- 1Ministry of Health and Social Protection, Higher Institute of Nursing Professions and Health Techniques, Guelmim, Morocco
- 2National Agency of Medicinal and Aromatic Plants, Taounate, Morocco
- 3Laboratory of Cell Biology and Molecular Genetics (LBCGM), Department of Biology, Faculty of Sciences, Faculty of Sciences, Ibn Zohr University, Agadir, Souss-Massa, Morocco
- 4Ministry of Health and Social Protection, Higher Institute of Nursing Professions and Health Techniques, Fez, Morocco
- 5Department of Chemistry, University of Helsinki, Helsinki, Finland
- 6Department of Pharmacognosy, College of Pharmacy, King Saud University, Riyadh, Saudi Arabia
- 7Laboratory of Biotechnology, Health, Agrofood and Environment (LBEAS), Faculty of Sciences Dhar El Mehraz, Sidi Mohamed Ben Abdellah University, Fez, Morocco
Introduction: The aim of this study was to evaluate the antioxidant, antimicrobial, and preservative efficacy of Thymus broussonetii Boiss. essential oil (EO) in a topically applied formulation using a challenge test.
Methods: The essential oil was extracted from the aerial part of T. broussonetii using hydrodistillation, and the obtained EO was further analyzed by gas chromatography/mass spectrometry (GC/MS). The antioxidant effect of the EO was evaluated using three methods: the inhibition of free radical 2,2-diphenyl-1-picrylhydrazyl (DPPH), β-carotene–linoleic acid, and the ferric reducing antioxidant power (FRAP) methods. The antimicrobial activity and the minimum inhibitory concentration (MIC) of this EO were assayed by the disk-diffusion method and the broth microdilution method, respectively. The preservative efficacy of T. broussonetii EO was assayed at 1% and 2% (v/w) in a topical cream formulation using a challenge test against standard-specific microorganisms recommended by the European Pharmacopoeia. Furthermore, the identified phytochemical compounds were docked for their effect on nicotinamide adenine dinucleotide phosphate oxidase, human casein kinase 1 alpha 1 (CSNK1A1), glycogen synthase kinase 3, Staphylococcus aureus nucleoside diphosphate kinase, Escherichia coli beta-ketoacyl-[acyl-carrier protein] synthase, Pseudomonas aeruginosa LasR ligand-binding domain, and sterol 14-alpha demethylase (CYP51) from Candida albicans. The ADME/toxicity was predicted by analyzing the absorption, distribution, metabolism, and excretion parameters.
Results and discussion: chemical composition of the EO revealed the presence of thymol (63.09%), p-cymene (11%), and γ-terpinene (8.99%) as the major components. The antioxidant assays revealed that the essential oil exhibited strong antioxidant activity, as indicated by the minimum inhibitory concentration IC50 (IC50 = 210 ± 0.3 μg/mL for the DPPH assay, IC50 = 145 ± 0.1 μg/mL for the β-carotene assay, and IC50 = 84 ± 0.21 μg/mL for the FRAP assay) when compared to quercetin and butylated hydroxytoluene (BHT) as controls. The investigated essential oil exhibited important antimicrobial activity against all the tested microorganisms, and the MICs of the EO against bacteria and fungi were 0.02%–1%. Moreover, the EO of T. broussonetii evaluated at 2% (v/w) in a cream formulation succeeded in satisfying the A criteria for preservation efficacy against S. aureus, E. coli, and Aspergillus brasiliensis but exhibited less efficacy against P. aeruginosa (1.78 log reduction in the number of CFU/g after 7 days of evaluation) and C. albicans (1.09 log reduction in the number of CFU/g after 14 days of evaluation) when compared to the synthetic preservative phenoxyethanol 1% (v/w). In silico results showed that the antimicrobial activity of T. broussonetii EO is mostly attributed to thymol, terpinen-4-ol, and aromadendrene, while the antioxidant activity is attributed to thymol. These results indicate that the EO of T. broussonetii possesses important antimicrobial and antioxidant properties and can, therefore, be used as a natural preservative ingredient in the cosmetic industry.
1 Introduction
The global market of food, cosmetics, and pharmaceutical products requires continuous tracking of harmful ingredients and microbial contamination for the sake of the safety of both the products and consumers, as these products greatly dominate the consumer’s health, directly or indirectly. Microbial contamination can lead to product degradation, constitute a risk to the health of the consumer, and potentially spread infection. For these reasons, the microbiological safety of pharmaceutical and cosmetic products must be of special interest to the industries (Hye et al., 2020). Preservatives are substances used to limit microbial growth and survival in cosmetic and pharmaceutical products in order to prolong their shelf life (Nowak-Lange et al., 2022). In recent years, there has been considerable interest in the search for natural antimicrobial alternatives, such as essential oils, to avoid the use of chemical agents (Johnson et al., 2000; Seo et al., 2002). Several studies have shown that thyme oils possess antimicrobial properties, with those of the phenol compound type being the most active (Labiad et al., 2022; Pinto et al., 2006; Saad et al., 2010; El Bouzidi et al., 2013). Nowadays, the use of synthetic preservatives has become common and has not been widely accepted by consumers, as they are aware that exposure to preservatives can lead to adverse effects on health, which is a major area of concern for researchers.
Chemicals with antimicrobial properties used as ingredients in cosmetics ensure their durability and safety. Polyphenolic compounds, peptides, essential oils, and plant extracts characterized by these properties are natural ingredients that can replace the synthetic components of cosmetics. The advantage of these compounds is that they exhibit antioxidant, anti-inflammatory, and soothing properties, enhancing the product’s value in addition to their antimicrobial properties.
Recently, essential oils (EOs) have gained increasing attention due to their therapeutic and preservative properties, which are beneficial for health and are generally considered safe according to the United States Agency for Food and Drug Products (Fancello et al., 2016). The EOs are natural substances that are composed of volatile secondary metabolites (Salehi et al., 2018). The study of the EOs’ chemical composition and the evaluation of their biological activities are necessary to confirm their use as preservatives in the food, pharmaceutical, and cosmetic fields. The genus Thymus is considered to be one of the eight most important genera in the Lamiaceae family, comprising approximately 215 species that are native to the Mediterranean basin (Pirbalouti et al., 2015). The majority of these oils are characterized by their richness in oxygenated monoterpenes, in particular, phenolic compounds such as thymol and its isomer carvacrol, accompanied by other more or less biologically active compounds such as eugenol, p-cymene, terpinene, linalool, geraniol, and borneol (Nikolić et al., 2014). Several studies linked Thymus EO’s chemical composition to its antimicrobial and antioxidant activities (Ballester-Costa et al., 2017).
Thymus broussonetii, endemic to Morocco, is an erect shrub with erect stems. Its leaves are stem-like, broadly ovate-lanceolate, and punctuated on both sides. Its flowers are wide and often purple-colored, and it is characterized by dense male inflorescences (Benabid, 2000). The severe exploitation to which this species is exposed can lead to its rarefaction and/or disappearance (Mehdioui and Kahouadji, 2007; Ozudogru et al., 2011). There is no study on the variation in the chemical composition of T. broussonetii EO. The Mediterranean flora, in general, and Morocco, in particular, boast a diversified flora with an endemism rate equal to 878 species, including several species of plants that have been little or not studied at all (Fennane and Tattu, 2008; Rankou et al., 2013). The present study is a contribution to the chemical composition and the antioxidant, antimicrobial, and preservative efficacy of T. broussonetii essential oil (EO) in topical cream formulations using the challenge test proposed by the European Pharmacopoeia against selected standard microorganisms that represent the potential contaminants of cosmetic products during manufacture or use. To the best of our knowledge, this is the first work to evaluate the preservative efficacy of the Moroccan endemic plant T. broussonetii Boiss.
2 Results and discussions
2.1 Chemical analysis
Essential oil obtained from the aerial part of T. broussonetii yielded 2.05% (v/w). The gas chromatography/mass spectrometry (GC/MS) analysis resulted in the identification of 11 compounds, representing more than 99.78% of the total oils and falling into the following three classes: monoterpene hydrocarbons, oxygenated monoterpenes, and sesquiterpene hydrocarbons (Table 1). Oxygenated monoterpenes such as thymol (63.09%) were the dominant constituents, followed by the two monoterpene hydrocarbons p-cymene (11%) and γ-terpinene (8.99%) Figure 1. The results showed that thymol was the most abundant compound in thyme oil. In contrast, p-cymene and γ-terpinene are two precursors of thymol (Nouri and Esmaeilian, 2012).
2.2 Antioxidant activity
The essential oil of T. broussonetii obtained from in vitro cultivated plants (Nordine et al., 2013) was subjected to screening for its possible antioxidant activity by three methods, namely, DPPH free radical scavenging, ferric ion reduction, and β-carotene–linoleic acid assays.
The results are illustrated in Table 2. EOs are qualified as natural antioxidants because of their ability to reduce and/or prevent the formation of free radicals. According to the IC50 values presented in Table 2, the antioxidant power of quercetin and butylated hydroxytoluene (BHT) is greater than that of the studied thyme. The tested T. broussonetii essential oil exhibited significant antioxidant activity, as indicated by the minimum inhibitory concentration (IC50). In addition, tests show that the EO has a good affinity with Fe3+ ions in the FRAP method. The antioxidant activity of T. broussonetii EO has already been indicated in several works, including Radi et al. (2021), Hamdani et al. (2014), El Bouzidi et al. (2013), and Jamali et al. (2012).
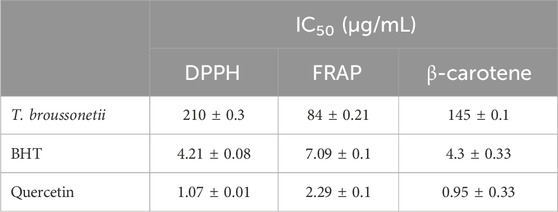
Table 2. IC50 of T. broussonetii EO, quercetin, and of BHT standard measured by DPPH, FRAP, and β-carotene methods. Data are expressed as the mean ± SD. The experiment was performed in a minimum of three replicates.
Furthermore, the results suggest that essential oils from T. broussonetii could be used as natural antioxidants in cosmetics and food systems. Generally, the antioxidant activity is the result of the interaction between all the chemical components of the EO (alcohols, phenols, and terpene and ketone compounds) acting in an antagonistic or synergistic way. Many studies have established an important relationship between the chemical composition of essential oils and their antioxidant activity, and it has been reported that the antioxidant activity of essential oils is related to their chemical compositions, particularly the presence of compounds containing a hydroxyl function as well as terpene alcohols and phenolic compounds (Xiaohua et al., 2023; Bouhdid et al., 2008; Fayed, 2009; Zhuang et al., 2009; Miladi et al., 2013).
According to this study, it is evident that the high antioxidant capacity of the studied EO of T. broussonetii is linked to its content of thymol, ɣ-terpinene, p-cymene, and α-pinene. In addition, the presence of minority compounds such as aromatic alcohols, ethers, ketones, and esters in the studied EO can influence the antioxidant activity.
2.3 Antimicrobial activity
As shown in Tables 3 and 4, the antimicrobial assays indicated that the essential oil was more effective against Gram-positive bacteria than against Gram-negative bacteria; the MICs of the essential oil against bacteria and fungi were 0.02%–1% (Figure 2). However, the essential oil exhibited a significant antibacterial effect against Staphylococcus aureus and Escherichia coli. Furthermore, GC/MS analysis showed that the major compound of the essential oil was thymol, which is proven to be responsible for the antimicrobial properties (Nielsen and Rios, 2000; Kiran Babu et al., 2007).
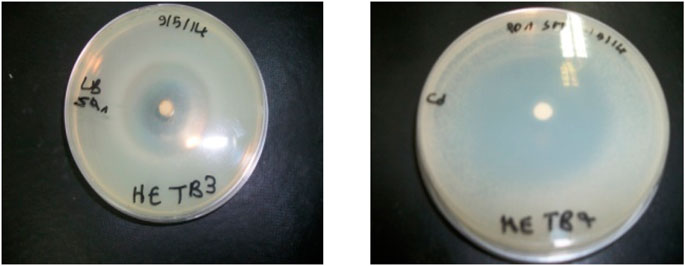
Figure 2. Plate inhibition zone assay showing the antimicrobial activity of Thymus broussonetii essential oil against S. aureus and yeast.
The phenolic compound thymol, which is present in high concentration (63.09%) in our essential oil sample, is well-known for its broad-spectrum antimicrobial activity (Chami et al., 2005; Mothana et al., 2011; Castilho et al., 2012; Kurdelas et al., 2012). Moreover, previous studies have also reported the potent antimicrobial efficacy of thymol (Nostro et al., 2004; Gutierrez-Larrainzar et al., 2012). The presence in the hydroxyl group of thymol and a system of delocalized electrons in its chemical structure is important for its antimicrobial effect against Gram-negative or positive bacteria (Nazzaro et al., 2013; Saad et al., 2013).
The mode of action of thymol, a phenolic monoterpenoid, has received a lot of attention from researchers. However, thymol interacts with the cell membrane, affecting its permeability and leading to the loss of membrane potential, cellular uptake of ethidium bromide, and leakage of potassium ions, ATP, and carboxyfluorescein (Xu et al., 2008). Other constituents have also been reported for their antimicrobial activity, such as (E)-caryophyllene (Maggi et al., 2009; Randrianarivelo et al., 2009; El Bouzidi et al., 2013). Other reports showed that terpinen-4-ol has a higher antibacterial effect than p-cymene (Kasujja, 2021).
2.4 Stability study
The visual appearance of the formulation was checked at the time of preparation and after 1 month. No significant difference in visual appearance was found after 1 month from the time of preparation (Figure 3). The color of the cream formulation at the time of preparation and after 1 month of storage at 25°C, 37°C, and 4°C was observed to be “white.” The pH evaluation is important to check the stability of a cream formulation. The pH values were not different at all temperatures for a period of 28 days. The pH values after this period were 5.7 at 25°C and 4°C and 5.8 at 37°C.
2.5 Challenge test
Preservatives are ingredients used to limit microbial growth and survival in cosmetic products. However, some chemical preservatives present adverse effects, such as hypersensitivity. Chemical preservatives have been replaced by other antimicrobial agents, such as essential oils and extracts (Gutierrez-Larrainzar et al., 2012). Based on the test results of the minimum inhibitory concentration and considering that high concentrations of preservatives can cause some adverse effects, the cream used in the challenge was added to 1% and 2% of T. broussonetii essential oil. The present study indicated a rapid decline in the number of CFU g-1 during the first few times evaluated after the contamination of the cream by all tested microbes (Figure 4, 5).
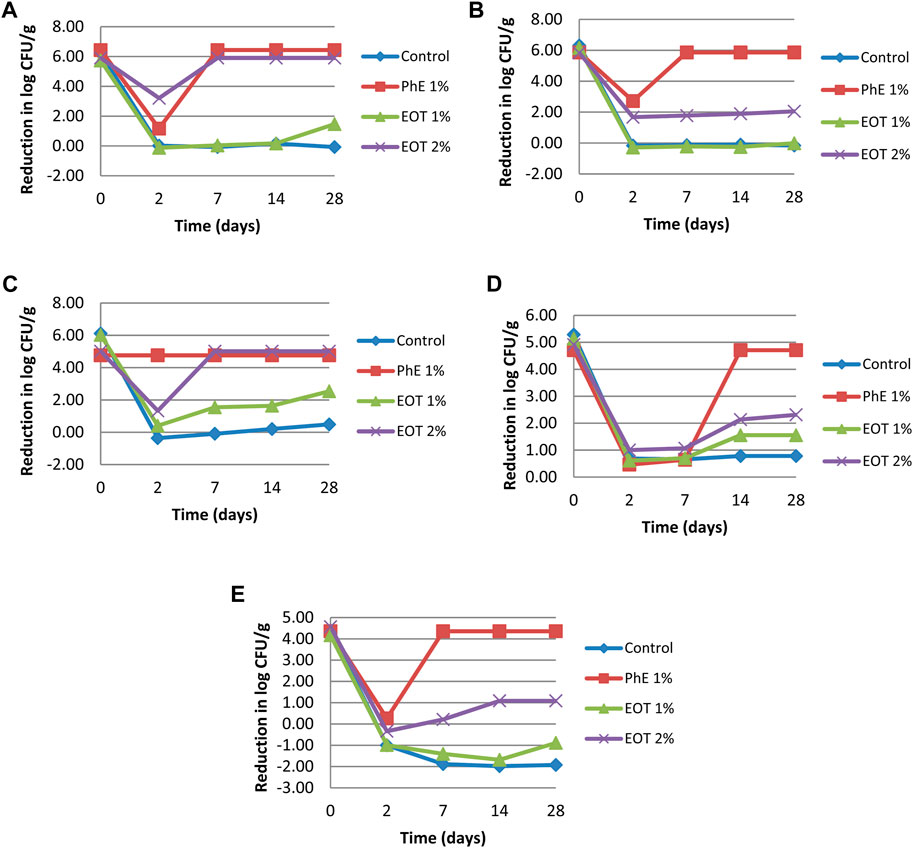
Figure 5. Reduction in log measured during the challenge test for each of the bacterial and fungal strains. (A) S. aureus, (B) P. aeruginosa, (C) E. coli, (D) A. brasiliensis, and (E) C. albicans; EOT 1%, cream with 1% essential oil, EOT 2%, cream with 2% (v/w) essential oil, PhE 1%, cream preserved with 1% (v/w) phenoxyethanol; control, cream without any preservative.
Our results demonstrated that the essential oil of T. broussonetii evaluated at 2% (v/w) in a cream formulation succeeded in satisfying the A criteria for preservation efficacy against all microbial strains (S. aureus, E. coli, and Aspergillus brasiliensis) except Pseudomonas aeruginosa (1.78 log reduction in the number of CFU g-1 after the 7th day of evaluation) and Candida albicans (1.09 log reduction in the number of CFU g-1 after 14 days of evaluation) in the cream study compared to phenoxyethanol 1% (v/w). Compared to other species of thyme, Manou et al. (1998) reported that the challenge test of Thymus vulgaris essential oil at 3% (v/w) revealed an unsatisfactory effect of preservation, and the required criteria were satisfactory only against the bacterial strains and partially against yeast but not against Aspergillus niger.
In general, in bacteria, structural changes in the outer membrane protein (OMP) composition may have an effect on the adhesive ability and pathogenic properties of the organisms. P. aeruginosa can develop an intrinsic resistance to a wide range of biocides, which is associated with the nature of its outer membrane (Verdial et al., 2023). This strain is known to be very persistent and predominant in cases of drug and cosmetic spoilage. In this context, we can explain the unsatisfactory effect of the essential oil of T. broussonetii against P. aeruginosa.
2.6 Molecular docking study
In the study of antioxidant activity, the molecule that demonstrated the highest effectiveness against NADPH was thymol, with a glide score of −6.388 kcal/mol. In the analysis of antimicrobial activity, the most active molecule against S. aureus nucleoside diphosphate kinase was terpinen-4-ol, with a score of −6.008 kcal/mol. Thymol also exhibited significant activity against E. coli beta-ketoacyl-[acyl-carrier protein] synthase, with a score of −6.83 kcal/mol. Additionally, in the case of the P. aeruginosa LasR ligand-binding domain, thymol was identified as the most active molecule, with a glide score of −7.406 kcal/mol. Lastly, the most active molecule against C. albicans was aromadendrene, with a glide score of −7.084 kcal/mol (Table 5).
Thymol is the most active molecule responsible for antioxidant and antimicrobial activities. In the active site of NADPH, thymol established a single hydrogen bond with the residue VAL 214, while it established two hydrogen bonds in the active site of glycogen synthase kinase 3 with the residue VAL 135. Moreover, in the active site of E. coli beta-ketoacyl-[acyl-carrier protein] synthase, it established a single hydrogen bond with the residue THR 302 and a Pi–Pi stacking bond with the residue HIE 298 (Figure 6).
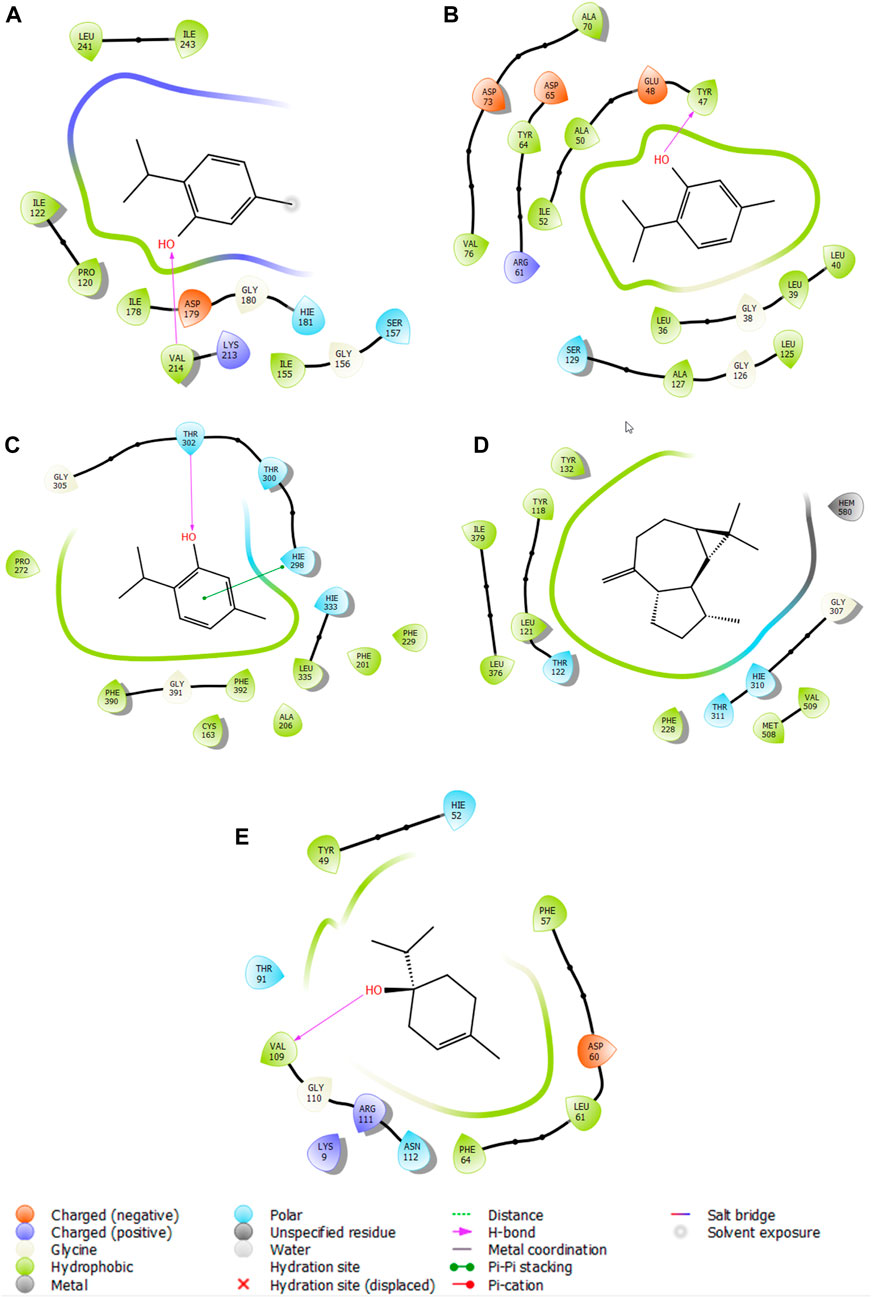
Figure 6. 2D viewer of ligand interactions with the active site. (A–C) Thymol interactions with the active site of NADPH, P. aeruginosa LasR ligand-binding domain, and E. coli beta-ketoacyl-[acyl-carrier protein] synthase, respectively. (D) Aromadendrene interactions with the active site of C. albicans. (E) Terpinen-4-ol interactions with the active site of S. aureus nucleoside diphosphate kinase.
5-Oxotetrahydrofuran-2-carboxylic acid was the most active molecule in the active site of human casein kinase 1 alpha 1, and it established two hydrogen bonds with residues ARG 186 and LYS 225 and a salt bridge type bond with residue LYS 225. Meanwhile, terpinen-4-ol was the most dynamic molecule in the active site of S. aureus nucleoside diphosphate kinase, and it established a single hydrogen bond with the residue VAL 109 (Figure 6).
2.7 ADME/toxicity prediction
The bioavailability of an active compound is determined by its absorption, distribution, metabolism, and excretion (ADME), and it is influenced by the physicochemical properties of the compound. All compounds identified in the T. broussonetii EO were evaluated for their ADME properties, with all compounds having a molecular mass below 500 mol. The number of donors and acceptors of hydrogen bonds for the essential oil were within the acceptable limits (≤5 and ≤10, respectively) (Table 6). The total surface area accessible to the solvent affects the oral bioavailability, and all molecules had acceptable values ranging from 300 to 1,000. The blood–brain partition coefficient determines a molecule’s ability to cross the blood–brain barrier, with an acceptable range for the predicted coefficient being −3 to 1.2. All the selected molecules met this criterion. The predicted percentage of oral absorption for all the compounds was approximately 100%, as indicated in Table 6.
Toxicity studies are important for evaluating the potential harm that a substance or product can cause to living organisms, including humans, animals, and the environment. These studies are critical for ensuring the safety of drugs, chemicals, pesticides, food additives, and consumer products.
In our study, the prediction of the compounds identified in T. broussonetii EO showed potential immunotoxicity for (E)-caryophyllene, mitochondrial membrane potential toxicity for thymol, and carcinogenicity for p-cymene (Table 7).
ProTox-II is a tool used for predicting the toxicity of chemical compounds. It is based on a set of predictive models that use various chemical and molecular descriptors to estimate the potential toxicity of a given compound. These models were developed using machine learning techniques and are trained on large datasets of known toxic and non-toxic compounds. The models used in ProTox-II are designed to predict various types of toxicity, including acute toxicity, skin and eye irritation, and mutagenicity. Tox21 (toxicology in the 21st century), used by ProTox-II, is a research program that aims to develop better ways to predict the potential toxicity of nuclear receptor signaling pathways and stress response pathways.
3 Conclusion
Several types of EOs and their individual components are used as natural antimicrobial compounds in order to reduce the impact of microbial activities in cosmetic products. Thyme essential oil has antimicrobial, antifungal, and antioxidant properties. Its antimicrobial and antioxidant activity has been studied almost exclusively by chemical testing in order to be able to use it for cosmetic preservation purposes. Compared to the Gram-negative bacteria, the Gram-positive bacterial strains are more sensitive to the EO. Thymol, p-cymene, and γ-terpinene are the major components present in the EO, which are responsible for maximizing the antimicrobial activity. According to the IC50 values, the tested T. broussonetii essential oil exhibited important antioxidant activity.
Our results indicate that T. broussonetii essential oil evaluated at 2% (v/w) in a cream formulation succeeded in satisfying the A criteria for preservation efficacy against S. aureus, E. coli, and A. brasiliensis but exhibited less efficacy against P. aeruginosa and C. albicans compared to the synthetic preservative phenoxyethanol at 1% (v/w). The molecular docking study revealed that T. broussonetii compounds might exert an antioxidant effect through NADPH oxidase inhibition. In silico results demonstrated that the antimicrobial activity of T. broussonetii EO is mostly attributed to thymol, terpinen-4-ol, and aromadendrene. These results indicate that the essential oil of T. broussonetii possesses important antimicrobial and antioxidant properties and can, therefore, be used as a natural preservative ingredient in the cosmetic industry.
4 Materials and methods
4.1 Plant material
The aerial parts of T. broussonetii Boiss. (Lamiaceae) obtained from an in vitro cultivated plant (Nordine et al., 2014) were freshly harvested and collected on April 2023 in the experimental garden of the National Agency of Medicinal and Aromatic Plants. This plant was produced through in vitro clonal propagation through direct shoot organogenesis. The plant was identified by a botanist, and a voucher specimen (INP. 564) was deposited at the herbarium of the National Institute of Medicinal and Aromatic Plants (NIMAP), Taounate, Morocco.
4.2 Essential oil extraction
The EO from the aerial part of T. broussonetii was extracted by hydrodistillation using a Clevenger-type apparatus (VWR, Radnor, PA, United States) for 3 hours. The process was repeated three times for each 100 g of the plant sample. The obtained essential oil was dried over anhydrous sodium sulfate. It was then stored at a temperature of 4°C in the dark until it was used.
4.3 Gas chromatography/mass spectrometry analysis of the essential oil
GC/MS analysis was performed using a gas chromatograph (TRACE GC Ultra) (Conquer Scientific, Poway, CA, United States) fitted to a mass spectrometer (Polaris Quadrupole-Ion Trap MS) (Conquer Scientific, Poway, CA, United States) using a Varian capillary column (TR5 CP-Sil 5 CB; 50 m length, 0.32 mm diameter, and film thickness 1.25 µm). Fragmentation was performed by electron impact at 70 eV. Helium (1.5 mL/min) was used as the carrier gas. A split-type injector was heated to a temperature of 200°C. The volume injected was 1 μL. The column temperature was programmed from 40°C to 280°C for 5 °C/min. The components were identified by comparison of their mass spectra with authentic reference compounds (1 mg/mL) where possible, by reference to WILEY275, NIST-MS, and the Adams terpene library (Adams, 2001), and by comparison of their retention time (RT) with those of authentic compounds or literature data. For semi-quantification purposes, the normalized peak area of each compound was used without any correction factors to establish abundances.
4.4 Formulation of the cream
In an attempt to determine the preservative effect exerted by the essential oil, the challenge test was performed in the following four final formulations: cream preserved with 1% essential oil (EOT 1%), cream preserved with 2% essential oil (EOT 2%), cream preserved with 1% synthetic preservative phenoxyethanol (PhE), and the same cream without any preservative as the control group. The composition and pH of the cream are indicated in Table 8.
4.5 Stability study
4.5.1 Visual appearance
The visual appearance (color and texture) of the cream was determined by taking 5 g of cream from each set (stored at 25°C, 37°C, and 4°C) in three transparent glass jars and checking the visual appearance. This process was carried out at the time of cream preparation (at zero time) and thereafter for 1 month.
4.5.2 pH of the formulation
The pH of the formulation was determined using the pH meter (Mettler Toledo Ingold Inc., Billerica, MA) (Bates Roger, 1973). The pH was checked at the time of cream preparation (zero time) and thereafter for 1 month. The cream jars stored at 25°C, 37°C, and 4°C were selected to check the pH of the cream to ensure its stability at different temperatures. Measurements were taken in triplicate. The pH meter was calibrated with standard buffer solutions (pH 4, 7, and 10) before each use.
4.6 Antioxidant activity
4.6.1 DPPH free radical scavenging activity
The DPPH radical-scavenging assay was determined according to the method reported by Burits and Bucar (2000). Essential oil (50 µL) at different concentrations (26.96–863 mg/mL) or MeOH (control) was mixed with 2 mL of 2,2-diphenyl-1-picrylhydrazyl (DPPH) methanol solution (60 mg/L). After 20 min of incubation in darkness at ambient temperature, the absorbance was measured at 517 nm. Butylated hydroxytoluene (BHT) and quercetin were used as the positive controls, all analyses were carried out in triplicate, and the results were expressed as the mean ± SD. The percentage inhibition of the DPPH radical was calculated according to the following formula:
Here, Ab is the absorption of the control sample (DPPH + methanol) and Aa is the absorption of the tested oil. The sample concentration providing 50% inhibition (IC50) was calculated by plotting the inhibition percentages against the concentrations of the sample.
4.6.2 Reducing power determination
The reducing power of the essential oil was determined according to the method reported by Miraliakbari and Shahidi (2008). Different concentrations of the oil in methanol (29.37–940 mg/mL) were mixed with phosphate buffer (500 μL, 0.2 mol/L, pH 6.6) and potassium ferricyanide [K3Fe(CN)6] (500 μL, 1%). The mixture was incubated at 50°C for 20 min. After incubation, 500 µL of trichloroacetic acid (10%, w/v) was added to the mixture to stop the reaction. The mixture was centrifuged at 650 g for 10 min, and then 500 µL of the supernatant was mixed with distilled water (500 µL) and ferric chloride (100 μL, 1 mg/mL). The oil concentration providing 0.5 absorbance (IC50) was calculated by plotting the absorbance at 700 nm against the corresponding oil concentration. BHT and quercetin were used as the reference compounds. The test was carried out in triplicate, and the IC50 values were reported as the means ± SD.
4.6.3 β-Carotene–linoleic acid assay
In this assay, the antioxidant capacity was determined by measuring the inhibition of the volatile organic compounds and the conjugated diene hydroperoxides arising from linoleic acid oxidation (Sahin et al., 2004). A stock solution of the β-carotene–linoleic acid mixture was prepared as follows: 0.5 mg of b-carotene was dissolved in 1 mL of chloroform (HPLC grade), and 25 µL of linoleic acid and 200 µL of Tween 40 were added. Chloroform was completely evaporated using a vacuum evaporator. Then, 100 mL of distilled water saturated with oxygen (30 min, 100 mL min-1) was added with vigorous shaking; 2.5 mL of this reaction mixture was dispersed into test tubes, and 350 µL portions of the essential oil prepared in methanol at different concentrations (19–302.7 μg/mL) were added. The emulsion system was incubated for up to 2 h at 50°C. The same procedure was repeated with the positive control BHT, quercetin, and a blank. After this incubation period, the absorbance of the mixtures was measured at 470 nm. Antioxidant capacities of the extracts were compared with those of BHT and quercetin at the same concentration, and the blank consisted of only 350 µL of methanol.
4.7 Antimicrobial activity
4.7.1 Microbial strains
The standard microbial strains were procured from the American Type Culture Collection (ATCC). The Gram-positive bacteria S. aureus ATCC 29213, Gram-negative bacteria E. coli ATCC 25922 and P. aeruginosa ATCC 27853, yeast C. albicans ATCC 10231, and mold A. brasiliensis ATCC 16404 was used in the microbiological tests and as the challenged microorganisms in the preservative effectiveness test.
4.7.2 Antimicrobial screening
The antimicrobial activity was performed using the agar-disk-diffusion method according to CLSI guidelines with some modifications (CLSI, 2012). Briefly, Petri dishes containing Mueller–Hinton agar (MHA) culture medium and Sabouraud dextrose agar (SDA) culture medium for bacteria and yeast, respectively, were inoculated with the microbial inoculums previously prepared. The disks (filter paper, 6 mm in diameter) placed in the center of each plate were impregnated with 10 μL of each essential oil. Negative control consisted of microbial suspensions and medium culture supplemented with agar (0.15% w/v). Streptomycin (10 µg/disc) was used as the positive control for the tested bacteria. The Petri dishes were placed at 4°C for 2 h to allow a better diffusion of molecules. After the plates were incubated at 37°C for 18–20 h for bacteria and at 30°C for 24–48 h for yeast, the diameters of the inhibition zones were measured (in mm), and the experiments were carried out in triplicate.
4.7.3 Antifungal screening
The antifungal activity was evaluated by agar-well diffusion against A. brasiliensis with slight modification (Schlumbaum et al., 1986). An agar-plot or cylinder was cut aseptically with a sterile cork borer and deposited in the center of the agar surface plate for inoculation. Then, a hole with a diameter of 8 mm was punched aseptically with a sterile cork borer or a tip, and 80 µL of the essential oil solution was introduced into the well. Then, the agar plates were incubated, and the antifungal activity was evaluated by measuring the zone of inhibition of fungal growth surrounding the well in mm.
4.7.4 Minimum inhibitory concentration
The determination of MIC was performed in a 96-well microplate using the micro-dilution assay according to Bouhdid et al. (2009), with slight modifications. First, each essential oil was serially diluted in Mueller–Hinton broth (MHB) for bacteria and Sabouraud dextrose broth (SDB) for fungi, supplemented with agar 0.15% (w/v), which was used as an emulsifier. The 12th well was considered the growth control. Then, 50 μL of bacterial inoculum was added to each well at a final concentration of 106 CFU/mL. After incubation at 37°C for 18–20 h, 5 μL of resazurin was added to each well as the bacterial growth indicator. After further incubation at 37°C for 2 h, the bacterial growth was revealed by the change in coloration from purple to pink. The MIC value was determined as the lowest concentration that prevented a change in resazurin color. Assays were conducted in triplicate.
4.7.5 Minimum microbiocidal concentration
The minimum microbiocidal concentration (MMC) (minimum bactericidal and fungicidal concentration) corresponded to the lowest concentration of the essential oil, yielding negative subcultures after incubation at 37°C for 24 h and 48 h to 5 days for yeast and mold, respectively. It is determined by spotting 2 μL from the negative wells on LB plates. Each assay was also conducted in triplicate.
4.8 Challenge test
The efficacy of the preservative test was determined following the standards proposed by the European Pharmacopoeia Commission concerning topical preparations, as described (European Pharmacopoeia Commission Efficacy of Antimicrobial Preservation, 2005). The cream formulations (20 g) were placed in sterile containers and separately inoculated with 0.2 mL of each bacterial and fungal suspension in order to obtain a final concentration of approximately 105–106 CFUg−1. The samples were gently shaken to ensure a homogeneous microorganism distribution and incubated at 25°C. Samples of 1 g were removed and placed into 9 mL of peptone solution (0.1%), and serial decimal dilutions were performed before inoculation in the microbial plates.
The same procedure was performed after 2, 7, 14, and 28 days of contact. Cell viability was determined using the plate count method in TSA or SDA, and the CFUs were counted after 2 or 5 days of incubation at 37°C and 25°C for bacteria and fungi, respectively. All determinations were performed in triplicate. The viability and growth ability of the inoculated cells were evaluated by a growth control, which consisted of 20 g cream samples without any preservatives separately inoculated with 0.2 mL of each bacterial and fungal suspension. In order to determine the preservative properties of the topical cream, a reduction of three log phases (3 log) from the initial bacterial count within 1 week and no increase thereafter up to 4 weeks is necessary. For fungi, a 2-log reduction within 2 weeks and no increase thereafter up to 1 month following the initial contamination is required.
4.9 Molecular docking
For the in silico evaluation of different biological activities of the T. broussonetii essential oil, we chose the enzyme NADPH for the antioxidant activity; human casein kinase 1 alpha 1 (CSNK1A1) and glycogen synthase kinase 3 for the healing activity; and S. aureus nucleoside diphosphate kinase, E. coli beta-ketoacyl-[acyl-carrier protein] synthase, Pseudomonas aeruginosa LasR ligand-binding domain, and sterol 14-alpha demethylase (CYP51) from C. albicans for the antimicrobial activity.
The molecular docking process was carried out using the Glide SP module of the Schrodinger Maestro program. This included using the program to execute LigPrep, PrepWizard, grid generation, and docking calculations and identifying key parameters such as the glide score, energy, emodel, and ligand efficiency.
The preparation of the ligands involved downloading the compounds identified in the T. broussonetii EO by GC/MS from PubChem in the SDF format. These ligands were then readied for docking calculations through the use of the LigPrep tool within the Maestro 11.5 version of the Schrödinger software program, utilizing the OPLS3 force field. The maximum number of stereoisomers produced for the ligand was 32, and this was determined by selecting the ionization states at pH 7.0 ± 2.0 (Aboul-Soud et al., 2022a; Slighoua et al., 2022).
To prepare the protein, the three-dimensional crystal structures of NADPH (PDB:2CDU), S. aureus nucleoside diphosphate kinase (PDB: 3Q8U), E. coli beta-ketoacyl-[acyl-carrier protein] synthase (PDB: 1FJ4), Pseudomonas aeruginosa LasR ligand-binding domain (PDB: 2UV0), and sterol 14-alpha demethylase (CYP51) from C. albicans (PDB: 5FSA) were obtained from the protein data bank in the PDB format. They were then built and refined using the Protein Preparation Wizard in Schrödinger Maestro v11.5. Hydrogen was added to the heavy atoms, selenomethionine was converted to methionine, and all water was removed. The protein was then minimized using the OPLS3 force field, with the maximum heavy atom RMSD set to 0.30 Å (Amrati et al., 2022; Aboul-Soud et al., 2022b).
The generation of the receptor grid involved launching the creation module by selecting a ligand atom, resulting in the creation of a default grid box. The ligand was then connected to the grid box generated from the protein using standard precision (SP).
SP flexible ligand docking was performed using the glide module of Schrödinger Maestro v 11.5, where penalties were imposed on non-cis/trans amide bonds. For the ligand atoms, the van der Waals scaling factor and partial charge cutoff were set at 0.80 and 0.15, respectively. The final score was determined using the energy-minimized poses and presented as a glide score. The best-docked pose for each ligand was recorded as the one with the lowest glide score value (Aboul-Soud et al., 2022a).
4.10 ADME/toxicity prediction
The prediction of ADME/toxicity was performed by analyzing the absorption, distribution, metabolism, and excretion parameters using the QikProp function in Maestro 11.5 of Schrödinger software. Various physicochemical and pharmacokinetic factors, such as molecular weight, hydrogen bond acceptor and donor, total solvent surface area, blood–brain partition coefficient, octanol/water partition coefficient, and aqueous solubility, were considered to support the hypothesis. Additionally, the ProTox-II server was utilized to investigate the organic toxicities and toxicological properties, including LD50, of the compounds identified in T. broussonetii EO (Banerjee et al., 2018).
4.11 Statistical analysis
Experiments were carried out in triplicate, and the values are expressed as the mean ± SD. Data were subjected to an analysis of variance (ANOVA). The level of significance was set at p < 0.05.
Data availability statement
The original contributions presented in the study are included in the article/supplementary materials; further inquiries can be directed to the corresponding author.
Author contributions
SB: conceptualization, data curation, formal analysis, investigation, methodology, software, and writing–original draft. FA: investigation, software, and writing–review and editing. MC: investigation and writing–review and editing. AG: formal analysis, resources, and writing–review and editing. RM: formal analysis, methodology, resources, and writing–review and editing. HA-Y: formal analysis, methodology, resources, and writing–review and editing. DB: methodology, supervision, and writing–review and editing.
Funding
The author(s) declare that financial support was received for the research, authorship, and/or publication of this article. The Research was funded by the Researchers Supporting Project program (RSP2024R119), King Saud University, Riyadh, Saudi Arabia.
Conflict of interest
The authors declare that the research was conducted in the absence of any commercial or financial relationships that could be construed as a potential conflict of interest.
Publisher’s note
All claims expressed in this article are solely those of the authors and do not necessarily represent those of their affiliated organizations, or those of the publisher, the editors, and the reviewers. Any product that may be evaluated in this article, or claim that may be made by its manufacturer, is not guaranteed or endorsed by the publisher.
References
Aboul-Soud, M. A., Ennaji, H., Kumar, A., Alfhili, M. A., Bari, A., Ahamed, M., et al. (2022a). Antioxidant, anti-proliferative activity and chemical fingerprinting of centaureacalcitrapa against breast cancer cells and molecular docking of caspase-3. Antioxidants 11 (8), 1514. doi:10.3390/antiox11081514
Aboul-Soud, M. A., Kumar, A., Alfhili, M. A., Bari, A., Ennaji, H., Ahamed, M., et al. (2022b). Apoptosis-mediated antiproliferative activity of Calligonum comosum against human breast cancer cells, and molecular docking analysis of its major polyphenolics to caspase-3. Front. Cell Dev. Biol 2009.
Adams, R. P. (2001) Identification of essential oil components by gas chromatography/mass spectrometry. Carol Stream, IL: Quadrupole. Allured Publ. Corp.
Amrati, F. E. Z., Elmadbouh, O. H. M., Chebaibi, M., Soufi, B., Conte, R., Slighoua, M., et al. (2022). Evaluation of the toxicity of Carallumaeuropaea (CE) extracts and their effects on apoptosis and chemoresistance in pancreatic cancer cells. J. Biomol. Struct. Dyn. 41, 8517–8534. doi:10.1080/07391102.2022.2135595
Ballester-Costa, C., Sendra, E., Fernández-López, J., Pérez-Álvarez, J. A., and Viuda-Martos, M. (2017). Assessment of antioxidant and antibacterial properties on meat homogenates of essential oils obtained from four Thymus species achieved from organic growth. Foods 6, 59. doi:10.3390/foods6080059
Banerjee, P., Eckert, A. O., Schrey, A. K., and Preissner, R. (2018). ProTox-II: a webserver for the prediction of toxicity of chemicals. Nucleic acids Res. 46 (W1), W257–W263. doi:10.1093/nar/gky318
Benabid, A. (2000) Flore et Ecosystèmes du Maroc: Evaluation et Préservation de la Biodiversité. Paris, France: Food and Agriculture Organization of the United Nations.
Bouhdid, S., Abrini, J., Zhiri, A., Espuny, M. J., and Manresa, A. (2009). Investigation of functional and morphological changes in Pseudomonas aeruginosa and Staphylococcus aureus cells induced byOriganum compactum essential oil. J. Appl. Microb. 106, 1558–1568. doi:10.1111/j.1365-2672.2008.04124.x
Bouhdid, S., Skali, S. N., Idaomar, M., Zhiri, A., Baudoux, D., Amensour, M., et al. (2008). Antibacterial and antioxidant activities of origanum compactum essential oil. Afri. J. Biot. 7, 1563–1570.
Burits, M., and Bucar, F. (2000). Antioxidant activity of Nigella sativa essential oil. Phyto. Res. 14, 323–328. doi:10.1002/1099-1573(200008)14:5<323::AID-PTR621>3.0.CO;2-Q
Castilho, P. C., Savluchinske-Feio, S., Weinhold, T. S., and Gouveia, S. C. (2012). Evaluation of the antimicrobial and antioxidant activities of essential oils, extracts and their main components from oregano from Madeira Island, Portugal. Food Control. 23, 552–558. doi:10.1016/j.foodcont.2011.08.031
Chami, N., Bennis, S., Chami, F., Aboussekhra, A., and Remmal, A. (2005). Study of anti-candidal activity of carvacrol and eugenol in vitro and in vivo. Oral Microbiol. Immun 20, 106–111. doi:10.1111/j.1399-302X.2004.00202.x
Clinical and Laboratory Standards Institute (CLSI) (2012) Performance standards for antimicrobial disk susceptibility tests, approved standard. eleventh edition. Wayne, Pennsylvania, USA: CLSI.
El Bouzidi, L., Jamali, C. A., Bekkouche, K., Hassani, L., Wohlmuth, H., Leach, D., et al. (2013). Chemical composition, antioxidant and antimicrobial activities of essential oils obtained from wild and cultivated Moroccan Thymus species. Ind. Crop. Prod. 43, 450–456. doi:10.1016/j.indcrop.2012.07.063
European Pharmacopoeia Commission Efficacy of Antimicrobial Preservation (2005) Group of experts No.1CM (microbial contamination). Strasbourg: Council of Europe.
Fancello, F., Petretto, G. L., Zara, S., Sanna, M. L., Addis, R., Maldini, M., et al. (2016). Chemical characterization, antioxidant capacity and antimicrobial activity against food related microor-ganisms of Citrus limon var. pompia leaf essential oil. LWT-Food Sci. Technol. 69, 579–585. doi:10.1016/j.lwt.2016.02.018
Fayed, S. A. (2009). Antioxidant and anticancer activities of citrus reticulate (petitgrain Mandarin) and Pelargonium graveolens (Geranium) essential oils. Res. J. Agr. Bio. Sci. 5, 740–747.
Fennane, M., and Tattou, M. I. (2008). Statistiques et commentaires sur l’inventaireactuel de la florevasculaire du Maroc. Flora 1986, 1989.
Gutierrez-Larrainzar, M., Rua, J., Caro, I., De Castro, C., de Arriaga, D., Garcia-Armesto, M. R., et al. (2012). Evaluation of antimicrobial and antioxidant activities of natural phenolic compounds against food borne pathogens and spoilage bacteria. Food Control 26, 555–563. doi:10.1016/j.foodcont.2012.02.025
Hamdani, I., Bouyanzer, A., Hammouti, B., Majidi, L., Costa, J., Paolini, J., et al. (2014). Chemical composition and antioxidant activity of essential oils of Thymus broussonetii Boiss. And Thymus algeriensis Boiss. From Morocco. Asian Pac. J. Trop. Dis. 4, 281–286. doi:10.1016/S2222-1808(14)60573-9
Hye, W. K., Yae, S. S., Tae, J. C., and Min, S. R. (2020). Risk factors influencing contamination of customized cosmetics made on-the-spot: evidence from the national pilot project for public health. Sc. Rep. 10, 1561. doi:10.1038/s41598-020-57978-9
Jamali, C. A., El Bouzidi, L., Bekkouche, K., Lahcen, H., Markouk, M., Wohlmuth, H., et al. (2012). Chemical composition and antioxidant and anticandidal activities of essential oils from different wild Moroccan Thymus species. Chem. Biodivers. 9, 1188–1197. doi:10.1002/cbdv.201200041
Johnson, C., Lods, L., Scholz, D., Dres, C., Dashnaw, R. A., Linstra, L., et al. (2000). Maintaining the integrity of cosmetic formulations via the utilization of endogenous signal molecules from plants. Agro. Food Ind. Hi-Tech, 23–25.
Kasujja, I. (2021). Critical evaluation of Melaleuca alternifolia: a review of the phytochemical profile, pharmacological attributes and medicinal properties in the botanical, human and global. Open J. Med. Chem. 11 (1), 1–15. doi:10.4236/ojmc.2021.111001
Kiran Babu, G. D., Shanmugum, V., Ravindranath, S. D., and Joshi, V. P. (2007). Comparison of chemical composition and antifungal activity of curcuma longa L. leaf oils produced by different water different water distillation techniques. Flavour Frag. J. 22, 191–196. doi:10.1002/ffj.1780
Kurdelas, R. R., López, S., Lima Feresin, G. E., Zygadl, J., Zacchino, S., López, M. L., et al. (2012). Chemical composition, anti-insect and antimicrobial activity of Baccharisdarwinii essential oil from Argentina, Patagonia. Ind. Crops Prod. 40, 261–267. doi:10.1016/j.indcrop.2012.03.024
Labiad, M. H., Belmaghraoui, W., Ghanimi, A., El-Guezzane, C., Chahboun, N., Harhar, H., et al. (2022). Biological properties and chemical profiling of essential oils of Thymus (vulgaris, algeriensis and broussonettii) grown in Morocco. Chem. Data Coll. 37, 100797. doi:10.1016/j.cdc.2021.100797
Maggi, F., Cecchini, C., Cresci, A., Coman, M. M., Tirillini, B., Sagratini, G., et al. (2009). Chemical composition and antimicrobial activity of the essential oil from Ferula glauca L. (F. communis L. subsp. glauca) growing in Marche (central Italy). Fitoterapia 80, 68–72. doi:10.1016/j.fitote.2008.10.001
Manou, I., Bouillard, L., Devleeschouwer, M. J., and Barel, A. O. (1998). Evaluation of the preservative properties of Thymus vulgaris essential oil in topically applied formulations under a challenge test. J. Appl. Microbiol. 84, 368–376. doi:10.1046/j.1365-2672.1998.00353.x
Mehdioui, R., and Kahouadji, A. (2007). Etude ethnobotaniqueauprès de la population riveraine de la forêtd’Amsittène: Cas de la Commune d’Imin’Tlit (Province d’Essaouira) Bull. L’institut Sci. Rabat Sect. Sci. La Vie. 29, 11–20.
Miladi, H., Ben Slama, R., Mili, D., Zouari, S., Bakhrouf, A., and Ammar, E. (2013). Essential oil of Thymus vulgaris L. and Rosmarinus officinalis L.: gas chromatography-mass spectrometry analysis, cytotoxicity and antioxidant properties and antibacterial activities against foodborne pathogens. Nat. Sci. 5, 11. doi:10.4236/ns.2013.56090
Miraliakbari, H., and Shahidi, F. (2008). Antioxidant activity of minor components of tree nut oils. Food Chem. 111, 421–427. doi:10.1016/j.foodchem.2008.04.008
Mothana, R. A. A., Hasson, S. S., Schultze, W., Mowitz, A., and Lindequist, U. (2011). Phytochemical composition and in vitro antimicrobial and antioxidant activities of essential oils of three endemic Soqotraen Boswellia species. Food Chem. 126, 1149–1154. doi:10.1016/j.foodchem.2010.11.150
Nazzaro, F., Fratianni, F., De Martino, L., Coppola, R., and De Feo, V. (2013). Effect of essential oils on pathogenic bacteria. Pharmaceuticals 6, 1451–1474. doi:10.3390/ph6121451
Nielsen, P. V., and Rios, R. (2000). Inhibition of fungal growth on bread by volatile components from spices and herbs, and the possible application in active packaging with special emphasis on mustard essential oil. Int. J. Food Microbiol. 60, 219–229. doi:10.1016/S0168-1605(00)00343-3
Nikolić, M., Glamočlija, J., Ferreira, I. C. F. R., Calhelha, R. C., Fernandes, Â., Marković, T., et al. (2014). Chemical composition, antimicrobial, antioxidant and antitumor activity of Thymus serpyllum L., Thymus algeriensis Boiss. and Reut and Thymus vulgaris L. essential oils. Ind. Crops Prod. 52, 183–190. doi:10.1016/j.indcrop.2013.10.006
Nordine, A., Hmamouchi, M., and El Meskaoui, A. (2014). In vitro clonal propagation through direct shoot organogenesis of Thymus broussonetii – a vulnerable aromatic and medicinal plant species. Int. J. Pharm. Res. Bio-Sc. 3, 425–439.
Nordine, A., Tlemcani, C. R., and El Meskaoui, A. (2013). Micropropagation of Thymus satureioidesCoss. an endangered medicinal plant of Morocco. J. Agr. Technol. 9, 421–435.
Nostro, A., Blanco, A. R., Cannatelli, M. A., Enea, V., Flamini, G., Morelli, I., et al. (2004). Susceptibility of methicillin-resistant staphylococci to oregano essential oil, carvacrol and thymol. FEMS Microbiol. Lett. 230, 191–195. doi:10.1016/S0378-1097(03)00890-5
Nouri, H., and Esmaeilian, Y. (2012). Essential oil, phenolic compounds and antioxidant activity of Thymus daenensis Celak. at different harvest times. J. Med. Plants Res. 6 (10), 2051–2055. doi:10.5897/JMPR12.002
Nowak-Lange, M., Niedziałkowska, K., and Lisowska, K. (2022). Cosmetic preservatives: hazardous micropollutants in need of greater attention. Int. J. Mol. Sci. 23 (22), 14495. doi:10.3390/ijms232214495
Ozudogru, E. A., Kaya, E., Kirdok, E., and Issever-Ozturk, S. (2011). In vitro propagation from young and mature explants of thyme (Thymus vulgaris and T. longicaulis) resulting in genetically stable shoots. Vitro Cell. Dev. Biol. Plant. 47, 309–320. doi:10.1007/s11627-011-9347-6
Pinto, E., Pina-Vaz, C., Salgueiro, L., Goncalves, M. J., Costa-de-Oliveira, S., Cavaleiro, A. P., et al. (2006). Antifungal activity of the essential oil of Thymus pulegioideson Candida, Aspergillus and dermatophyte species. J. Med. Microbiol. 55, 1367–1373. doi:10.1099/jmm.0.46443-0
Pirbalouti, A. G., Bistghani, Z. E., and Malekpoor, F. (2015). An overview on genus Thymus. J. Herb. Drugs 6, 93–100.
Radi, F. Z., Bouhrim, M., Mechchate, H., Al-zahrani, M., Qurtam, A. A., Aleissa, A. M., et al. (2021). Phytochemical analysis, antimicrobial and antioxidant properties of Thymus zygis L. And Thymus willdenowii Boiss. Essential oils. Plants 11, 15. doi:10.3390/plants11010015
Randrianarivelo, R., Sarter, S., Odoux, E., Brat, P., Lebrun, M., Romestand, B., et al. (2009). Composition and antimicrobial activity of essential oils ofCinnamosmafragrans. Food Chem. 114, 680–684. doi:10.1016/j.foodchem.2008.10.007
Rankou, H., Culham, A., Jury, S. L., and Christenhusz, M. J. (2013). The endemic flora of Morocco. Phytotaxa 78, 1–69. doi:10.11646/phytotaxa.78.1.1
Saad, A., Fadli, M., Bouaziz, M., Benharref, A., Mezrioui, N. E., and Hassani, L. (2010). Anticandidal activity of the essential oils of Thymus maroccanus and Thymus broussonetii and their synergism with amphotericin B and fluconazole. Phytomed 17, 1057–1060. doi:10.1016/j.phymed.2010.03.020
Saad, N. Y., Muller, C. D., and Lobstein, A. (2013). Major Bioactivities and mechanism of action of essential oils and their components. Flavour Frag. J. 28, 269–279. doi:10.1002/ffj.3165
Sahin, F., Güllüce, M., Daferera, D., Sökmen, A., Sökmen, M., Polissiou, M., et al. (2004). Biological activities of the essential oils and methanol extract of Origanum vulgare ssp. vulgare in the Eastern Anatolia region of Turkey. Food Control 15, 549–557. doi:10.1016/j.foodcont.2003.08.009
Salehi, B., Mishra, A. P., Shukla, I., Sharifi-Rad, M., Contreras, M., Segura-Carretero, A., et al. (2018). Thymol, thyme, and other plant sources: health and potential uses. Phytother. Res. 32, 1688–1706. doi:10.1002/ptr.6109
Schlumbaum, A., Maueh, F., Vogeli, V., and Boller, V. (1986). Plant chitinases are potent inhibitors of fungal growth. Nat 324, 365–367. doi:10.1038/324365a0
Seo, S. B., Ryu, C. S., Ahn, G. W., Kim, H. B., Jo, B. K., Kim, S. H., et al. (2002). Development of natural preservative system using the mixture of chitosan-Inula helenium L. extract. Int. J. Cosmet. Sc. 24, 195–206. doi:10.1046/j.1467-2494.2002.00139.x
Slighoua, M., Chebaibi, M., Mahdi, I., Amrati, F.E.-z., Conte, R., Cordero, M. A. W., et al. (2022). The LC-MS/MS identification and analgesic and wound healing activities of Lavandula officinalis chaix. Vivo Silico Approaches. Plants 11 (23), 3222. doi:10.3390/plants11233222
Verdial, C., Serrano, I., Tavares, L., Gil, S., and Oliveira, M. (2023). Mechanisms of antibiotic and biocide resistance that contribute to Pseudomonas aeruginosa persistence in the hospital environment. Biomed 11 (4), 1221. doi:10.3390/biomedicines11041221
Xiaohua, C., Shufeng, S., Fei, Y., Hai, J., Guanjie, Z., Shan, T., et al. (2023). Antioxidant activities of essential oils and their major components in scavenging free radicals, inhibiting lipid oxidation and reducing cellular oxidative stress. Mol 28 (11), 4559. doi:10.3390/molecules28114559
Xu, J., Zhou, F., Ji, B. P., Pei, R. S., and Xu, N. (2008). The antibacterial mechanism of carvacrol and thymol against Escherichia coli. Lett. Appl. Microbiol. 47, 174–179. doi:10.1111/j.1472-765X.2008.02407.x
Keywords: Thymus broussonetii Boiss. essential oil, antioxidant, antimicrobial, natural preservative, molecular docking, gas chromatography/mass spectrometry
Citation: Boukhira S, Amrati FE-Z, Chebaibi M, Grafov A, Mothana RA, Al-Yousef HM and Bousta D (2024) The chemical composition and the preservative, antimicrobial, and antioxidant effects of Thymus broussonetii Boiss. essential oil: an in vitro and in silico approach. Front. Chem. 12:1402310. doi: 10.3389/fchem.2024.1402310
Received: 17 March 2024; Accepted: 30 April 2024;
Published: 04 July 2024.
Edited by:
Hong Chen, Luoyang Normal University, ChinaReviewed by:
Yajie Guo, Sun Yat-sen University, ChinaAizhamal Baiseitova, Gyeongsang National University, Republic of Korea
Copyright © 2024 Boukhira, Amrati, Chebaibi, Grafov, Mothana, Al-Yousef and Bousta. This is an open-access article distributed under the terms of the Creative Commons Attribution License (CC BY). The use, distribution or reproduction in other forums is permitted, provided the original author(s) and the copyright owner(s) are credited and that the original publication in this journal is cited, in accordance with accepted academic practice. No use, distribution or reproduction is permitted which does not comply with these terms.
*Correspondence: Smahane Boukhira, c21haGFuZS5ib3VraGlyYUB1c21iYS5hYy5tYQ==