd-Alla, H. I., Shalaby, N. M., et al. (2019). Chemical composition and protective role of Pulicaria Undulata (L.) CA Mey. subsp. Undulata against gastric ulcer induced by ethanol in rats. Heliyon 5, e01359. doi:10.1016/j.heliyon.2019.e01359
George, N., Andersson, A. A., Andersson, R., and Kamal-Eldin, A. (2020). Lignin is the main determinant of total dietary fiber differences between date fruit (Phoenix Dactylifera L.) varieties. NFS J. 21, 16–21. doi:10.1016/j.nfs.2020.08.002
Gholampour, A., and Ozbakkaloglu, T. (2020). A review of natural fiber composites: properties, modification and processing techniques, characterization, applications. J. Mater. Sci. 55, 829–892. doi:10.1007/s10853-019-03990-y
Guambo, M. P. R., Spencer, L., Vispo, N. S., Vizuete, K., Debut, A., Whitehead, D. C., et al. (2020). Natural cellulose fibers for surgical suture applications. Polymers 12, 3042. doi:10.3390/polym12123042
Hassanabadi, Z., Mohayeji, M., Sharififar, F., Mirtadzadini, M., and Mehrafarin, A. (2023). Evaluation of morphologic and genetic diversity of Pulicaria Gnaphalodes populations collected from south-east of Iran. Agric. Biotechnol. J. 15, 145–166. doi:10.22103/jab.2023.20590.1445
Ilangovan, M., Guna, V., Hu, C., Nagananda, G., and Reddy, N. (2018). Curcuma longa L. plant residue as a source for natural cellulose fibers with antimicrobial activity. Ind. Crops Prod. 112, 556–560. doi:10.1016/j.indcrop.2017.12.042
Jagadeesh, P., Puttegowda, M., Mavinkere Rangappa, S., and Siengchin, S. (2021). A review on extraction, chemical treatment, characterization of natural fibers and its composites for potential applications. Polym. Compos. 42, 6239–6264. doi:10.1002/pc.26312
Kale, R. D., Alemayehu, T. G., and Gorade, V. G. (2018). Extraction and characterization of lignocellulosic fibers from Girardinia Bullosa (Steudel) wedd (Ethiopian Kusha plant). J. Nat. Fibers. 17, 906–920. doi:10.1080/15440478.2018.1539940
Kamaruddin, Z. H., Jumaidin, R., Ilyas, R. A., Selamat, M. Z., Alamjuri, R. H., and Yusof, F. M. (2022). Influence of alkali treatment on the mechanical, thermal, water absorption, and biodegradation properties of Cymbopogan Citratus fiber-reinforced, thermoplastic cassava starch–palm wax composites. Polymers 14, 2769. doi:10.3390/polym14142769
Kamkar, A., Ardekani, M. R. S., Shariatifar, N., Misagi, A., Nejad, A. S. M., and Jamshidi, A. H. (2013). Antioxidative effect of Iranian Pulicaria Gnaphalodes L. extracts in soybean oil. S. Afr. J. Bot. 85, 39–43. doi:10.1016/j.sajb.2012.12.001
Kandimalla, R., Kalita, S., Choudhury, B., Devi, D., Kalita, D., Kalita, K., et al. (2016). Fiber from ramie plant (Boehmeria Nivea): a novel suture biomaterial. Mater. Sci. Eng. C 62, 816–822. doi:10.1016/j.msec.2016.02.040
Karimah, A., Ridho, M. R., Munawar, S. S., Adi, D. S., Damayanti, R., Subiyanto, B., et al. (2021). A review on natural fibers for development of eco-friendly bio-composite: characteristics, and utilizations. J. Mater. Res. Technol. 13, 2442–2458. doi:10.1016/j.jmrt.2021.06.014
Kasote, D. M., Jayaprakasha, G. K., and Patil, B. S. (2019). Leaf disc assays for rapid measurement of antioxidant activity. Sci. Rep. 9, 1884. doi:10.1038/s41598-018-38036-x
Kasote, D. M., Nawaz, M. A., Usman, K., Ullah, N., and Alsafran, M. (2024). A critical review on Pulicaria species occurring in Qatar: traditional uses, phytochemistry and biological activities. Phytochem. Rev., 1–52. doi:10.1007/s11101-024-09932-0
Kazemi, M., Ibrahimi Khoram Abadi, E., and Tohidi, R. (2022). Nutritional characteristics of some medicinal-range plant species grazed by small ruminants in Torbat-e Jam region of Iran. J. Rangel. Sci. 13, 1–8. doi:10.57647/j.jrs.2023.1304.33
Koohestani, B., Darban, A., Mokhtari, P., Yilmaz, E., and Darezereshki, E. (2019). Comparison of different natural fiber treatments: a literature review. Int. J. Environ. Sci. Tech. 16, 629–642. doi:10.1007/s13762-018-1890-9
Kulandaivel, N., Muralikannan, R., and Kalyanasundaram, S. (2018). Extraction and characterization of novel natural cellulosic fibers from pigeon pea plant. J. Nat. Fibers. 17, 769–779. doi:10.1080/15440478.2018.1534184
Loganathan, T. M., Sultan, M. T. H., Ahsan, Q., Jawaid, M., Naveen, J., Shah, A. U. M., et al. (2020). Characterization of alkali treated new cellulosic fibre from Cyrtostachys Renda. J. Mater. Res. Technol. 9, 3537–3546. doi:10.1016/j.jmrt.2020.01.091
Lokantara, I., Suardana, N., Surata, I., and Winaya, I. (2020). A review on natural fibers: Extraction process and properties of grass fibers. Int. J. Mech. Eng. Technol. 1, 84–91. doi:10.34218/IJMET.11.1.2020.009
Ma, M., Luo, S., Hu, Z., Tang, Z., and Zhou, W. (2016). Antioxidant properties of naturally brown-colored cotton fibers. Text. Res. J. 86, 256–263. doi:10.1177/0040517515588270
Mahmud, S., Hasan, K. F., Jahid, M. A., Mohiuddin, K., Zhang, R., and Zhu, J. (2021). Comprehensive review on plant fiber-reinforced polymeric biocomposites. J. Mater. Sci. 56, 7231–7264. doi:10.1007/s10853-021-05774-9
Maran, M., Kumar, R., Senthamaraikannan, P., Saravanakumar, S., Nagarajan, S., Sanjay, M., et al. (2022). Suitability evaluation of Sida mysorensis plant fiber as reinforcement in polymer composite. J. Nat. Fibers 19, 1659–1669. doi:10.1080/15440478.2020.1787920
Merlini, C., Soldi, V., and Barra, G. M. (2011). Influence of fiber surface treatment and length on physico-chemical properties of short random banana fiber-reinforced castor oil polyurethane composites. Polym. Test. 30, 833–840. doi:10.1016/j.polymertesting.2011.08.008
Naqvi, S. A. R., Shah, S. M. A., Kanwal, L., Saeed, M., Atta-Ul-Haq, , Nisar, J., et al. (2020). Antimicrobial and antihypercholesterolemic activities of pulicaria gnaphalodes. Dose-Response 18, 155932582090485. doi:10.1177/1559325820904858
Ngoup, T., Efeze, N. D., Kanaa, T., Mbang, J. P. E., Segovia, C., Nga, N., et al. (2024). Physical, chemical and mechanical characterization of Sida Rhombifolia fibers from the center region of Cameroon for their potential use in textiles and composites. J. Nat. Fibers 21, 2294478. doi:10.1080/15440478.2023.2294478
Pedro, A. C., Paniz, O. G., Fernandes, I. D. a.A., Bortolini, D. G., Rubio, F. T. V., Haminiuk, C. W. I., et al. (2022). The importance of antioxidant biomaterials in human health and technological innovation: a review. Antioxidants 11, 1644. doi:10.3390/antiox11091644
Pokhriyal, M., Rakesh, P. K., Rangappa, S. M., and Siengchin, S. (2023). Effect of alkali treatment on novel natural fiber extracted from Himalayacalamus falconeri culms for polymer composite applications. Biomass Convers. Biorefin., 1–17. doi:10.1007/s13399-023-03843-4
Ponnu Krishnan, P., and Selwin Rajadurai, J. (2017). Microscopical, physico-chemical, mineralogical, and mechanical characterization of Sansevieria zeylanica fibers as potential reinforcement of composite structures. J. Compos. Mater. 51, 811–829. doi:10.1177/0021998316653461
Pourhossein Alamdary, M., Baharfar, R., and Tavakoli, S. (2023). Isolation of secondary metabolites from Pulicaria gnaphalodes (vent.) Boiss. And evaluation of their anti-proliferative activity. Polycycl. Aromat. Comp. 43, 8307–8318. doi:10.1080/10406638.2022.2149933
Pradhan, R., Palai, B. K., Thatoi, D. N., Elayaperumal, A., and Nalla, J. S. (2023). Characterization of novel cellulosic fibers extracted from Hibiscus canescens stem. Biomass Convers. Biorefin., 1–16. doi:10.1007/s13399-023-04645-4
Raia, R. Z., Iwakiri, S., Trianoski, R., Andrade, A. S. D., and Kowalski, E. L. (2021). Effects of alkali treatment on modification of the Pinus fibers. Materia-Brazil 26, e12936. doi:10.1590/S1517-707620210001.1236
Rathinavelu, R., and Paramathma, B. S. (2023). Examination of characteristic features of raw and alkali-treated cellulosic plant fibers from Ventilago maderaspatana for composite reinforcement. Biomass Convers. Biorefin. 13, 4413–4425. doi:10.1007/s13399-022-03461-6
Ravindran, D., Sr, S. B., Padma, S., Indran, S., and Divya, D. (2020). Characterization of natural cellulosic fiber extracted from Grewia Damine flowering plant’s stem. Int. J. Biol. Macromol. 164, 1246–1255. doi:10.1016/j.ijbiomac.2020.07.225
Sa, Y., Guo, Y., Feng, X., Wang, M., Li, P., Gao, Y., et al. (2017). Are different crystallinity-index-calculating methods of hydroxyapatite efficient and consistent? New J. Chem. 41, 5723–5731. doi:10.1039/C7NJ00803A
Senthamaraikannan, P., and Kathiresan, M. (2018). Characterization of raw and alkali treated new natural cellulosic fiber from Coccinia Grandis. L. Carbohydr. Polym. 186, 332–343. doi:10.1016/j.carbpol.2018.01.072
Senthamaraikannan, P., and Saravanakumar, S. (2023). Evaluation of characteristic features of untreated and alkali-treated cellulosic plant fibers from Mucuna Atropurpurea for polymer composite reinforcement. Biomass Convers. Biorefin. 13, 11295–11309. doi:10.1007/s13399-022-03736-y
Tavares, T. D., Antunes, J. C., Ferreira, F., and Felgueiras, H. P. (2020). Biofunctionalization of natural fiber-reinforced biocomposites for biomedical applications. Biomolecules 10, 148. doi:10.3390/biom10010148
Thomsen, A. B., Rasmussen, S., Bohn, V., Nielsen, K. V., and Thygesen, A. (2005). Hemp raw materiala: the effect of cultivar, growth conditions and pretreatment on the chemical composition of the fibres. Denmark: Risø DTU-National Laboratory for Sustainable Energy Roskilde.
Valls Calm, G. (2017). Study of the influence of different enzymatic treatments of flax on fiber composition.
Vijay, R., Singaravelu, D. L., Vinod, A., Sanjay, M., Siengchin, S., Jawaid, M., et al. (2019). Characterization of raw and alkali treated new natural cellulosic fibers from Tridax Procumbens. Int. J. Biol. Macromol. 125, 99–108. doi:10.1016/j.ijbiomac.2018.12.056
Whistler, R. L., Martin, A. R., and Harris, M. (1940). Pectic substance in cotton and its relation to the properties of the fibre. Tex. Res. 10, 269–279. doi:10.1177/004051754001000702
Zadali, R., Baghery, M., Abbasi, M., Yavari, N., Miran, M., and Ebrahimi, S. N. (2022). Anticonvulsant activity of Iranian medicinal plants and molecular docking studies of isolated phytochemicals. S. Afr. J. Bot. 149, 646–657. doi:10.1016/j.sajb.2022.06.044
Zamora-Mendoza, L., Guamba, E., Miño, K., Romero, M. P., Levoyer, A., Alvarez-Barreto, J. F., et al. (2022). Antimicrobial properties of plant fibers. Molecules 27, 7999. doi:10.3390/molecules27227999
Keywords: Pulicaria gnaphalodes, natural fiber, alkali treatment, antioxidant activity, lignocellulosic
Citation: Alsafran M, Sadasivuni KK, Haneesh JM and Kasote DM (2024) Extraction and characterization of natural fibers from Pulicaria gnaphalodes plant and effect of alkali treatment on their physicochemical and antioxidant properties. Front. Chem. 12:1437277. doi: 10.3389/fchem.2024.1437277
Received: 23 May 2024; Accepted: 16 July 2024;
Published: 02 August 2024.
Edited by:
Djalal Trache, Ecole Militaire Polytechnique (EMP), AlgeriaReviewed by:
Ahmad Adlie Shamsuri, Putra Malaysia University, MalaysiaCopyright © 2024 Alsafran, Sadasivuni, Haneesh and Kasote. This is an open-access article distributed under the terms of the Creative Commons Attribution License (CC BY). The use, distribution or reproduction in other forums is permitted, provided the original author(s) and the copyright owner(s) are credited and that the original publication in this journal is cited, in accordance with accepted academic practice. No use, distribution or reproduction is permitted which does not comply with these terms.
*Correspondence: Deepak M. Kasote, ZC5rYXNvdGVAcXUuZWR1LnFh
Disclaimer: All claims expressed in this article are solely those of the authors and do not necessarily represent those of their affiliated organizations, or those of the publisher, the editors and the reviewers. Any product that may be evaluated in this article or claim that may be made by its manufacturer is not guaranteed or endorsed by the publisher.
Research integrity at Frontiers
Learn more about the work of our research integrity team to safeguard the quality of each article we publish.