- 1National Institute for Agrarian and Veterinariay Research (INIAV), Vairão, Portugal
- 2Veterinary and Animal Research Centre (CECAV), University of Trás-os-Montes and Alto Douro, Vila Real, Portugal
- 3Centre of Biological Engineering, University of Minho, Braga, Portugal
- 4LABBELS–Associate Laboratory, Braga, Portugal
- 5LEPABE—Laboratory for Process Engineering, Environment, Biotechnology and Energy, Faculty of Engineering, University of Porto, Porto, Portugal
- 6ALiCE—Associate Laboratory in Chemical Engineering, Faculty of Engineering, University of Porto, Porto, Portugal
Enterotoxigenic Escherichia coli (ETEC) is the major bacterial cause of diarrheal diseases in pigs, particularly at young ages, resulting in significant costs to swine farming. The pathogenicity of ETEC is largely dependent on the presence of fimbriae and the ability to produce toxins. Fimbriae are responsible for their initial adhesion to the intestinal epithelial cells, leading to the onset of infection. In particular, the F4 type (K88) fimbriae are often attributed to neonatal infections and have also been associated with post-weaning diarrheal infections. This disease is traditionally prevented or treated with antibiotics, but their use is being severely restricted due to the emergence of resistant bacteria and their impact on human health. Emerging approaches such as aptamers that target the F4-type fimbriae and block the initial ETEC adhesion are a promising alternative. The aim of this study is to assess the effectiveness of two aptamers, Apt31 and Apt37, in controlling ETEC infection in the G. mellonella in vivo model. Initially, the dissociation constant (KD) of each aptamer against ETEC was established using real-time quantitative PCR methodology. Subsequently, different concentrations of the aptamers were injected into Galleria mellonella to study their toxicity. Afterwards, the anti-ETEC potential of Apt31 and Apt37 was assessed in the larvae model. The determined KD was 81.79 nM (95% CI: 31.21–199.4 nM) and 50.71 nM (95% CI: 26.52–96.15 nM) for the Apt31 and Apt37, respectively, showing no statistical difference. No toxicity was observed in G. mellonella following injection with both aptamers at any concentration. However, the administration of Apt31 together with ETEC-F4+ in G. mellonella resulted in a significant improvement of approximately 30% in both larvae survival and health index compared to ETEC-F4+ alone. These findings suggest that aptamers have promising inhibitory effect against ETEC infections and pave the way for additional in vivo studies.
1 Introduction
Swine enteric colibacillosis is a disease characterized by an intestinal infection caused by the colonization of enterotoxigenic Escherichia coli (ETEC). This infection mostly causes illness or death in neonatal and weaned pigs, making it responsible for significant economic losses worldwide (Castro et al., 2022). Porcine ETEC pathotypes are characterized by the expression of specific fimbriae adhesins that mediate bacterial colonization of the intestinal mucosal surface. When both the immunological systems and the gut microbiota are poorly developed, ETEC colonizes and produce heat-labile (LT) and/or heat-stable (ST, including STa/STb subtypes) enterotoxins that can have local and systemic effects (Barros et al., 2023; Luppi, 2017). Some pathotypes of Shiga toxin (Stx or VT), namely, type 2e (Stx2e), are also frequently found in ETEC strains (Luppi et al., 2016). The most commonly detected fimbriae in ETEC are F4 (previously known as K88) and F18 (García et al., 2020; Luise et al., 2019). Intestinal colonization and disease caused by ETEC depend on F4 or F18-specific receptors. ETEC-F4 is usually associated with post-weaning diarrhea (PWD) in recently weaned piglets occurring 2–3 days after weaning, whereas F18 is commonly found in association with diarrhea 2–6 weeks after weaning (Luise et al., 2019). The age-dependent expression of F4 and F18 receptors in the small intestine may explain why ETEC-F4+ infection mainly occurs immediately after weaning and during the neonatal period, whereas ETEC-F18+ infection mainly occurs later in the post-weaning period (Luppi, 2017; Luppi et al., 2016).
PWD is usually prevented or treated with antibiotics, but, in recent years, their use has been highly restricted due to the growing phenomenon of antimicrobial resistance (Barros et al., 2023). It is therefore important to begin the transition to other, more sustainable, approaches. In this regard, novel strategies such as aptamers, which are small single-stranded oligonucleotides capable of binding to target molecules, seem to be a promising alternative to block the initial adhesion mediated by fimbriae in ETEC. Li and colleagues reported two aptamers, Apt31 and Apt37, for ETEC with F4-type fimbriae capable of specifically detecting these bacteria (Li et al., 2011). The SELEX methodology followed directed the selection towards the fimbriae protein and demonstrated the applicability of the selected aptamers as diagnostic recognition molecules (Li et al., 2011). Although the binding model between the aptamers and the F4 fimbriae has not been studied, the observed interaction means that the aptamers, after binding, have a spatial significance in the potential additional binding of the ETECs. Of note that it has been described that the interaction of aptamers with their targets results mainly from the compatibility of physical structures and/or the stacking of chemical groups stabilized by hydrogen bonds, electrostatic interactions, hydrophobic effects, π-π stacking, van der Waals forces, or combinations of these different forces (Oliveira et al., 2022). Therefore, the physicochemical interaction can block receptor-binding domains of the fimbriae from interacting with receptors and, thus, neutralize their role in the initial adhesion of ETEC strains and consequently the development of infection (Keefe et al., 2010; Zhou and Rossi, 2017).
However, until now the potential of aptamers to block ETEC-mediated infections in animal models has not been evaluated. As a proof of concept, the present study focuses on evaluating the anti-ETEC potential of two aptamers previously described in the literature, Apt31 and Apt37 (Li et al., 2011), using an in vivo G. mellonella larval model (Ferreira et al., 2023). It is important to highlight that Galleria mellonella larvae have gained attention as an alternative model to study bacterial infections due to their genetic similarity to mammals and their convenience and cost-effectiveness compared to traditional mammalian models (Ménard et al., 2021). Additionally, the immune response of G. mellonella shares similarities with mammalian innate immunity, making it a suitable model for evaluating potential therapeutic interventions (Tsai et al., 2016).
2 Materials and methods
2.1 Strains and culture maintenance
Two ETEC strains, SP11 and SP31, which encompass the most predominant fimbriae linked to PWD, namely, F4 and F18, were included in this study. Strain SP11 encodes F4, along with the enterotoxins STb and LT, while strain SP31 encodes F18 and the enterotoxin Stx2e. Other additional bacterial strains were included herein as controls: E. coli strain K12 (wild-type); Klebsiella pneumoniae strain ATCC 43816 and Staphylococcus aureus strain ATCC 25923. All bacterial strains were grown on Tryptic Soy Agar (TSA) (Liofilchem, Roseto degli Abruzzi, Italy) for 18–24 h at 37°C and cryopreserved at −80°C in cryovials composed of Tryptic Soy Broth (TSB) (Merck, Darmstadt, Germany) and 20% (v/v) of Glycerol (Biochem Chemopharma, Cosne-Cours-sur-Loire, France).
2.2 Determination of KD of Apt31 and Apt37 against ETEC-F4+
The dissociation constant (KD) was determined for the two selected DNA aptamers (Apt31, Apt37) with proven affinity for the F4-fimbriae type, considering the selection conditions reported by the authors (Li et al., 2011). Their sequence (depicted in Table 1) is composed of two primer sites on both sides with 18 nucleotides and a central portion with 60 nucleotides that distinguish both aptamers. The main objective of this step was to establish a comparison between the reported KD and the one obtained using the qPCR methodology used by our group. Briefly, it was used a binding buffer (BB) containing 138 mM NaCl (Biochem Chemopharma), 20 mM Tris-HCL pH 7.4 (NZYtech, Lisbon, Portugal), 2.7 mM KCl (Merck), 1 mM CaCl2 (Scharlau, Barcelona, Spain), 0.5 mM MgCl2 (Merck), and 0.1 mg mL-1 of DNA salmon sperm (Invitrogen, Waltham, United States), and a washing buffer (WS) composed of BB+ 0.05% (v/v) Tween 20 (VWR, Portland, United States) to guarantee the reported physicochemical conditions to aptamers functionality. At first, 10 nM concentrations (400, 300, 200, 150, 100, 50, 10, 5, 1, 0) of both aptamers (Eurogentec, Seraing, Belgium) were prepared with BB in 1.5 mL low binding microcentrifuge tubes, then heated at 95°C for 5 min, cooled in ice for 10 min, and lastly maintained at room temperature for 15 min. After, the mentioned concentrations of aptamers (100

Table 1. Single-stranded DNA aptamers sequence (5′- 3′) of Apt31 and Apt37 according to Li et al., 2011.
To quantify the bound aptamer in the eluted samples, a real-time quantitative PCR (qPCR) methodology using SYBR green was conducted with the following conditions: initial polymerase activation/denaturation (95.0°C, 2 min); 25 amplification cycles (denaturation at 95°C, 5 s; annealing at 60°C, 15 s). At the end of each cycle, a SYBR green read was performed. For this protocol, 20 μL reactions containing 1× NZYSupreme qPCR Green Master (NZYTech), 0.4
The KD was determined by a non-linear regression analysis
2.3 Evaluation of aptamers toxicity in Galleria mellonella model
Larval survival experiments were adapted with minor modifications from previous studies (Araújo et al., 2022; Ferreira et al., 2023). The larvae were maintained on a diet of pollen grains and bees wax at 25°C in the darkness. Worms of the last instar, weighing approximately 250 mg, were selected for the following experiments. The toxicity of Apt31 and Apt37 was evaluated by injecting a range of concentrations (1µM, 10 μM, 20 µM). A control of PBS 1× was also included herein. For each condition tested, each larva (n = 10 × 4 groups) was injected with 5 µL of suspension into the haemolymph via the hindmost left proleg, previously sanitized with 70% (v/v) of ethanol, using a micro syringe. After the injections, the larvae were placed in Petri dishes and stored in the dark at 37°C. Survival was monitored for 4 days (each 24 h) and if the larvae did not move in response to touch, they were considered dead. The G. mellonella health index, which assesses four main parameters: larval activity, cocoon formation, melanization, and survival, classified with a score determined in a previous study (Loh et al., 2013). First, larval survival is analysed, if the larva is alive it is scored as 2, if it is dead it is scored as 0. In terms of larval activity, larvae are scored between 0 and 3, where three means that larvae show movement without stimulation and 0 means that larvae do not show any movement. Cocoon formation is scored between 0 and 1, where 1 is a full cocoon and 0 is no cocoon. Melanisation is scored between 1 and 4, where 4 is given if the larva retains its original creamy colour and 1 if the larva is completely dark. All experiments were performed in triplicate and at least three independent assays were performed. Ethical approval is not required for the study of this animal model.
2.4 Evaluation of the anti-ETEC potential of aptamers in infected Galleria mellonella
The effectiveness of each aptamer, Apt31 and Apt37, in controlling ETEC infection was evaluated using also the G. mellonella model. For that, ETEC strains, SP11 and SP31, were grown in TSB at 37°C and 120 rpm, and in the exponential phase, the cells were centrifuged, washed, and suspended in PBS (pH 7), and the cell density was adjusted to the final concentration of 108 CFU mL-1 in PBS. The cell suspensions were normalized using OD600nm and the CFU. mL-1. Other bacterial strains (E. coli strain K12; K. pneumoniae and S. aureus) were also included in the assay as controls, using the same concentration of 108 CFU mL-1 in PBS. Per bacterial strain, each larva (n = 10 × 4 groups) was injected with 5 µL of suspension into the haemolymph via the hindmost as described in section 2.3. Of note that each set of larvae was injected with the Apt31 or Apt37 together with each bacterial strain individually (final concentration 500 nM) (Soundy and Day, 2020). As a control negative, one group of larvae was injected with the same volume of PBS only, while another group with bacteria only (positive control). Survival over time and G. mellonella health index were evaluated as described in the previous section. To investigate the potential of Apt31 and Apt37 to control the progression of bacterial infection, some larvae infected with the SP11 strain and treated with both aptamers were sacrificed at 48 and 72 h for histological processing. The fat body of each larva was removed by a ventral midline incision with a scalpel blade. The fat bodies were placed in 10% (v/v) neutral buffered formalin (PanRaec AppliChem, Barcelona, Spain) and stored at 4°C for 24 h to fix the structures. Paraffin blocks were sectioned at 4–5 µm using a microtome (Accu-Cut® SRM™, Labometer, Lisbon, Portugal) and the sections were stained with the periodic acid Schiff (PAS) (Bio-Optica Milano spa, Milan, Italy). Tissue sections were observed and photographed using a Leica DM1000 LED microscope coupled to a digital camera (Leica, Microsystems, Wetzlar, Germany).
2.5 Statistical analysis
The KD experiments were performed in duplicate with two independent assays, using the following equation,
3 Results
3.1 KD values for Apt31 and Apt37 against ETEC-F4+
The kinetic parameters of the two aptamers, Apt31 and Apt37, with a reported affinity for F4-fimbriae were determined by the qPCR methodology (Li et al., 2011). The binding data for the aptamer concentrations tested (0–400 nM) against a fixed concentration of ETEC strain SP11 suspensions (
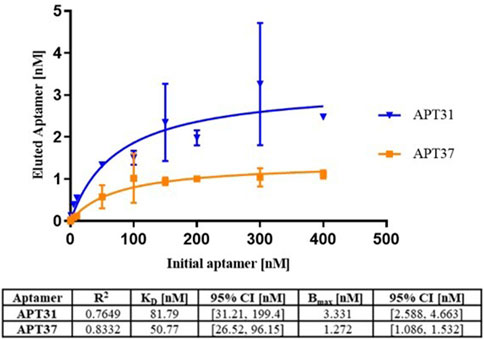
Figure 1. Values of the dissociation constant (KD) for Apt31 (represented in blue) and for Apt37 (represented in orange). The information in the table below reports the R-squared (R2), dissociation constant (KD) and maximum specific binding (Bmax) with the corresponding 95% confidence interval.
3.2 Assessment of anti-ETEC potential of Apt31 and Apt37 using the Galleria mellonella larval in vivo model
First, G. mellonella larvae were injected with different concentrations of Apt31 and Apt37 to assess their respective levels of toxicity. Based on data shown in Figures 2, 3, no toxicity in the G. mellonella model was evidenced for both aptamers evaluated (Apt31, Apt37). In fact, no effect was observed on the larval health index and larval survival up to 96 h post-injection for any of the concentrations of each aptamer tested.
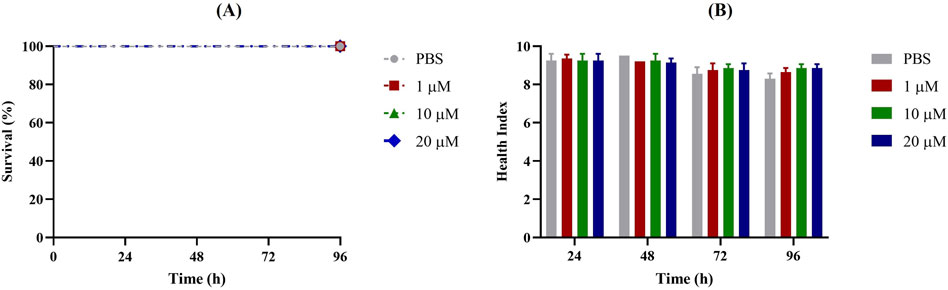
Figure 2. Toxicity of Apt31 of non-infected Galleria mellonella. Survival curves (A) and health index score (B) of G. mellonella administrated with 1, 10 and 20 μM of aptamer concentrations. As a negative control, larvae were injected only with PBS.
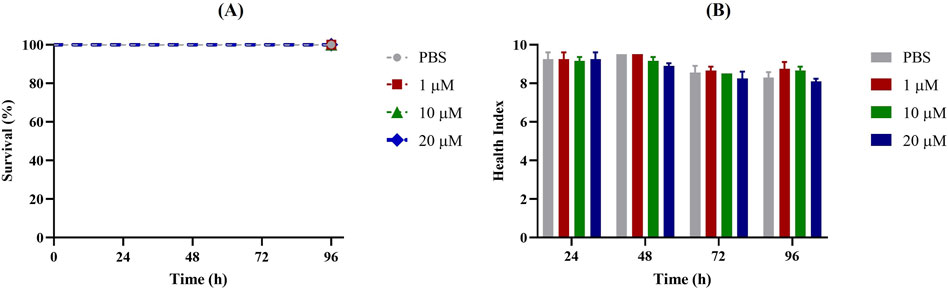
Figure 3. Toxicity of Apt37 of non-infected Galleria mellonella. Survival curves (A) and health index score (B) of G. mellonella administrated with 1, 10 and 20 μM of aptamer concentrations. As a negative control, larvae were injected only with PBS.
Afterwards, the G. mellonella larvae model was used to study in vivo the effects of Apt31 and Apt37 against ETEC-F4+ infection. For that, G. mellonella infected with ETEC strains (SP11; SP31) and other bacterial species (E. coli K12; S. aureus; K. pneumoniae) were treated with both aptamers at a concentration of 500 nM. It is important to remark that each set of larvae was injected with the Apt31 or Apt37 together with each bacterial strain individually. Figure 4 shows the results obtained after 72 h of post-infection for 3 E. coli strains, two ETECs (Figures 4A, B) and one no-pathogenic strain (Figure 4C). In G. mellonella infected with E. coli strain SP11 (F4-fimbriae type), it is noteworthy that a single dose of Apt31 enhances larval survival by 33% over 72 h (p < 0.05) (Figure 4A). In contrast, when the larvae were treated with Apt37, a slight decrease in larvae survival was observed, but this difference is not statistically different from G. mellonella infected with SP11 alone or SP31 alone (Figures 4A, B, respectively). However, such an effect was not due to the toxicity of the aptamer, as it alone does not show any toxicity up to 20
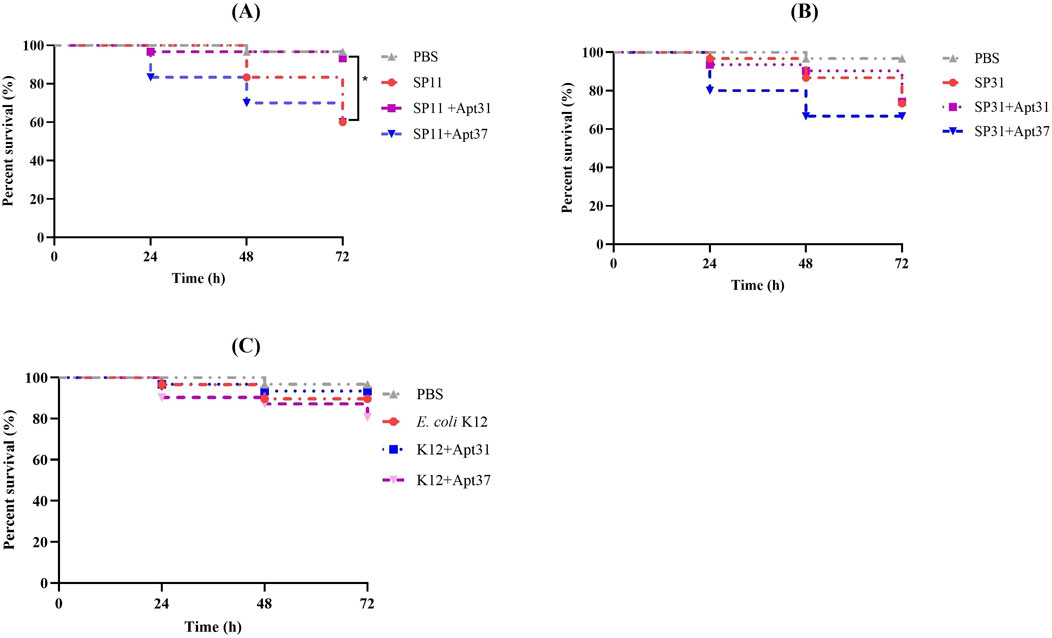
Figure 4. Effect of the DNA aptamers on the survival of infected Galleria mellonella. Survival curves of G. mellonella treated with 500 nM of Apt31 and Apt37. Larvae were infected with ETEC strain SP11 (A), ETEC strain SP31 (B), Escherichia coli strain K12 (C). As a negative control, larvae were injected only with PBS. *Significant difference between positive control (larvae infected only with bacteria) and treated with aptamers (p < 0.05).
The G. mellonella health index was also determined, through the evaluation of larval activity, cocoon formation, melanization, and survival. The results are presented in Figure 5. It should be noted that when G. mellonella was infected with SP11 and treated with Apt31, the health index increased after 48 h (by around 17%) and 72 h (by around 30%) (p < 0.05), which corroborates the observed survival rates (Figure 4). In the case of SP31, when G. mellonella was treated with Apt31, no differences were observed compared to the control (Figure 5B). In contrast, with Apt37, a decrease is observed in the larvae health index for all times tested (p < 0.05) (Figure 5B). For the wild-type strain, E. coli K12, the health index in the larvae injected only with bacteria and with aptamers was similar to the PBS, emphasizing the lower virulence related to this strain (Figure 5C). However, it can be seen that in the case of Apt37, the health index was significantly lower compared to the positive control (p < 0.05), revealing that Apt37 does not have a positive effect against E. coli. For the other species tested, S. aureus and K. pneumoniae, the health index in the bacteria control was extremely low. For S. aureus, no differences were observed between the aptamers and bacteria control over 72 h of infection (Supplementary Figure S2A). For G. mellonella infection with K. pneumoniae, no differences were observed between control and aptamer treatment at any time point tested, except at 24 h when Apt37 was used to treat G. mellonella infection (p < 0.05) (Supplementary Figure S2B).
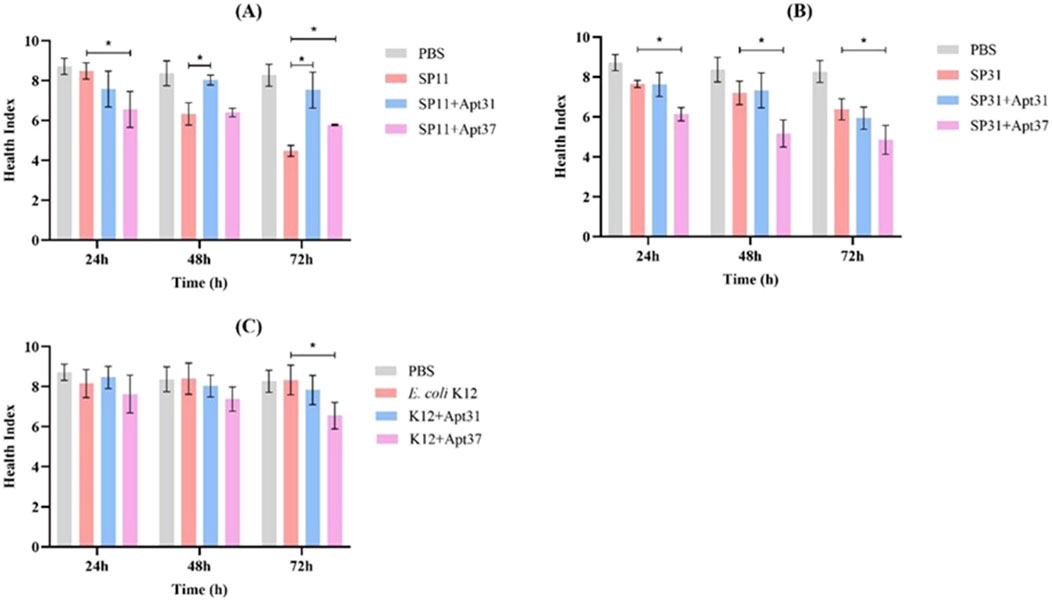
Figure 5. Effect of the DNA aptamers on the health index scores of infected Galleria mellonella. Larvae were infected with ETEC strain SP11 (A), ETEC strain SP31 (B), Escherichia coli strain K12 (C), and treated with 500 nM of Apt31 and Apt37. As a negative control, larvae were injected only with PBS. *Significant difference among positive control (larvae infected only with bacteria) and treated with aptamers (p < 0.05).
The progression of the effect of the aptamers on SP11 infection was also evaluated by observation of the fat body of G. mellonella after histological procedures, as shown in Figure 6. The presence of a bacterial agglomerated system can be observed in the fatty body of SP11-infected larvae after 72 h without aptamer treatment. Additionally, the histological images showed a significant reduction in both the quantity and size of SP11 agglomerates following 48 and 72 h of Apt31 treatment. In the case of Apt37, a huge quantity of SP11 was observed in the fat body after 72 h, which corroborates the survival rates (Figure 4) and health index (Figure 5) results obtained, revealing the lack of efficacy of this aptamer to control the SP11 infection.
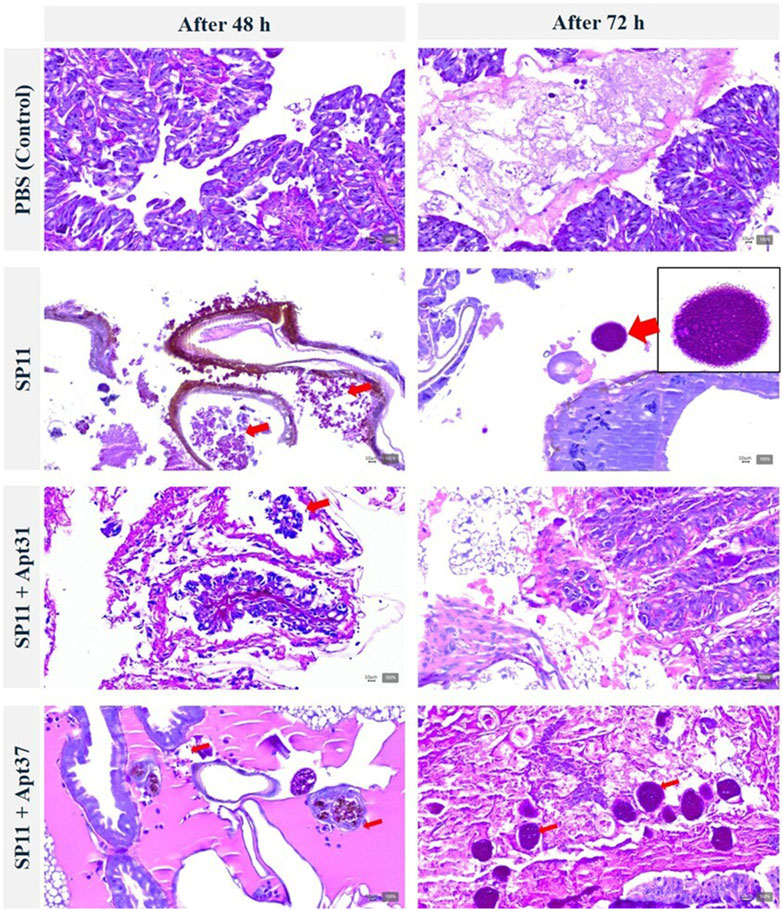
Figure 6. Effect of DNA aptamers on the progression of ETEC SP11 infection in Galleria mellonella fat body. Histological images of larvae infected with SP11 (at 48 and 72 h) and treated with Apt31 and Apt37. The larvae sections were labeled with periodic acid Schiff coloration. Red arrows are an example of ETEC colonization. The magnification images were at ×200.
4 Discussion
The rise of antibiotic resistance among bacterial pathogens, including ETEC, poses a serious threat to public health. Traditional antibiotic treatments for ETEC infections are becoming less effective due to the emergence of resistant strains (Christaki et al., 2020). Therefore, alternative approaches to combat bacterial-associated infections are urgently needed to tackle ETEC-associated morbidity and mortality without contributing to the spread of antibiotic resistance.
Over the last decade, aptamers emerged as a promising tool for diagnostic or even therapeutic purposes (Afrasiabi et al., 2020; Araújo et al., 2024). Aptamers therapeutic effect has been demonstrated for other bacterial pathogens, with promising results that could eventually give rise to new drugs in the future. For instance, aptamers for S. aureus (Ommen et al., 2022), Pseudomonas aeruginosa (Wang et al., 2018) and Streptococcus spp. (Hosseini et al., 2022) have proven that their direct administration and/or aptamer-targeted drug delivery is able to inhibit the formation of biofilms and the development of infections (Araújo et al., 2024). In addition, several aptamers evolved for diagnostic purposes, that target relevant virulence features of bacterial pathogens, might also present potential as therapeutic molecules (Nimjee et al., 2017). This work took advantage of this and selected aptamers previously applied in the detection of F4-fimbriae protein that could also be applied as a biomolecular solution against disease caused by ETEC-F4+ bacteria. Since F4-fimbriae are involved in the initial attachment to the epithelial cell wall of the small intestine, assisting the bacteria in anchoring and proliferating, the aptamers can block the progression of ETEC-F4+ infection by inhibiting the anchoring process (Li et al., 2011).
In this study, we propose to investigate the efficacy of DNA aptamers in blocking ETEC toxicity using the G. mellonella larval model in vivo. By exploiting the unique properties of aptamers, such as their high binding affinity and specificity, we aimed to selectively interfere with critical virulence factors produced by ETEC, thereby attenuating its pathogenic effects. It is important to highlight that the aptamers used herein, Apt31 and Apt37 were obtained from a SELEX (Systematic Evolution of Ligands by Exponential Enrichment) procedure carried out by Li and colleagues (Li et al., 2011). These aptamers were selected directly for the pure F4-fimbrial protein, and the KD values and specificity were previously reported.
The re-evaluation of the KD values of the aptamers with our methodology against the whole bacterial cell (Figure 1) showed that both aptamers, Apt31 and Apt37, have a high binding affinity with values of 81.79 nM (95% CI: 31.21–199.4 nM) and 50.71 nM (95% CI: 26.52–96.15 nM), respectively. KD of both aptamers (Apt31, Apt37) to these bacterial cells was not statistically different (p > 0.05) and did not differ statistically from the reported KD values determined using pure F4 fimbriae, in which Apt31 has KD of 36 ± 8 nM whereas Apt37 has 25 ± 4 nM (Li et al., 2011). Even so, the variance in the KD values between our method and those in the original work may be attributed to the differences in the target employed. The original work used fimbrial protein F4 isolate, whereas we used the whole cell of ETEC-F4 type. Additionally, discrepancies may arise from slight variations in methodology, as well as in biological and physicochemical conditions. It is also important to note that the KD determination methodology used in this study uses whole bacteria as a target, so there is a possibility that part of the aptamer quantified, after elution, was result of internalization and not just binding to the F4-fimbrial protein present on the membrane. Even so, this portion of the quantified aptamer should be reduced, since the direct internalization of nucleic acids in prokaryotic cells, especially in Gram-negative bacteria and unlike eukaryotic cells, is very limited, due to the complexity of the membrane and the lack of internalization mechanisms (Benizri et al., 2019; Kauss et al., 2020; Readman et al., 2017).
The research findings revealed that the Apt31 was the sole candidate to demonstrate a positive impact in vivo on the G. mellonella model. It increased the survival rate (Figure 4) and improved the health index of larvae (Figure 5) by around 30%, while also reducing the density of cells present through the fat body (Figure 6). These results suggest a potential higher level of pharmacokinetic activity (Nimjee et al., 2017). Regarding Apt37, the results were unexpected, as Apt37 had a more detrimental effect on G. mellonella compared to SP11 (Figure 4A) or SP31 alone (Figure 4B). Further research is crucial to uncover the mechanism behind the aptamer-bacterial cell interaction.
As a correlation between Apt31 treatment and improved survival of infected larvae was observed, this study suggests that aptamers may be a promising treatment option for ETEC infections in mammals. Even so, being a DNA aptamer, its stability (half-life) in vivo will be reduced (perhaps to minutes), since susceptibility to enzymatic degradation by nucleases present in practically all biological environments is one of the main limitations of the application of aptamers in therapeutic solutions. The future development of an anti-ETEC solution with these aptamers will require post-SELEX improvement with nucleic acid mimics (NAMs) to increase their chemical and biological stability and guarantee their functionality in vivo. Even so, this study shows that by targeting key virulence factors, aptamers can effectively neutralize bacterial pathogenicity offering a promising approach for the treatment of ETEC-related diarrheal illness (Ferreira et al., 2023). These findings highlight the potential of aptamers as emerging biotechnological approaches for combating bacterial infections.
5 Conclusion
In conclusion, this study highlights the promise of aptamers as a novel inhibitory strategy to control ETEC infections and underscores the significance of using alternative model systems like G. mellonella larvae for preclinical evaluation of bacterial agents. Apt31 established a proof-of-concept of the efficiency of aptamers to fight against ETEC in our tested conditions. Nevertheless, further research is necessary for making aptamer endure the gastric passage, being delivered in the intestinal lumen of the piglets. Additionally, investigating the pharmacokinetics and immunogenicity of aptamers in mammalian models will be crucial for evaluating their translational potential as therapeutic agents.
Data availability statement
The original contributions presented in the study are included in the article/Supplementary Material, further inquiries can be directed to the corresponding author.
Author contributions
MB: Conceptualization, Data curation, Investigation, Methodology, Writing–original draft, Writing–review and editing. JC: Conceptualization, Data curation, Investigation, Methodology, Supervision, Validation, Writing–original draft, Writing–review and editing. DA: Conceptualization, Data curation, Investigation, Methodology, Supervision, Validation, Writing–original draft, Writing–review and editing. RO: Conceptualization, Data curation, Investigation, Methodology, Supervision, Validation, Writing–original draft, Writing–review and editing. AC: Investigation, Methodology, Writing–original draft, Writing–review and editing. SS: Conceptualization, Data curation, Methodology, Supervision, Writing–original draft, Writing–review and editing. DO-M: Conceptualization, Supervision, Writing–original draft, Writing–review and editing. CA: Conceptualization, Data curation, Funding acquisition, Investigation, Methodology, Project administration, Resources, Supervision, Validation, Writing–original draft, Writing–review and editing.
Funding
The author(s) declare that financial support was received for the research, authorship, and/or publication of this article. This work was financially supported by: APTAcoli (PTDC/CVT-CVT/4620/2021), funded by FEDER funds through COMPETE2020–Programa Operacional Competitividade e Internacionalização (POCI); national funds through FCT/MCTES (PIDDAC): LEPABE, UIDB/00511/2020 (DOI: 10.54499/UIDB/00511/2020) and UIDP/00511/2020 (DOI: 10.54499/UIDP/00511/2020) and ALiCE, LA/P/0045/2020 (DOI: 10.54499/LA/P/0045/2020), and by FCT under the scope of the strategic funding of UIDB/04469/2020 unit (CEB). MB and AC thank FCT for the PhD Grants, 2023.04664.BDANA and 2023.03705.BDANA, respectively. JC also thanks FCT for the CEEC Individual (https://doi.org/10.54499/2022.06886.CEECIND/CP1737/CT0001).
Acknowledgments
The authors would like to acknowledge the company ALS Life Sciences Portugal, S.A. for providing the ETEC strains designated by SP11 and SP31.
Conflict of interest
The authors declare that the research was conducted in the absence of any commercial or financial relationships that could be construed as a potential conflict of interest.
The author(s) declared that they were an editorial board member of Frontiers, at the time of submission. This had no impact on the peer review process and the final decision.
Publisher’s note
All claims expressed in this article are solely those of the authors and do not necessarily represent those of their affiliated organizations, or those of the publisher, the editors and the reviewers. Any product that may be evaluated in this article, or claim that may be made by its manufacturer, is not guaranteed or endorsed by the publisher.
Supplementary material
The Supplementary Material for this article can be found online at: https://www.frontiersin.org/articles/10.3389/fchem.2024.1425903/full#supplementary-material
References
Afrasiabi, S., Pourhajibagher, M., Raoofian, R., Tabarzad, M., and Bahador, A. (2020). Therapeutic applications of nucleic acid aptamers in microbial infections. J. Biomed. Sci. 27 (1), 6. doi:10.1186/S12929-019-0611-0
Araújo, D., Mil-Homens, D., Henriques, M., and Silva, S. (2022). Anti-EFG1 2′-OMethylRNA oligomer inhibits Candida albicans filamentation and attenuates the candidiasis in Galleria mellonella. Mol. Ther. - Nucleic Acids 27, 517–523. doi:10.1016/j.omtn.2021.12.018
Araújo, D., Silva, A. R., Fernandes, R., Serra, P., Barros, M. M., Campos, A. M., et al. (2024). Emerging approaches for mitigating biofilm-formation-associated infections in farm, wild, and companion animals. Pathogens 13 (4), 320. doi:10.3390/PATHOGENS13040320
Barros, M. M., Castro, J., Araújo, D., Campos, A. M., Oliveira, R., Silva, S., et al. (2023). Swine colibacillosis: global epidemiologic and antimicrobial scenario. Antibiot. (Basel). 12 (4), 682. doi:10.3390/antibiotics12040682
Benizri, S., Gissot, A., Martin, A., Vialet, B., Grinstaff, M. W., and Barthélémy, P. (2019). Bioconjugated oligonucleotides: recent developments and therapeutic applications. Bioconjugate Chem. 30 (2), 366–383. doi:10.1021/acs.bioconjchem.8b00761
Castro, J., Margarida Barros, M., Araújo, D., Maria Campos, A., Oliveira, R., Silva, S., et al. (2022). Swine enteric colibacillosis: current treatment avenues and future directions. Front. Veterinary Sci. 9, 981207. doi:10.3389/fvets.2022.981207
Christaki, E., Marcou, M., and Tofarides, A. (2020). Antimicrobial resistance in bacteria: mechanisms, evolution, and persistence. J. Mol. Evol. 88 (1), 26–40. doi:10.1007/S00239-019-09914-3
Ferreira, A., Silva, D., Almeida, C., Rodrigues, M. E., Silva, S., Castro, J., et al. (2023). Effect of phage vB_EcoM_FJ1 on the reduction of ETEC O9:H9 infection in a neonatal pig cell line. Veterinary Res. 54 (1), 26. doi:10.1186/s13567-023-01157-x
García, V., Gambino, M., Pedersen, K., Haugegaard, S., Olsen, J. E., and Herrero-Fresno, A. (2020). F4- and F18-positive enterotoxigenic Escherichia coli isolates from diarrhea of postweaning pigs: genomic characterization. Appl. Environ. Microbiol. 86 (23), e01913-20–e01920. doi:10.1128/AEM.01913-20
Hosseini, B., Behbahani, M., Dini, G., Mohabatkar, H., and Keyhanfar, M. (2022). Investigating the anti-streptococcal biofilm effect of ssDNA aptamer-silver nanoparticles complex on a titanium-based substrate. RSC Adv. 12 (38), 24876–24886. doi:10.1039/D2RA04112J
Kauss, T., Arpin, C., Bientz, L., Vinh Nguyen, P., Vialet, B., Benizri, S., et al. (2020). Lipid oligonucleotides as a new strategy for tackling the antibiotic resistance. Sci. Rep. 2020 10 (1), 1054–1059. doi:10.1038/s41598-020-58047-x
Keefe, A. D., Pai, S., and Ellington, A. (2010). Aptamers as therapeutics. Nat. Rev. Drug Discov. 9 (7), 537–550. doi:10.1038/nrd3141
Li, H., Ding, X., Peng, Z., Deng, L., Wang, D., Chen, H., et al. (2011). Aptamer selection for the detection of Escherichia coli K88. Can. J. Microbiol. 57 (6), 453–459. doi:10.1139/w11-030
Loh, J. M. S., Adenwalla, N., Wiles, S., and Proft, T. (2013). Galleria mellonella larvae as an infection model for group A streptococcus. Virulence 4 (5), 419–428. doi:10.4161/viru.24930
Luise, D., Lauridsen, C., Bosi, P., and Trevisi, P. (2019). Methodology and application of Escherichia coli F4 and F18 encoding infection models in post-weaning pigs. J. Animal Sci. Biotechnol. 10 (1), 53. doi:10.1186/S40104-019-0352-7
Luppi, A. (2017). Swine enteric colibacillosis: diagnosis, therapy and antimicrobial resistance. Porc. Health Manag. 3, 16–18. doi:10.1186/s40813-017-0063-4
Luppi, A., Gibellini, M., Gin, T., Vangroenweghe, F., Vandenbroucke, V., Bauerfeind, R., et al. (2016). Prevalence of virulence factors in enterotoxigenic Escherichia coli isolated from pigs with post-weaning diarrhoea in Europe. Porc. Health Manag. 2, 20. doi:10.1186/s40813-016-0039-9
Ménard, G., Rouillon, A., Cattoir, V., and Donnio, P. Y. (2021). Galleria mellonella as a suitable model of bacterial infection: past, present and future. Front. Cell. Infect. Microbiol. 11, 782733. doi:10.3389/FCIMB.2021.782733
Nimjee, S. M., White, R. R., Becker, R. C., and Sullenger, B. A. (2017). Aptamers as therapeutics. Annu. Rev. Pharmacol. Toxicol. 57, 61–79. doi:10.1146/annurev-pharmtox-010716-104558
Oliveira, R., Pinho, E., Sousa, A. L., DeStefano, J. J., Azevedo, N. F., and Almeida, C. (2022). Improving aptamer performance with nucleic acid mimics: de novo and post-SELEX approaches. Trends Biotechnol. 40 (5), 549–563. doi:10.1016/J.TIBTECH.2021.09.011
Ommen, P., Hansen, L., Hansen, B. K., Vu-Quang, H., Kjems, J., and Meyer, R. L. (2022). Aptamer-targeted drug delivery for Staphylococcus aureus biofilm. Front. Cell. Infect. Microbiol. 12, 814340. doi:10.3389/fcimb.2022.814340
Readman, J. B., Dickson, G., and Coldham, N. G. (2017). Tetrahedral DNA nanoparticle vector for intracellular delivery of targeted peptide nucleic acid antisense agents to restore antibiotic sensitivity in cefotaxime-resistant Escherichia coli. Nucleic Acid. Ther. 27 (3), 176–181. doi:10.1089/NAT.2016.0644
Soundy, J., and Day, D. (2020). Delivery of antibacterial silver nanoclusters to Pseudomonas aeruginosa using species-specific DNA aptamers. J. Med. Microbiol. 69 (4), 640–652. doi:10.1099/jmm.0.001174
Tsai, C. J. Y., Loh, J. M. S., and Proft, T. (2016). Galleria mellonella infection models for the study of bacterial diseases and for antimicrobial drug testing. Virulence 7 (3), 214–229. doi:10.1080/21505594.2015.1135289
Wang, S., Mao, B., Wu, M., Liang, J., and Deng, L. (2018). Influence of aptamer-targeted antibiofilm agents for treatment of Pseudomonas aeruginosa biofilms. Ant. van Leeuwenhoek, Int. J. General Mol. Microbiol. 111 (2), 199–208. doi:10.1007/s10482-017-0941-4
Keywords: ETEC, F4 fimbriae, aptamers, virulence, in vivo blocking, Galleria mellonella
Citation: Barros MM, Castro J, Araújo D, Oliveira R, Campos AM, Silva S, Outor-Monteiro D and Almeida C (2024) Application of DNA aptamers to block enterotoxigenic Escherichia coli toxicity in a Galleria mellonella larval model. Front. Chem. 12:1425903. doi: 10.3389/fchem.2024.1425903
Received: 30 April 2024; Accepted: 21 August 2024;
Published: 29 August 2024.
Edited by:
Yiyang Dong, Beijing University of Chemical Technology, ChinaReviewed by:
Rosa Bellavita, University of Naples Federico II, ItalyHaixiang Yu, Duke University, United States
Yulia Gerasimova, University of Central Florida, United States
Copyright © 2024 Barros, Castro, Araújo, Oliveira, Campos, Silva, Outor-Monteiro and Almeida. This is an open-access article distributed under the terms of the Creative Commons Attribution License (CC BY). The use, distribution or reproduction in other forums is permitted, provided the original author(s) and the copyright owner(s) are credited and that the original publication in this journal is cited, in accordance with accepted academic practice. No use, distribution or reproduction is permitted which does not comply with these terms.
*Correspondence: Carina Almeida, Y2FyaW5hLmFsbWVpZGFAaW5pYXYucHQ=