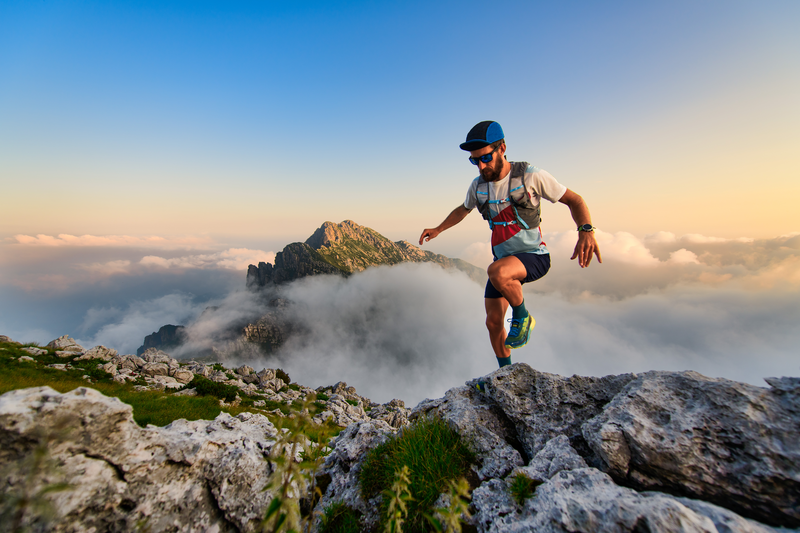
94% of researchers rate our articles as excellent or good
Learn more about the work of our research integrity team to safeguard the quality of each article we publish.
Find out more
EDITORIAL article
Front. Chem. , 05 March 2024
Sec. Theoretical and Computational Chemistry
Volume 12 - 2024 | https://doi.org/10.3389/fchem.2024.1384385
This article is part of the Research Topic Computational and Experimental Insights in Proton and Ion Translocating Bioenergetic Systems View all 5 articles
Editorial on the Research Topic
Computational and experimental insights in proton and ion translocating bioenergetic systems
As a follow up of the Research Topic–“Computational and Experimental Insights in Redox-Coupled Proton Pumping in Proteins”, this Research Topic showcases how different experimental and computational approaches can be exploited to understand the molecular mechanisms of bioenergetic systems (Wikström et al., 2023a).
Proteins performing redox-coupled proton pumping are key players in energy transduction in all living organisms. They are mostly observed in diverse respiratory chains (Marreiros et al., 2016; Calisto and Pereira, 2021). One of the most studied redox-coupled proton pumping proteins are oxygen reductases, the last enzymes of aerobic respiratory chains. These are found in all three domains of life, eukaryotes, prokaryotes and archaea and belong to the heme-copper oxidase superfamily or to the cytochrome bd-type family. The heme-copper oxidase superfamily has been divided primarily into three sub-types, A, B and C-type oxidases (Pereira et al., 2001). Mårten Wikström, in 1977, showed for the first time that mammalian cytochrome c oxidase, which belongs to type A, is a redox-driven proton pump (Wikström, 1977). We have come a long way since then and several aspects of its catalytic cycle are now well-understood (see Figure 1; Wikström et al. (2023b)).
FIGURE 1. Catalytic cycle of A-type cytochrome c oxidase (Wikström et al., 2023a). Figure used with permission from the publisher.
In this Research Topic, Shimada et al. discuss the recent experimental findings which have significantly improved our understanding of the reaction mechanism of the mitochondrial enzyme. This is a contribution from Yoshikawa’s group that provided us with the breakthrough of the determination of the first structure of A-type mitochondrial cytochrome c oxidase (Tsukihara et al., 1995). There is convergence on several aspects of the catalytic cycle of oxidase, as discussed elegantly by Shimada et al. However, some central questions remain open–such as the role of the H-pathway of proton transfer in the proton pumping mechanism of A-type cytochrome c oxidase. Site directed mutagenesis studies, proton pumping experiments and computer simulation studies (Malkamäki et al., 2019; Maréchal et al., 2020; Reidelbach et al., 2021) suggest that H-pathway is not the proton pumping pathway in the yeast mitochondrial oxidase. Also, notably the exit route for pumped protons and the structure of the active site in resting oxidized state of the enzyme remains unclear. But new structural insights based on serial femtosecond X-ray crystallography studies by Ishigami et al. (2023) suggest that the conserved cross-linked tyrosine is neutral in the resting O state of the enzyme, in agreement with the proposals by Blomberg (Blomberg, 2021), whereas the origin of this proton maybe internal (Sharma and Wikström, 2016).
To obtain detailed molecular insights into enzyme mechanisms, computational approaches are often applied in combination with high resolution structural data (see Lee et al., 2022; Bergh et al., 2024). On one hand, physics based classical molecular dynamics (MD) simulations can help in providing high spatial and temporal resolution of biological processes, chemical reactions, on the other hand, can be studied with QM (Quantum Mechanics)-based computational approaches. Noodleman et al. discuss the dioxygen reduction reaction and proton pumping mechanism of B-type oxidases from Thermus thermophilus. They showcase the strength of computational approaches such as classical MD simulations and QM-based cluster calculations in understanding the mechanism of B-type oxygen reductase. The putative proton loading site, proton transfer pathways, exit route for pumped protons and active site intermediates of B-type oxidases, which share similarities and differences with the relatively well-understood A-type oxidases (Wikström and Sharma, 2019), are discussed.
Proton transfer reactions, which are central to redox-coupled proton pumping proteins have also been studied with hybrid QM/MM methods, combined with free energy analysis when required (see Mandal et al., 2023; Zdorevskyi et al., 2023). Protons can transfer rapidly along a hydrogen bonded pathway involving amino acid residues and water molecules. However, hydrogen bonds can be short-lived and undergo rearrangements during the catalytic cycle of a protein. This is the essence of the work by Bertalan and Bondar, who discuss graph-based approaches to study hydrogen bond networks in proteins with special focus on rhodopsins. Structural snapshots obtained from serial femtosecond crystallography were subjected to graph computations and hydrogen bond restructuring was resolved, giving novel insights into protein function. This work highlights integration of graph-based computational approaches to structural biology experiments with a possibility of extension also to MD simulation trajectories.
Cytochrome bd-type enzymes have found increasing interest in the last years. These enzymes are electrogenic and catalyze the reduction of molecular oxygen to water by recruiting protons from the cytoplasmic side of the membrane. However, they do not actively pump protons across, and in this way can be considered less efficient than heme-copper oxidases, which generate proton motive force both by pumping protons and recruiting substrate protons and electrons from the opposite sides of the membrane. Importantly, cytochrome bd-type oxidases are discussed to be defence factors in bacteria and are important antimicrobial drug targets (Friedrich et al., 2022). They turned out to show a high degree of diversity as shown by structural (Safarian et al., 2016; Safarian et al., 2019; Theßeling et al., 2019), electrochemical (Nikolaev et al., 2021) and also phylogenetic studies (Murali et al., 2021). The high diversity of these enzymes is confirmed with a new cryo-EM structure of cytochrome bd from Corynebacterium glutamicum (Grund et al.) that shares structural characteristics with the Mycobacterium tuberculosis bd-type enzyme.
VS: Writing–original draft, Writing–review and editing. PH: Writing–original draft, Writing–review and editing. MP: Writing–original draft, Writing–review and editing.
The authors declare that the research was conducted in the absence of any commercial or financial relationships that could be construed as a potential conflict of interest.
All claims expressed in this article are solely those of the authors and do not necessarily represent those of their affiliated organizations, or those of the publisher, the editors and the reviewers. Any product that may be evaluated in this article, or claim that may be made by its manufacturer, is not guaranteed or endorsed by the publisher.
Bergh, C., Rovšnik, U., Howard, R., and Lindahl, E. (2024). Discovery of lipid binding sites in a ligand-gated ion channel by integrating simulations and cryo-EM. Elife 12, RP86016. doi:10.7554/eLife.86016
Blomberg, M. R. (2021). The redox-active tyrosine is essential for proton pumping in cytochrome c oxidase. Front. Chem. 9, 640155. doi:10.3389/fchem.2021.640155
Calisto, F., and Pereira, M. M. (2021). The Ion-Translocating nrfD-like subunit of energy-transducing membrane complexes. Front. Chem. 9, 663706. doi:10.3389/fchem.2021.663706
Friedrich, T., Wohlwend, D., and Borisov, V. B. (2022). Recent advances in structural studies of cytochrome bd and its potential application as a drug target. Int. J. Mol. Sci. 23 (6), 3166. doi:10.3390/ijms23063166
Ishigami, I., Sierra, R. G., Su, Z., Peck, A., Wang, C., Poitevin, F., et al. (2023). Structural insights into functional properties of the oxidized form of cytochrome c oxidase. Nat. Commun. 14 (1), 5752. doi:10.1038/s41467-023-41533-x
Lee, Y., Haapanen, O., Altmeyer, A., Kühlbrandt, W., Sharma, V., and Zickermann, V. (2022). Ion transfer mechanisms in Mrp-type antiporters from high resolution cryoEM and molecular dynamics simulations. Nat. Commun. 13 (1), 6091. doi:10.1038/s41467-022-33640-y
Malkamäki, A., Meunier, B., Reidelbach, M., Rich, P. R., and Sharma, V. (2019). The H channel is not a proton transfer path in yeast cytochrome c oxidase. Biochimica Biophysica Acta (BBA)-Bioenergetics 1860 (9), 717–723. doi:10.1016/j.bbabio.2019.07.012
Mandal, M., Saito, K., and Ishikita, H. (2023). Substitution of Ca 2+ and changes in the H-bond network near the oxygen-evolving complex of photosystem II. Phys. Chem. Chem. Phys. 25 (8), 6473–6480. doi:10.1039/d2cp05036f
Maréchal, A., Xu, J. Y., Genko, N., Hartley, A. M., Haraux, F., Meunier, B., et al. (2020). A common coupling mechanism for A-type heme-copper oxidases from bacteria to mitochondria. Proc. Natl. Acad. Sci. 117 (17), 9349–9355. doi:10.1073/pnas.2001572117
Marreiros, B. C., Calisto, F., Castro, P. J., Duarte, A. M., Sena, F. V., Silva, A. F., et al. (2016). Exploring membrane respiratory chains. Biochimica Biophysica Acta (BBA)-Bioenergetics 1857 (8), 1039–1067. doi:10.1016/j.bbabio.2016.03.028
Murali, R., Gennis, R. B., and Hemp, J. (2021). Evolution of the cytochrome bd oxygen reductase superfamily and the function of CydAA’in Archaea. ISME J. 15 (12), 3534–3548. doi:10.1038/s41396-021-01019-4
Nikolaev, A., Safarian, S., Thesseling, A., Wohlwend, D., Friedrich, T., Michel, H., et al. (2021). Electrocatalytic evidence of the diversity of the oxygen reaction in the bacterial bd oxidase from different organisms. Biochimica Biophysica Acta (BBA)-Bioenergetics 1862 (8), 148436. doi:10.1016/j.bbabio.2021.148436
Pereira, M. M., Santana, M., and Teixeira, M. (2001). A novel scenario for the evolution of haem–copper oxygen reductases. Biochimica Biophysica Acta (BBA)-Bioenergetics 1505 (2-3), 185–208. doi:10.1016/s0005-2728(01)00169-4
Reidelbach, M., Zimmer, C., Meunier, B., Rich, P. R., and Sharma, V. (2021). Electron transfer coupled to conformational dynamics in cell respiration. Front. Mol. Biosci. 8, 711436. doi:10.3389/fmolb.2021.711436
Safarian, S., Hahn, A., Mills, D. J., Radloff, M., Eisinger, M. L., Nikolaev, A., et al. (2019). Active site rearrangement and structural divergence in prokaryotic respiratory oxidases. Science 366 (6461), 100–104. doi:10.1126/science.aay0967
Safarian, S., Rajendran, C., Müller, H., Preu, J., Langer, J. D., Ovchinnikov, S., et al. (2016). Structure of a bd oxidase indicates similar mechanisms for membrane-integrated oxygen reductases. Science 352 (6285), 583–586. doi:10.1126/science.aaf2477
Sharma, V., and Wikström, M. (2016). The role of the K-channel and the active-site tyrosine in the catalytic mechanism of cytochrome c oxidase. Biochimica Biophysica Acta (BBA)-Bioenergetics 1857 (8), e2–e1115. doi:10.1016/j.bbabio.2016.04.014
Theßeling, A., Rasmussen, T., Burschel, S., Wohlwend, D., Kägi, J., Müller, R., et al. (2019). Homologous bd oxidases share the same architecture but differ in mechanism. Nat. Commun. 10 (1), 5138. doi:10.1038/s41467-019-13122-4
Tsukihara, T., Aoyama, H., Yamashita, E., Tomizaki, T., Yamaguchi, H., Shinzawa-Itoh, K., et al. (1995). Structures of metal sites of oxidized bovine heart cytochrome c oxidase at 2.8 Å. Science 269 (5227), 1069–1074. doi:10.1126/science.7652554
Wikström, M., Gennis, R. B., and Rich, P. R. (2023b). Structures of the intermediates in the catalytic cycle of mitochondrial cytochrome c oxidase. Biochimica Biophysica Acta (BBA)-Bioenergetics 1864 (2), 148933. doi:10.1016/j.bbabio.2022.148933
Wikström, M., Pecorilla, C., and Sharma, V. (2023a). The mitochondrial respiratory chain. Enzymes 54, 15–36. doi:10.1016/bs.enz.2023.05.001
Wikström, M., and Sharma, V. (2019). “Cytochrome c oxidase—remaining questions about the catalytic mechanism,” in Oxygen production and reduction in artificial and natural systems. Editors J. Barber, A. V. Ruban, and P. J. Nixon (World Scientific), 135–145.
Wikström, M. K. (1977). Proton pump coupled to cytochrome c oxidase in mitochondria. Nature 266 (5599), 271–273. doi:10.1038/266271a0
Keywords: bioenergetics, mitochondrial dysfunction, electron transport chain, computer simulations, biophysics
Citation: Sharma V, Hellwig P and Pereira M (2024) Editorial: Computational and experimental insights in proton and ion translocating bioenergetic systems. Front. Chem. 12:1384385. doi: 10.3389/fchem.2024.1384385
Received: 09 February 2024; Accepted: 28 February 2024;
Published: 05 March 2024.
Edited and reviewed by:
Sam P. De Visser, The University of Manchester, United KingdomCopyright © 2024 Sharma, Hellwig and Pereira. This is an open-access article distributed under the terms of the Creative Commons Attribution License (CC BY). The use, distribution or reproduction in other forums is permitted, provided the original author(s) and the copyright owner(s) are credited and that the original publication in this journal is cited, in accordance with accepted academic practice. No use, distribution or reproduction is permitted which does not comply with these terms.
*Correspondence: Vivek Sharma, dml2ZWsuc2hhcm1hQGhlbHNpbmtpLmZp; Petra Hellwig, aGVsbHdpZ0B1bmlzdHJhLmZy; Manuela Pereira, bW1wZXJlaXJhQGNpZW5jaWFzLnVsaXNib2EucHQ=
Disclaimer: All claims expressed in this article are solely those of the authors and do not necessarily represent those of their affiliated organizations, or those of the publisher, the editors and the reviewers. Any product that may be evaluated in this article or claim that may be made by its manufacturer is not guaranteed or endorsed by the publisher.
Research integrity at Frontiers
Learn more about the work of our research integrity team to safeguard the quality of each article we publish.