- 1Division of Agricultural Chemicals, ICAR-IARI, New Delhi, India
- 2The Graduate School, ICAR-IARI, New Delhi, India
- 3Division of Plant Pathology, ICAR-IARI, New Delhi, India
Fungal diseases cause substantial loss to agricultural crops, affecting both quantities and quality. Although several methods are used for preventing disease incidence, fungicides remain crucial for higher yields and better quality. But in the past, the efficacy of several fungicides has decreased due to increased cases of fungicide resistant. In our pursuit of new effective fungicides, we synthesised a series of twenty 2-Indazol-1-yl-chromen-4-one derivatives (6a- 6t). The characterization of synthesized compounds was performed by several spectroscopic methods including Infrared, Nuclear Magnetic Resonance (1H and 13C) and HRMS. Out of 20 synthesised compounds, 19 (6b- 6t) were found to be novel. All synthesised indazolylchromones showed very good antifungal activity against Sclerotium rolfsii and Fusarium oxysporum. Among the tested compounds, 6t and 6f exhibited very good fungicidal activity against S. rolfsii with an ED50 of 10.10 mg L-1 and 16.18 mg L-1, respectively. In case of Fusarium oxysporum compound 6f displayed good’ activity with an ED50 value of 27.82 mg L-1. Molecular docking study was done to predict the binding sites of most active compounds, 6t and 6f with Cytochrome P450 14alpha -sterol demethylase (CYP51) enzyme using molsoft software. The acute toxicity predictions the of synthesized compounds for fish (LC50,96 Hr), daphnid (LC50, 48 Hr) and green algae (EC50, 96Hr) and the chronic toxicity predictions (ChV) were assessed using Ecological Structure Activity Relationship (ECOSAR) model. As per ECOSAR prediction, all the chemicals are inside AD and not missing predictions.
1 Introduction
Fungi represent one of the most harmful groups of phytopathogens, responsible for 80% of plant diseases (Agrios, 2005). Sclerotium rolfsii is a soil-borne fungus that generally occurs worldwide in hot and moderately warm places causing disease on hundreds of plant species. Typically, S. rolfsii attacks the plant near the surface of soil (Kator et al., 2015). The common diseases caused by S. rolfsii are foot rot, stem rot, root rot and wilt. Another important soil borne fungal pathogen is Fusarium oxysporum which causes fusarium wilt, a serious threat for agriculture (Fisher et al., 2012). F oxysporum is among the top ten most destructive fungal plant pathogens in the world (Dean et al., 2012). F oxysporum produces chlamydospores in the soil and survive for a longer time. It penetrates the roots, spread throughout the tissues, interfere with xylem and hinders waterflow resulting in wilting of plants.
Fungicides have been used for over two centuries for managing plant diseases, but in the recent past, many instances of fungicide resistance have led to the loss of many important fungicides (Hollomon, 2015). As a result, synthesis of new molecules has become increasingly important for crop protection. One of the approaches to address the problem of fungicide resistance is the development of hybrid fungicide molecules by combining different scaffolds. The hybrid molecules have multiple sites of action which minimizes the chances of fungicide resistance.
Chromones are natural compounds, mainly obtained from plants. Chromone nucleus is a widely distributed pharmacophore in various important synthetic and natural drugs (Simin et al., 2002; Yadav et al., 2005; Aggarwal et al., 2013; Stompor et al., 2015; Badawy et al., 2015; Kaushik et al., 2021).
Azole fungicides have been widely used in agriculture are considered to be moderate risk fungicides for resistance development. Indazole is one of the important class of azoles. Indazoles are very rare in nature but a large number of synthetic indazole derivatives has been reported to have herbicidal activity (Hwang et al., 2005), insecticidal activity (Lahm et al., 2002), and fungicidal activity (Shakil et al., 2013; Tang et al., 2019). Thus, in the present study we synthesized indazolylchromones by combining the indazole moiety with chromones.
2 Experimental
2.1 Chemicals and instruments
The chemicals required for synthesis of indazolylchromones were obtained from manufacturers. All the chemicals were utilized as received and not purified unless stated otherwise. The TLC plates (20 × 20 cm) coated with silica gel (having F254 fluorescence indicator) were used for reaction monitoring. 100–200 mesh size silica gel was used for column chromatography. Melting points of synthesised compounds were measured by Buchi M-560, reported values were not adjusted/corrected. NMR spectra of (1H and 13C) of synthesised compounds were recorded by JEOL JNM-ECZ400/SI. The mass spectrometry was carried out by ABSCIEX triple TOFTM 5600+ having Turbo Ion Spray. ED50 values were estimated with the SPSS statistical package. Molecular docking was carried out using molsoft software.
2.2 Synthesis of 2-indazol-1-yl-chromen-4-one derivatives (6a- 6t)
2-hydroxyacetophenone derivatives 2a-2n were synthesised by reacting 2- hydroxyacetophenone 1 and bromoalkanes or iodoalkanes of different chain lengths in a molar ratio of 1:1.2 by continuous stirring for 6 h at 60 °C in the presence of K2CO3 and acetone. These 2-hydroxyacetophenone derivatives 2a-2t (1 mmol) on reaction with N,N-dimethylformamidedimethylacetal (DMFDMA) (2 mmol, 2 molequiv) overnight at 90 °C resulted in formation of enaminones 3a-3t. The chromones 4a-4t were synthesized by reacting enaminones 3a-3t, (1 mmol) and iodine (2 mmol, 2 molequiv), in 30 mL CHCl3 at room temperature for 8 h with continuous stirring. The pure chromones were obtained in 67%–89% yield by column chromatography of synthesised crude compounds. Finally, conjugated addition of 3-iodochromones 4a-4t with indazole 5 gave 2-Indazolyl-chromones 6a-6t in the yield range of 76%–94%. (Scheme 1, Table 1). All synthesized 2-Indazol-1-yl-chromen-4-one derivatives were characterized using IR, 1H-NMR, and 13C-NMR analysis. The spectral analysis of all synthesised compounds has been provided in supporting document.
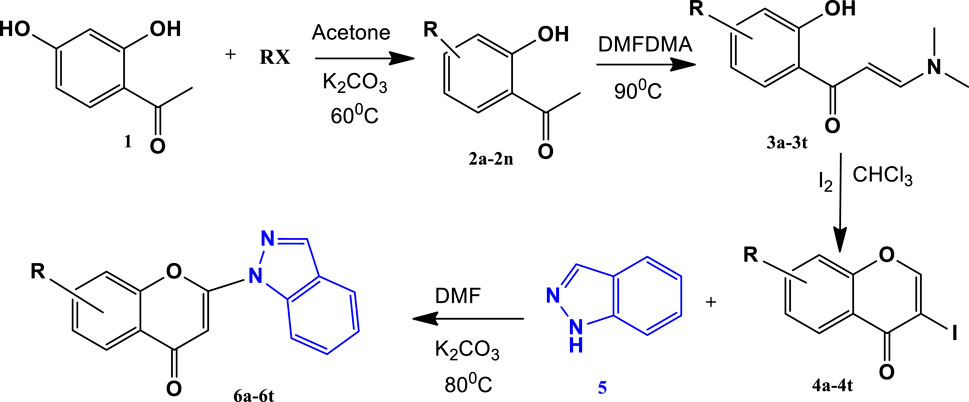
Scheme 1. Synthesis of 2-Indazolyl-chromone (Gamill, 1979; Sugita et al., 1996; Kaushik et al., 2021).
2.3 Bioefficacy evaluation
2.3.1 Test fungus
Two fungal strains, F. oxysporum ITCC8113 and S. rolfsii ITCC 6866, were obtained from the Indian Type Culture Collection (ITCC) centre, Division of Plant Pathology, ICAR-Indian Agricultural Research Institute, New Delhi, India. The strains were kept at a temperature of 27 °C for 4–7 days on Potato Dextrose Agar (PDA) slants. Before conducting the fungicidal bioassay, sub-culturing was performed on Petri plates.
2.3.2 In vitro fungicidal activity
A stock solution of each compound, with a concentration of 10,000 mg L-1, was prepared using DMSO (Dimethylsulphoxide). From the stock solution, five different test concentrations were obtained through serial dilution, namely, 100 mg L-1, 50 mg L-1, 25 mg L-1, 12.5 mg L-1, and 6.25 mg L-1 for S. rolfsii and at 200 mg L-1, 100 mg L-1, 50 mg L-1, 25 mg L-1 and 12.5 mg L-1 for F. oxysporum. Commerical available fungicide Hexaconazole 5% SC and Carbendazim 50% WP were used as positive control.
The fungicidal bioassay was conducted in a laboratory setting using the poisoned food technique (Nene et al., 1979). To determine the effectiveness of the antifungal treatment, the percentage of growth inhibition was computed using Abbott’s formula (Abbott, 1925).
To calculate the corrected % inhibition (IC), the following formula was employed:
I denote the % inhibition, CF = (90 - C)/C x 100, where 90 is the Petriplate diameter (in mm) and C represents the growth of mycelium (in mm) in control.
The ED50 (mg L-1) values, which indicate the Effective Dose for 50% inhibition, were determined using the SPSS statistical package (v16.0).
2.4 Molecular docking
Molecular docking was carried out to predict the interaction of most active compounds, 6t and 6f with Cytochrome P450 14alpha -sterol demethylase (CYP51) enzyme using molsoft software and the respective docking scores were calculated to compare the interaction of compounds with the lanosterol 14α-demethylase (LDM) enzyme.
2.5 Aquatic toxicity prediction
The aquatic toxicity potentials of the synthesized compounds were assessed using Ecological Structure Activity Relationship (ECOSAR) model. It is a free and easy to use computer programme developed by US EPA. It predicts the median lethal concentration (LC50), median effective concentration (EC50), and chronic value (ChV) for various species.
3 Result and discussion
3.1 Synthesis and characterization of individual compounds
In the present work, total twenty indazolylchromones (6a- 6t) were synthesized, out of which 19 compounds (6b- 6t) were found novel. The indazolylchromones were synthesized by 4 steps synthetic scheme (scheme- I). In the last step, conjugated addition of 3-iodochromones 4a-4t with Indazole 5 is the Michael type addition reaction where indazole act as Michael donor (nucleophile) and chromone as Michael acceptor. In the first step indazole attacks chromone ring at 2-position and gives an intermediate A, which on removal of H-1 results in the formation of 2-(1-Indazolyl) chromones (Sugita et al., 1996).
The above synthetic method resulted in the synthesis of 2-(1-Indazolyl) chromones in 76%–94% yield. All the synthesised compounds were characterised by IR, 1H NMR and 13C NMR. In all synthesised compounds 6a-6t, typical peak at δ 6.83–6.93 (1H, s, H-3) appeared due to elimination of iodine from 3- Iodochromones. The peaks at δ 8.21–8.35 (2H, s, H- 3′), δ 7.74–8.19 (1H, d, J = 8, H-4′), δ 7.31–7.48 (1H, t, J = 8, H-5′), δ 7.55–7.67 (1H, t, J = 7.2, H- 6′) and δ 7.66–7.85 (1H, d, J = 8, H-7′) were assigned to protons of indazolyl ring in 1H-NMR spectra of all the compounds and validates the synthesis of indazolylchromones. In 13C-NMR, the peaks at δ 96.10–96.42 (C-3), 123.76–125–85 (C-5′), 121.76–123.99 (C-4′), 155.20–157.96 (C-2), 129.06–135.57 (C- 6′), 114.31–119.07 (C-7’) and at δ 176.44–177.23 for carbonyl carbon (C=O) were prominent for all the synthesised compounds. In IR spectra stretching of 1,675–1,677 (C=O), 1,365–1,368 (C-N) and 1,532–1,537 (pyrone ring C=C stretch) supported the NMR data. The spectroscopic data revealed that in the above-synthesized compounds, the N atom at the one-position of the indazole was linked to chromone ring at C-2 position.
3.2 In vitro antifungal activity
All the synthesised indazolylchromones (6a- 6t) exhibited antifungal activity against S. rolfsii (Table 2), but compound 6t (Figure 1) exhibited the highest activity (ED50 = 10.10 mg L-1) and performed at par with that of Hexaconazole 5% SC (ED50 = 8.57 mg L-1), a commercial fungicide. Among the alkoxy derivatives of indazolylchromones (6a- 6n), butoxy derivative 6f, 2-Indazol-1-yl-7-butoxy chromen-4-one, was found most active with ED50 = 16.18 mg L-1. It was observed that alkoxy derivatives having even number of carbon chain length were more active as compared to odd number of carbon chain length. This trend was observed up to carbon chain length of C- 6. After C-6 there was no regular trend was observed (Figure 2).
In case of F. oxysporum, all the indazolylchromones (6a- 6t) showed antifungal activity (Table 3), compound 6f with ED50 = 27.82 mg L-1was found most active (Figure 3), but that was less than commercial fungicide carbendazim 50% WP (ED50 = 9.01 mg L-1). Antifungal activity of synthesised compounds (6a- 6t) against F. oxysporum was less than their antifungal activity against S. rolfsii.
3.3 Molecular docking
The docking study of most potent compounds, 6f and 6t revealed that both compounds have different orientations, which in turn results in different modes of binding in the active site of CYP51.
In Figure 4, it has been observed that in compound 6f, the indazole ring is having π-π with TRY 76 and hydrophobic interactions with MET 79, PHE 255, PHE 78, VAL 434, LEU 321 residues. The chromone ring was found to have hydrophobic interactions with ALA 256 and HEM 460 residues. The butoxy chain of chromone ring was observed to be engaged in hydrophobic interactions with LYS 97, PHE 83, LEU 100 and ARG 96 residues.
In case of compound 6t (Figure 5), it has been observed that the indazole ring is having π-π with TRY 76 and hydrophobic interactions with PHE 78, LEU 321and HEM 460 residues. The chromone ring was found to have hydrophobic interactions with GLN 72, ALA 73, MET 79 THR 80 and PHE 83 residues.
In commercial fungicide hexaconazole (Figure 6), triazole ring showed hydrophobic interaction with HEM 460, HIS 259, THR 260 and ALA 256 residues. The benzyl ring was found to be engaged with PHE 255, HIE 101 PHE 83 and LEU 100 residues.
The docking studies revealed that compound 6t with highest negative binding energy value (−17.77 kcal mol−1) showed better binding with lanosterol enzyme as compared to compound 6f (−17.65 kcal mol−1). These docking studies supports the in vitro bioassay result where compound 6t (ED 50 = 10.10 mg L-1) exhibited higher fungicidal activity than compound 6f (ED 50 = 16.18 mg L-1).
3.4 Aquatic toxicity
The acute toxicity predictions for fish (LC50,96 Hr), daphnid (LC50, 48 Hr) and green algae (EC50, 96Hr) and the chronic toxicity predictions (ChV) were compared and tabulated in table 5. Fish LC50 values varied from 1.64 × 10−6 to 0.685 mg/L whereas the chronic toxicity values varied from 1.86 × 10−4 to 0.042 mg/L among the different compounds, the highest toxicity was shown by the alkoxy substituted compounds. Among the alkoxy substituted compounds, the toxicity was found to be increasing with increase in the alkyl chain. Lowest toxicities were exhibited by compounds with halo substitutions. Among the halogenated compounds, dichlorinated compound exhibited the highest toxicity but less toxic as compared to alkoxy derivatives (Table 4). As per ECOSAR prediction, all the chemicals are inside AD and not missing predictions. It was observed that the acute as well as chronic toxicity values have negative linear correlations with the log KOW values (Figures 7, 8).
4 Conclusion
In conclusion, a series of twenty compounds were synthesised and characterised successfully. Out of 20 compounds 19 were found novel (6b- 6t). All the synthesized compounds were found to have fungicidal activity against S. rolfsii and F. oxysporum. It was observed that the synthesised compounds have less effectiveness against F. oxysporum. Compound 6t and 6f were found to be very effective against Sclerotium rolfsii. Also, molecular docking studies support the in vitro bioassay result where compound 6t with highest negative binding energy value (−17.77 kcal mol−1) showed better binding with lanosterol enzyme. Therefore, these two compounds could be used for effective management of Sclerotium rolfsii.
Data availability statement
The original contributions presented in the study are included in the article/Supplementary Material, further inquiries can be directed to the corresponding author.
Author contributions
RK: Methodology, Validation, Writing–original draft, Writing–review and editing, Data curation, Formal Analysis. NS: Conceptualization, Writing–review and editing. NN: Methodology, Software, Writing–review and editing. DK: Formal Analysis, Methodology, Writing–review and editing. VR: Conceptualization, Methodology, Writing–review and editing. KT: Methodology, Writing–review and editing. PK: Methodology, Validation, Writing–original draft, Writing–review and editing, Conceptualization, Supervision.
Funding
The author(s) declare that no financial support was received for the research, authorship, and/or publication of this article.
Acknowledgments
Author (RK) is thankful to ICAR-IARI, New Delhi, India for providing all the facilities for research work.
Conflict of interest
The authors declare that the research was conducted in the absence of any commercial or financial relationships that could be construed as a potential conflict of interest.
Publisher’s note
All claims expressed in this article are solely those of the authors and do not necessarily represent those of their affiliated organizations, or those of the publisher, the editors and the reviewers. Any product that may be evaluated in this article, or claim that may be made by its manufacturer, is not guaranteed or endorsed by the publisher.
Supplementary material
The Supplementary Material for this article can be found online at: https://www.frontiersin.org/articles/10.3389/fchem.2024.1411187/full#supplementary-material
References
Abbott, W. S. (1925). A method of computing the effectiveness of an insecticide. J. Econ. Entomol. 18, 265–267. doi:10.1093/jee/18.2.265a
Aggarwal, N., Sharma, V., Kaur, H., and Ishar, M. P. S. (2013). Synthesis and evaluation of some novel chromone based dithiazoles as antimicrobial agents. Int. J. Med. Chem. 815453. doi:10.1155/2013/815453
Badawy, Y. M., El-Sayed, A. F., Soliman, F. S., and Mohy-Aldin, M. S. (2015). Synthesis and herbicidal activity of heterocyclic azlactone and imidazolinone derivatives. Alex Sci. Exch. J. 36, 390–401. doi:10.21608/ASEJAIQJSAE.2015.2957
Dean, R., Van Kan, J. A. L., Pretorius, Z. A., Hammond-Kosack, K. E., Di Pietro, A., Spanu, P. D., et al. (2012). The top 10 fungal pathogens in molecular plant pathology. Mol. Plant Pathol. 13 (4), 804–430. doi:10.1111/j.1364-3703.2012.00822.x
Fisher, M. C., Henk, D. A., Briggs, C. J., Brownstein, J. S., Madoff, L. C., McCraw, S. L., et al. (2012). Emerging fungal threats to animal, plant and ecosystem health. Nature 484 (7393): 186–194. doi:10.1038/nature10947
Gammill, R. B. (1979). A new and efficient synthesis of 3-halogenated 4H-1-benzopyran-4-ones. Synth 11, 901–903. doi:10.1055/s-1979-28869
Hollomon, D. W. (2015). Fungicide resistance: facing the challenge-a review. Plant Prot. Sci. 51 (4), 170–176. doi:10.17221/42/2015-pps
Hwang, I. T., Kim, H. R., Jeon, D. J., Hong, K. S., Song, J. H., Chung, C. K., et al. (2005). New 2-phenyl-4, 5, 6, 7-tetrahydro-2-H-indazole derivatives as paddy field herbicides. Pest Manag. Sci. 61 (5), 483–490. doi:10.1002/ps.992
Kator, L., Hosea, Z. Y., and Oche, O. D. (2015). Sclerotium rolfsii: causative organism of southern blight, stem rot, white mold and sclerotia rot disease. Ann. Biol. Res. 6 (11), 78–89.
Kaushik, P., Shakil, N. A., and Rana, V. S. (2021). Synthesis, biological evaluation and QSAR studies of 3- iodochromone derivatives as potential fungicides. Front. Chem. 9, 636882–882. doi:10.3389/fchem.2021.636882
Lahm, G. P., Harrison, C. R., Daub, J. P., Shapiro, R., Long, J. K., Allen, D. E., et al. (2002). Synthesis and biological activity of indazole insecticides. Synth. Chem. Agrochem. 4 (11), 110–120. doi:10.1021/bk-2002-0800.ch011
Nene, Y. L., and Thapliyal, P. N. (1979). “Fungicides in plant disease control,” in Evaluation of fungicides. Editors Y. L. Nene, and P. N. Thapliyal (New Delhi: Oxford and IBH Publishing Co), 406–428.
Shakil, N. A., Singh, M. K., Sathiyendiran, M., Kumar, J., and Padaria, J. C. (2013). Microwave synthesis,characterization and bio-efficacy evaluation of novel chalcone based 6-carbethoxy-2-cyclohexen-1-one and 2H-indazol-3-ol derivatives. Eur. J. Med. Chem. 59, 120–131. doi:10.1016/j.ejmech.2012.10.038
Simin, K., Ali, Z., Khaliq-Uz-Zaman, S. M., and Ahmad, V. U. (2002). Structure and biological activity of a new rotenoid from Pongamia pinnata. Nat. Prod. Lett. . 16 (5), 351–357. doi:10.1080/10575630290033114
Stompor, M., Dancewicz, K., Gabrys, B., and Anioł, M. (2015). Insect antifeedant potential of xanthohumol, isoxanthohumol, and their derivatives. J. Agric. Food Chem. 63 (30), 6749–6756. doi:10.1021/acs.jafc.5b02025
Sugita, Y., and Yokoe, I. (1996). Reaction of 3-iodochromone with nucleophile 1. Reaction of 3-iodochromone with azoles. Heterocycles 43 (11), 2503–2511. doi:10.3987/com-96-7615
Tang, Z., Li, X., Yao, Y., Qi, Y., Wang, M., Dai, N., et al. (2019). Design, synthesis, fungicidal activity and molecular docking studies of novel 2-((2-hydroxyphenyl) methylamino) acetamide derivatives. Bioorg. Med. Chem. 27 (12), 2572–2578. doi:10.1016/j.bmc.2019.03.040
Keywords: indazolylchromones, NMR, fungus, molecular docking, ECOSAR
Citation: Kundu R, Shakil NA, Narayanan N, Kamil D, Rana VS, Tripathi KP and Kaushik P (2024) Novel indazolylchromones: synthesis, fungicidal evaluation, molecular docking and aquatic toxicity prediction. Front. Chem. 12:1411187. doi: 10.3389/fchem.2024.1411187
Received: 02 April 2024; Accepted: 24 May 2024;
Published: 11 June 2024.
Edited by:
Kamaldeep Paul, Thapar Institute of Engineering & Technology, IndiaReviewed by:
Tanmay Pati, Rensselaer Polytechnic Institute, United StatesPrakash Sekar, Syganture Discovery Ltd, United Kingdom
Copyright © 2024 Kundu, Shakil, Narayanan, Kamil, Rana, Tripathi and Kaushik. This is an open-access article distributed under the terms of the Creative Commons Attribution License (CC BY). The use, distribution or reproduction in other forums is permitted, provided the original author(s) and the copyright owner(s) are credited and that the original publication in this journal is cited, in accordance with accepted academic practice. No use, distribution or reproduction is permitted which does not comply with these terms.
*Correspondence: Parshant Kaushik, cGFyc2hhbnRhZ3JpY29AZ21haWwuY29t