- 1Department of Biotechnology, Khalsa College, Amritsar, India
- 2Department of Biotechnology, Guru Nanak Dev University, Amritsar, India
Crop growth and yield are negatively impacted by increased biotic stress in the agricultural sector due to increasing global warming and changing climatic patterns. The host plant’s machinery is exploited by biotic stress, which is caused by organisms like bacteria, fungi, viruses, insects, nematodes, and mites. This results in nutrient deprivation, increased reactive oxygen species and disturbances in physiological, morphological, and molecular processes. Although used widely, conventional disease management strategies like breeding, intercropping, and chemical fertilizers have drawbacks in terms of time commitment and environmental impact. An environmentally beneficial substitute is offered by the developing field of nanotechnology, where nanoparticles such as zinc oxide are gaining popularity due to their potential applications as antimicrobials and nano-fertilizers. This review delves into the biological synthesis of ZnO nanoparticles employing plants and microbes, function of ZnO nanoparticles in biotic stress mitigation, elucidating their effectiveness and toxicological implications in agricultural. This study supports a cautious approach, stressing the prudent application of ZnO nanoparticles to avoid possible toxicity, in line with the larger global agenda to end hunger, guarantee food security, and advance sustainable agriculture.
1 Introduction
Extensive global warming and change in climate patterns increase the incidences of biotic stress in crops, which negatively impact their growth and yield. The term “biotic stress” describes the detrimental effect of bacteria, fungi, viruses, insects, nematodes, and mites, etc. on the plant growth, development, and yield (Pandey et al., 2017; Masri and Kiss, 2023). These agents generally utilized the host plant machinery for their growth and survival, which in turn causes nutrients deprivation, increases ROS, disturbs the physiological, morphological and molecular functioning of the plants and ultimately lead to their death (Gull et al., 2019; Chaudhary et al., 2022). Moreover, during the postharvest processing of grains and fruits, pathogenic infections in the plants are the primary source of significant economic loss. The projected yearly loss of crop yield due to pests and pathogens is estimated as US$220billion (Singh et al., 2023). By 2050, crop productivity is expected to rise by 60%–100%. In order to feed the world’s anticipated 9.7 billion people different disease management techniques like breeding, intercropping, development of stress resilient varieties, and usage of chemical fertilizers are widely employed to mitigate the biotic stress (Lau et al., 2022; Mohan et al., 2023). However, these approaches are time-consuming and extensive application of chemical pesticides, insecticides and herbicides increase the problem of pathogens resistance, soil degradation and also effects human health (Lau et al., 2022). The United Nations has embraced a mandate encompassing 17 Sustainable Development Goals (SDGs) directed towards fostering global sustainable development, with a fundamental aim to ensure worldwide peace, prosperity, and advancement. Goal 2 of this collective initiative seeks to eliminate hunger, establish food security, enhance nutritional standards, and advocate for sustainable agriculture practices (https://sdgs.un.org/goals).
Nanotechnology branch has emerged as an ecologically friendly alternative for conventional agricultural chemicals/pesticides/herbicides (Hajong et al., 2019; Khan et al., 2021a; Shams et al., 2023). Different nanoparticles like silver, copper oxide, silicon oxide, nano-calcite, magnesium oxide, zinc oxide, and titanium dioxide, etc. have been reported as potential nano-fertilizers, antibacterial, and antifungal nanomaterials (Khan et al., 2021b; Mohan et al., 2023; Tortella et al., 2023). The main mechanism by which these nanoparticles kills the pathogenic microorganisms includes inhibition of biofilm, cell membrane damage, formation of reactive oxygen species (ROS) and reactive nitrogen species (RNS) free radicals (peroxides, superoxide, hydroperoxyl, hydroxyl radical, singlet oxygen, nitric oxide, peroxynitrite). Once the ROS and RNS get accumulated in the microbial cell it will damage the structure and functioning of biological macromolecules like proteins, nucleic acids and lipids (Alavi and Yarani, 2023). In the era of green technology, nanoparticles as nano-fertilizers are an emerging field. The patent analysis data by selecting “Any field” in “Field” option and using “Nanofertilizers” keywords (https://patentscope.wipo.int/search/en/result.jsf?_vid=P11-LS5W5W-53175) revealed that India is leading in this area (Figure 1), but patents related to the application of zinc oxide nanoparticles as fertilizers/pesticides are limited. The annual production of ZnO NPs is estimated to be between 550 and 33,400 tonnes, and these are the third most used metal containing nanomaterial (Faizan et al., 2021). Keeping this in mind, nowadays, researchers from all over the world have been shelling out serious attention to ZnO NPs because of their nano-size, ionic nature, crystal structure, dissolution rate, ability to cross plant cell-wall, and large surface area-to- volume ratio (Miri et al., 2019; Verma et al., 2023). These nano-sized ZnO NPs can easily interacts with bacterial/fungal cell surface and core, and thereby enters the pathogens and exhibits antimicrobial activity. The size, shape, surface to volume ratio, and quantity of oxygen vacancy sites of ZnO NPs all directly affects their antimicrobial efficacy (Sirelkhatim et al., 2015; Happy et al., 2019; Ogunyemi et al., 2019). Therefore, in the present epoch of nanotechnology, the utilization of ZnO NPs have been extensively investigated.
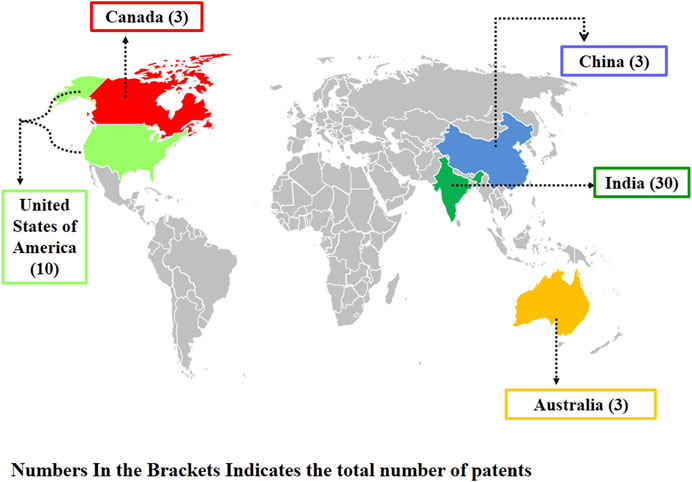
Figure 1. Worldwide distribution of Patents in the field of utilization of nanoparticles as “Nanofertilizers”.
No doubt, the ZnO NPs have broad application in the disease management but from the literature analysis it has been observed that research in this area is very limited. Researcher faced many challenges for utilization of these nanoparticles for different plants. For example, it is unclear how these nanoparticles work under biotic stress for various plants, and the best synthesis method for ZnO NPs, optimum size and shape, and most important best concentration for better results without causing any toxicity to plants. Keeping all these blind spot in view, this review highlights various important studies for better utilization of ZnO NPs. Firstly, we described the biological methods for the synthesis of ZnO NPs. Secondly, we have elaborated their role as antimicrobial agent in biotic stress mitigation in different crop plants. Lastly, an opinion for the utilization of these nanoparticles in a strict manner to avoid the toxicity of these nanoparticles for plants health is also highlighted. This study is also contributing important role in zero hunger goal of SDGs for better tomorrow by enhancing the yield and growth of crop by eliminating the biotic stress.
2 Synthesis of ZnO NPs
Generally, the synthesis of ZnO NPs is carried out by chemical, physical, and biological techniques. Chemical approach involves extensive utilization of co-precipitation, precipitation, water-oil microemulsion, hydrothermal, cellulothermal, sonochemical, sol-gel processing, colloidal, and polyol methods. Furthermore, physical approach is based upon thermal evaporation, ultrasonic irradiation, laser ablation, and arc plasma method (Al-darwesh et al., 2024). However, all these techniques are costly, shows lack of repeatability and dependability, based upon additional supplementation of capping and reducing agents, require toxic chemicals, high temperature and pressure for the process (Balogun et al., 2024). Moreover, toxic chemicals used in these processes sometimes resides inside the synthesized nanoparticles, that in turn reduces the activity of NPs (Kharde et al., 2024).
In order to overcome these drawbacks over the past 10 years, there has been an apparent interest in the biological approach for the synthesis of ZnO NPs. The main reason for the successful utilization of this method is based upon the lower energy and cost requirements for the process, lack of usage of hazardous chemicals, renewable feedstocks, and less release of noxious secondary waste (Mirzaei and Darroudi, 2017; Bandeira et al., 2020). In this context, the following section provides the comprehensive details regarding the green synthesis of ZnO NPs using plant, bacteria, fungi, and algal extracts.
2.1 Plant extract
In the scientific research community, plants offer a cheap, safe, biocompatible, and readily available green material for the synthesis of various types of metallic nanoparticles. Different plant parts like leaves, roots, flowers, stem, and seed husk are rich deposits of natural reducing and capping agents like carbohydrates, amino acids, vitamins, lipids, proteins, secondary metabolites (steroids, flavonoids, coumarins, terpenoids, tannins) that plays an important role in the fabrication, and stabilization of synthesized nanoparticles (Priya et al., 2021; Sidhu et al., 2022) (Table 1; Figure 2].
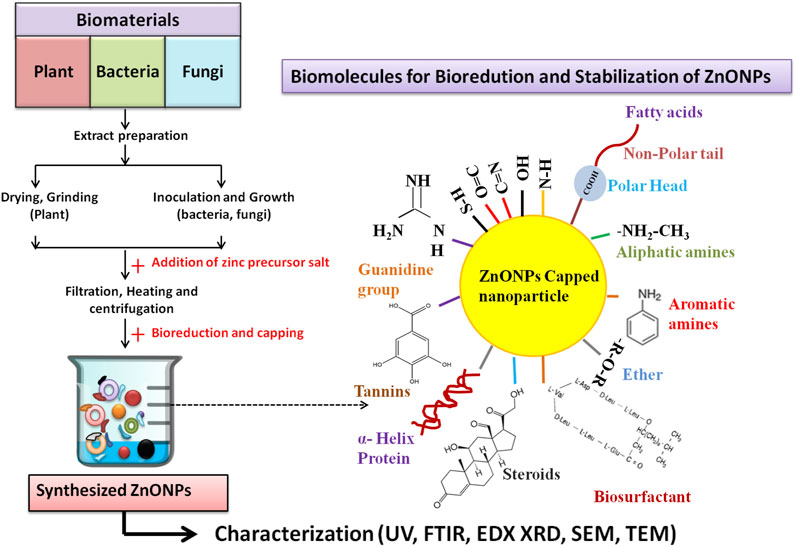
Figure 2. Schematic representation of green synthesis of ZnO NPs by plant, bacteria and fungi. Bold arrows (→) indicates the different steps involved in the synthesis process; (→) dotted arrow indicates the biological reduction, capping and stabilization of synthesized ZnO NPs by natural biomolecules present in the extracts.
Selvaraj et al. (2020) highlighted the synthesis of ZnO NPs by using aqueous leaf extract of Sesbania grandiflora. The fourier transform infrared spectroscopy (FTIR) spectra of synthesized NPs showed broad peaks in the region at 3,441 and 3,105 cm−1, 1,611 and 1,555 cm−1, and 1,440 and 1,392 cm−1 that mainly corresponds to the vibrations of–OH, C=O, and -C-H functional groups. This clearly indicates the bioreduction of ZnO NPs by the biomolecules present in the fresh leaf extract. In terms of the antimicrobial efficacy of the synthesized ZnO NPs, leaf extract based NPs showed significant inhibition of Pseudomonas aeruginosa and Staphylococcus aureus pathogens growth as compared to chemically synthesized ZnO NPs. Furthermore, Ogunyemi et al. (2019) team synthesized ZnO NPs by using the three different plant extracts, i.e., Matrocaria chamomilla L. flowers, Olea europaea leaves, and Lycopersicon esculentum M. fruit. The results of FTIR spectra of ZnO NPs synthesized by three types of extracts showed broad bands of O-H, CH3, C=O, C-O-H, and C-N vibrations confirming the presence of terpenes, flavonoids, tannins, glycosides, and alkaloids in the extract that acts as reducing and capping agents for the synthesis of NPs. The ZnO NPs were also effective in retarding the growth of Xanthomonas oryzae pv. oryzae (Xoo) strain GZ 0003 by inhibiting biofilm formation, bacterial cell membrane, and swimming motility, that in turn helps in controlling bacterial blight disease. In the same year, Kahsay et al. (2019) reported the facile, green strategy for the synthesis of ZnO NPs by using aqueous leaf extract of Dolichos Lablab L. The possible functional groups involved in the reduction of ZnO NPs were confirmed by FTIR analysis. –OH, C-H, C=O, C-O, N-O stretching vibrations clearly indicates that alcohol or phenol, alkane, amide, carboxylic, ester groups from the alkaloid, phenol, flavonoid, amino acid, protein, terpenoid and saponin phyto-constituents of the extract were responsible for the stabilization of ZnO NPs. Additionally, 2.5–10 mg/mL concentration of synthesized ZnO NPs also inhibits Bacillus pumilus and Sphingonomas paucimobilis pathogen effectively as same as standard ciprofloxacin antibiotic. Interestingly, Thi et al. (2020) shed lights on the efficient way of synthesis of ZnO NPs by using Citrus sinensis aqueous fruit peel extract. The FTIR analysis of the synthesized ZnO NPs at different annealing temperatures (300°C–900°C) showed common C=C, and C=O functional groups. Further, it has been observed that 0.025 mg/mL concentration of ZnO NPs were effective in producing ROS in the water suspension that in turn excellently retards the activity of Escherichia coli and S. aureus. Furthermore, research work carried out by Ahmad et al. (2020); Alamdari et al. (2020); Dhandapani et al. (2020); Naseer et al. (2020); Pillai et al. (2020); Selim et al. (2020) provides the biogenic approach for the synthesis of ZnO NPs by using Eucalyptus globules, Sambucus ebulus, Melia azedarach, Cassia fistula, M. azedarach, Beta vulgaris, Cinnamomum tamala, Cinnamomum verum, Brassica oleracea var. Italica, Deverra tortuosa plants. It has been observed that in the case of E. globules leaves extract the FTIR analysis of the synthesized ZnO NPs revealed that presence of C-H groups were mainly associated with the aromandendrenemyrtenal, borneol, camphene, carvacrol, citronellal, citronellyl acetate, cryptone-α-terpenyl acetate essential oil components, which helps in the capping and stabilization of the synthesized NPs. Additionally, flavonoids, protein molecules and other phytochemicals help in the biological reduction of the ZnO NPs.
Similarly, Abomuti et al. (2021); Faisal et al. (2021); Jayachandran et al. (2021); Pachaiappan et al. (2021); Ramesh et al. (2021) also provides attention on the green bio-fabrication of ZnO NPs by using aqueous leaf extract of Salvia officinalis L., fruit extracts of Myristica fragrans, plant extract of Cayratia pedata, leaf extract of Justicia adhatoda, and Cassia auriculata. A deep analysis of various functional groups involved in the biological synthesis process revealed that rosmarinic acid, chlorogenic acid, ellagic acid, and luteolin 7-glucoside, polysaccharides, pectins, cellulose, alkynes, terpenoids, and flavonoids had a profound effect on the reduction of ZnO NPs. Intriguingly, Aldeen et al. (2022) first time reports the synthesis of ZnO NPs by using aqueous extract of Phoenix roebelenii palm leaves. In this work, time dependent FTIR spectral analysis was performed for determining the mechanism of stabilization of ZnO NPs by the biomolecules in the extract. The existence of phenolic compounds such as quercetin 3-O-glucoside, apigenin 6,8-di-C-β-glucopyranoside, luteolin 3′-O-β-glucopyranoside, quercetin, luteolin, apigenin, baicalein, p-hydroxybenzoic acid, caffeic acid and vanillic acid functions both as chelating and capping agents that prevents the aggregation of synthesized ZnO NPs. Recently, Yadav et al. (2024); Easmin et al. (2024); Harshitha et al. (2024); Islam et al. (2024); Nazneen and Sultana, 2024; Vithalani and Bhatt, 2024; Wu et al. (2024) also highlights the biological synthesis of ZnO NPs by using extracts of Evolvulus alsinoides, Carica papaya peel, Cymodocea serrulata, Allium cepa L. peel, Cissus quadrangularis L. stem, Allium sativum, and Aloe vera plants. In continuation to the fabrication of the synthesized ZnO NPs, phytochemicals such as squalene, piperine, unsaturated fatty acids, polyphenols, alkaloids, carboxylic acids acts as a bio-reduction agents.
Overall, the presence of natural reducing, capping, fabricating, and stabilizing agents in the plants extracts bypass the toxic chemicals for the synthesis of the ZnO NPs.
2.2 Microbes based synthesis of ZnO NPs
Microorganisms based biological synthesis of metallic nanoparticles is considered as an inexpensive, simple, highly productive, and biocompatible strategy (Abdo et al., 2021). Several reports showed that microbes utilizes inherent intracellular and extracellular mechanism for the synthesis of metallic nanoparticles. The presence of metal ion transporters, enzymes, co-enzymes, polysaccharides, and proteins over the microbial cell provide the surface for binding of the metal ions, followed by the reduction of Zn2+ by NADH- dependent electron transfer (Mohd Yusof et al., 2019; Sidhu et al., 2022) [Table 1; Figure 2].
Rajabairavi et al. (2017) reported the synthesis of ZnO NPs by using endophytic bacteria Sphingobacterium thalpophilum. The results speculates that proteins present in the bacterial culture supernatant helps in the bio-reduction/fabrication of ZnO NPs by electrostatic and hydrophobic interactions. Furthermore, Ebadi et al. (2019); Jayabalan et al. (2019) also demonstrates the green synthesis of ZnO NPs by using cyanobacterium Nostoc sp. EA03 and Pseudomonas putida culture. FTIR spectrum revealed that the fatty acids, and phenolic OH amino acids (tyrosine, phenylalanine) of the bacterial extracellular proteins contribute in the capping of the ZnO NPs. Mahdi et al. (2021) and his coworkers also elucidate the biosynthesis of ZnO NPs using Lactococcus lactis NCDO1281(T) and Bacillus sp. PTCC 1538 strains. The characterization of ZnO NPs by both the strains showed that the cell membrane proteins were mainly involved in the synthesis and stabilization of NPs in the culture medium. Moreover, Abdo et al. (2021) also highlighted the green synthesis of ZnO NPs using P. aeruginosa NMj15. In this research, the functional groups (C=O, O-H, NH, and SH) of metabolites present in the cell free filtrate of the strain acted as biocatalysts, reducing and stabilizing agents for the synthesis of ZnO NPs. Additionally, Faisal et al. (2022); EL-GHWAS (2022) also presented the ZnO NPs synthesis by using Paraclostridium benzoelyticum strain 5,610 and Bacillus foraminis. The FTIR analysis findings demonstrated that the carbonyl groups from the amino acids residues of the proteins made a substantial contribution in the capping of the nanoparticles. Recently, Ashour and Abd- Elhalim (2024), El-Refai et al. (2024) synthesized ZnO NPs using Priestia megaterium and Bacillus paramycoides bacteria. The results point out that the ZnO NPs were coated with the C, N, O, Cl, K, and Na atoms which clearly indicate that enzymes and proteins present in the cell free supernatant help in the reduction of Zn metal ions.
Besides bacteria, fungal extract is also used for the synthesis of ZnO NPs. Rajan et al. (2016) reported the synthesis of ZnO NPs by using fungus Aspergillus fumigatus JCF. It has been observed that synthesized ZnO NPs were mainly coated with N-H, C=C, C=O functional groups. All these functional moieties were from the aliphatic, aromatic amino acids, and proteins present in the fungal filtrate. Furthermore, another strain of Aspergillus, i.e., Aspergillus niger has also been used for the synthesis and bio-fabrication of ZnO NPs (Gao et al., 2019). The results imply that the strong aromatic rings and carboxylic acids of the fungus biomolecules helps in the reduction and capping process of the ZnO NPs. Sumanth et al. (2020) point up the mycogenic synthesis of ZnO NPs by using Xylaria acuta extract. The FTIR spectrum showed that the OH groups of hydroxyl and polyphenols were mainly involved in the utilization of ZnO NPs. Moreover, the culture filtrate of the endophytic fungus Alternaria tenuissima has also been used as an eco-friendly and cost-effective method for the synthesis of ZnO NPs (Abdelhakim et al., 2020). Phenols and Primary amine groups of active metabolites were mainly involved in the synthesis and capping of the ZnO NPs. Sharma et al. (2021); Abdelkader et al. (2022) illustrated the Phanerochaete chrysosporium and A. niger mediated synthesis of ZnO NPs. It has been noted that the carboxylic acids, phenolic acids, and amides in the enzymatic proteins of the fungal extract prevents the aggregation of ZnO NPs and helps in the stabilization of NPs.
No doubt microorganisms are extensively used for the synthesis of ZnO NPs, but still there are many drawbacks of this methodology. Firstly, isolation, selection and screening of microbes is time consuming. Secondly, mechanism of reduction and capping of NPs varies microbe to microbe. Most importantly, metal salt precursors sometimes get precipitated in the culture medium used for microbe growth. Furthermore, optimization of pH, temperature, and time for reaction set up is also crucial for the synthesis of ZnO nanoparticles. Therefore, in order to enhance the yield of ZnO NPs and the efficacy of this nano-factory further investigation in terms of detailed biochemical and molecular mechanism is required.
3 Role of ZnO NPs in biotic stress management
3.1 ZnO NPs as antimicrobial agents
ZnO NPs exhibit notable antifungal and antibacterial properties owing to their abrasive surface texture, smaller particle size, higher surface reactivity, and greater specific surface area (Singh et al., 2018). Previous investigations conducted by various research groups showed that metallic nanoparticles interfere with the cellular processes of the microbes and also cause cell immobilization (Janaki et al., 2015). Firstly, Zn2+ ions releases from ZnO NPs that inhibits the bacterial active transport, metabolism and enzyme activity. Secondly, ZnO NPs also interacts with the bacterial cell surface through electrostatic interaction, therefore stimulates the leakage of intracellular content. Thirdly, ZnO NPs also reacts with hydroxyl group and absorbs water that in turn induces the formation of ROS such as superoxide anion (O2−), hydroxyl ion (OH−), and hydrogen peroxide (H2O2). Out of all these ROS, H2O2 easily penetrates the bacterial cell membrane and damages the structure, integrity and function of biologically important biomolecules like ribosomes, lipids, proteins, nucleic acids, hence the bacterial cell dies (Mohd Yusof et al., 2019) (Figure 3).
Dimkpa et al. (2013) explored the antifungal efficacy of ZnO NPs suspension (500 mg Zn/l) against Fusarium graminearum pathogen, observing that the treatment with such higher level of Zn solution significantly reduced the 75% growth of the fungus on the MBA (mung bean agar) medium as compared to the control. The main reason behind the inhibitory action of ZnO NPs was the release of soluble Zn ions from the nanoparticles. Moreover, ZnO NPs synthesized by using the leaf extract of Parthenium hysterophorus L. also showed its antifungal activity against plant fungal pathogens including Aspergillus flavus, Fusarium culmorum and A. niger. It has been observed that 50 μg/mL concentration of 27 ± 5 nm size of ZnO NPs showed maximum zone of inhibition against A. flavus with inhibition diameter (24.66 ± 0.57 mm) (Rajiv et al., 2013). Kairyte et al. (2013) also explored the antibacterial activity of photo-activated ZnO NPs against E. coli O157:H7, Listeria monocytogenes ATCL3C 7644 and plant pathogenic fungus Botrytis cinerea. It has been observed that illumination of 1 × 10−3 M ZnO NPs with light (λ = 400 nm; 173 kJ/m2) significantly reduced the E. coli and L. monocytogenes population from 7log with 10 min–60 min incubation time. Meanwhile, treatment of fungus with 5 × 10−3 M concentration of photo-activated NPs reduced the mycelial growth by 58%. Interestingly, Narendhran and Sivaraj (2016) also studied the antifungal activity (against plant pathogens) of biogenic ZnO NPs (synthesized by using aqueous extract of Lantana aculeata Linn. Leaf). Results of well diffusion assay showed the highest zone of inhibition (21 ± 1.0 mm) against A. niger (MTCC: 10,180) followed by Fusarium oxysporum (MTCC: 3,326) (19 ± 1.0 mm) at 100 μg/mL concentration of ZnO NPs.
Anbukkarasi et al. (2015) investigated the antimicrobial activity of ZnO NPs against bacterial pathogens (Bacillus subtilis, Streptococcus pneumonia, Staphylococcus epidermidis, Klebsiella pneumonia, Salmonella typhi, and E. coli) and fungal pathogens (A. niger, and Candida albicans). Results indicate that 10 µg, 20µg, 40 µg, 60 µg and 80 µg concentration of ZnO NPs showed higher zone of growth inhibition against S. epidermidis (32 mm) followed by E. coli (31 mm), while no significant growth inhibition was observed against fungal pathogens. This underscores that ZnO NPs synthesized from Emblica officinalis is more effective against bacterial pathogens. Furthermore, antimicrobial activity of 20–25 mm size of 20, 40, 60, 80 and 100 μg/mL concentration of ZnO NPs was also studied against gram positive (S. aureus), gram negative bacteria (Salmonella typhimurium), and fungus (A. flavus and A. fumigatus). It has been noted that 40 μg/mL is the minimum inhibitory concentration (MIC) of ZnO NPs against both the bacterial pathogens. However, for fungal pathogens 60 μg/mL and 80 μg/mL concentration of ZnO NPs showed the excellent anti-fungal activity as compared to the 20, 40, and 100 μg/mL concentration (Navale et al., 2015). Another study also highlights the antibacterial activity of green synthesized ZnO NPs (using Brassica oleraceae leaf extract) against E. coli, V. cholera, Clostridium botulinum, S. aureus, and B. subtilis. It has been observed that highest zone of inhibition was observed in S. aureus (15 mm) followed by B. subtilis (14.5 mm), E. coli (13 mm), C. botulinum (10 mm), and V. cholera (9.5 mm) at 75 μg/mL concentration of ZnO NPs (Raj et al., 2015). Similar work also showed the antibacterial activity of green synthesized ZnO NPs (using aqueous peel extract of A. vera) against pathogenic bacteria, namely, E. coli (MTCC-41), S. epidermidis (MTCC-3382), S. epidermidis (MTCC-3382), Klebsiella pneumoniae (MTCC-3384), and fungi, A. niger (MTCC-404) and Aspergillus oryzae (MTCC-3107). Results revealed that synthesized ZnO NPs were only effective against E. coli (14 mm zone of inhibition) and A. niger (15 mm zone of inhibition). However no antimicrobial activity was observed in the case of other pathogens (Chaudhary et al., 2019) (Table 2).
Overall, we can conclude that ZnO NPs generally disturbs the metabolic processes of the microbes, generates oxidative stress, proton motive force over cell wall and oxidized the important cell wall structures which in turn lead to the death of the pathogens. This antimicrobial activity of these nanoparticles can be utilized to control fungal and bacterial pathogens in the agricultural fields.
3.2 Effect of ZnO NPs in disease mitigation
Plants have evolved defense systems that trigger a range of signal transduction and perception pathways in order to protect themselves against biotic stress (Masri and Kiss, 2023). ZnO NPs also induce antioxidants machinery, osmolytes, and systemic resistance in plants in order to provide resistance against pathogens (Tortella et al., 2023) (Figure 4). These nanoparticles inhibit the pathogens by disturbing membrane potential, inducing DNA damage, cell damage, inducing ROS, cell cycle arrest, and by direct attachment to the cell surface (Khan et al., 2021a; Kalra et al., 2022).
A work carried out by Nandhini et al. (2019) evaluated the efficacy of green synthesized ZnO nanoparticles against downy mildew (caused by Sclerospora graminicola) disease in pearl millet. It has been observed that seed treatment and foliar spray with 50 ppm nanoparticles inhibited spore germination and reduced 35% disease incidence as compared to control plants. Furthermore, a significant increase in the activities of defense enzymes such as polyphenyl oxidase (PPO), peroxidase (POX), phenylalanine aminolyase (PAL), and lipoxygenase (LOX) were observed in the ZnO NPs treated plants. This clearly indicates that nanoparticles treatment provide disease protection by inducing systemic resistance in plants. Similarly, a research work conducted by Khan and Siddiqui (2021) showed the beneficial role of ZnO NPs (200 mg/L) in the disease management in B. vulgaris L. caused by Meloidogyne incognita, Rhizoctonia solani, and Pectobacterium betavasculorum. However, a significant reduction in the disease indices, nematode multiplication and root galling, along with an increase in the level of defensive antioxidants like superoxide dismutase (SOD), catalase (CAT), PAL and PPO were observed in the nanoparticles treated plants. Moreover, ZnO NPs also exhibit antiviral property and retard the growth of tobacco mosaic virus (ToMV) in tomato plants (Abdelkhalek and Al- Askar, 2020; Sofy et al., 2021). The foliar application of ZnO NPs (100 μg/mL) reduced viral accumulation and disease severity by 90.21% in affected tomato plants. Notably, the transcript level of genes including pathogenesis-related (PR-1), PAL, chalcone synthase (CHS), and peroxidase (POD) were also upregulated in the nanoparticles treated plants (Abdelkhalek and Al- Askar, 2020). Similarly in Sofy et al. (2021) research it has been noted that ZnO NPs (50 mg/L and 100 mg/L) application reduced the level of oxidative stress markers malondialdehyde (MDA), hydrogen peroxide (H2O2)and oxygen (O2) and promotes the activity of enzymatic (CAT, SOD, POX, ascorbate peroxidase (APX), glutathione reductase (GR), LOX, carbonic anhydrase (CA) and nitrate reductase (NR) and non-enzymatic antioxidants ascorbic acid (AsA), glutathione (GSH), proline and phenol).
Apart from viral infection, in the case of bacteria (Pseudomonas syringae pv. tomato, Xanthomonas campestris pv. vesicatoria, Pectobacterium carotovorum subsp. and Ralstonia solanacearum) and fungus (F. oxysporum f. sp. lycopersici and Alternaria solani) effected tomato plants, foliar and seed priming with ZnO NPs (0.20 mL-1) reduced disease incidence and improved photosynthetic pigments (total chlorophyll and carotenoids) and proline content in the diseased plants (Parveen and Siddiqui, 2021). Furthermore, antibacterial potential of ZnO NPs were also observed in the tomato plants. The results of concentration (0, 1, 4, and 8%) dependent application of ZnO NPs reduced the R. solanacearum population in the soil, disease severity and had increased shoot, root length, and fresh biomass (Khan et al., 2021b). Additionally, the ZnO NPs loaded hydrogel also showed beneficial role in protecting the pepper plant from pathogenic fungi (F. oxysporum). Application of hydrogel significantly reduced (77%) the wilt disease symptoms and also increased the chlorophyll, carotenoid content, osmolytes (soluble sugars, proteins, and proline), total phenolic content and the activity of POX and PPO in the diseased plants (Abdelaziz et al., 2021). Besides the hydrogel treatment results, similar results were also observed with ZnO NPs application or controlling Fusarium wilt disease in eggplant (Abdelaziz et al., 2022). Moreover, Farhana et al. (2022) research speculates that ZnO NPs also modulates the antioxidant machinery in chickpea for alleviating Fusarium wilt disease. It has been observed that seed priming significantly reduced the disease incidence by 90% and increased the quantities of sugars, phenol, flavonoids, total proteins and antioxidants (SOD, POD and CAT). Shams et al. (2023) also highlighted the efficacy of foliar spray of ZnO NPs against Fusarium wilt disease in cherry tomato. The results showed that 3000 ppm concentration of ZnO NPs reduced disease severity index (92.6%) and enhanced micronutrient (Mn, Zn, and Fe), chlorophyll content, and PAL enzyme activity. Another work carried out by Mazhar et al. (2023) showed that foliar application of ZnO NPs (10, 20, 30, and 40 mg/L) reduced the negative effect of blast fungus (Magnaporthe oryzae) and simultaneously promote the rice growth. ZnO NPs significantly decreased the lesion numbers, sporulation intensity, MDA, H2O2 level and also improved the total chlorophyll, activity of antioxidant enzymes like SOD, PAL, POX, and CAT.
However, the exact mechanism of action of ZnO NPs in reducing the pathogens attack is not clear yet and it varies from plant to plant. But overall from these cited literature studies, we can extrapolate that ZnO NPs usually reduces the bacterial, fungal, and viral infection by inducing systemic based resistance pathway, production of antioxidants, non-antioxidants enzymes, osmolytes (Table 3).
4 Toxicity of ZnO nanoparticles
Recently ZnO NPs have been widely used in agricultural industries, but their toxicity is becoming a serious concern. There is very limited literature available on the toxicity of ZnO NPs in plants. Higher concentration of ZnO NPs exposure causes bioaccumulation in the plant tissue, exerts oxidative stress, nutrient imbalance, photosynthesis inhibition and causes lipid peroxidation of the plant cells leading to cell membrane damage (Amooaghaie et al., 2017; Song and Kim, 2020; Kang et al., 2024). As per a well-known mechanism that ZnO NPs can easily interacts with the plant defensive molecules like metallothionein and fully blocks their binding sites with Fe2+ and Cu2+ ions which in turns create ROS in the plants (Hou et al., 2018). It has been noted that the antimicrobial activity of ZnO NPs generally depends upon the concentration. It is important to evaluate the optimum concentration, dose and size of NPs to be used in agriculture (Shaw and Hossain, 2013).
The cytotoxicity of ZnO NPs, increase with the increase in the diameter of the particles. The toxic effect of ZnO NPs were also observed in maize plants at high doses, ranging from 800 mg/kg to 3,200 mg/kg, resulting in reduced growth of plants shoots and roots. Furthermore, mineral uptake by the shoots and roots, as well as the content of chlorophyll (a and b) and carotenoids also decreased (Liu et al., 2015). Furthermore, the concentrations between 500 mg and 1500 mg/L range were found to have detrimental effects on stem explant culture and seed germination of Brassica nigra. It has been observed that the presence of ZnO NPs in culture media inhibited the seed germination and reduced root length (Zafar et al., 2016). ZnO NPs of ∼85 nm with a concentration of 200–800 mg/L were found to induce cell death in root cells of A. cepa, Vicia faba, and Nicotiana tabacum plants. This induction was associated with increased levels of ROS production, resulting in DNA damage, H2O2 production, and lipid peroxidation (Ghosh et al., 2016). Moreover, Hordeum vulgare L. seedlings treated with 20 mg and 40 mg/kg concentrations of ZnO NPs decreased the level of chlorophyll content, SOD, APX, proline, and glutathione content as compared to control seedlings (Doğaroğlu and Köleli, 2017).
Similarly, in Solanum lycopersicum L. ZnO NPs (200mg–800 mg dm-3) negatively affect the growth of plant shoot, root and reduced the content of chlorophyll a and b. Moreover, gene expression analysis indicated downregulation of key photosynthesis genes such as sedoheptulose-1,7-bisphosphatase (SBPASE) and fructose-1,6-bisphosphatase (FBPASE) and chlorophyll synthesis genes including chlorophyllide a oxygenase (CAO), chlorophyll synthase (CHLG), magnesium-protoporphyrin IX monomethylester [oxidative] cyclase (CRD1), magnesium-chelatase subunit ChlI (CHL1), protoporphyrinogen oxidase (HEMG), 5-aminolevulinate dehydratase (HEMB), and porphobilinogen deaminase (HEMC) when plants were exposed to high concentrations of toxic ZnO NPs (Wang et al., 2018). Additionally, another research work conducted by Czyżowska and Barbasz (2019) on the callus of wheat varieties reported that applying ZnO NPs (130 nm diameter and 12 mg dm-3 concentration) reduced the activity of SOD and generates oxidative stress (Table 4; Figure 5). Furthermore, their research demonstrated that the harmful effects of ZnO NPs on plants were also heightened in the presence of UV radiation.
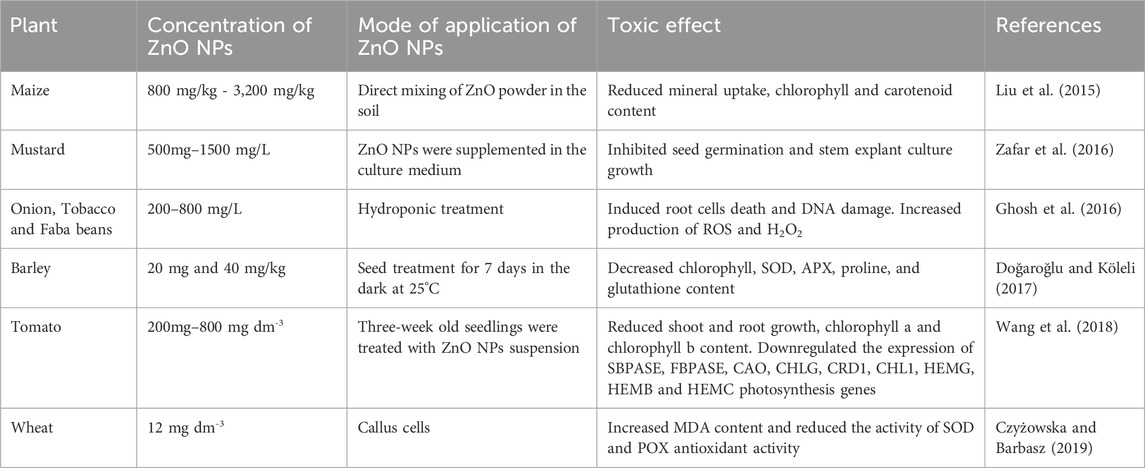
Table 4. Toxic effect of ZnO NPs over physiological, biochemical and molecular functioning of different plants.
In summary, toxicity of these nanoparticles can be overcome by optimizing the size and shape of the nanoparticles. Thus, by keeping all these parameters in consideration toxicity of nanoparticles can be reduced.
5 Conclusion
In conclusion, the pressing issue of biotic stress in agriculture, compounded by global warming and shifting climate patterns, needs new and sustainable crop management measures. This review explored into the possibility of zinc oxide nanoparticles (ZnO NPs) as a viable solution to biotic stress due to their antibacterial characteristics and role as nano-fertilizers. The biological synthesis of ZnO NPs, which utilizes plants and microorganisms, provides an environmentally friendly and possibly scalable approach to produce these nanoparticles.
ZnO NPs are highly effective in treating a variety of pathogens, including bacteria, fungi, viruses, and insects, by affecting their physiological and molecular processes. Despite the fact that these particles have numerous applications on plants, there have been reports of their possible toxicity. The potential toxicity of ZnO nanoparticles on plants can be reduced by employing strategies such as dosing optimization, controlled release systems, nanoparticle surface modification, and combination with other agents can be used to ensure effective biotic stress mitigation while minimizing adverse effects. Additionally, using targeted delivery and combining with sustainable methods improves safety and efficacy. However, the use of these nanoparticles in agriculture is still in its early stages, with numerous problems that must be addressed. These include studying the mechanisms of action of ZnO NPs under various biotic stress circumstances, optimizing synthesis methods, defining the most effective nanoparticle size and shape, and establishing safe concentration limits to avoid plant toxicity. Future research should focus on resolving these limitations in order to fully utilize ZnO NPs in agricultural applications. Rigorous testing and regulation will be required to ensure their safety and sustainability.
To summarize, while ZnO NPs provide intriguing options for biotic stress alleviation, their use should be addressed with caution. Comprehensive research and careful implementation are required to maximize the advantages while limiting the dangers. By improving our understanding and application of ZnO NPs, we can help to build a more sustainable and resilient agricultural sector, thereby aiding worldwide efforts to secure food production and promote environmental sustainability.
Author contributions
NV: Conceptualization, Data curation, Formal Analysis, Investigation, Methodology, Visualization, Writing–original draft, Writing–review and editing. PK: Conceptualization, Data curation, Formal Analysis, Investigation, Methodology, Visualization, Writing–original draft, Writing–review and editing. AS: Conceptualization, Data curation, Formal Analysis, Supervision, Validation, Writing–review and editing.
Funding
The author(s) declare that no financial support was received for the research, authorship, and/or publication of this article.
Conflict of interest
The authors declare that the research was conducted in the absence of any commercial or financial relationships that could be construed as a potential conflict of interest.
Publisher’s note
All claims expressed in this article are solely those of the authors and do not necessarily represent those of their affiliated organizations, or those of the publisher, the editors and the reviewers. Any product that may be evaluated in this article, or claim that may be made by its manufacturer, is not guaranteed or endorsed by the publisher.
References
Abdelaziz, A. M., Dacrory, S., Hashem, A. H., Attia, M. S., Hasanin, M., Fouda, H. M., et al. (2021). Protective role of zinc oxide nanoparticles based hydrogel against wilt disease of pepper plant. Biocatal. Agric. Biotechnol. 35, 102083. doi:10.1016/j.bcab.2021.102083
Abdelaziz, A. M., Salem, S. S., Khalil, A. M., El-Wakil, D. A., Fouda, H. M., and Hashem, A. H. (2022). Potential of biosynthesized zinc oxide nanoparticles to control Fusarium wilt disease in eggplant (Solanum melongena) and promote plant growth. BioMetals 35 (3), 601–616. doi:10.1007/s10534-022-00391-8
Abdelhakim, H. K., El-Sayed, E. R., and Rashidi, F. B. (2020). Biosynthesis of zinc oxide nanoparticles with antimicrobial, anticancer, antioxidant and photocatalytic activities by the endophytic Alternaria tenuissima. J. Appl. Microbiol. 128 (6), 1634–1646. doi:10.1111/jam.14581
Abdelkader, D. H., Negm, W. A., Elekhnawy, E., Eliwa, D., Aldosari, B. N., and Almurshedi, A. S. (2022). Zinc oxide nanoparticles as potential delivery carrier: green synthesis by Aspergillus niger endophytic fungus, characterization, and in vitro/in vivo antibacterial activity. Pharmaceuticals 15 (9), 1057. doi:10.3390/ph15091057
Abdelkhalek, A., and Al-Askar, A. A. (2020). Green synthesized ZnO nanoparticles mediated by Mentha spicata extract induce plant systemic resistance against Tobacco mosaic virus. Appl. Sci. 10 (15), 5054. doi:10.3390/app10155054
Abdo, A. M., Fouda, A., Eid, A. M., Fahmy, N. M., Elsayed, A. M., Khalil, A. M. A., et al. (2021). Green synthesis of Zinc Oxide Nanoparticles (ZnO-NPs) by Pseudomonas aeruginosa and their activity against pathogenic microbes and common house mosquito, Culex pipiens. Materials 14 (22), 6983. doi:10.3390/ma14226983
Abomuti, M. A., Danish, E. Y., Firoz, A., Hasan, N., and Malik, M. A. (2021). Green synthesis of zinc oxide nanoparticles using salvia officinalis leaf extract and their photocatalytic and antifungal activities. Biology 10 (11), 1075. doi:10.3390/biology10111075
Ahmad, H., Venugopal, K., Rajagopal, K., De Britto, S., Nandini, B., Pushpalatha, H. G., et al. (2020). Green synthesis and characterization of zinc oxide nanoparticles using Eucalyptus globules and their fungicidal ability against pathogenic fungi of apple orchards. Biomolecules 10 (3), 425. doi:10.3390/biom10030425
Alamdari, S., Sasani Ghamsari, M., Lee, C., Han, W., Park, H. H., Tafreshi, M. J., et al. (2020). Preparation and characterization of zinc oxide nanoparticles using leaf extract of Sambucus ebulus. Appl. Sci. 10 (10), 3620. doi:10.3390/app10103620
Alavi, M., and Yarani, R. (2023). ROS and RNS modulation: the main antimicrobial, anticancer, antidiabetic, and antineurodegenerative mechanisms of metal or metal oxide nanoparticles. Nano Micro Biosyst. 2 (1), 22–30. doi:10.22034/nmbj.2023.382133.1012
Al-darwesh, M. Y., Ibrahim, S. S., and Mohammed, M. A. (2024). A review on plant extract mediated green synthesis of zinc oxide nanoparticles and their biomedical applications. Results Chem. 7, 101368. doi:10.1016/j.rechem.2024.101368
Aldeen, T. S., Mohamed, H. E. A., and Maaza, M. (2022). ZnO nanoparticles prepared via a green synthesis approach: physical properties, photocatalytic and antibacterial activity. J. Phys. Chem. Solids. 160, 110313. doi:10.1016/j.jpcs.2021.110313
Amooaghaie, R., Norouzi, M., and Saeri, M. (2017). Impact of zinc and zinc oxide nanoparticles on the physiological and biochemical processes in tomato and wheat. Botany 95 (5), 441–455. doi:10.1139/cjb-2016-0194
Anbukkarasi, V., Srinivasan, R., and Elangovan, N. (2015). Antimicrobial activity of green synthesized zinc oxide nanoparticles from Emblica officinalis. Int. J. Pharm. Sci. Rev. Res. 33 (2), 110–115.
Ashour, M. A., and Abd-Elhalim, B. T. (2024). Biosynthesis and biocompatibility evaluation of zinc oxide nanoparticles prepared using Priestia megaterium bacteria. Sci. Rep. 14 (1), 4147. doi:10.1038/s41598-024-54460-8
Balogun, S. W., Oyeshola, H. O., Ajani, A. S., James, O. O., Awodele, M. K., Adewumi, H. K., et al. (2024). Synthesis, characterization, and optoelectronic properties of zinc oxide nanoparticles: a precursor as electron transport layer. Heliyon 10 (9), e29452. doi:10.1016/j.heliyon.2024.e29452
Bandeira, M., Giovanela, M., Roesch-Ely, M., Devine, D. M., and da Silva Crespo, J. (2020). Green synthesis of zinc oxide nanoparticles: a review of the synthesis methodology and mechanism of formation. Sustain. Chem. Pharam 15, 100223. doi:10.1016/j.scp.2020.100223
Chaudhary, A., Kumar, N., Kumar, R., and Salar, R. K. (2019). Antimicrobial activity of zinc oxide nanoparticles synthesized from Aloe vera peel extract. SN Appl. Sci. 1, 136–139. doi:10.1007/s42452-018-0144-2
Chaudhary, P., Agri, U., Chaudhary, A., Kumar, A., and Kumar, G. (2022). Endophytes and their potential in biotic stress management and crop production. Front. Microbiol. 13, 933017. doi:10.3389/fmicb.2022.933017
Czyżowska, A., and Barbasz, A. (2019). Effect of ZnO, TiO2, Al2O3 and ZrO2 nanoparticles on wheat callus cells. Acta Biochim. Pol. 66 (3), 365–370. doi:10.18388/abp.2019_2775
Dhandapani, K. V., Anbumani, D., Gandhi, A. D., Annamalai, P., Muthuvenkatachalam, B. S., Kavitha, P., et al. (2020). Green route for the synthesis of zinc oxide nanoparticles from Melia azedarach leaf extract and evaluation of their antioxidant and antibacterial activities. Biocatal. Agric. Biotechnol. 24, 101517. doi:10.1016/j.bcab.2020.101517
Dimkpa, C. O., McLean, J. E., Britt, D. W., and Anderson, A. J. (2013). Antifungal activity of ZnO nanoparticles and their interactive effect with a biocontrol bacterium on growth antagonism of the plant pathogen Fusarium graminearum. Biometals 26, 913–924. doi:10.1007/s10534-013-9667-6
Doğaroğlu, Z. G., and Köleli, N. (2017). TiO2 and ZnO nanoparticles toxicity in barley (Hordeum vulgare L.). Clean–Soil, Air, Water 45 (11), 1700096. doi:10.1002/clen.201700096
Easmin, S., Bhattacharyya, M., Pal, K., Das, P., Sahu, R., Nandi, G., et al. (2024). Papaya peel extract-mediated green synthesis of zinc oxide nanoparticles and determination of their antioxidant, antibacterial, and photocatalytic properties. Bioprocess Biosyst. Eng. 47 (1), 65–74. doi:10.1007/s00449-023-02945-7
Ebadi, M., Zolfaghari, M. R., Aghaei, S. S., Zargar, M., Shafiei, M., Zahiri, H. S., et al. (2019). A bio-inspired strategy for the synthesis of zinc oxide nanoparticles (ZnO NPs) using the cell extract of cyanobacterium Nostoc sp. EA03: from biological function to toxicity evaluation. RSC Adv. 9 (41), 23508–23525. doi:10.1039/C9RA03962G
El-Ghwas, D. E. (2022). Characterization and biological synthesis of zinc oxide nanoparticles by new strain of Bacillus foraminis. Biodiversitas 23 (1), 548–553. doi:10.13057/biodiv/d230159
El-Refai, H. A., Saleh, A. M., Mohamed, S. I., Aboul Naser, A. F., Zaki, R. A., Gomaa, S. K., et al. (2024). Biosynthesis of zinc oxide nanoparticles using Bacillus paramycoides for in vitro biological activities and in vivo assessment against hepatorenal injury induced by CCl4 in rats. Appl. Biochem. Biotechnol., 1–21. doi:10.1007/s12010-023-04817-y
Faisal, S., Jan, H., Shah, S. A., Shah, S., Khan, A., Akbar, M. T., et al. (2021). Green synthesis of zinc oxide (ZnO) nanoparticles using aqueous fruit extracts of Myristica fragrans: their characterizations and biological and environmental applications. ACS omega 6 (14), 9709–9722. doi:10.1021/acsomega.1c00310
Faisal, S., Rizwan, M., Ullah, R., Alotaibi, A., Khattak, A., Bibi, N., et al. (2022). Paraclostridium benzoelyticum bacterium-mediated zinc oxide nanoparticles and their in vivo multiple biological applications. Oxid. Med. Cell. Longev. 2022, 1–15. doi:10.1155/2022/5994033
Faizan, M., Yu, F., Chen, C., Faraz, A., and Hayat, S. (2021). Zinc oxide nanoparticles help to enhance plant growth and alleviate abiotic stress: a review. Curr. Protein Pept. Sci. 22 (5), 362–375. doi:10.2174/1389203721666201016144848
Farhana, , Munis, M. F. H., Alamer, K. H., Althobaiti, A. T., Kamal, A., Liaquat, F., et al. (2022). ZnO nanoparticle-mediated seed priming induces biochemical and antioxidant changes in chickpea to alleviate Fusarium wilt. J. Fungi 8 (7), 753. doi:10.3390/jof8070753
Gao, Y., Arokia Vijaya Anand, M., Ramachandran, V., Karthikkumar, V., Shalini, V., Vijayalakshmi, S., et al. (2019). Biofabrication of zinc oxide nanoparticles from Aspergillus niger, their antioxidant, antimicrobial and anticancer activity. J. Clust. Sci. 30, 937–946. doi:10.1007/s10876-019-01551-6
Ghosh, M., Jana, A., Sinha, S., Jothiramajayam, M., Nag, A., Chakraborty, A., et al. (2016). Effects of ZnO nanoparticles in plants: cytotoxicity, genotoxicity, deregulation of antioxidant defenses, and cell-cycle arrest. Mutat. Res. Genet. Toxicol. Environ. Mutagen. 807, 25–32. doi:10.1016/j.mrgentox.2016.07.006
Gull, A., Lone, A. A., and Wani, N. U. I. (2019). Biotic and abiotic stresses in plants. Abiotic biotic stress plants, 1–19.
Hajong, M., Devi, N. O., Debbarma, M., and Majumder, D. (2019). “Nanotechnology: an emerging tool for management of biotic stresses in plants,” in Plant nanobionics: volume 2, approaches in nanoparticles, biosynthesis, and toxicity. Editor R. Prasad (Springer Nature), 299–335.
Happy, A., Soumya, M., Kumar, S. V., Rajeshkumar, S., Sheba, R. D., Lakshmi, T., et al. (2019). Phyto-assisted synthesis of zinc oxide nanoparticles using Cassia alata and its antibacterial activity against Escherichia coli. Biochem. Biophys. Rep. 17, 208–211. doi:10.1016/j.bbrep.2019.01.002
Harshitha, V. R. S., Ilangovar, I. G. K., Suresh, V., Pitchiah, S., and Sivaperumal, P. (2024). Synthesis of zinc oxide nanoparticles from Cymodocea serrulata leaf extract and their biological activities. Cureus 16 (3), e55521. doi:10.7759/cureus.55521
Hou, J., Wu, Y., Li, X., Wei, B., Li, S., and Wang, X. (2018). Toxic effects of different types of zinc oxide nanoparticles on algae, plants, invertebrates, vertebrates and microorganisms. Chemosphere 193, 852–860. doi:10.1016/j.chemosphere.2017.11.077
Islam, M. F., Miah, M. A. S., Huq, A. O., Saha, A. K., Mou, Z. J., Mondol, M. M. H., et al. (2024). Green synthesis of zinc oxide nano particles using Allium cepa L. waste peel extracts and its antioxidant and antibacterial activities. Heliyon 10 (3), e25430. doi:10.1016/j.heliyon.2024.e25430
Janaki, A. C., Sailatha, E., and Gunasekaran, S. (2015). Synthesis, characteristics and antimicrobial activity of ZnO nanoparticles. Acta A. Mol. Biomol. Spectrosc. 144, 17–22. doi:10.1016/j.saa.2015.02.041
Jayabalan, J., Mani, G., Krishnan, N., Pernabas, J., Devadoss, J. M., and Jang, H. T. (2019). Green biogenic synthesis of zinc oxide nanoparticles using Pseudomonas putida culture and its in vitro antibacterial and anti-biofilm activity. Biocatal. Agric. Biotechnol. 21, 101327. doi:10.1016/j.bcab.2019.101327
Jayachandran, A., Aswathy, T. R., and Nair, A. S. (2021). Green synthesis and characterization of zinc oxide nanoparticles using Cayratia pedata leaf extract. BB Rep. 26, 100995. doi:10.1016/j.bbrep.2021.100995
Kahsay, M. H., Tadesse, A., RamaDevi, D., Belachew, N., and Basavaiah, K. (2019). Green synthesis of zinc oxide nanostructures and investigation of their photocatalytic and bactericidal applications. RSC Adv. 9 (63), 36967–36981. doi:10.1039/C9RA07630A
Kairyte, K., Kadys, A., and Luksiene, Z. (2013). Antibacterial and antifungal activity of photoactivated ZnO nanoparticles in suspension. J. Photochem. Photobiol. B Biol. 128, 78–84. doi:10.1016/j.jphotobiol.2013.07.017
Kalra, K., Chhabra, V., and Prasad, N. (2022). Antibacterial activities of zinc oxide nanoparticles: a mini review. J. Phys. Conf. Ser. 2267 (1), 012049. doi:10.1088/1742-6596/2267/1/012049
Kang, M., Liu, Y., Weng, Y., Wang, H., and Bai, X. (2024). A critical review on the toxicity regulation and ecological risks of zinc oxide nanoparticles to plants. Environ. Sci. Nano. 11, 14–35. doi:10.1039/D3EN00630A
Khan, M., Khan, A. U., Hasan, M. A., Yadav, K. K., Pinto, M. M., Malik, N., et al. (2021). Agro-nanotechnology as an emerging field: a novel sustainable approach for improving plant growth by reducing biotic stress. Appl. Sci. 11 (5), 2282. doi:10.3390/app11052282
Khan, M. R., and Siddiqui, Z. A. (2021). Role of zinc oxide nanoparticles in the management of disease complex of beetroot (Beta vulgaris L.) caused by Pectobacterium betavasculorum, Meloidogyne incognita and Rhizoctonia solani. Hortic. Environ. Biotechnol. 62, 225–241. doi:10.1007/s13580-020-00312-z
Khan, R. A. A., Tang, Y., Naz, I., Alam, S. S., Wang, W., Ahmad, M., et al. (2021). Management of Ralstonia solanacearum in tomato using ZnO nanoparticles synthesized through Matricaria chamomilla. Plant Dis. 105 (10), 3224–3230. doi:10.1094/PDIS-08-20-1763-RE
Kharde, H. S., Gadhave, S. R., Pawar, G. J., and Ranmale, A. R. (2024). Green synthesis and characterization of zinc oxide nanoparticles by using numerous plants: a review. J. Adv. Sci. Res. 15 (03), 11–14. doi:10.55218/JASR.2024150303
Lau, S. E., Teo, W. F. A., Teoh, E. Y., and Tan, B. C. (2022). Microbiome engineering and plant biostimulants for sustainable crop improvement and mitigation of biotic and abiotic stresses. Discov. Food 2 (1), 9. doi:10.1007/s44187-022-00009-5
Liu, X., Wang, F., Shi, Z., Tong, R., and Shi, X. (2015). Bioavailability of Zn in ZnO nanoparticle-spiked soil and the implications to maize plants. J. Nanopart. Res. 17, 175. doi:10.1007/s11051-015-2989-2
Mahdi, Z. S., Talebnia Roshan, F., Nikzad, M., and Ezoji, H. (2021). Biosynthesis of zinc oxide nanoparticles using bacteria: a study on the characterization and application for electrochemical determination of bisphenol A. Inorg. Nano-Met. Chem. 51 (9), 1–9. doi:10.1080/24701556.2020.1835962
Masri, R., and Kiss, E. (2023). The role of NAC genes in response to biotic stresses in plants. Physiol. Mol. Plant Pathol. 126, 102034. doi:10.1016/j.pmpp.2023.102034
Mazhar, M. W., Ishtiaq, M., Maqbool, M., and Hussain, S. A. (2023). Foliar application of zinc oxide nanoparticles improves rice yield under biotic stress posed by Magnaporthe oryzae. Arch. Phytopathol. Plant Prot. 56 (14), 1093–1111. doi:10.1080/03235408.2023.2267658
Miri, A., Mahdinejad, N., Ebrahimy, O., Khatami, M., and Sarani, M. (2019). Zinc oxide nanoparticles: biosynthesis, characterization, antifungal and cytotoxic activity. Mat. Sci. Eng. C 104, 109981. doi:10.1016/j.msec.2019.109981
Mirzaei, H., and Darroudi, M. (2017). Zinc oxide nanoparticles: biological synthesis and biomedical applications. Ceram. Int. 43 (1), 907–914. doi:10.1016/j.ceramint.2016.10.051
Mohan, N., Ahlawat, J., Sharma, L., Pal, A., Rao, P., Redhu, M., et al. (2023). Engineered nanoparticles a novel approach in alleviating abiotic and biotic stress in millets: a complete study. Plant Stress 10, 100223. doi:10.1016/j.stress.2023.100223
Mohd Yusof, H., Mohamad, R., Zaidan, U. H., and Abdul Rahman, N. A. (2019). Microbial synthesis of zinc oxide nanoparticles and their potential application as an antimicrobial agent and a feed supplement in animal industry: a review. J. animal Sci. Biotechnol. 10, 57–22. doi:10.1186/s40104-019-0368-z
Nandhini, M., Rajini, S. B., Udayashankar, A. C., Niranjana, S. R., Lund, O. S., Shetty, H. S., et al. (2019). Biofabricated zinc oxide nanoparticles as an eco-friendly alternative for growth promotion and management of downy mildew of pearl millet. Crop Prot. 121, 103–112. doi:10.1016/j.cropro.2019.03.015
Narendhran, S., and Sivaraj, R. (2016). Biogenic ZnO nanoparticles synthesized using L. aculeata leaf extract and their antifungal activity against plant fungal pathogens. Bull. Mat. Sci. 39, 1–5. doi:10.1007/s12034-015-1136-0
Naseer, M., Aslam, U., Khalid, B., and Chen, B. (2020). Green route to synthesize Zinc Oxide Nanoparticles using leaf extracts of Cassia fistula and Melia azadarach and their antibacterial potential. Sci. Rep. 10 (1), 9055. doi:10.1038/s41598-020-65949-3
Navale, G. R., Thripuranthaka, M., Late, D. J., and Shinde, S. S. (2015). Antimicrobial activity of ZnO nanoparticles against pathogenic bacteria and fungi. JSM Nanotechnol. Nanomed. 3 (1), 1033.
Nazneen, S., and Sultana, S. (2024). Green synthesis and characterization of Cissus quadrangularis. L stem mediated zinc oxide nanoparticles. Plant Sci. Archives 1 (05). doi:10.5147/PSA.2024.9.1.01
Ogunyemi, S. O., Abdallah, Y., Zhang, M., Fouad, H., Hong, X., Ibrahim, E., et al. (2019). Green synthesis of zinc oxide nanoparticles using different plant extracts and their antibacterial activity against Xanthomonas oryzae pv. oryzae. Artif. Cells Nanomed. Biotechnol. 47 (1), 341–352. doi:10.1080/21691401.2018.1557671
Pachaiappan, R., Rajendran, S., Ramalingam, G., Vo, D. V. N., Priya, P. M., and Soto-Moscoso, M. (2021). Green synthesis of zinc oxide nanoparticles by Justicia adhatoda leaves and their antimicrobial activity. Chem. Eng. Technol. 44 (3), 551–558. doi:10.1002/ceat.202000470
Pandey, P., Irulappan, V., Bagavathiannan, M. V., and Senthil-Kumar, M. (2017). Impact of combined abiotic and biotic stresses on plant growth and avenues for crop improvement by exploiting physio-morphological traits. Front. Plant Sci. 8, 537. doi:10.3389/fpls.2017.00537
Parveen, A., and Siddiqui, Z. A. (2021). Zinc oxide nanoparticles affect growth, photosynthetic pigments, proline content and bacterial and fungal diseases of tomato. Arch. Phytopathol. Plant Prot. 54 (17-18), 1519–1538. doi:10.1080/03235408.2021.1917952
Pillai, A. M., Sivasankarapillai, V. S., Rahdar, A., Joseph, J., Sadeghfar, F., Rajesh, K., et al. (2020). Green synthesis and characterization of zinc oxide nanoparticles with antibacterial and antifungal activity. J. Mol. Struct. 1211, 128107. doi:10.1016/j.molstruc.2020.128107
Priya, N., Kaur, K., and Sidhu, A. K. (2021). Green synthesis: an eco-friendly route for the synthesis of iron oxide nanoparticles. Front. Nanotechnol. 3, 655062. doi:10.3389/fnano.2021.655062
Raj, A., Lawrence, R. S., Jalees, M., and Lawrence, K. (2015). Anti-bacterial activity of zinc oxide nanoparticles prepared from Brassica oleraceae leaves extract. Int. J. Adv. Res. 3 (11), 322–328.
Rajabairavi, N., Raju, C. S., Karthikeyan, C., Varutharaju, K., Nethaji, S., Hameed, A. S. H., et al. (2017). “Biosynthesis of novel zinc oxide nanoparticles (ZnO NPs) using endophytic bacteria Sphingobacterium thalpophilum,” in Recent trends in materials science and applications: nanomaterials, crystal growth, thin films, quantum dots, & spectroscopy (Proceedings ICRTMSA 2016) (Springer International Publishing), 245–254. doi:10.1007/978-3-319-44890-9_23
Rajan, A., Cherian, E., and Baskar, G. (2016). Biosynthesis of zinc oxide nanoparticles using Aspergillus fumigatus JCF and its antibacterial activity. Int. J. Mod. Sci. Technol. 1 (2), 52–57.
Rajiv, P., Rajeshwari, S., and Venckatesh, R. (2013). Bio-Fabrication of zinc oxide nanoparticles using leaf extract of Parthenium hysterophorus L. and its size-dependent antifungal activity against plant fungal pathogens. Spectrochimica Acta Part A Mol. Biomol. Spectrosc. 112, 384–387. doi:10.1016/j.saa.2013.04.072
Ramesh, P., Saravanan, K., Manogar, P., Johnson, J., Vinoth, E., and Mayakannan, M. (2021). Green synthesis and characterization of biocompatible zinc oxide nanoparticles and evaluation of its antibacterial potential. Sens. Bio-Sens. Res. 31, 100399. doi:10.1016/j.sbsr.2021.100399
Selim, Y. A., Azb, M. A., Ragab, I., and Hm Abd El-Azim, M. (2020). Green synthesis of zinc oxide nanoparticles using aqueous extract of Deverra tortuosa and their cytotoxic activities. Sci. Rep. 10 (1), 3445. doi:10.1038/s41598-020-60541-1
Selvaraj, M., N, H., and P.P, V. (2020). In vitro biocompatibility and antimicrobial activities of zinc oxide nanoparticles (ZnO NPs) prepared by chemical and green synthetic route— a comparative study. BioNanoSci 10, 112–121. doi:10.1007/s12668-019-00698-w
Shams, A. H., Helaly, A. A., Algeblawi, A. M., and Awad-Allah, E. F. (2023). Efficacy of seed-biopriming with trichoderma spp. and foliar spraying of ZnO-nanoparticles induce cherry tomato growth and resistance to Fusarium wilt disease. Plants 12 (17), 3117. doi:10.3390/plants12173117
Sharma, J. L., Dhayal, V., and Sharma, R. K. (2021). White-rot fungus mediated green synthesis of zinc oxide nanoparticles and their impregnation on cellulose to develop environmental friendly antimicrobial fibers. 3 Biotech. 11 (6), 269. doi:10.1007/s13205-021-02840-6
Shaw, A. K., and Hossain, Z. (2013). Impact of nano-CuO stress on rice (Oryza sativa L.) seedlings. Chemosphere 93 (6), 906–915. doi:10.1016/j.chemosphere.2013.05.044
Sidhu, A. K., Verma, N., and Kaushal, P. (2022). Role of biogenic capping agents in the synthesis of metallic nanoparticles and evaluation of their therapeutic potential. Front. Nanotechnol. 3, 801620. doi:10.3389/fnano.2021.801620
Singh, A., Singh, N. Á., Afzal, S., Singh, T., and Hussain, I. (2018). Zinc oxide nanoparticles: a review of their biological synthesis, antimicrobial activity, uptake, translocation and biotransformation in plants. J. Mat. Sci. 53 (1), 185–201. doi:10.1007/s10853-017-1544-1
Singh, B. K., Delgado-Baquerizo, M., Egidi, E., Guirado, E., Leach, J. E., Liu, H., et al. (2023). Climate change impacts on plant pathogens, food security and paths forward. Nat. Rev. Microbiol. 21, 640–656. doi:10.1038/s41579-023-00900-7
Sirelkhatim, A., Mahmud, S., Seeni, A., Kaus, N. H. M., Ann, L. C., Bakhori, S. K. M., et al. (2015). Review on zinc oxide nanoparticles: antibacterial activity and toxicity mechanism. Nano-micro Lett. 7, 219–242. doi:10.1007/s40820-015-0040-x
Sofy, A. R., Sofy, M. R., Hmed, A. A., Dawoud, R. A., Alnaggar, A. E. A. M., Soliman, A. M., et al. (2021). Ameliorating the adverse effects of tomato mosaic tobamovirus infecting tomato plants in Egypt by boosting immunity in tomato plants using zinc oxide nanoparticles. Molecules 26 (5), 1337. doi:10.3390/molecules26051337
Song, U., and Kim, J. (2020). Zinc oxide nanoparticles: a potential micronutrient fertilizer for horticultural crops with little toxicity. Hortic. Environ. Biotechnol. 61, 625–631. doi:10.1007/s13580-020-00244-8
Sumanth, B., Lakshmeesha, T. R., Ansari, M. A., Alzohairy, M. A., Udayashankar, A. C., Shobha, B., et al. (2020). Mycogenic synthesis of extracellular zinc oxide nanoparticles from Xylaria acuta and its nanoantibiotic potential. Int. J. Nanomedicine 15, 8519–8536. doi:10.2147/IJN.S271743
Thi, T. U. D., Nguyen, T. T., Thi, Y. D., Thi, K. H. T., Phan, B. T., and Pham, K. N. (2020). Green synthesis of ZnO nanoparticles using orange fruit peel extract for antibacterial activities. RSC Adv. 10 (40), 23899–23907. doi:10.1039/D0RA04926C
Tortella, G., Rubilar, O., Pieretti, J. C., Fincheira, P., de Melo Santana, B., Fernández-Baldo, M. A., et al. (2023). Nanoparticles as a promising strategy to mitigate biotic stress in agriculture. Antibiotics 12 (2), 338. doi:10.3390/antibiotics12020338
UN (2015). The 2030 agenda for sustainable development. Available at: https://sdgs.un.org/goals (Accessed February 03, 2024).
Verma, N., Kaushal, P., Gahalot, D., Sidhu, A. K., and Kaur, K. (2023). Mechanistic aspect of zinc oxide nanoparticles in alleviating abiotic stress in plants—a sustainable agriculture approach. BioNanoScience 13 (4), 1645–1661. doi:10.1007/s12668-023-01192-0
Vithalani, P., and Bhatt, N. (2024). Green synthesis of zinc oxide nanoparticles using allium sativum plant extract and photocatalytic activity of rhodamine b green synthesis of zinc oxide nanoparticles using allium sativum plant extract and photocatalytic activity of rhodamine B. Environ. Ecol. 42 (2), 374–382. doi:10.60151/envec/KSHT7592
Wang, X. P., Li, Q. Q., Pei, Z. M., and Wang, S. C. (2018). Effects of zinc oxide nanoparticles on the growth, photosynthetic traits, and antioxidative enzymes in tomato plants. Biol. Plant. 62, 801–808. doi:10.1007/s10535-018-0813-4
WIPO (2024). Patent analysis. Available at: https://patentscope.wipo.int/search/en/result.jsf?_vid=P11-LS5W5W-53175 (Accessed February 03, 2024).
Wu, C., Zhang, T., Ji, B., Chou, Y., and Du, X. (2024). Green synthesis of zinc oxide nanoparticles using Aloe vera leaf extract and evaluation of the antimicrobial and antioxidant properties of the ZnO/regenerated cellulose film. Cellulose 31, 4849–4864. doi:10.1007/s10570-024-05914-9
Yadav, A., Jangid, N. K., and Khan, A. U. (2024). Biogenic synthesis of ZnO nanoparticles from Evolvulus alsinoides plant extract. J. Umm Al-Qura Univ. Appl. Sci. 10 (1), 51–57. doi:10.1007/s43994-023-00076-z
Keywords: zinc oxide nanoparticle, green synthesis, antimicrobial activity, biotic stress, agriculture, toxicity
Citation: Verma N, Kaushal P and Sidhu AK (2024) Harnessing biological synthesis: Zinc oxide nanoparticles for plant biotic stress management. Front. Chem. 12:1432469. doi: 10.3389/fchem.2024.1432469
Received: 14 May 2024; Accepted: 20 June 2024;
Published: 11 July 2024.
Edited by:
Mahendra Rai, Sant Gadge Baba Amravati University, IndiaReviewed by:
Shiva Prasad Kollur, Amrita Vishwa Vidyapeetham, IndiaMehran Alavi, Razi University, Iran
Copyright © 2024 Verma, Kaushal and Sidhu. This is an open-access article distributed under the terms of the Creative Commons Attribution License (CC BY). The use, distribution or reproduction in other forums is permitted, provided the original author(s) and the copyright owner(s) are credited and that the original publication in this journal is cited, in accordance with accepted academic practice. No use, distribution or reproduction is permitted which does not comply with these terms.
*Correspondence: Amanpreet K. Sidhu, YW1hbi5wcmVldDE4MDdAZ21haWwuY29t