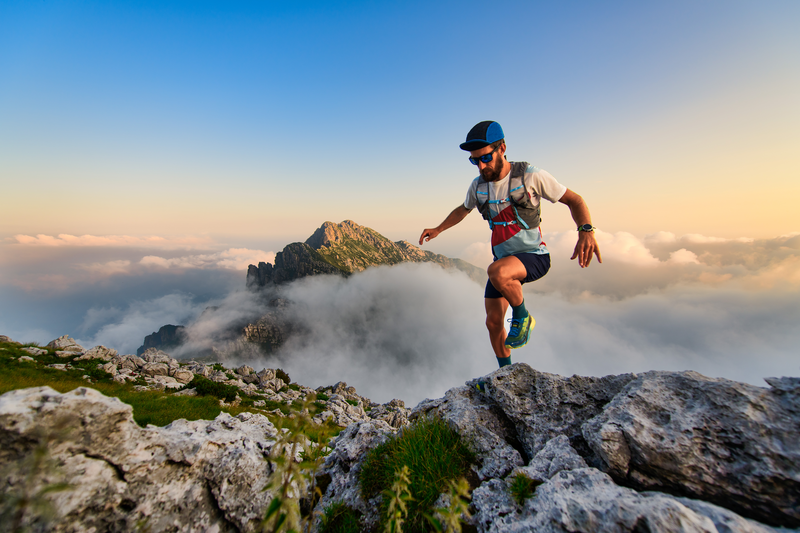
94% of researchers rate our articles as excellent or good
Learn more about the work of our research integrity team to safeguard the quality of each article we publish.
Find out more
ORIGINAL RESEARCH article
Front. Chem. , 29 February 2024
Sec. Organic Chemistry
Volume 12 - 2024 | https://doi.org/10.3389/fchem.2024.1376948
This article is part of the Research Topic Women in Chemistry 2023 View all 11 articles
The transition-metal free S-vinylation of thiophenols by vinylbenziodoxolones (VBX) constituted an important step forward in hypervalent iodine-mediated vinylations, highlighting the difference to vinyliodonium salts and that the reaction outcome was influenced by the substitution pattern of the benziodoxolone core. In this study, we report several new classes of hypervalent iodine vinylation reagents; vinylbenziodazolones, vinylbenziodoxolonimine and vinyliodoxathiole dioxides. Their synthesis, structural and electronic properties are described and correlated to the S-vinylation outcome, shedding light on some interesting facets of these reagents.
Hypervalent iodine reagents have been shown to be powerful reagents for chemoselective transformations under both transition metal-catalyzed and metal-free conditions. (Wirth, 2016; Yoshimura and Zhdankin, 2016; Flores et al., 2019; Olofsson et al., 2019). The use of iodonium salts has enabled transfer of aryl, alkynyl and vinyl groups to a variety of nucleophiles. (Merritt and Olofsson, 2009; Charpentier et al., 2015; Li et al., 2016; Rajkiewicz and Kalek, 2018; Villo et al., 2019; Declas et al., 2020; Dahiya et al., 2022; Le Du and Waser, 2023; Mironova et al., 2023; Yoshimura et al., 2023). New classes of alkenes have been accessed through the combination of vinyliodonium salts with metal catalysts, (Skucas and MacMillan, 2012; Holt and Gaunt, 2015; Sheng et al., 2017; Yuan et al., 2019), whereas metal-free applications with those reagents remain scarce due to difficulties in controlling the reaction outcome. (Ochiai et al., 2001; Hara et al., 2006; Kepski et al., 2019). Recent developments in the field have shown that benziodoxolones (BX), which are iodine (III) compounds with a cyclic core, possess improved stability and often have more easily controlled reactivity. (Yoshimura et al., 2023). Indeed, the utility of trifluoromethyl-BX (Togni’s reagent) and ethynyl-BX (EBX) have been efficiently demonstrated in the last decades. (Charpentier et al., 2015; Hari et al., 2019; Le Du and Waser, 2023).
In 2016, our group reported the synthesis and first applications of vinyl-BX (VBX, 1) (Stridfeldt et al., 2016), which showed enhanced reactivity and selectivity compared to vinyliodonium salts. (Figure 1A), (Stridfeldt et al., 2016; Declas et al., 2020). Transition metal-free applications include S- and P-vinylation methodologies, (Castoldi et al., 2020a; Castoldi et al., 2020b; Di Tommaso et al., 2022), as well as photocatalytic C-vinylations with redox active compounds (Pal et al., 2023) and others. (Davies et al., 2017; Le Vaillant et al., 2018; Liu et al., 2018; Jiang and Studer, 2019; Li et al., 2019; Liu et al., 2020). Our one-pot synthesis of VBX is shown in Figure 1B i, and the scope was later expanded to include β-heteroatom-functionalized VBX through addition of a nucleophile and a proton over EBX (Figure 1B ii). (Frei et al., 2014; Caramenti et al., 2019; Shimbo et al., 2019; Tessier et al., 2019; Wu et al., 2019; Liu et al., 2020; Declas et al., 2022) In parallel, the corresponding vinylbenziodoxoles with a bis(CF3)alkoxy moiety (VBO) were introduced by Yoshikai and coworkers, and have proved superior in some applications. (Wu et al., 2016; Wu J. et al., 2017; Shimbo et al., 2019; Wu et al., 2019; Chai et al., 2021; Wang et al., 2022). VBO can be synthesized from TfO-BO and mono- or di-substituted alkynes (Figure 1B iii). (Wu B. et al., 2017; Ding et al., 2020; Ura et al., 2020; Chai et al., 2021; Declas et al., 2022; Kikuchi et al., 2022; Wang et al., 2022) Recently, Waser presented a one-pot synthesis of ethynyl bis(trifluoromethyl)iodoxole (EBO) directly from the corresponding iodoarene. (Milzarek et al., 2023). This method also included the synthesis of a VBO reagent, simplifying the access to such targets (Figure 1B iv).
FIGURE 1. (A) The use of iodonium salts compared to VBX; (B) Selected synthetic routes to VBX and VBO; (C) Workflow of this investigation.
The reactivity of hypervalent alkynylating reagents with substituted aromatic ring cores, as well as variations of the “side-arm”, which binds to the iodine centre, have been explored. (Fernández González et al., 2013; Shimbo et al., 2019). While we evaluated the influence of core-substituents on VBX in the S-vinylation of thiols, (Castoldi et al., 2020b), there are no broad studies investigating the structural and electronic effects of varying these groups on vinylating reagents. (Mironova et al., 2023). Herein, we report the synthesis of several new VBO 2, as well as the synthesis of novel compound classes vinylbenziodazolones (VBZ, 3), vinylbenziodoxolonimine (VBXI, 4), and vinyliodoxathiole dioxides (VBT, 5) (Figure 1C). It should be noted that the benziodazolone (Le Du et al., 2021) and benziodoxathiole (Koser et al., 1993; Koposov et al., 2006) cores have been reported in other hypervalent iodine reagents, whereas the benziodoxolonimine is a novel side-arm. Additionally, we have synthesised several novel ortho-functionalized iodonium salts 6, which serve as good comparisons in the studies. Finally, a vinylbenziodoxolone-type reagent with a six-membered side-arm (VBX6 7) was synthesized to evaluate the effect of the side-arm length and conjugation with the core. We have determined their crystal structures, as well as their reduction potentials, and correlated these parameters with the reagents’ reactivity under the reported S-vinylation conditions.
Several core-substituted VBX reagents 1 were synthesised according to literature methods (Stridfeldt et al., 2016; Boelke et al., 2017), Scheme 1A). The synthesis of novel VBO reagents 2 started from anilines 8, which underwent a Friedel-Crafts reaction to access the amino benzyl alcohols 9 (Scheme 1B). A subsequent Sandmeyer reaction produced the required iodoarenes 10 in good yields. (Amey and Martin, 1979). The benziodoxole core was formed through oxidative chlorination and hydrolysis, without isolation in between the steps, to form hydroxy-BO 11. The vinyl moiety was introduced from the corresponding boronic acid using TMSOTf and pyridine (Boelke et al., 2017) to form VBO 2 (yields from 10 given in Scheme 1B). It should be noted that ortho-substituted compound 2f was incredibly unstable, making isolation and analysis difficult (See the Supplementary Material for details).
SCHEME 1. Synthesis of hypervalent vinylation reagents. (A) VBX reagents 1; (B) VBO reagents 2 (2a already reported), yields from 10 given; (C) VBZ reagents 3, VBXI reagent 4 and VBT reagents 5; (D) Acyclic iodonium salts 6 and VBX6 7.
Variations of the side-arm were next investigated to obtain novel compound classes for vinylation. The synthesis of VBZ 3 proceeded in good yields from 2-iodophenyl N-tosylbenzamides 12a-c, using our one-pot method developed for VBX (Stridfeldt et al., 2016) with mCPBA/triflic acid and (E)-styrylboronic acid (Scheme 1C). To our surprise, reactions with the ortho-methyl-substituted substrate 12d behaved differently, and resulted in the formation of the novel compound class VBXI 4, which has an I-O hypervalent bond instead of the expected I-N bond. It appears that the sterical congestion caused by the ortho-methyl group promotes formation of the BXI core as opposed to the BZ core. Products 5 were obtained from 2-iodophenyl sulfonic acids 12d-e in moderate yields due to incomplete conversion of the starting material.
For the sake of comparison to their cyclic counterparts, a series of vinyliodonium salts 6 with ortho functionalities were also synthesised from the iodoarenes 13 (Scheme 1D). Our one-step method (Stridfeldt et al., 2016) without the basic workup was used to obtain these compounds in good to high yields. Interestingly, when the one-pot synthesis of VBX6 7 was attempted, product formation alongside an inseparable impurity was observed. (See the Supplementary Material for details) However, when isolated compound 6e was treated with an aqueous basic solution, 7 could be isolated in good yields with high purity. This strategy was also attempted for the synthesis of VBX7 from 6d, but was unsuccessful (See the Supplementary Material for details).
The S-vinylation protocol developed by our group was used to evaluate the vinylating reagents, as this reaction had already proved sensitive to the VBX core structure. (Castoldi et al., 2020b). 4-Bromothiophenol was thus vinylated with reagents 1-7 to provide thioether 14 with vinyl iodide 15 sometimes formed as side-product (Scheme 2). The result obtained with the novel VBX reagent 1f followed the trend in the original work, (Castoldi et al., 2020b), in which reagents with electron-donating groups (EDG) gave higher yields than those with electron-withdrawing groups (EWG). VBO reagents 2 performed markedly worse, with yields of 14 ranging from 25% to 51%. VBZ 3 behaved similarly to VBX, with 3c providing the highest yield of 90%, whereas VBXI 4 and VBT 5 gave significantly lower yields, which could be due to solubility problems. E/Z ratios were recorded in of each of these reactions, but there was no observed trend with regards to the reagent used (See the Supplementary Material for details).
SCHEME 2. Comparison of vinylating reagents 1-7 in the S-vinylation of 4-bromothiophenol. Yields are NMR yields, which were calculated by using 1,3,5 trimethoxybenzene as internal standard.
Additionally, the compounds with ortho-substituents performed worse than their counterparts (1a vs 1g, 2a vs 2f). Due to the rapid decomposition of those reagents during isolation and analysis, we hypothesise that the ortho-substituents increase the reactivity of these compounds, especially since the majority of product in these reactions were the vinyl iodide 15. Indeed, ortho-substitution in hypervalent iodine compounds has earlier been reported to cause considerable reactivity changes. (Guilbault and Legault, 2012; Malmgren et al., 2013; Abazid and Nachtsheim, 2020). Additionally, VBXI 4 produced a very poor yield of 14 in comparison to its analogues VBZ 3, possibly due to its BXI core. Similar to what was reported with acyclic vinyliodonium salts in the original S-vinylation paper, (Castoldi et al., 2020b), reagents 6 provided little product, with 15 again being the major product in these reactions. VBX6 provided a much lower yield than VBX, indicating the importance of the 5-membered ring for the application of this reagent.
To evaluate how the core substituents and side-arms influenced the structure, we collected single crystal X-ray diffraction data on selected compounds (Figure 2). Much of the crystal structure data in the literature focusses on vinyliodonium salts, (Hinkle and McDonald, 2002; Ochiai et al., 2007; Yoshimura et al., 2021), and varied substitution patterns on the vinyl groups of VBO (Wu et al., 2016; Wu J. et al., 2017; Wu et al., 2019; Ding et al., 2020; Pisella et al., 2020; Chai et al., 2021; Laskar et al., 2021; Wu et al., 2023) and VBX. (Stridfeldt et al., 2016; Caramenti et al., 2019; Tessier et al., 2019). On the other hand, there appears to be no crystal structure investigations on the effect of cyclic vinylation reagents with different side-arms and core-substituents, as well as non-covalent interactions in ortho-substituted iodonium salts, on reaction outcome.
FIGURE 2. Novel crystal structures of reagents 1-7 compared to structures in the literature (1a (Stridfeldt et al., 2016), 2a (Pisella et al., 2020) and 6f (Clegg and Harrington, 1999)).
We evaluated the effect of the side-arm by comparison of core-unsubstituted VBX 1a, VBO 2a, VBZ 3a and VBT 5a, which showed very similar C1-I bond lengths, 2.127, 2.118, 2.127 and 2.112 Å respectively. The same is also true for C3-I bond lengths, as they measured at 2.100, 2.103, 2.140 and 2.128 Å respectively. However, X-I bond lengths were drastically different. 1a and 3a were somewhat similar with bond lengths of 2.510 and 2.581 Å respectively, whilst 2a had a shorter bond length (2.346 Å) and 5a has a longer bond length (2.649 Å). This is perhaps indicative of the increased trans effect caused by this functional group. (Ochiai et al., 2006). Additionally, measured X-I-C3 bond angles showed that all compounds expressed a T-shaped conformation with 1a having the smallest angle, 165.9°, and VBO 2b the largest, 170.9°. The X-ray crystal structure of VBX6 7 showed a strained 6-membered ring in the side-arm, with similar bond lengths and hypervalent bond angle with VBX 1a. However, 7 has a C2-C1-I-C3 bond angle of 36.4°, which is far higher than that of 1a (4.3°).
To ascertain whether core-substituents made a measurable difference on any structural properties, several analogues of each class of vinylating reagent were also crystallised. Generally, the same trends followed within each class of compounds. Interestingly, the crystallographic data of ortho-substituted VBXI 4 showed a markedly lower X-I-C3 bond angle of 155.7°, which is the lowest angle of any hypervalent iodine vinylating reagent in the literature. Additionally, 4 has a C2-C1-I-C3 bond angle of 38.5°, which is far from the more idealised angle of 1°–8° for the other compounds. These two measurements show that the ortho-methyl substituent induces sufficient steric strain to disrupt the hypervalent bond and ultimately leads to the formation of an I-O bond, as opposed to the I-N bond found in VBZ 3. We hypothesise that this key difference contributes to the reagent’s poor reactivity under the S-vinylation conditions. Furthermore, this characteristic likely contributes to their unstable and over-reactive nature as seen in Scheme 2. Interestingly, iodonium salt 6a had similar C1-I (2.123 Å) and C3-I (2.073 Å) bond lengths to other compounds. Though, it has a much longer X-I bond length (2.673 Å), which is unsurprising considering the methyl ester ligand is not covalently bound, but it is markedly smaller than the X-I bond length of 6f (The crystal structure data of 6f is included as comparison to 6a; we did not use 6f in other parts of the study) (2.879 Å). This shows that whilst not having a covalently bound group will affect the structural properties, a much more significant effect will be observed when non-ligating substituents are used in the ortho position to the iodine.
Next, the possible correlation between structural parameters and reaction outcome was investigated (Figure 3). Firstly, the X-I bond lengths were plotted against the yield of S-vinylation, showing an upward slope from 2.341 Å (2e) to 2.581 Å (3a), which was proceeded by a downward slope towards 2.673 Å of 6a (ester-bound iodonium) (Figure 3A). Interestingly, 4 and 7 were outliers to this trend (circled, hollow diamond). Thus, reagents with X-I bond lengths of ∼2.55 Å represent a “sweet-spot” under these reaction conditions. Then the hypervalent bond angles (X-I-C3) were plotted against reaction yields (Figure 3B). Within this parameter, it was observed that the higher the angle, and thus closer to the idealised 180°, the worse the reagent performed, with a peak of ∼165°. Again, reagent 4 was an outlier in this trend. These results show that there is indeed a link between these two structural parameters and the reaction yield, and that both electronic and steric factors influence the reaction outcome.
FIGURE 3. Selected structural parameters vs reaction outcome. Circled points are outliers. (A) X-I bond lengths vs reaction outcome (B) X-I-C3 bond angles vs reaction yield.
We also wanted to investigate how the reduction potentials of the reagents were affected by substituents of the aromatic ring core and the side-arm (Figure 4). Whilst there have been some reports of redox potentials of hypervalent iodine reagents in the literature, (Kokkinidis et al., 1989; Kokkinidis et al., 1991; Choi et al., 2015; Le Vaillant and Waser, 2017; De et al., 2019; Ramkumar et al., 2023), and even a computational study, (Radzhabov et al., 2020), there is currently no data on vinylation reagents and certainly no quantitative studies linking this parameter to reaction outcome.
To begin, we measured the potentials of each of the reagent used in this study. The higher the reduction potential (closer the value to 0 V (vs Fc/Fc+)), the more easily the reagent can be reduced. It was found that there was a tight range of reduction potentials within each class of reagent. VBX ranged from −1.33 V (1f) to −1.59 V (1c), which matches that EWG should make the reagent easier to reduce. Considering its strong likeness to 1a, VBX6 7 had a very different potential of −1.61 V. Whilst VBZ 3a-c had slightly higher potentials between −1.32 and −1.48 V, VBXI 4 (−1.21 V) and VBT 5 (−1.21 and −1.17 V) had lower potentials. The lowest reduction potentials were measured for VBO 2, ranging from 2c (−1.98 V) to 2f (−1.68 V). As controls, the potentials of some vinyliodonium salts 6 were measured. Direct comparison of the uncyclized and cyclised analogues (6c vs 1e and 6e vs 6) showed that the iodonium salts were indeed much easier to reduce. This was, however, not the case for 6b (−1.16 V), which was very similar to its cyclised VBT counterpart 5b (−1.17 V).
Next, to ascertain whether there is a relationship between reduction potentials of the vinylation reagents and their yield in S-vinylation, the two were plotted against each of other (Figure 5). Firstly, we plotted the VBO, VBZ and only the EDG-substituted VBX reagents, as EWG-substituted VBX performed poorly in S-vinylation (Figure 5A). Reagents with lower reduction potentials were found to have a positive effect on the reaction yield. Secondly, we plotted the EWG-substituted VBX reagents, VBT reagents and iodonium salts (Figure 5B). In this case, lower reduction potential resulted in lowered reaction yield together with increased levels of vinyl iodide (see data in Scheme 2). This is interesting because the less reactive reagents (e.g., VBO) provided lower reaction yields, whereas the more reactive reagents (e.g., iodonium salts) gave low yields and more vinyl iodide. Furthermore, the results suggest that a reagent with a reduction potential between −1.3 and −1.5 V, represents the peak of this reaction, with potentials on either side being ultimately detrimental for the reagents. However, this could be due to the reaction itself being optimised on VBX 1a. Curiously however, if both plots are overlayed with the previously excluded reagents, it becomes clear that there are some outliers to this trend (green triangles, Figure 5C). These are VBX6 7 and ortho-substituted VBO 2f and VBXI 4, and the results might reflect the lack of idealised T-shaped conformation or conjugation in those structures. Clearly the reduction potential does not account for the change in T-shaped conformation and steric factors very well. Overall, it appears that the reduction potentials can be a good signifier for the efficiency of the vinylation reagent in the S-vinylation under these reaction conditions, but further reaction optimisation could potentially alter the outcome. Finally, a fine correlation was observed between X-I bond lengths and reduction potentials (Figure 5D), signifying that X-I bond lengths could affect the reduction potentials greatly.
FIGURE 5. Reduction potentials of selected reagents plotted against S-vinylation. (A) Plot of 1a–1d, 2a–2e and 3. (B) Plot of 1e–1g, 5 and 6. (C) Overlay of plots (A, B), including the outlier compounds 2f, 4 and 7. (D) Plot of X-I bond lengths vs reduction potentials.
For general experimental and instrumental methods, synthetic procedures, and full compound characterization, see the Supplementary Material.
In conclusion, the synthesis of novel hypervalent iodine-based vinylation reagents has been reported, including the new compound classes VBZ, VBXI, VBT and VBX6. These reagents were evaluated in the S-vinylation of 4-bromothiophenol and VBZ performed similarly to VBX, whilst VBO, VBXI, VBT and iodonium salts proved inferior. Crystal structures of selected reagents were measured, as well as electronic potentials of all the reagents. Crystal structure data showed that there was a correlation between certain parameters and reaction outcome, and ortho-substituents were found to perturb the reagent’s structure and hence destabilise it. Additionally, reduction potentials were plotted against reaction outcome, which showed a sweet spot of about −1.4 V, when ignoring certain outliers in the study. Additionally, there was a correlation between reduction potentials and X-I bond length. We believe that further investigations of properties vs reaction outcome could result in a method for predicting reaction outcome with hypervalent iodine reagents.
The original contributions presented in the study are included in the article/Supplementary Material, further inquiries can be directed to the corresponding author.
SD: Conceptualization, Validation, Writing–original draft, Writing–review and editing, Investigation, Methodology, Visualization. ED: Conceptualization, Investigation, Methodology, Validation, Supervision, Writing–review and editing. AP: Investigation, Writing–review and editing. AI: Investigation, Writing–review and editing, Validation, Visualization. BO: Conceptualization, Funding acquisition, Resources, Supervision, Validation, Writing–original draft, Writing–review and editing, Project administration, Visualization.
The author(s) declare financial support was received for the research, authorship, and/or publication of this article. Carl Trygger Foundation (20:316), the Swedish Research Council (2019-04232) and the Swedish Foundation for Strategic Research (SSF) are kindly acknowledged for financial support.
The authors declare that the research was conducted in the absence of any commercial or financial relationships that could be construed as a potential conflict of interest.
All claims expressed in this article are solely those of the authors and do not necessarily represent those of their affiliated organizations, or those of the publisher, the editors and the reviewers. Any product that may be evaluated in this article, or claim that may be made by its manufacturer, is not guaranteed or endorsed by the publisher.
The Supplementary Material for this article can be found online at: https://www.frontiersin.org/articles/10.3389/fchem.2024.1376948/full#supplementary-material
Abazid, A. H., and Nachtsheim, B. J. (2020). A triazole-substituted aryl iodide with omnipotent reactivity in enantioselective oxidations. Angew. Chem. Int. Ed. 59, 1479–1484. doi:10.1002/anie.201912023
Amey, R. L., and Martin, J. C. (1979). Synthesis and reaction of substituted arylalkoxyiodinanes: formation of stable bromoarylalkoxy and aryldialkoxy heterocyclic derivatives of tricoordinate organoiodine(III). J. Org. Chem. 44, 1779–1784. doi:10.1021/jo01325a007
Bhaskar Pal, K., Di Tommaso, E. M., Inge, A. K., and Olofsson, B. (2023). Stereospecific photoredox-catalyzed vinylations to functionalized alkenes and C-glycosides**. Angew. Chem. Int. Ed. 62, e202301368. doi:10.1002/anie.202301368
Boelke, A., Caspers, L. D., and Nachtsheim, B. J. (2017). NH2-Directed C–H alkenylation of 2-vinylanilines with vinylbenziodoxolones. Org. Lett. 19, 5344–5347. doi:10.1021/acs.orglett.7b02630
Caramenti, P., Declas, N., Tessier, R., Wodrich, M. D., and Waser, J. (2019). Stereoselective synthesis of alkyl-aryl-vinyl- and alkynyl-substituted Z-enamides and enol ethers. Chem. Sci. 10, 3223–3230. doi:10.1039/c8sc05573d
Castoldi, L., Di Tommaso, E. M., Reitti, M., Gräfen, B., and Olofsson, B. (2020b). Electrophilic vinylation of thiols under mild and transition metal-free conditions. Angew. Chem. Int. Ed. 59, 15512–15516. doi:10.1002/anie.202002936
Castoldi, L., Rajkiewicz, A. A., and Olofsson, B. (2020a). Transition metal-free and regioselective vinylation of phosphine oxides and H-phosphinates with VBX reagents. Chem. Commun. 56, 14389–14392. doi:10.1039/d0cc05992g
Chai, J., Ding, W., Wang, C., Ito, S., Wu, J., and Yoshikai, N. (2021). Ritter-type iodo(iii)amidation of unactivated alkynes for the stereoselective synthesis of multisubstituted enamides. Chem. Sci. 12, 15128–15133. doi:10.1039/d1sc05240c
Charpentier, J., Früh, N., and Togni, A. (2015). Electrophilic trifluoromethylation by use of hypervalent iodine reagents. Chem. Rev. 115, 650–682. doi:10.1021/cr500223h
Choi, W. J., Choi, S., Ohkubo, K., Fukuzumi, S., Cho, E. J., and You, Y. (2015). Mechanisms and applications of cyclometalated Pt(ii) complexes in photoredox catalytic trifluoromethylation. Chem. Sci. 6, 1454–1464. doi:10.1039/c4sc02537g
Dahiya, A., Sahoo, A. K., Chakraborty, N., Das, B., and Patel, B. K. (2022). Updates on hypervalent-iodine reagents: metal-free functionalisation of alkenes, alkynes and heterocycles. Org. Biomol. Chem. 20, 2005–2027. doi:10.1039/d1ob02233d
Davies, J., Sheikh, N. S., and Leonori, D. (2017). Photoredox imino functionalizations of olefins. Angew. Chem. Int. Ed. 56, 13361–13365. doi:10.1002/anie.201708497
De, A., Santra, S., Hajra, A., Zyryanov, G. V., and Majee, A. (2019). Visible-light-induced regioselective C(sp3)-H acyloxylation of aryl-2H-azirines with (Diacetoxy)iodobenzene. J. Org. Chem. 84, 11735–11740. doi:10.1021/acs.joc.9b01625
Declas, N., Maynard, J. R. J., Menin, L., Gasilova, N., Götze, S., Sprague, J. L., et al. (2022). Chem. Sci. 20, 12808–12817. doi:10.1039/D2SC04558C
Declas, N., Pisella, G., and Waser, J. (2020). Vinylbenziodoxol(on)es: synthetic methods and applications. Helv. Chim. Acta 103, e2000191. doi:10.1002/hlca.202000191
Ding, W., Chai, J., Wang, C., Wu, J., and Yoshikai, N. (2020). Stereoselective access to highly substituted vinyl ethers via trans-difunctionalization of alkynes with alcohols and iodine(III) electrophile. J. Am. Chem. Soc. 142, 8619–8624. doi:10.1021/jacs.0c04140
Di Tommaso, E. M., Norrby, P., and Olofsson, B. (2022). Explaining regiodivergent vinylations with vinylbenziodoxolones**. Angew. Chem. Int. Ed. 61, e202206347. doi:10.1002/anie.202206347
Fernández González, D., Brand, J. P., Mondière, R., and Waser, J. (2013). Ethynylbenziodoxolones (EBX) as reagents for the ethynylation of stabilized enolates. Adv. Synth. Catal. 355, 1631–1639. doi:10.1002/adsc.201300266
Flores, A., Cots, E., Bergès, J., and Muñiz, K. (2019). Enantioselective iodine(I/III) catalysis in organic synthesis. Adv. Synth. Catal. 361, 2–25. doi:10.1002/adsc.201800521
Frei, R., Wodrich, M. D., Hari, D. P., Borin, P. A., Chauvier, C., and Waser, J. (2014). Fast and highly chemoselective alkynylation of thiols with hypervalent iodine reagents enabled through a low energy barrier concerted mechanism. J. Am. Chem. Soc. 136, 16563–16573. doi:10.1021/ja5083014
Guilbault, A. A., and Legault, C. Y. (2012). Drastic enhancement of activity in iodane-based α-tosyloxylation of ketones: iodine(III) does the hypervalent twist. ACS Catal. 2, 219–222. doi:10.1021/cs200612s
Hara, S., Guan, T., and Yoshida, M. (2006). Stereoselective generation of (E)- and (Z)-2-Fluoroalkylidene-Type carbenoids from (2-fluoro-1-alkenyl)iodonium salts and their application for stereoselective synthesis of fluoroalkenes. Org. Lett. 8, 2639–2641. doi:10.1021/ol0608707
Hari, D. P., Nicolai, S., and Waser, J. (2019). in Patai’s Chemistry of functional groups. Editors B. Olofsson, I. Marek, and Z. Rappoport (United States: Wiley Online Library), 523–580.
Hinkle, R. J., and McDonald, R. (2002). (Z)-2-Methylbuten-1-yl(aryl)iodonium trifluoromethanesulfonates containing electron-withdrawing groups on the aryl moiety. Acta Crystallogr. Sect. C Cryst. Struct. Commun. 58, o117–o121. doi:10.1107/s0108270101019084
Holt, D., and Gaunt, M. J. (2015). Copper-catalyzed oxy-alkenylation of homoallylic alcohols to generate functional syn-1,3-diol derivatives. Angew. Chem. Int. Ed. 54, 7857–7861. doi:10.1002/anie.201501995
Jiang, H., and Studer, A. (2019). Transition-metal-free three-component radical 1,2-amidoalkynylation of unactivated alkenes. Chem. Eur. J. 25, 516–520. doi:10.1002/chem.201805490
Kepski, K., Rice, C. R., and Moran, W. J. (2019). Cyclic vinyl(aryl)iodonium salts: synthesis and reactivity. Org. Lett. 21, 6936–6939. doi:10.1021/acs.orglett.9b02540
Kikuchi, J., Maesaki, K., Sasaki, S., Wang, W., Ito, S., and Yoshikai, N. (2022). Stereoselective synthesis of β-Alkoxy-β-amido vinylbenziodoxoles via iodo(III)etherification of ynamides. Org. Lett. 24, 6914–6918. doi:10.1021/acs.orglett.2c02570
Kokkinidis, G., Hatzigrigoriou, E., Sazou, D., and Varvoglis, A. (1991). Electrochemical reduction of some hypervalent iodine compounds. Electrochim. Acta 36, 1391–1395. doi:10.1016/0013-4686(91)85324-z
Kokkinidis, G., Papadopoulou, M., and Varvoglis, A. (1989). Electrochemical reduction of [bis(acyloxy)iodo]arenes. Electrochim. Acta 34, 133–139. doi:10.1016/0013-4686(89)87078-1
Koposov, A. Y., Litvinov, D. N., Zhdankin, V. V., Ferguson, M. J., McDonald, R., and Tykwinski, R. R. (2006). Eur. J. Org. Chem. 21, 4791–4795. doi:10.1002/ejoc.200600683
Koser, G. F., Sun, G., Porter, C. W., and Youngs, W. J. (1993). Synthesis of 1H-1-(1-alkynyl)-5-methyl-1,2,3-benziodoxathiole 3,3-dioxides: alkynyl(aryl)iodonium sulfonates with heterocyclic iodine. J. Org. Chem. 58, 7310–7312. doi:10.1021/jo00077a071
Laskar, R. A., Ding, W., and Yoshikai, N. (2021). Iodo(III)-Meyer–Schuster rearrangement of propargylic alcohols promoted by benziodoxole triflate. Org. Lett. 23, 1113–1117. doi:10.1021/acs.orglett.1c00039
Le Du, E., Duhail, T., Wodrich, M. D., Scopelliti, R., Fadaei-Tirani, F., Anselmi, E., et al. (2021). Structure and reactivity of N-heterocyclic alkynyl hypervalent iodine reagents. Chem. Eur. J. 27, 10979–10986. doi:10.1002/chem.202101475
Le Du, E., and Waser, J. (2023). Recent progress in alkynylation with hypervalent iodine reagents. Chem. Commun. 59, 1589–1604. doi:10.1039/d2cc06168f
Le Vaillant, F., Garreau, M., Nicolai, S., Gryn’ova, G., Corminboeuf, C., and Waser, J. (2018). Fine-tuned organic photoredox catalysts for fragmentation-alkynylation cascades of cyclic oxime ethers. Chem. Sci. 9, 5883–5889. doi:10.1039/c8sc01818a
Le Vaillant, F., and Waser, J. (2017). Decarboxylative alkynylation and cyanation of carboxylic acids using photoredox catalysis and hypervalent iodine reagents. Chimia 71, 226–230. doi:10.2533/chimia.2017.226
Li, M., Li, W., Lin, C. D., Wang, J. H., and Wen, L. R. (2019). One base for two shots: metal-free substituent-controlled synthesis of two kinds of oxadiazine derivatives from alkynylbenziodoxolones and amidoximes. J. Org. Chem. 84, 6904–6915. doi:10.1021/acs.joc.9b00659
Li, Y., Hari, D. P., Vita, M. V., and Waser, J. (2016). Cyclic hypervalent iodine reagents for atom-transfer reactions: beyond trifluoromethylation. Angew. Chem. Int. Ed. 55, 4436–4454. doi:10.1002/anie.201509073
Liu, B., Alegre-Requena, J. V., Paton, R. S., and Miyake, G. M. (2020). Unconventional reactivity of ethynylbenziodoxolone reagents and thiols: scope and mechanism. Chem. Eur. J. 26, 2386–2394. doi:10.1002/chem.201904520
Liu, B., Lim, C.-H., and Miyake, G. M. (2018). Light-Driven Intermolecular Charge Transfer Induced Reactivity of Ethynylbenziodoxol(on)e and Phenols. J. Am. Chem. Soc. 140, 12829–12835. doi:10.1021/jacs.8b05870
Malmgren, J., Santoro, S., Jalalian, N., Himo, F., and Olofsson, B. (2013). Arylation with unsymmetrical diaryliodonium salts: a chemoselectivity study. Chem. Eur. J. 19, 10334–10342. doi:10.1002/chem.201300860
Merritt, E. A., and Olofsson, B. (2009). Diaryliodonium salts: a journey from obscurity to fame. Angew. Chem. Int. Ed. 48, 9052–9070. doi:10.1002/anie.200904689
Milzarek, T. M., Ramirez, N. P., Liu, X. Y., and Waser, J. (2023). One-pot synthesis of functionalized bis(trifluoromethylated)benziodoxoles from iodine(i) precursors. Chem. Commun. 59, 12637–12640. doi:10.1039/d3cc04525k
Mironova, I. A., Noskov, D. M., Yoshimura, A., Yusubov, M. S., and Zhdankin, V. V. (2023). Aryl-Akynyl-and alkenylbenziodoxoles: synthesis and synthetic applications. Molecules 28, 2136–2179. doi:10.3390/molecules28052136
Ochiai, M., Hirobe, M., Yoshimura, A., Nishi, Y., Miyamot, K., and Shiro, M. (2007). Internal delivery of soft chlorine and bromine Atoms: stereoselective synthesis of (E)-β-Halogenovinyl(aryl)-λ3-iodanes through domino λ3-iodanation−1,4-halogen Shift−Fluorination of alkynes. Org. Lett. 9, 3335–3338. doi:10.1021/ol071345q
Ochiai, M., Sueda, T., Miyamoto, K., Kiprof, P., and Zhdankin, V. V. (2006). Trans influences on hypervalent bonding of aryl λ3-iodanes: their stabilities and isodesmic reactions of benziodoxolones and benziodazolones. Angew. Chem. Int. Ed. 45, 8203–8206. doi:10.1002/anie.200603055
Ochiai, M., Yamamoto, S., Suefuji, T., and Chen, D.-W. (2001). Stereoselective synthesis of (Z)-Enethiols and their Derivatives: vinylic SN2 reaction of (E)-Alkenyl(phenyl)-λ3-iodanes with thioamides. Org. Lett. 3, 2753–2756. doi:10.1021/ol016356c
B. Olofsson, I. Marek, and Z. Rappoport (2019). “The Chemistry of hypervalent halogen compounds,” Patai’s Chemistry of functional groups (United States: Wiley Online Library).
Pisella, G., Gagnebin, A., and Waser, J. (2020). Copper-catalyzed oxyvinylation of diazo compounds. Org. Lett. 22, 3884–3889. doi:10.1021/acs.orglett.0c01150
Radzhabov, M. R., Sheremetev, A. B., and Pivina, T. S. (2020). Oxidative ability of organic iodine(iii) reagents: a theoretical assessment. New J. Chem. 44, 7051–7057. doi:10.1039/d0nj00837k
Rajkiewicz, A. A., and Kalek, M. (2018). N-heterocyclic carbene-catalyzed olefination of aldehydes with vinyliodonium salts to generate α,β-unsaturated ketones. Org. Lett. 20, 1906–1909. doi:10.1021/acs.orglett.8b00447
Ramkumar, N., Baumane, L., Zacs, D., and Veliks, J. (2023). Merging copper(I) photoredox catalysis and iodine(III) Chemistry for the oxy-monofluoromethylation of alkenes. Angew. Chem. Int. Ed. 62, e202219027. doi:10.1002/anie.202219027
Sheng, J., Wang, Y., Su, X., He, R., and Chen, C. (2017). Copper-catalyzed [2+2+2] modular synthesis of multisubstituted pyridines: alkenylation of nitriles with vinyliodonium salts. Angew. Chem. Int. Ed. 56, 4824–4828. doi:10.1002/anie.201700696
Shimbo, D., Shibata, A., Yudasaka, M., Maruyama, T., Tada, N., Uno, B., et al. (2019). Synthesis of cis-β-Amidevinyl benziodoxolones from the ethynyl benziodoxolone–chloroform complex and sulfonamides. Org. Lett. 21, 9769–9773. doi:10.1021/acs.orglett.9b03990
Skucas, E., and MacMillan, D. W. C. (2012). Enantioselective α-vinylation of aldehydes via the synergistic combination of copper and amine catalysis. J. Am. Chem. Soc. 134, 9090–9093. doi:10.1021/ja303116v
Stridfeldt, E., Seemann, A., Bouma, M. J., Dey, C., Ertan, A., and Olofsson, B. (2016). Synthesis, characterization and unusual reactivity of vinylbenziodoxolones—novel hypervalent iodine reagents. Chem. Eur. J. 22, 16066–16070. doi:10.1002/chem.201603955
Tessier, R., Ceballos, J., Guidotti, N., Simonet-Davin, R., Fierz, B., and Waser, J. (2019). “Doubly orthogonal” labeling of peptides and proteins. Chem 5, 2243–2263. doi:10.1016/j.chempr.2019.06.022
Ura, T., Shimbo, D., Yudasaka, M., Tada, N., and Itoh, A. (2020). Synthesis of phenol-derived cis-vinyl ethers using ethynyl benziodoxolone. Chem. Asian J. 15, 4000–4004. doi:10.1002/asia.202001102
Villo, P., and Olofsson, B. (2019). in Patai’s Chemistry of functional groups. Editors B. Olofsson, I. Marek, and Z. Rappoport (Chem: Hypervalent Halogen Compd), 461–522.
Wang, C. S., Tan, P. S. L., Ding, W., Ito, S., and Yoshikai, N. (2022). Regio- and stereoselective synthesis of enol carboxylate, phosphate, and sulfonate esters via iodo(III)functionalization of alkynes. Org. Lett. 24, 430–434. doi:10.1021/acs.orglett.1c04123
Wu, B., Wu, J., and Yoshikai, N. (2017b). Benziodoxole triflate as a versatile reagent for iodo(III)cyclization of alkynes. Chem. Asian J. 12, 3123–3127. doi:10.1002/asia.201701530
Wu, J., Deng, X., Hirao, H., and Yoshikai, N. (2016). Pd-catalyzed conversion of alkynyl-λ3-iodanes to alkenyl-λ3-iodanes via stereoselective 1,2-iodine(III) shift/1,1-hydrocarboxylation. J. Am. Chem. Soc. 138, 9105–9108. doi:10.1021/jacs.6b06247
Wu, J., Deng, X., and Yoshikai, N. (2019). Stereocontrolled synthesis of halovinylbenziodoxoles by hydro- and iodochlorination of ethynylbenziodoxoles. Chem. Eur. J. 25, 7839–7842. doi:10.1002/chem.201901543
Wu, J., Xu, K., Hirao, H., and Yoshikai, N. (2017a). Pd-Catalyzed, ligand-enabled stereoselective 1,2-iodine(III) shift/1,1-carboxyalkynylation of alkynylbenziodoxoles. Chem. A Eur. J. 23, 1521–1525. doi:10.1002/chem.201605772
Wu, J., Xu, K., Hirao, H., and Yoshikai, N. (2023). Private communication to CSD. CCDC Number. 1498944.
Yoshimura, A., Huss, C. D., Liebl, M., Rohde, G. T., Larson, S. M., Frahm, G. B., et al. (2021). Preparation, structure, and reactivity of pseudocyclic β-trifluorosulfonyloxy vinylbenziodoxolone derivatives. Adv. Synth. Catal. 363, 3365–3371. doi:10.1002/adsc.202100341
Yoshimura, A., Saito, A., and Zhdankin, V. V. (2023). Recent progress in synthetic applications of cyclic hypervalent iodine(III) reagents. Adv. Synth. Catal. 365, 2653–2675. doi:10.1002/adsc.202300275
Yoshimura, A., and Zhdankin, V. V. (2016). Advances in synthetic applications of hypervalent iodine compounds. Chem. Rev. 116, 3328–3435. doi:10.1021/acs.chemrev.5b00547
Keywords: alkenes, benziodoxolones, hypervalency, reduction potential, X-ray crystallography, structure-reactivity analysis, VBX
Citation: Doobary S, Di Tommaso EM, Postole A, Inge AK and Olofsson B (2024) Structure-reactivity analysis of novel hypervalent iodine reagents in S-vinylation of thiols. Front. Chem. 12:1376948. doi: 10.3389/fchem.2024.1376948
Received: 26 January 2024; Accepted: 19 February 2024;
Published: 29 February 2024.
Edited by:
Maria Manuel Marques, Universidade Nova de Lisboa, PortugalReviewed by:
Ramesh Mamidala, A pharmaceutical company, United StatesCopyright © 2024 Doobary, Di Tommaso, Postole, Inge and Olofsson. This is an open-access article distributed under the terms of the Creative Commons Attribution License (CC BY). The use, distribution or reproduction in other forums is permitted, provided the original author(s) and the copyright owner(s) are credited and that the original publication in this journal is cited, in accordance with accepted academic practice. No use, distribution or reproduction is permitted which does not comply with these terms.
*Correspondence: Berit Olofsson, YmVyaXQub2xvZnNzb25Ac3Uuc2U=
Disclaimer: All claims expressed in this article are solely those of the authors and do not necessarily represent those of their affiliated organizations, or those of the publisher, the editors and the reviewers. Any product that may be evaluated in this article or claim that may be made by its manufacturer is not guaranteed or endorsed by the publisher.
Research integrity at Frontiers
Learn more about the work of our research integrity team to safeguard the quality of each article we publish.