- 1Faculty of Pharmaceutical Sciences, Sojo University, Kumamoto, Japan
- 2Department of Cell Pathology, Graduate School of Medical Sciences, Faculty of Life Sciences, Kumamoto University, Kumamoto, Japan
- 3Department of Food and Life Science, School of Agriculture, Tokai University, Kumamoto, Japan
Introduction: As inhibitors of advanced glycation end products (AGEs), such as pyridoxamine, significantly inhibit the development of retinopathy and neuropathy in rats with streptozotocin-induced diabetes, treatment with AGE inhibitors is believed to be a potential strategy for the prevention of aging, age-related diseases, and lifestyle-related diseases, including diabetic complications. In the present study, the MeOH extract of Epimedii Herba (EH; aerial parts of Epimedium spp.) was found to inhibit the formation of Nε-(carboxymethyl)lysine (CML) and Nω-(carboxymethyl) arginine (CMA) during the incubation of collagen-derived gelatin with ribose.
Materials and methods: EH was purchased from Uchida Wakan-yaku Co., and a MeOH extract was prepared. Several steps of column chromatography purified the extract. Each fraction was tested for inhibitory activity by ELISA using monoclonal antibodies for CML and CMA.
Results: After activity-guided fractionation and purification by column chromatography, three new prenylflavonoids [named Koreanoside L (1), Koreanoside E1 (2), and Koreanoside E2 (3)] and 40 known compounds (4–43) were isolated from EH, and their inhibitory effects against CML and CMA formation were tested. Among these, epimedokoreanin B (8), epimedonin E (21), epicornunin B (22), and epicornunin F (24) inhibited the formation of both CML and CMA, with epimedokoreanin B (8) having the most potent inhibitory effect among the isolated compounds. To obtain the structure–activity relationships of 8, the phenolic hydroxy groups of 8 were methylated by trimethylsilyl-diazomethane to afford the partially and completely methylated compounds of 8. Prenyl derivatives of propolis (artepillin C, baccharin, and drupanin) were used in the assay.
Discussion: As only 8 showed significant activity among these compounds, the catechol group of the B ring and the two prenyl groups attached to the flavanone skeleton were essential for activity. These data suggest that 8 could prevent the clinical complications of diabetes and age-related diseases by inhibiting AGEs.
1 Introduction
In recent years, preventive medicine has begun to play an important role in the aging population globally. Inhibiting the formation of advanced glycation end products (AGEs), which are involved in the progression of lifestyle-related diseases such as diabetic complications (Lin et al., 2011) and atherosclerosis (Torres et al., 2015), and aging-related diseases such as osteoporosis (Brandt et al., 2022) and Alzheimer’s disease (Barnard et al., 2014), is an effective method for the prevention of these diseases using natural products (Al-Musayeib et al., 2011; Harris et al., 2011; Aljohi et al., 2018; Tominaga et al., 2020). Nε-(Carboxymethyl) lysine (CML), a major antigenic AGE structure, accumulates in several human and animal tissues during aging (Araki et al., 1992; Schleicher et al., 1997), and in patients with various diseases, including diabetic nephropathy (Vlassara et al., 1994; Rabbani and Thornalley, 2018) and encephalopathy. Nω-(Carboxymethyl) arginine (CMA) is an acid-labile AGE structure discovered in the enzymatic hydrolysate of glycated collagen (Iijima et al., 2000). Collagen is an important protein that constitutes body tissues; however, it has been reported that when collagen becomes an AGE, it decreases both in strength and flexibility (Kitamura et al., 2021). CMA accumulation in tissue proteins may contribute to the pathophysiology of aging and age-related diseases (Mera et al., 2008; Kinoshita et al., 2019).
Epimedii Herba (EH) has been used in traditional Chinese Medicine to treat erectile dysfunction, dysuria, waist and knee pain, infertility, and angina pectoris (Wu et al., 2003; Li C. et al., 2015; Chen et al., 2015; Qian et al., 2024). In Japan, the crude drugs listed in the Japanese Pharmacopeia and EH extracts are usually included in energy drinks for tonicity. Its main ingredients are prenylated flavonoids (Ma et al., 2011; Li et al., 2021), especially icariin (Figure 1), which suppresses nerve degeneration, improves cognitive function in neurological disorders (Guo et al., 2010), and has neuroprotective effects (Li et al., 2022). It has also been suggested that icariin inhibits AGE-derived neuropathy in PC12 cells (Zhao et al., 2019); RAGE might be a potential target for Epimedium’s anti-neuroinflammatory role in vascular dementia, which is an insight from network pharmacology and molecular simulation (Yuan et al., 2023), and extracts including icariin may reduce the risk of atherosclerosis by inhibiting the formation of AGEs on HDL (Kim and Shim, 2019). However, these studies were comparisons using readily available icariin, and the anti–glycation activity of the main body of EH was not considered due to the limited number of samples used in these evaluations. We have previously shown that some compounds isolated from EH have significant inhibitory effects on AGE formation (Nakashima et al., 2016). In this study, the isolation and purification of EH’s prenylflavonoid compounds was continued; 40 known compounds and three new compounds were isolated, and their chemical structures were determined. Based on the results of CML and CMA production inhibitory activity tests on 35 prenylflavonoids, of which quantities were available, the chemical structure characteristics necessary for the production inhibitory activity were summarized by synthesis of derivatives and comparison with related natural products and are reported.
2 Materials and methods
2.1 General experimental procedures
The optical rotation was measured using a P-1020 polarimeter (JASCO Co. Ltd., Tokyo, Japan). 1H- and 13C-NMR spectra were measured in pyridine-d5 and chloroform-d using a JEOL ECA 500 NMR spectrometer (JEOL Ltd., Tokyo, Japan) at 500 MHz and 125 MHz, respectively. The chemical shift (δ) was reported in parts per million (ppm). The J value was reported in Hz, using either pyridine-d5 as an internal standard for 1H NMR (7.20 ppm) and 13C NMR (123.5 ppm) or chloroform-d as an internal standard for 1H NMR (7.26 ppm) and 13C NMR (77.0 ppm). High-resolution electrospray ionization mass spectrometry (HRESIMS) spectra were recorded with a JMS-T100LP spectrometer (JEOL Ltd.). IR spectra were recorded using Jasco FT/IR-4200 spectrophotometer (JASCO Co. Ltd.). Preparative HPLC was performed on a Shimadzu HPLC system equipped with an LC-20AT pump (Shimazu Co. Ltd., Kyoto, Japan), JASCO 830-RI detector (JASCO), OR-2090 Plus chiral detector (JASCO), and Sugai U-620 column heater (Sugai Chemie Inc., Wakayama, Japan); COSMOSIL 5C18 AR-II, COSMOSIL π NAP (5 μm, ϕ10 × 250 mm, Nacalai Tesque Inc., Kyoto, Japan), and Atlantis Prep T3, SunFire Prep C18, X-Bridge Prep C18 (5 μm, ϕ10 × 250 mm, Waters Co., Milford, MA, United States) columns at a flow rate of 2.0 mL/min; and Triart PFP and Triart Phenyl (5 μm, ϕ4.6 × 250 mm, YMC Co. Ltd., Kyoto, Japan) columns at a flow rate of 1.0 mL/min, with each column temperature at 40 °C. For the analysis of sugar moieties, HPLC was performed on the Shodex RS-Pak DC-613 (5 μm, ϕ6.0 × 150 mm, Resonac Corp., Tokyo, Japan) column at a flow rate of 1.0 mL/min and a column temperature of 80°C. TLC was performed using pre-coated silica gel 60 F254 plates (Merck Ltd., Frankfurt, Germany). Detection was achieved by spraying the plates with 10% H2SO4 followed by heating. Column chromatography was carried out on MCI gel CHP20P (Mitsubishi Chemical Co., Tokyo, Japan), Sephadex LH-20 (GE Healthcare Bioscience Co., Uppsala, Sweden), μ-Bonda Pak C18 (ϕ25 × 200 mm, Waters Co.), silica gel 60 columns (230–400 mesh, Merck Ltd.), and Amberlite MB-3 (Organo Co., Tokyo, Japan).
2.2 Plant material
The aerial parts of Epimedium spp. were purchased from Uchida Wakan-yaku Co., Ltd. (Tokyo, Japan), inspected by Uchida Wakan-yaku, and certified as Epimedii Herba (EH; lot number: C1S1504) according to the specifications of the Japanese Pharmacopeia. A voucher specimen was deposited in the herbarium of the Faculty of Pharmaceutical Sciences, Sojo University (SJU1103).
2.3 Extraction and isolation
EH (3.0 kg) was extracted twice with MeOH by sonication for 6 h (30 min × 12) at 25 °C. The extract was concentrated under reduced pressure to obtain a residue (485 g). The residue was partitioned between n-hexane and 80% MeOH, after which the 80% MeOH layer was concentrated to yield a residue (408 g), which was loaded onto an MCI-gel CHP20P column and eluted with an H2O–MeOH gradient (0, 50, and 100% MeOH) to yield three fractions (frs. 1–3). Fr. 3 (65.0 g) was further applied to the MCI gel CHP20P column and eluted with an H2O–MeOH gradient (40, 50, 60, 70, 80, 90, and 100% MeOH) to yield eight fractions (frs. 3-1 to 3-8). Fr. 3-4 (9.0 g) was loaded onto a Sephadex LH-20 column (eluted with MeOH) to yield five fractions (frs. 3-4-1 to 3-4-5). A portion of fr. 3-4-3 (500 mg) was loaded for μ-Bonda Pak C18 column chromatography and eluted with an H2O–MeOH gradient (50, 60, 70, 80, 90% MeOH) to give six fractions (frs. 3-4-3-1 to 3-4-3-6). Fr. 3-4-3-3 (41.4 mg) was subjected to SiO2 (CHCl3: MeOH: H2O = 20:1:0 to 8:2:0.2 (v/v)) to yield six fractions (frs. 3-4-3-3-1 to 3-4-3-3-6). Fr. 3-4-3-3-3 (16.3 mg) was subjected to preparative HPLC [Atlantis Prep. T3 C18 (eluted with 70% MeOH)] to obtain compounds 2 (5.1 mg) and 3 (5.5 mg). Fr. 3-4-4 (660 mg) was subjected to Sephadex LH-20 chromatography (eluted with MeOH) to yield five fractions (frs. 3-4-4-1 to 3-4-4-5). Fr. 3-4-4-2 (285.9 mg) was subjected to μ-Bonda Pak C18 column chromatography and eluted with an H2O–MeOH gradient (60, 70, 80, 90% MeOH) to give nine fractions (frs. 3-4-4-2-1 to 3-4-4-2-9). Fr. 3-4-4-2-8 (3.0 mg) was also purified via preparative HPLC [X-Bridge Prep C18 (eluted with 80% MeOH)] to yield compound 1 (2.5 mg). The detailed isolation procedures for the other known compounds (4–43) is described in the Supplementary Material.
Koreanoside L (1): Yellow amorphous powder; Rf value 0.33 (solvent CHCl3: MeOH: H2O = 9:1:0.1); [α]D −204 (c = 0.15, MeOH); 1H and 13C NMR (pyridine-d5, 500, and 125 MHz) data in Table 1; Positive ESIMS: m/z 533 [M + Na]+; HRESIMS 533.1461 [M + Na]+ (calculated for C27H26NaO10: 533.1424). IR (KBr) νmax 3420, 2926, 1660, 1598, 1258 cm-1
Koreanoside E1 (2): Yellow amorphous powder; Rf value 0.56 (solvent CHCl3: MeOH: H2O = 8:2:0.2); [α]D −92.1 (c = 0.27, MeOH); 1H and 13C NMR (pyridine-d5, 500 and 125 MHz) data in Table 1; Negative ESIMS: m/z 529 [M-H]-; HRESIMS 529.1726 [M-H]- (calculated for C27H29O11: 529.1710). IR (KBr) νmax 3567, 2925, 1654, 1179 cm-1
Koreanoside E2 (3): Yellow amorphous powder; Rf value 0.56 (solvent CHCl3: MeOH: H2O = 8:2:0.2); [α]D −48.5 (c = 0.15, MeOH); 1H and 13C NMR (pyridine-d5, 500 and 125 MHz) data in Table 1; Negative ESIMS: m/z 529 [M-H]-; HRESIMS 529.1726 [M-H]- (calculated for C27H29O11: 529.1710). IR (KBr) νmax 3567, 2924, 1610, 1259, 1180 cm-1
2.4 Acid hydrolysis of compound 1
Compound 1 (1.0 mg) was hydrolyzed with 2 M HCl: dioxane = 1:1 solvent (1 mL) at 95°C in a pear-shaped flask for 1.5 h and H2O (2 mL) was added to the mixture, and it was evaporated to dryness under vacuum to obtain a residue. The residue was loaded onto Amberlite MB-3 (ϕ15 × 40 mm), eluted with H2O, subjected to an MCI gel CHP20P (ϕ15 × 40 mm), and the water elute was evaporated to dryness under vacuum. The residue was dissolved in CH3CN: H2O = 3:1 solution (20 μL) and analyzed by HPLC [Shodex RS-Pak DC-613 (ϕ6.0 × 150 mm, eluted with CH3CN: H2O = 3:1), flow rate 1.0 mL/min, 70 °C] connected to an optical rotatory detector. Then, by comparing the retention time and polarity of the standard [l-rhamnose: tR = 4.5 min (−)], the constituent sugars of compound 1 was identified as l-rhamnose [tR = 4.5 min (−)].
2.5 Enzymatic hydrolysis of compounds 2 and 3
Compounds 2 and 3 (2.5 mg each) were dissolved in DMSO (40 μL) and mixed with PBS (pH 6.2; 360 μL). Naringinase (10 mg; Sigma-Aldrich Corp., Saint Louis, MO, United States) was added to the mixtures and shaken at 40 °C, 120 rpm, for 24 h. The mixtures were centrifuged to remove the supernatant and the precipitate was dissolved in a small amount of pyridine and loaded onto SiO2 [ϕ10 × 130 mm, CHCl3: MeOH: H2O = 9:1:0.1 (v/v)] to obtain aglycones 2a (1.9 mg, 73% yield) and 3a (2.0 mg, 75% yield), respectively. HPLC analyzed each supernatant from compounds 2 and 3, coupled with an optical rotatory detector, to identify l-rhamnose [tR = 4.5 min (−)].
Compound 2a: Rf value: 0.78 (solvent CHCl3: MeOH: H2O = 9:1:0.1), [α]D +5.5 (c = 0.038), 1H-NMR (500 MHz, CDCl3) [δH 8.12 (2H, d, J = 9.1 Hz, H-2′, 6′), 7.02 (2H, d, J = 9.1 Hz, H-3′, 5′), 6.44 (1H, s, H-6), 5.09, 4.95 (each 1H, br s, H-14a,b), 4.47 (1H, br d, J = 8.6 Hz, H-12), 3.89 (3H, s, 4′-OMe), 3.34 (1H, br d, J = 15.4 Hz, H-11a), 3.02 (1H, dd, J = 8.6, 15.4 Hz, H-11b), 1.87 (3H, s, H-15)].
Compound 3a: Rf value: 0.78 (solvent CHCl3: MeOH: H2O = 9:1:0.1), [α]D −14.0 (c = 0.038), 1H-NMR (500 MHz, CDCl3) [δH 8.17 (2H, d, J = 6.9 Hz, H-2′, 6′), 7.03 (2H, d, J = 6.9 Hz, H-3′, 5′), 5.04, 4.88 (each 1H, br s, H-14a,b), 4.39 (1H, br d, J = 8.6 Hz, H-12), 3.90 (3H, s, 4′-OMe), 3.24 (1H, dd, J = 2.9, 14.9 Hz, H-11a), 3.04 (1H, dd, J = 9.1, 14.9 Hz, H-11b), 1.85 (3H, s, H-15)].
2.6 Synthesis of MTPA esters of compounds 2a and 3a
Compound 2a (1.5 mg) was placed in a pear-shaped flask and dissolved in MeOH (200 μL); 2 M trimethylsilyl (TMS)-diazomethane 800 μL (67 eq) at 0 °C was added, and the reaction was stirred for 1 h at 20 °C. The reactant was purified using SiO2 [ϕ10 × 70 mm, CHCl3: MeOH = 50:1 (v/v)] to obtain compound 2b (1.0 mg, 60% yield). Next, 2b (1.0 mg, 2.34 μmol) was placed in a pear-shaped flask sealed with nitrogen purge, and CH2Cl2 (200 μL), (R)-(−)-α-methoxy-α-(trifluoromethyl)phenylacetyl chloride [(R)-(−)-MTPA-Cl, ca. 18% in dichloromethane, ca. 1.0 mol/L; TCI Reagents, Tokyo, Japan], was added to 70 μL (70 μmol, 30 eq) dimethylaminopyridine (DMPA), water-soluble carbodiimide (WSC), and triethylamine (TEA) and reacted for 1 h. The reactant was purified using SiO2 [ϕ10 × 100 mm, CHCl3: MeOH = 50:1 (v/v)] and HPLC [Triart PFP (ϕ4.6 × 250 mm, eluted with 85% MeOH)] to give 2b-(S)-MTPA ester (2b-S, 0.8 mg, 53% yield). Similarly, 2a (1.6 mg) was reacted with the (S)-(+)-MTPA-Cl reagent to give 2b-(R)-MTPA ester (2b-R, 0.5 mg, 38% yield). Compound 3a was methylated and purified using the same method as that used for 2a to obtain 3b. After that, 3b was reacted with (R)-(−)-MTPA-Cl and (S)-(+)-MTPA-Cl in the above methods to give MTPA ester 3b-S (1.5 mg, 47% yield) and 3b-R (0.5 mg, 35% yield), respectively.
Compound 2b-S (= compound 3b-R): Rf value: 0.78 (solvent CHCl3: MeOH = 100:1), 1H-NMR (500 MHz, CDCl3) [δH 8.02 (2H, d, J = 9.1 Hz, H-2′, 6′), 6.97 (2H, d, J = 9.1 Hz, H-3′, 5′), 6.28 (1H, s, H-6), 5.79 (1H, br d, J = 6.3 Hz, H-12), 5.11, 5.01 (each 1H, br s, H-14a,b), 4.00, 3.89, 3.88, 3.86 (each 3H, s, 4’, 3, 5, 7-OMe), 3.40 (1H, dd, J = 10.3, 15.3 Hz, H-11a), 3.01 (1H, dd, J = 4.0, 15.3 Hz, H-11b), 1.89 (3H, s, H-15)]
Compound 2b-R (= compound 3b-S): Rf value: 0.78 (solvent CHCl3: MeOH = 100:1), 1H-NMR (500 MHz, CDCl3) [δH 8.01 (2H, d, J = 7.4 Hz, H-2′, 6′), 6.88 (2H, d, J = 7.4Hz, H-3′, 5′), 6.37 (1H, s, H-6), 5.79 (1H, dd, J = 4.0, 9.7 Hz, H-12), 4.99, 4.96 (each 1H, br s, H-14a,b), 4.02, 3.92, 3.89, 3.88 (each 3H, s, 4’, 3, 5, 7-OMe), 3.43 (1H, dd, J = 10.3, 13.8 Hz, H-11a), 3.03 (1H, dd, J = 4.0, 14.3 Hz, H-11b), 1.74 (3H, s, H-15)]
2.7 Partial methylation of phenolic hydroxyl groups in epimedokoreanin B
Epimedokoreanin B (EK-B, isolated compound number 39, 20 mg, 47.4 μmol) was placed in a pear flask and dissolved in MeOH (200 μL). Then, 500 μL (5 eq) of approximately 2 M TMS-diazomethane solution in diethyl ether (TCI Reagents, Tokyo, Japan) was added at 0 °C, and this mixture was allowed to react for 1 h at 20 °C. The reactants were purified using silica gel SiO2 [ϕ10 × 70 mm, eluted with hexane: acetone = 3:1 (v/v)] and HPLC [Triart PFP (ϕ4.6 × 250 mm, eluted with 100% MeOH)] to yield dimethoxy EK-B (1.6 mg, 11% yield), trimethoxy EK-B (2.6 mg, 25% yield), and tetramethoxy EK-B (5.5 mg, 19% yield), respectively.
Dimethoxy EK-B (A): Rf value: 0.60 (solvent hexane: acetone = 2:1), 1H-NMR (500 MHz, CDCl3) [δH 7.41 (1H, s, H-2′), 7.34 (1H, s, H-6′), 6.60 (1H, s, H-3), 6.42 (1H, s, H-6), 5.27 (1H, t, J = 6.4 Hz, H-12), 4.90 (1H, t, J = 6.3 Hz, H-2″), 3.94, 3.90 (each 3H, s, 4’, 7-OMe), 3.52 (2H, d, J = 8.3 Hz, H-11), 4.92 (2H, d, J = 7.5 Hz, H-1″), 1.84 (3H, s, H-15), 1.80 (3H, s, H-5″), 1.71 (3H, s, H-4”), 1.69 (3H, s, H-14)].
Trimethoxy EK-B (B): Rf value: 0.75 (solvent hexane: acetone = 2:1), 1H-NMR (500 MHz, CDCl3) [δH 7.41 (1H, s, H-2′), 7.34 (1H, s, H-6′), 6.60 (1H, s, H-3), 6.42 (1H, s, H-6), 5.27 (1H, t, J = 6.4 Hz, H-12), 4.90 (1H, t, J = 6.3 Hz, H-2″), 3.94, 3.91, 3.90 (each 3H, s, 3′, 4’, 7-OMe), 3.52 (2H, d, J = 8.3 Hz, H-11), 4.92 (2H, d, J = 7.5 Hz, H-1″), 1.84 (3H, s, H-15), 1.80 (3H, s, H-5″), 1.71 (3H, s, H-4”), 1.69 (3H, s, H-14)].
Tetramethoxy EK-B (C): Rf value: 0.20 (solvent hexane: acetone = 2:1), 1H-NMR (500 MHz, CDCl3) [δH 7.41 (1H, s, H-2′), 7.34 (1H, s, H-6′), 6.60 (1H, s, H-3), 6.42 (1H, s, H-6), 5.27 (1H, t, J = 6.4 Hz, H-12), 4.90 (1H, t, J = 6.3 Hz, H-2″), 3.94, 3.91, 3.90, 3.90 (each 3H, s, 3′, 4’, 5, 7-OMe), 3.52 (2H, d, J = 8.3 Hz, H-11), 4.92 (2H, d, J = 7.5 Hz, H-1″), 1.84 (3H, s, H-15), 1.80 (3H, s, H-5″), 1.71 (3H, s, H-4”), 1.69 (3H, s, H-14)].
2.8 Determination of the inhibitory effects of compounds on CML and CMA formations
Gelatin (2 mg/mL) and ribose (30 mM) were incubated with the tested compounds in PBS for CML and in 100 mM sodium phosphate buffer for CMA at 37 °C for 7 days, followed by the determination of CML and CMA formation using a noncompetitive enzyme-linked immunosorbent assay (ELISA).
2.9 ELISA
ELISA was performed as previously described (Sugawa et al., 2016). Briefly, each well of a 96-well microtiter plate was coated with 100 μL of the sample in PBS, blocked with 0.5% gelatin, and washed three times with PBS containing 0.05% Tween-20 (washing buffer). The wells were incubated with 100 μL of anti-CML antibody 6D12 (0.1 μg/mL) or anti-CMA antibody 3F5 (1.0 μg/mL) dissolved in washing buffer for 1 h. The wells were then washed three times with washing buffer and incubated with horseradish peroxidase-conjugated anti-mouse IgG antibodies, followed by incubation with 1,2-phenylenediamine dihydrochloride. The reaction was terminated by the addition of 100 μL of 1 M sulfuric acid and the absorbance at 492 nm was read by a micro-ELISA plate reader.
2.10 Statistics
All data are representative of two or three independent experiments. Data are expressed as mean (SD). The Mann–Whitney U test was used for two-group comparisons. Statistical significance was set at p < 0.05.
3 Result and discussion
3.1 Isolation and determination of new compounds (1–3) from EH
Following activity-guided fractionation of the three fractions prepared in a previous report (Nakashima et al., 2016) (frs. 1, 2, and 3), fr. Three was further separated by column chromatography combined with MCI gel CHP20P, Sephadex LH-20, μ-Bondapak C18, and preparative HPLC. Then, 43 candidate prenylated flavonoid derivatives, including new compounds (1–3), were isolated to test the inhibition of CML and CMA accumulation.
Compound 1 was obtained as an amorphous yellow powder. The molecular formula (C27H26O10) was established based on 1H-, 13C-NMR, HRESIMS (m/z 533.1461 [M + Na]+, and calculated for C27H26O10Na: 533.1448). In the 1H-NMR data (Table 1), exo-methylene protons [δH 5.29, 5.89 (each 1H, s, H-14)], olefinic methyl protons [δH 2.17 (3H, s, H-15)], and a tri-substitute olefinic proton [δH 7.27 (1H, s, H-11)] suggested a 2-(prop-1-en-2-yl) furan moiety. In addition, two para-coupled aromatic protons [δH 8.29 (2H, d, J = 8.6 Hz, H-2′, 6′), 7.27 (2H, d, J = 8.6 Hz, H-3′,5′)], a methoxy group signal [δH 3.82 (3H, s)], an isolated anomeric proton signal [δH 6.23 (1H, d, J = 1.8 Hz, H-1 of rhamnose)], and a doublet methyl protons signal [δH 1.41 (3H, d, J = 6.3 Hz, H-6 of rhamnose)] were observed (Table 1). The 13C NMR and HMQC data indicated 27 carbon resonances (Table 1), corresponding to a flavone derivative with an exo-methylene group, 2-(prop-1-en-2-yl) furan, and deoxyhexose moiety. Compound 1 was acid-hydrolyzed, and the resulting sugar fraction was analyzed by HPLC connected to an optical rotatory detector, and the peak of L-(−)-rhamnose was observed. The heteronuclear multiple bond correlations (HMBCs) were found between H-11 (δH 7.27) and C-7 (δC 159.1), C-8 (δC 110.7), C-9, C-12 (δC 156.9), and C-13 (δC 132.7), as well as those between H-14 (δH 5.29, 5.89) and C-12 (δC 156.9), H-15 (δH 2.17) and C-12, C-13, and C-14 (δC 113.7). Thus, compound 1 confirmed that the 2-(prop-1-en-2-yl) furan moiety was connected to C-7 and C-8. Further, HMBCs were also found between the H-1 of rhamnose (δH 6.23) and C-3 (δC 136.6), the methoxy group signal (δH 3.82), and C-4’ (δC 162.0). Therefore, the rhamnose was connected at C-3, and the methoxy group was connected at C-4 (Figure 2). Thus, the structure of one was deduced as 3-O-α-L-rhamnopyranosyl-5-hydroxy-2-(4-methoxyphenyl)-8-(prop-1-en-2-yl)-2H-pyran-2-yl)oxy)-4H-furo [2,3-h]chromen-4-one, and named koreanoside L.
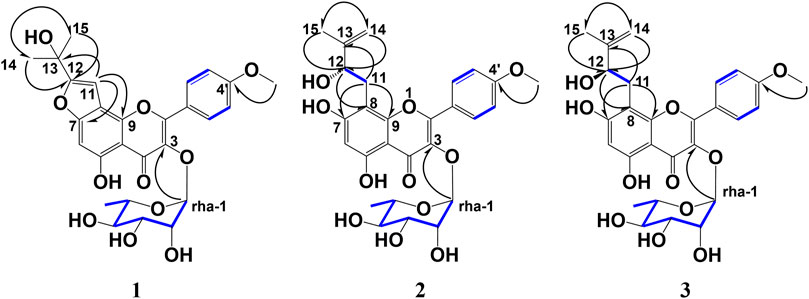
Figure 2. Key HMBC and 1H–1H COSY correlations of koreanoside L (1), koreanoside E1 (2), and koreanoside E2 (3).
Compound 2 was obtained as a yellow amorphous powder and the rotation value was [α]D −92.1° (c = 0.27). The molecular formula (C27H30O11) of two was established based on 1H, 13C-NMR, and HRESIMS (m/z 529.1726 [M−H]−; it was calculated for C27H29O11: 529.1726). In the 1H-NMR spectrum (Table 1), compound 2 was found in an O-methyl group (δH 3.67, 3H, s), AA’BB’ coupling system [δH 8.20 (2H, d, J = 8.6 Hz, H-2′,6′), and δH 7.07 (2H, d, J = 8.6 Hz, H-3′, 5′)]; an aromatic H-atom singlet [δH 6.81 (1H, s, H-6)], a tertiary methyl group [δH 2.00 (3H, s, H-15)], exo-methylene protons [δH 5.24 and 4.89 (1H, each s, H-14a, b)], an O-bearing CH [δH 4.92 (dd, J = 5.7, 12.3 Hz, H-12)], and a methylene group [δH 3.43 (2H, m, H-11)] were observed. These results suggested the presence of a 3-methyl-but-3-en-2-ol group. In addition, the anomeric proton signal [δH 6.16 (1H, br s)] suggested the presence of a rhamnose moiety. 13C-NMR data indicated that it was a flavone derivative with a 3-methyl-but-3-en-2-ol group and rhamnose moiety. HMBCs were found between the anomeric proton (δH 6.16) and C-3 (δC 130.5), the methylene proton of the 3-methyl-but-3-en-2-ol group (δH 3.43) and C-7 (δC 164.0), C-9 (δC 155.2), and the hydroxy methine proton at H-12 (δH 4.92) and C-8 (δC 105.1), and the O-methyl proton (δH 3.67) and C-4’ (δC 161.8). Thus, the planar structure of compound 3 was determined as shown in Figure 2. While compound 3 has been reported as koreanoside E and the NMR data were in good agreement (Li X. et al., 2015), the absolute steric structure of C-12 was not discussed. To determine the absolute steric structure of C-12, a modified Mosher method was applied to compound 2 (Ohtani et al., 1991; Tabopda et al., 2008). At first, to cleave the rhamnose moiety, compound 3 was treated with enzymatic hydrolysis using naringinase, and an aglycone 2a in 73% yield was obtained. The supernatant of the reactant was analyzed using HPLC connected to an optical rotatory detector, and the L-(−)-rhamnose peak was observed. 2a was obtained as a yellow amorphous powder and the rotation value was [α]D +5.5 (c = 0.038). The structure of 2a was confirmed by 1H-NMR data. The phenolic hydroxyl group of 2a was methylated with TMS-diazomethane. The reactant was purified using SiO2 and preparative HPLC to obtain methylated 2a (compound 2b) in 87% yield. Finally, compound 2b was reacted with (R)-(−)-α-methoxy-alpha-(trifluoromethyl) phenylacetyl chloride [(R)-(−)-MTPA-Cl] and (S)-(+)-MTPA-Cl to give MTPA esters 2b-S and 2b-R in 53% and 38% yields, respectively. Both compounds were measured using 1H-NMR and adapted to the modified Mosher method. ΔδH of 2b-S and 2b-R were H-15 (+0.15), 14a, b (+0.12, +0.05); and H-11a, b (each −0.02), H-6 (−0.09), 7-OMe (−0.03), 5′-OMe (−0.03), and 3′-OMe (−0.04), respectively. It is evident that protons with >0 are located on the right side of the MTPA plane and those with <0 are on the left side (Figure 3A). Therefore, the absolute conformation C-12 of compound 2 was determined as S. Compound 2 was assigned the trivial name, koreanoside E1.
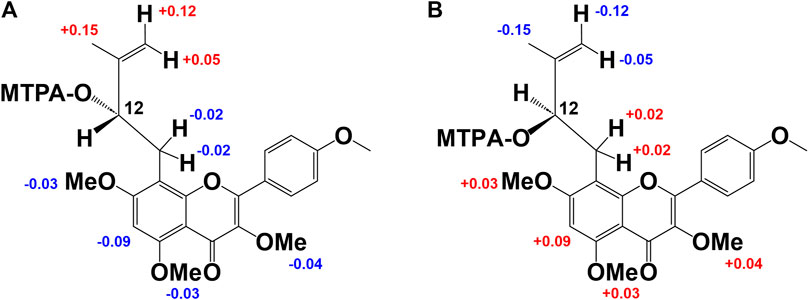
Figure 3. Modified Mosher’s method results of derivative from 2b and 3b. Difference of the 1H-NMR chemical shifts of (A): 2b-S and 2b-R and (B): 3b-S and 3b-R [Δδ (in ppm) = δS - δR].
Compound 3 was similar to compound 2 in the 1H-NMR spectrum, but its rotation value was different ([α]D −48.5° (c = 0.36)). The 13C-NMR date of three and two were identical but C-3 (δC 130.5–133.7), C-4 (δC 178.8–179.4), and C-15 (δC 18.1–19.9) data were shifted lower in the field. Because of the possibility of stereoisomerism at the C-12 position, compound 3 was hydrolyzed by naringinase to get an aglycone 3a in 75% yield. Compound 3a was obtained as a yellow amorphous powder and the rotation value was [α]D −14.0 (c = 0.038). The 1H-NMR of 3a was identical to that of 2a. The supernatant of reactant was also analyzed by HPLC connected to an optical rotatory detector, and the peak of L-(−)-rhamnose was observed. To adapt the modified Mosher method, the phenolic hydroxyl groups of 3a were methylated and purified to yield 3b. Subsequently, 3b was reacted with (R)-(−)-MTPA-Cl to give MTPA ester 3b-S in 47% yield. The 1H-NMR data of 3b-S was superimposable to that of 2b-R, so that it was applied to the advanced Mosher method because the 1H-NMR signals of 2b-S were the same as those of 3b-R. As a result, the distribution of chemical shifts, as shown in Figure 3B, was observed, and the absolute conformation at C-12 of compound 3 was determined as R. Compound 3 was given the trivial name koreanoside E2. This indicated that compound 3 was a diastereomer of compound 2 because of the rhamnose bond to C-3, which could be separated by HPLC.
The known compounds (4–43) were isolated by column chromatography, as described in the Supplementary Material, and were identified as prenylated flavonoids or flavanones (Supplementary Figures S1–S6). Compound 4 was identified as koreanoside I, which was obtained from Epimedium koreanum as an antipulmonary fibrosis compound (Zhao et al., 2022). Compound 18 was icariin, which is the main component of EH. Compounds 7, 8, and 9 were related to icariin, and their terminal sugar moieties attached to the C-2 of rhamnose were glucose, rhamnose, and xylose, respectively. These were called epimedins A (7), B (8), and C (9) (Oshima et al., 1987; Mizuno et al., 1988). Compound 10 was identified as epimedin I (10) (Sun et al., 1998; Zhao et al., 2007), which had glucose attached to the C-3 of rhamnose in icariin. Compounds 11, 12, 13, and 14 were diacetyl compound 10 with a terminal glucose moiety. Compounds 11, 12, and 13 were attached to two acetyl groups at C-2, 6; C-3, 6; and C-4, six of glucose, respectively. Compound 14 was attached to an acetyl group at C-6 of glucose, and the glucose of compound 28 was substituted with a hydroxy group at C-7 in compound 14. These have been reported to be epimedin K (11), epimedin L (12), caohuoside B (13), epimedokoreanoside I (14), and korepimedoside A (28), respectively (Zhao et al., 2007). Compounds 6, 18, 19, and 20 were diglycosides with hydroxyl groups at C-3 and prenyl groups at C-8. They were identified as cuhuoside (6) (Zhao et al., 2007), icariin (18) (Xia et al., 2010), sagittatoside A (19) (Mizuno et al., 1988), and 2″-O-rhamnosyl icariside II (20) (Zhang et al., 2007), respectively. Compounds 25, 26, and 27 had two acetyl groups on the terminal glucose of korepimedoside A (28). The positions of the acetyl groups were C-2, 6; C-3, 6; and C-4, 6, respectively. They were reported as korepimeosides A (25) and B (26) (Li et al., 2016), and epimedigrandioside A (27) (Zulfiqar et al., 2017), respectively. Compounds 5, 16, 17, 21, 22, 23, 24, and 30 were monoglycosides with rhamnose attached to the C-3 of the C-ring or glucose attached to the C-7 of the A-ring. They were identified as koreanoside F (16), G (17) (Choi et al., 2019), epimedoside C (5), pherodendroside (21) (Li et al., 1998), caohuoside C (22) (Zhao et al., 2007), icariside II (23) (Xia et al., 2010), icarisoside A (24) (Li et al., 2016), and icariside I (30) (Xia et al., 2010), respectively. The other isolated compounds were prenylated aglycones of flavonoids or flavanones. They were identified as epimedokoreanin C (15) (Li et al., 1994), 8-prenyl kaempferol (29), 8-prenyl luteolin (31) (Dong et al., 2007), epicornunin B (32), F (33) (Pang et al., 2018), gaocaonin E (34), euchrestaflavanone A (35) (Nakahara et al., 2003)[48], epimedonin C (36) (Jin et al., 2014), 8,5′-diprenyl apigenin (37), broussonol D (38) (Zhang et al., 2001), epimedokoreanin B (39) (Li et al., 1994), epimedonin E (40), F (43) (Nakashima et al., 2016), 4′-O-methyl limonianin (41), and limonianin (42) (Bacher et al., 2010).
3.2 The inhibitory effects of compounds on CML and CMA formation
Of the 43 compounds isolated and determined from EH, 1 mM DMSO solutions were prepared for 35 compounds, which were evaluated for their inhibitory activity against CML and CMA formation (Table 2). The results showed that samples 7, 8, 9, 10, 16, 17, 21, 22, and 24 had vigorous CML production inhibitory activity of more than 80% at 10 μM (Figure 4A). All the nine prenylflavonoids that exhibited significant inhibitory activity were aglycones. For further comparison, when the sample concentration was examined at 1.0 μM, samples 8, 21, 22, and 24 showed more potent inhibition than pyridoxamine (PM) and luteolin as the positive controls (Figure 4B). Inhibitory formation of CMA also showed significant activity in the same compounds at 10 μM (Figure 4C). In contrast, weak inhibitory activity was observed for samples 8, 21, and 22, and the other compounds displayed no activity at 1.0 μM (Figure 4D).
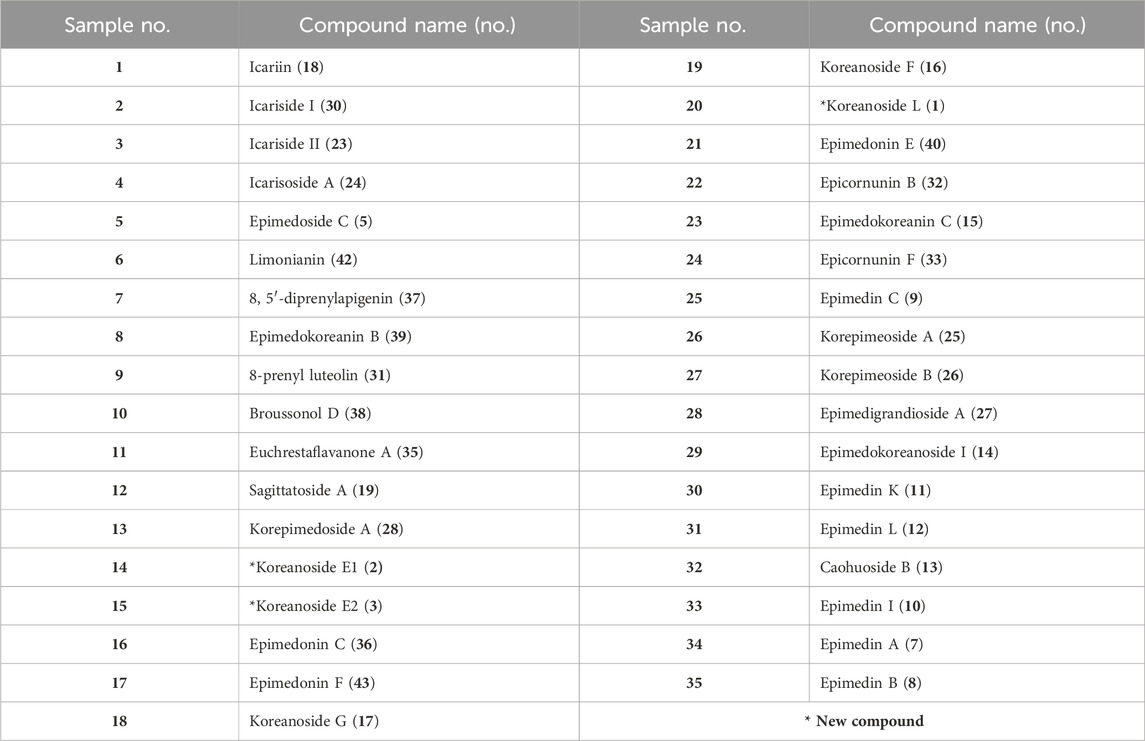
Table 2. Prenylflavonoids from Epimedii Herba used for inhibiting activity test against CML and CMA formation.
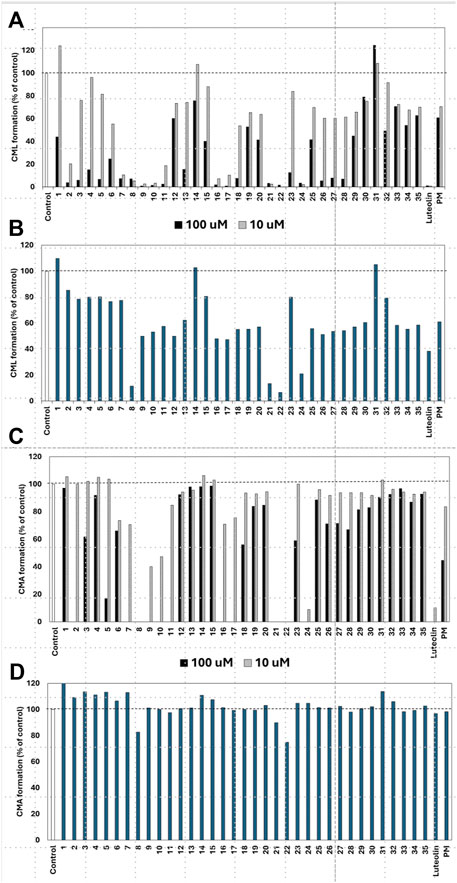
Figure 4. Effect of compounds from Epimedii Herba on CML formation. Gelatin (2.0 mg/mL) and ribose (30 mM) were incubated with the samples [(A): 100 and 10 μM, (B) 1.0 μM] in 10 mM phosphate buffer at 37°C for 7 days. The CML content was determined using noncompetitive ELISA. Effect of compounds from Epimedii Herba on CMA formation. Gelatin (2.0 mg/mL) and ribose (30 mM) were incubated with samples [(C): 100 and 10 μM, (D) 1.0 μM] in 100 mM sodium phosphate buffer at 37°C for 7 days. The CMA content was determined using noncompetitive ELISA (mean ± SD, n = 3).
When the compounds evaluated for activity were divided into glycosides and aglycones, the CML and CMA production inhibitory activities of the aglycons tended to be more pronounced. Compounds with vigorous CML inhibitory activity also showed intense CMA inhibitory activity, especially aglycones of the flavanone skeleton without an oxygen functional group at the three-position. However, among the glycosides, icariside I (2) and epimedoside C (5), in which glucose is attached only at the seven-position of the flavonol backbone, were active (Figures 4A, C). In contrast, no activity was observed for limonianin (6) or epimedokoreanin C (EK-C, 23), even as aglycones. The γ,γ-dimethylallyl group was on the dimethylpyrane ring of 6. Comparing 6 with epimedonin C (16), 16 showed activity even when a dimethylpyran ring was present on the B ring. In contrast, 16 and 23, which share the same A- and C-ring moieties, showed a marked decrease in activity when the B ring became a cyclopentane ring. Furthermore, when comparing the activity at lower concentrations, epimedokoreanin B (8), epimedonin E (21), epicornunin B (22), and F (24) (Figure 4B) showed greater muscular CML inhibitory activity at 1 μM than PM and luteolin, which were used as control drugs. These four compounds also showed vigorous CMA production inhibitory activity of more than 80% at 10 μM (Figure 4C). Based on these results, the standard chemical structure of prenylflavonoids with both CML and CMA formation inhibitory activity is an aglycon of the luteolin-type flavanone skeleton, with prenyl groups at the eight-position of the A ring and the 5′position of the B ring and a catechol group on the B ring.
Instead of gelatin, type I collagen, which is present in the dermis, has been used to inhibit CML and CMA production. Four compounds, EK-B (8), epimedonin E (21), epicornunin B (22), and F (24) that showed significant inhibitory activity in the gelatin evaluation system, were used in this experiment. Prenylflavonoids (8, 21, 22, and 24) in Figure 5A were added to a mixture of ribose (30 mM) and type I collagen (1.5 mg/mL), incubated at 37°C for 7 days, and then measured by ELISA using monoclonal anti-CML and anti-CMA antibodies. The amounts of CML and CMA generated in the type I collagen were determined by ELISA using monoclonal anti-CML and anti-CMA antibodies, respectively (Figure 5B; C). The results showed that the four prenylflavonoid compounds in EH had significant inhibitory activity against both CML and CMA. In particular, EK-B (8), epimedonin E (21), and epicornunin B (22) almost wholly inhibited CML formation even at concentrations of 1 μM (Figure 5B). These compounds also inhibit CMA entirely at a concentration of 10 μM (Figure 5C). In contrast, epicornunin F (24) showed inhibitory activity against CML and CMA formation, comparable to that of luteolin, which was used as a control drug.
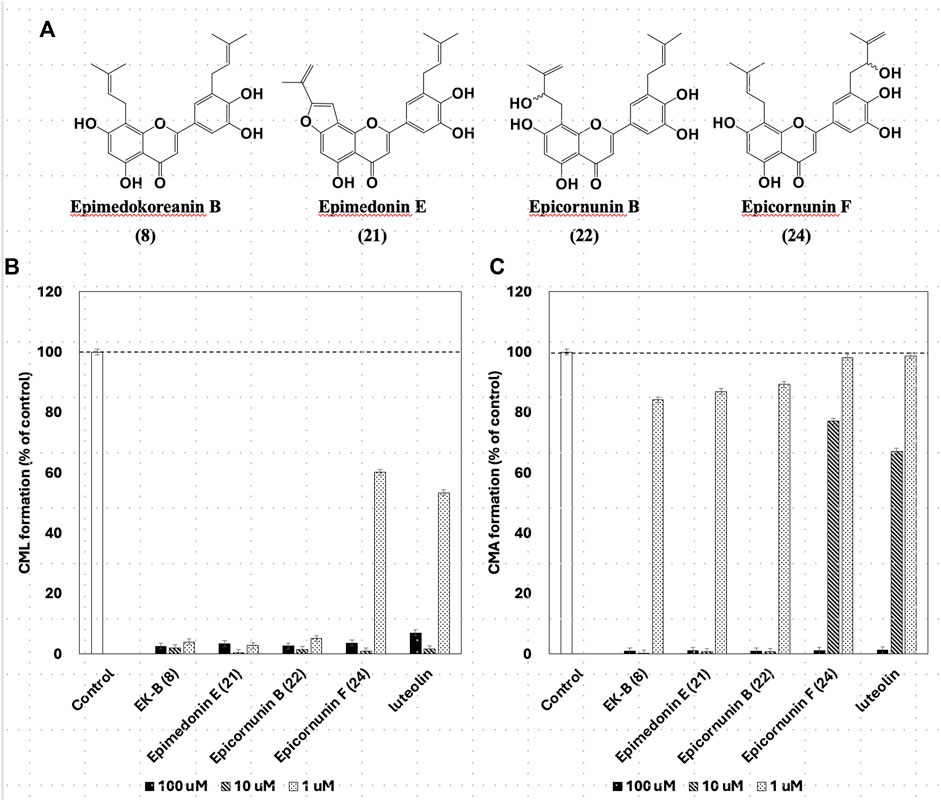
Figure 5. (A) The structures have strong inhibition activity of CML and CML formation. Effect of compounds from Epimedii Herba on CML (B) and CMA (C) formation. Type I collagen (1.5 mg/mL) and ribose (30 mM) were incubated with the compounds (100, 10, and 1 μM) in (B) 10 mM phosphate buffer; in (C) 100 mM sodium phosphate buffer at 37°C for 7 days. The CML and CMA content was determined using noncompetitive ELISA (mean ± SD, n = 3).
Gelatin is a hydrolyzed and solubilized form of collagen that has the same amino acid sequence as collagen but a different steric structure. The CML and CMA inhibitory activities of the four prenylflavonoids (Figure 5A) were also observed in collagen, which has a different conformation. In other words, gelatin used as a mediocre protein reproduced the same inhibitory activity as expensive collagen. Thus, the usefulness of gelatin in screening prenylflavonoids for inhibition of CML and CMA production was confirmed.
3.3 Structure–activity relationships on CML and CMA formations
All compounds with significant inhibitory activity against CML and CMA production (Figure 5A) contained a catechol group. To investigate the importance of the catechol group, we methylated the hydroxyl group of 8, which had the most potent inhibitory activity and the highest yield. We then measured the inhibitory effects on CML and CMA formation. First, the four phenol hydroxyl groups of 8 were partially methylated using TMS-diazomethane. The reaction was monitored using silica gel TLC and stopped when the fully methylated form (C) was formed (Figure 6A). The resulting mixture containing the partially methylated product was separated and purified using silica gel to isolate the three products, along with raw material recovery. The chemical structures of the partially methylated forms A, B, and the fully methylated form C were determined from various NMR data. The yield of each compound was 11% for A, 25% for B, and 19% for C. The derivatives obtained (A–C) were tested for their CML and CMA formation inhibitory activities. All the methylated compounds showed lower CML and CMA formation inhibitory activities than 8 (Figures 6B, C). Compared to compounds A, B, and C, the CML formation inhibitory activity (Figure 6B, at 10 μM) was significantly reduced when the hydroxyl group attached to the C-5 in ring A was methylated as shown in compound C. Silica gel TLC (Hexane: acetone = 2:1 (v/v)) analysis of compound B, in which the hydroxyl group at position five of the B ring remains, and the fully methylated compound C showed that the Rf value of compound B was 0.75. In contrast, that of compound C was as low as 0.20. Furthermore, in HPLC analysis [column: YMC C18 (ϕ10 × 250 mm); flow rate: 2.0 mL/min; temperature: 40 °C; detection: RI], the retention time of compound C was 14.5 min, which was shorter than that of compound B (19.9 min). Thus, methylation of the hydroxyl group at the five-position of the A ring causes a marked change in the chemical and physical properties owing to the loss of the hydrogen bond with the carbonyl oxygen at the four-position of the C ring. Comparison of the CML and CMA inhibitory activities of eight reaffirmed the importance of the catechol group of the flavonoid B ring. They suggested that the hydrogen bond between the hydroxyl group at the five-position of the A ring and the carbonyl group at the four-position of the C ring is essential for the inhibitory activity against CML and CMA formation. Next, we focused on the prenyl group, a substructure other than the catechol group of 8, which exhibits inhibitory activity. Therefore, we examined its contribution to the inhibitory activities of CML and CMA formation. In this study, three prenylated cinnamic acid derivatives, artepillin C, baccharin, and drupanin (Figure 7A), which are the main components of propolis (Rodrigues et al., 2020), were used as prenyl-related compounds for the inhibitory activity test. As shown in Figures 7B, C, the prenylated cinnamic acid derivatives used in this study did not inhibit CML or CMA formation. This suggests that neither prenylated cinnamic acid derivatives (two prenyl groups for artepillin C and one for drupanin) nor their aromatic esters (baccharin) were active; rather, the binding of prenyl groups to the flavonoid backbone, which contains phenolic hydroxyl groups, was responsible for the inhibition of CML and CMA formation. This suggests that the binding of a prenyl group to the flavonoid skeleton may enhance the inhibitory activity against CML and CMA formation.
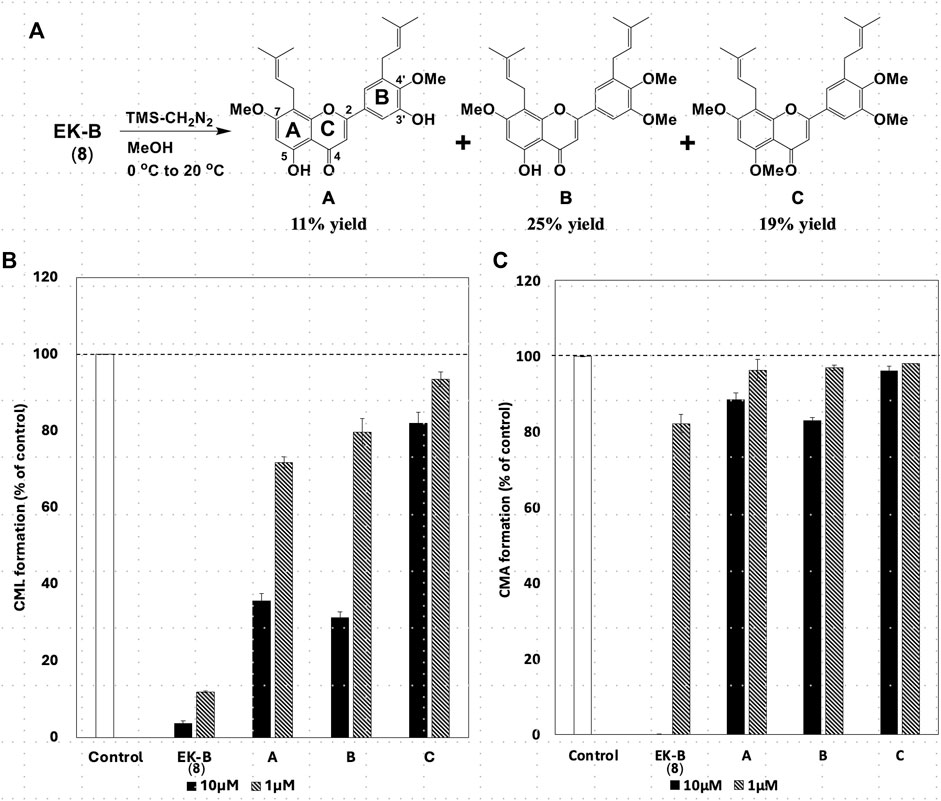
Figure 6. (A) Partial methylation of EK-B (8). Effect of methylated compounds (A–C) derived from EK-B (8) on (B) CML and (C) CMA formation. Gelatin (2.0 mg/mL) and ribose (30 mM) were incubated with the compounds (10 and 1 μM) in (B) 10 mM phosphate buffer; in (C) 100 mM sodium phosphate buffer at 37°C for 7 days. The CML and CMA content was determined using noncompetitive ELISA. Data are presented as the mean ± SD.
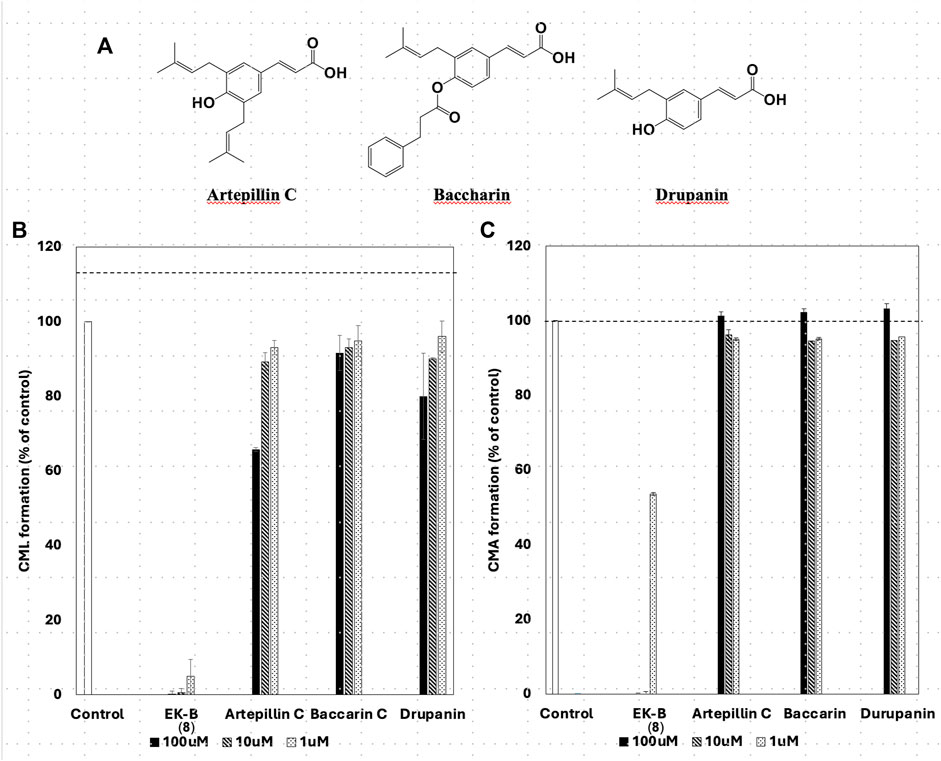
Figure 7. Chemical structures of prenylated cinnamic acid derivatives (PCAs) (A) artepillin C, baccharin, and drupanin. Comparative study of the effect of EK-B (8) and PCAs on (B) CML and (C) CMA formation. Gelatin (2.0 mg/mL) and ribose (30 mM) were incubated with the compounds (100, 10, and 1 μM) in (B) 10 mM phosphate buffer; in (C) 100 mM sodium phosphate buffer at 37°C for 7 days. The CML and CMA content was determined using noncompetitive ELISA. Data are presented as the mean ± SD.
In summary, the structure–activity relationship of the prenyl-related compounds from EH revealed that a catechol group in the B ring and prenyl groups at the eight and 5′positions are essential for the inhibitory activity against CML and CMA formation, that the hydroxyl group at the five-position is hydrogen bonded, and that the three-position does not have an oxygen functional group. In other words, compound 8, which had the highest yield (0.084%) and the most potent inhibitory activity against CML and CMA formation, was found to be the active compound in the extract. Our findings are still experimental results at the test-tube level. However, we were able to clarify the partial structure of the prenylflavonoids required for anti-glycation activity. Although further studies should be conducted in animal models of diseases related to diabetes, atherosclerosis, and osteoporosis, these results suggest that compound 8 could be used as a therapeutic compound because it inhibits AGE formation and prevents the development of diabetic complications, such as diabetic nephropathy, retinopathy, and neuropathy, and age-related diseases, such as Alzheimer’s disease.
Data availability statement
The raw data supporting the conclusion of this article will be made available by the authors, without undue reservation.
Author contributions
KN: Conceptualization, Data curation, Formal Analysis, Funding acquisition, Investigation, Methodology, Writing–original draft, Writing–review and editing. HM: Conceptualization, Data curation, Formal Analysis, Investigation, Methodology, Writing–review and editing. HY: Conceptualization, Methodology, Supervision, Writing–review and editing. YF: Conceptualization, Data curation, Formal Analysis, Investigation, Methodology, Writing–review and editing. RN: Conceptualization, Methodology, Resources, Supervision, Writing–review and editing. TI: Conceptualization, Data curation, Formal Analysis, Funding acquisition, Investigation, Methodology, Project administration, Resources, Supervision, Writing–original draft, Writing–review and editing.
Funding
The author(s) declare that financial support was received for the research, authorship, and/or publication of this article. This work was supported by JSPS KAKENHI, Grant Numbers 20K07122 to TI and 22K20730 to KN.
Acknowledgments
We would like to thank Editage (http://www.editage.com) for editing and reviewing this manuscript for English language.
Conflict of interest
The authors declare that the research was conducted in the absence of any commercial or financial relationships that could be construed as a potential conflict of interest.
Publisher’s note
All claims expressed in this article are solely those of the authors and do not necessarily represent those of their affiliated organizations, or those of the publisher, the editors and the reviewers. Any product that may be evaluated in this article, or claim that may be made by its manufacturer, is not guaranteed or endorsed by the publisher.
Supplementary material
The Supplementary Material for this article can be found online at: https://www.frontiersin.org/articles/10.3389/fchem.2024.1407934/full#supplementary-material
Abbreviations
AGEs, Advanced glycation end products; EH, Epimedii Herba; HMBCs, Heteronuclear multiple bond correlations; HMQ, Heteronuclear multiple quantum coherence; HDL, High density lipoprotein; HRESIMS, High-resolution electrospray ionization mass spectrometry; MeOH, Methanol; CMA, Nω-(carboxymethyl) arginine; CML, Nε-(carboxymethyl)lysine.
References
Aljohi, A., Matou-Nasri, S., Liu, D., Al-Khafaji, N., Slevin, M., and Ahmed, N. (2018). Momordica charantia extracts protect against inhibition of endothelial angiogenesis by advanced glycation endproducts in vitro. Food and Funct. 9 (11), 5728–5739. doi:10.1039/C8FO00297E
Al-Musayeib, N., Perveen, S., Fatima, I., Nasir, M., and Hussain, A. (2011). Antioxidant, anti-glycation and anti-inflammatory activities of phenolic constituents from Cordia sinensis. Molecules 16 (12), 10214–10226. doi:10.3390/molecules161210214
Araki, N., Ueno, N., Chakrabarti, B., Morino, Y., and Horiuchi, S. (1992). Immunochemical evidence for the presence of advanced glycation end products in human lens proteins and its positive correlation with aging. J. Biol. Chem. 267 (15), 10211–10214. doi:10.1016/s0021-9258(19)50004-7
Bacher, M., Brader, G., Greger, H., and Hofer, O. (2010). Complete 1H and 13C NMR data assignment of new constituents from Severinia buxifolia. Magn. Reson Chem. 48 (1), 83–88. doi:10.1002/mrc.2548
Barnard, N. D., Bush, A. I., Ceccarelli, A., Cooper, J., de Jager, C. A., Erickson, K. I., et al. (2014). Dietary and lifestyle guidelines for the prevention of Alzheimer's disease. Neurobiol. Aging 35, S74–S78. doi:10.1016/j.neurobiolaging.2014.03.033
Brandt, I. A. G., Jessen, M. H., Rimestad, D. E., Højgaard, M. K. F., and Vestergaard, P. (2022). Advanced glycation end products and bone - how do we measure them and how do they correlate with bone mineral density and fractures? A systematic review and evaluation of precision of measures. Bone 165, 116569. doi:10.1016/j.bone.2022.116569
Chen, X. J., Tang, Z. H., Li, X. W., Xie, C. X., Lu, J. J., and Wang, Y. T. (2015). Chemical constituents, quality control, and bioactivity of Epimedii folium (yinyanghuo). Am. J. Chin. Med. 43 (5), 783–834. doi:10.1142/s0192415x15500494
Choi, H. M., Kim, J. Y., Li, Z. P., Jenis, J., Ban, Y. J., Baiseitova, A., et al. (2019). Effectiveness of prenyl group on flavonoids from Epimedium koreanum nakai on bacterial neuraminidase inhibition. Molecules 24 (2), 317. doi:10.3390/molecules24020317
Dong, X., Fan, Y., Yu, L., and Hu, Y. (2007). Synthesis of four natural prenylflavonoids and their estrogen-like activities. Arch. Pharm. Weinh. 340 (7), 372–376. doi:10.1002/ardp.200700057
Guo, J., Li, F., Wu, Q., Gong, Q., Lu, Y., and Shi, J. (2010). Protective effects of icariin on brain dysfunction induced by lipopolysaccharide in rats. Phytomedicine 17 (12), 950–955. doi:10.1016/j.phymed.2010.03.007
Harris, C. S., Beaulieu, L.-P., Fraser, M.-H., McIntyre, K. L., Owen, P. L., Martineau, L. C., et al. (2011). Inhibition of advanced glycation end product formation by medicinal plant extracts correlates with phenolic metabolites and antioxidant activity. Planta Med. 77 (02), 196–204. doi:10.1055/s-0030-1250161
Iijima, K., Murata, M., Takahara, H., Irie, S., and Fujimoto, D. (2000). Identification of Nω-carboxymethylarginine as a novel acid-labile advanced glycation end product in collagen. Biochem. J. 347, 23–27. Pt 1. doi:10.1042/0264-6021:3470023
Jin, Q., Lee, C., Lee, J. W., Yeon, E. T., Lee, D., Han, S. B., et al. (2014). 2-Phenoxychromones and prenylflavonoids from Epimedium koreanum and their inhibitory effects on LPS-induced nitric oxide and interleukin-1β production. J. Nat. Prod. 77 (7), 1724–1728. doi:10.1021/np400831p
Kim, J.-Y., and Shim, S. H. (2019). Epimedium koreanum extract and its flavonoids reduced atherosclerotic risk via suppressing modification of human HDL. Nutrients 11 (5), 1110. doi:10.3390/nu11051110
Kinoshita, S., Mera, K., Ichikawa, H., Shimasaki, S., Nagai, M., Taga, Y., et al. (2019). Nω-(Carboxymethyl)arginine is one of the dominant advanced glycation end products in glycated collagens and mouse tissues. Oxidative Med. Cell. Longev. 2019, 9073451. doi:10.1155/2019/9073451
Kitamura, K. I., Hirayama, J., Tabuchi, Y., Minami, T., Matsubara, H., Hattori, A., et al. (2021). Glyoxal-induced formation of advanced glycation end-products in type 1 collagen decreases both its strength and flexibility in vitro. J. Diabetes Investig. 12 (9), 1555–1559. doi:10.1111/jdi.13528
Li, B., Lima, M. R. M., Nie, Y., Xu, L., Liu, X., Yuan, H., et al. (2021). HPLC-DAD fingerprints combined with multivariate analysis of Epimedii folium from major producing areas in eastern asia: effect of geographical origin and species. Front. Pharmacol. 12, 761551. doi:10.3389/fphar.2021.761551
Li, C., Li, Q., Mei, Q., and Lu, T. (2015a). Pharmacological effects and pharmacokinetic properties of icariin, the major bioactive component in Herba Epimedii. Life Sci. 126, 57–68. doi:10.1016/j.lfs.2015.01.006
Li, J.-Y., Li, H.-M., Liu, D., Chen, X.-Q., Chen, C.-H., and Li, R.-T. (2016). Three new acylated prenylflavonol glycosides from Epimedium koreanum. Phytochem. Lett. 17, 206–212. doi:10.1016/j.phytol.2016.07.029
Li, L. R., Sethi, G., Zhang, X., Liu, C. L., Huang, Y., Liu, Q., et al. (2022). The neuroprotective effects of icariin on ageing, various neurological, neuropsychiatric disorders, and brain injury induced by radiation exposure. Aging (Albany NY) 14 (3), 1562–1588. doi:10.18632/aging.203893
Li, W., Zhang, R., and Xiao, P. (1994). Epimedokoreanin B and epimedokoreanin C from the aerial parts of Epimedium koreanum nakai. Acta Pharm. Sin. 29 (11), 835–839.
Li, W.-K., Xiao, P.-G., and Pan, J.-Q. (1998). Complete assignment of 1H and 13C NMR spectra of ikarisoside A and epimedoside C. Magnetic Reson. Chem. 36 (4), 303–304. doi:10.1002/(SICI)1097-458X(199804)36:4<303::AID-OMR187>3.0.CO;2-I
Li, X., Zhou, C., Chen, C. H., Li, R. T., and Lee, K. H. (2015b). Flavonoids isolated from heat-processed Epimedium koreanum and their anti-HIV-1 activities. Helvetica Chim. Acta 98, 1177–1187. doi:10.1002/hlca.201500123
Lin, C.-C., Li, C.-I., Liu, C.-S., Lin, W.-Y., Fuh, M.M.-T., Yang, S.-Y., et al. (2011). Impact of lifestyle-related factors on all-cause and cause-specific mortality in patients with type 2 diabetes: the taichung diabetes study. Diabetes Care 35 (1), 105–112. doi:10.2337/dc11-0930
Ma, H., He, X., Yang, Y., Li, M., Hao, D., and Jia, Z. (2011). The genus Epimedium: an ethnopharmacological and phytochemical review. J. Ethnopharmacol. 134 (3), 519–541. doi:10.1016/j.jep.2011.01.001
Mera, K., Fujiwara, Y., Otagiri, M., Sakata, N., and Nagai, R. (2008). Immunological Detection of nω-(carboxymethyl)arginine by a specific antibody. Ann. N. Y. Acad. Sci. 1126, 155–157. doi:10.1196/annals.1433.000
Mizuno, M., Sakakibara, N., Hanioka, S., Iinuma, M., Tanaka, T., Liu, X.-S., et al. (1988). Flavonol glycosides from Epimedium sagittatum. Phytochemistry 27 (11), 3641–3643. doi:10.1016/0031-9422(88)80783-0
Nakahara, K., Roy, M. K., Ono, H., Maeda, I., Ohnishi-Kameyama, M., Yoshida, M., et al. (2003). Prenylated flavanones isolated from flowers of Azadirachta indica (the neem tree) as antimutagenic constituents against heterocyclic amines. J. Agric. Food Chem. 51 (22), 6456–6460. doi:10.1021/jf034666z
Nakashima, K., Miyashita, H., Yoshimitsu, H., Fujiwara, Y., Nagai, R., and Ikeda, T. (2016). Two new prenylflavonoids from Epimedii Herba and their inhibitory effects on advanced glycation end-products. J. Nat. Med. 70 (2), 290–295. doi:10.1007/s11418-015-0962-0
Ohtani, I., Kusumi, T., Kashman, Y., and Kakisawa, H. (1991). High-field FT NMR application of Mosher's method. The absolute configurations of marine terpenoids. J. Am. Chem. Soc. 113 (11), 4092–4096. doi:10.1021/ja00011a006
Oshima, Y., Okamoto, M., and Hikino, H. (1987). Epimedins A, B and C, flavonoid glycosides of Epimedium koreanum herbs. Heterocycles 26, 935–938. doi:10.3987/r-1987-04-0935
Pang, X., Yin, S. S., Yu, H. Y., Zhang, Y., Wang, T., Hu, L. M., et al. (2018). Prenylated flavonoids and dihydrophenanthrenes from the leaves of Epimedium brevicornu and their cytotoxicity against HepG2 cells. Nat. Prod. Res. 32 (19), 2253–2259. doi:10.1080/14786419.2017.1405410
Qian, H. Q., Wu, D. C., Li, C. Y., Liu, X. R., Han, X. K., Peng, Y., et al. (2024). A systematic review of traditional uses, phytochemistry, pharmacology and toxicity of Epimedium koreanum Nakai. J. Ethnopharmacol. 318, 116957. Pt B. doi:10.1016/j.jep.2023.116957
Rabbani, N., and Thornalley, P. J. (2018). Advanced glycation end products in the pathogenesis of chronic kidney disease. Kidney Int. 93 (4), 803–813. doi:10.1016/j.kint.2017.11.034
Rodrigues, D. M., De Souza, M. C., Arruda, C., Pereira, R. A. S., and Bastos, J. K. (2020). The role of baccharis dracunculifolia and its chemical profile on green propolis production by Apis mellifera. J. Chem. Ecol. 46 (2), 150–162. doi:10.1007/s10886-019-01141-w
Schleicher, E. D., Wagner, E., and Nerlich, A. G. (1997). Increased accumulation of the glycoxidation product N(epsilon)-(carboxymethyl)lysine in human tissues in diabetes and aging. J. Clin. Investigation 99 (3), 457–468. doi:10.1172/JCI119180
Sugawa, H., Ohno, R.-i., Shirakawa, J.-i., Nakajima, A., Kanagawa, A., Hirata, T., et al. (2016). Eucommia ulmoides extracts prevent the formation of advanced glycation end products. Food and Funct. 7 (6), 2566–2573. doi:10.1039/C5FO01563D
Sun, P., Chen, Y., Shimizu, N., and Takeda, T. (1998). Studies on the constituents of Epimedium koreanum. III. Chem. Pharm. Bull. 46 (2), 355–358. doi:10.1248/cpb.46.355
Tabopda, T. K., Ngoupayo, J., Awoussong, P. K., Mitaine-Offer, A. C., Ali, M. S., Ngadjui, B. T., et al. (2008). Triprenylated flavonoids from Dorstenia psilurus and their α-glucosidase inhibition properties. J. Nat. Prod. 71 (12), 2068–2072. doi:10.1021/np800509u
Tominaga, Y., Sugawa, H., Hirabayashi, K., Ikeda, T., Hoshi, Y., and Nagai, R. (2020). Drosera tokaiensis extract containing multiple phenolic compounds inhibits the formation of advanced glycation end-products. Arch. Biochem. Biophys. 693, 108586. doi:10.1016/j.abb.2020.108586
Torres, N., Guevara-Cruz, M., Velázquez-Villegas, L. A., and Tovar, A. R. (2015). Nutrition and atherosclerosis. Archives Med. Res. 46 (5), 408–426. doi:10.1016/j.arcmed.2015.05.010
Vlassara, H., Bucala, R., and Striker, L. (1994). Pathogenic effects of advanced glycosylation: biochemical, biologic, and clinical implications for diabetes and aging. Lab. Invest. 70 (2), 138–151.
Wu, H., Lien, E. J., and Lien, L. L. (2003). Chemical and pharmacological investigations of Epimedium species: a survey. Prog. Drug Res. 60, 1–57. doi:10.1007/978-3-0348-8012-1_1
Xia, Q., Xu, D., Huang, Z., Liu, J., Wang, X., Wang, X., et al. (2010). Preparation of icariside II from icariin by enzymatic hydrolysis method. Fitoterapia 81 (5), 437–442. doi:10.1016/j.fitote.2009.12.006
Yuan, P., Chen, W., Wang, X., Li, L., Peng, Z., Mu, S., et al. (2023). RAGE: a potential target for Epimedium’s anti-neuroinflammation role in vascular dementia—insights from network pharmacology and molecular simulation. J. Biomol. Struct. Dyn., 1–20. doi:10.1080/07391102.2023.2259480
Zhang, H., Zhao, T., Yin, W., and Wu, M. (2007). An NMR study on 3"'-Carbonyl-2"-β-L-quinovosyl IcarisideⅡ. Chin. J. MAGNETIC Reson. 24 (1), 1–8.
Zhang, P. C., Wang, S., Wu, Y., Chen, R. Y., and Yu, D. Q. (2001). Five new diprenylated flavonols from the leaves of Broussonetia kazinoki. J. Nat. Prod. 64 (9), 1206–1209. doi:10.1021/np010283o
Zhao, H.-Y., Fan, L., Zhou, L., Han, J., Wang, B.-R., and Guo, D.-A. (2007). 8-(3,3-Dimethylallyl)-Substituted flavonoid glycosides from the aerial parts of Epimedium koreanum. Helvetica Chim. Acta 90 (11), 2186–2195. doi:10.1002/hlca.200790226
Zhao, S. Y., Liao, L. X., Tu, P. F., Li, W. W., and Zeng, K. W. (2019). Icariin inhibits AGE-induced injury in PC12 cells by directly targeting apoptosis regulator bax. Oxid. Med. Cell Longev. 2019, 1–12. doi:10.1155/2019/7940808
Zhao, Y.-D., Zhang, X., Yang, W.-Y., Zhang, R.-Q., Mu, L.-T., Han, L., et al. (2022). New anti-pulmonary fibrosis prenylflavonoid glycosides from Epimedium koreanum. Chin. J. Nat. Med. 20 (3), 221–228. doi:10.1016/S1875-5364(21)60116-4
Keywords: Epimedii Herba, prenylflavonoid, advanced glycation end products, N ε -(carboxymethyl)lysine, N ω -(carboxymethyl) arginine
Citation: Nakashima K, Miyashita H, Yoshimitsu H, Fujiwara Y, Nagai R and Ikeda T (2024) Prenylflavonoids isolated from Epimedii Herba show inhibition activity against advanced glycation end-products. Front. Chem. 12:1407934. doi: 10.3389/fchem.2024.1407934
Received: 27 March 2024; Accepted: 07 May 2024;
Published: 31 May 2024.
Edited by:
Bun Chan, Nagasaki University, JapanReviewed by:
Aizhamal Baiseitova, Gyeongsang National University, Republic of KoreaBing Chen, Fujian Medical University, China
Copyright © 2024 Nakashima, Miyashita, Yoshimitsu, Fujiwara, Nagai and Ikeda. This is an open-access article distributed under the terms of the Creative Commons Attribution License (CC BY). The use, distribution or reproduction in other forums is permitted, provided the original author(s) and the copyright owner(s) are credited and that the original publication in this journal is cited, in accordance with accepted academic practice. No use, distribution or reproduction is permitted which does not comply with these terms.
*Correspondence: Tsuyoshi Ikeda, dGlrZWRhQHBoLnNvam8tdS5hYy5qcA==