- 1Department of Chemistry, Yasouj University, Yasouj, Iran
- 2Department of Chemical Engineering, Iran University of Science and Technology (IUST), Tehran, Iran
Synthesis and catalytic application of polymeric-based nanocomposites are important subjects among researchers due to their high lipophilicity as well as high chemical and mechanical stability. In the present work, a novel nanocomposite material involving ionic liquid and high-density polyethylene supported tungstate (PE/IL-WO4=) is synthesized, characterized and its catalytic application is investigated. The coacervation method was used to incorporate 1-methyl-3-octylimidazolium bromide ([MOIm] [Br]) ionic liquid in high-density polyethylene, resulting in a PE/IL composite. Subsequently, tungstate was anchored on PE/IL to give PE/IL-WO4= catalyst. The PXRD, FT-IR, EDX, TGA, and SEM analyses were used to characterize the PE/IL-WO4= composite. This material demonstrated high catalytic efficiency in the synthesis of bioactive tetrahydrobenzo[a]xanthen-11-ones under green conditions. The recoverability and leching tests were performed to investigate the stability and durability of the designed PE/IL-WO4= catalyst under applied conditions.
1 Introduction
Nowadays, one of the most important challenges in organic chemistry is the synthesis and development of chemically stable, highly efficient, and recoverable catalysts (Oozeerally et al., 2018; Jiang et al., 2020; Chen et al., 2023; Jain et al., 2023). To improve the activity and recoverability of homogeneous catalysts, a wide variety of solid materials have been developed as support (Franco et al., 2020; Saito and Kobayashi, 2020; Das et al., 2021). Some attractive supports that have been used for the heterogenization of the catalysts are molecular sieves (Rimaz et al., 2022; Gao et al., 2023), magnetic nanoparticles (Xie and Wang, 2021; Xie and Li, 2023), montmorillonite (Chellapandi and Madhumitha, 2022; Liu et al., 2023), commercial silica (Peron et al., 2021; Chandrashekhar et al., 2022), two-dimensional manganese dioxide (MnO2) (Das et al., 2020), and metal-organic frameworks (MOFs) (Goetjen et al., 2020; Lin et al., 2022). In particular, polymeric materials have drawn a lot of interest as potential catalytic support due to their high stability, easy synthesis and functionalization, and strong corrosion resistance (Gokmen and Du Prez, 2012; Shi et al., 2013; Aziz and Islam, 2018; Jiang et al., 2021; Jiang et al., 2022). Moreover, functionalized polymers are extensively employed in various industries such as packaging (Wan et al., 2020), transportation (Sarkar et al., 2019), biomedical engineering (Szymczyk-Ziółkowska et al., 2020), sporting goods (Sharma et al., 2020), electronics (Zhang et al., 2020), energy storage (Zhang et al., 2021), and water treatment (Khodakarami and Bagheri, 2021; Das et al., 2023). Some recently developed catalysts in this matter are Pd/PVPy (Fusini et al., 2020), Poly-NHC-2–Pd2+ (Xu et al., 2015), Au-NWs@Pd@PEI (Xue et al., 2018), PS-TRIP (Clot-Almenara et al., 2016), and Pd@PANI (Wang et al., 2019), PEEK-TBD (Shi et al., 2023). Among different polymers, polyethylene (PE) is widely regarded as a highly versatile material owing to its exceptional workability, chemical inertness, affordability, high resistance to elevated temperatures, and extensive compatibility with various processing techniques. Therefore, PE is a promising candidate for the immobilization of homogeneous catalysts (Pribyl et al., 2019; Mohebbi and Farajzadeh, 2020; Kargar et al., 2022). Different studies such as LDPE-supported ZVI (Mossmann et al., 2019), PEt@Zn/IL (Zaki et al., 2021), and PEolig-NHC-Ru (Hobbs et al., 2011) have been recently reported in this regard. However, the catalyst leaching and inaccessibility to the active catalytic sites are limitations of the most of the aforementioned systems. Therefore, the design and development of an effective and robust PE-supported catalytic system is an important objective in this matter.
On the other hand, ionic liquids (ILs) are extremely important compounds with a wide range of potential applications because of their hydrophobicity that can be adjusted, excellent solubility with numerous compounds, and negligible vapor pressure. These compounds have a high ability to stabilize polar and charged catalysts due to their inherent ionic nature. For instance, imidazolium-based ionic liquids are highly effective in stabilizing transition metal complexes, thereby enhancing their catalytic activities (Ni and Headley, 2010; Karimi et al., 2018; Gao et al., 2021; Taheri et al., 2023). Thus, combining imidazolium-based ILs with polyethylene supports provides remarkable properties, including high activity, selectivity, and reproducibility.
The synthesis of tetrahydrobenzo[a]xanthen-11-ones has also gained significant attention from chemists due to their notable biological features such as antibacterial, antiviral, anti-tumor, and antimalaria activities (Chibale et al., 2003; Nandi et al., 2011; Mohammadi et al., 2014; Soliman and Khatab, 2018). Tetrahydrobenzo[a]xanthen-11-ones are synthesized via condensation of aromatic aldehydes, 1,3-dicarbonyl compounds, and β-naphthols in the presence of acid catalysts such as NaHSO4-SiO2 (Das et al., 2007), p-toluenesulfonic acid (Janardhan et al., 2012), InCl3/P2O5 (Nandi et al., 2009), Caro’s acid-silica gel (Karimi et al., 2010), ruthenium chloride (Tabatabaeian et al., 2011), MSNBA-5 (Ray et al., 2014) and phenylboronic acid (Goswami et al., 2011). There have been many reported methods to prepare tetrahydrobenzo[a]xanthen-11-ones (Oskooie et al., 2011; Mirjalili et al., 2012; Mondal et al., 2012; Moosavi-Zare et al., 2013; Bahrami et al., 2014). However, these have several drawbacks, including high catalyst loading, low yields of the desired products and the use of pricey ligands, time-consuming workups, challenging product and catalyst separation, and the use of dangerous solvents. Therefore, it is very important to develop an environmentally benign and highly efficient method for the synthesis of tetrahydrobenzo[a]xanthen-11-ones.
In view of the above and considering the advantages of ionic liquid/polymer composites, this study presents the synthesis of a newly developed nanocomposite consisting of polyethylene and an ionic liquid, which serves as a support for tungstate (PE/IL-WO4=). Furthermore, the catalytic efficacy of this nanocomposite in the environmentally friendly synthesis of tetrahydrobenzo[a]xanthen-11-ones is investigated.
2 Experimental section
2.1 Synthesis of PE/IL
The synthesis of the [MOIm] [Br] ionic liquid was done by using a previously reported procedure (Kargar and Elhamifar, 2020). The PE/IL was subsequently synthesized using the coacervation method as described below. In the first step, 1 g of high-density polyethylene (PE) was dissolved in 15 mL of xylene at reflux temperature for 30 min. Afterward, IL (0.4 g) was added to the obtained mixture, and it was heated to reflux for 2 h. Next, the resulting mixture was precipitated in methanol at 4°C. The product was washed completely with MeOH, dried at 70°C for 6 h, and denoted as PE/IL.
2.2 Synthesis of PE/IL-WO4= catalyst
To do this, 1 g of PE/IL was added to DMSO and thoroughly dispersed under ultrasonic irradiation for 30 min. Then 0.30 g (0.82 mmol) of Na2WO4.4H2O was added, and the resulting mixture was stirred at room temperature for 24 h. After filtration, complete washing with EtOH, and drying at 70°C for 5 h, the PE/IL-WO4= product was obtained.
2.3 Synthesis of tetrahydrobenzo[a]xanthen-11-ones in the presence of PE/IL-WO4= catalyst
To do this, 0.10 mol% of PE/IL-WO4= catalyst (based on the amount of W) was added to a mixture of aldehyde (1 mmol), 2-naphthol (1 mmol), dimedone (1 mmol), and ethanol (5 mL). This combination was stirred under reflux conditions. TLC was utilized to monitor the progress of the reaction. After the reaction was finished, the catalyst was removed via filtration, and the pure products were obtained by recrystallizing the residue in EtOH.
2.4 IR, 1H NMR and 13C NMR data of tetrahydrobenzo[a]xanthen-11-ones
2.4.1 9,9-Dimethyl-12-phenyl-8,9,10,12-tetrahydrobenzo[a]xanthen-11-one
White solid; M. P.: 151°C–152°C. FT-IR (KBr, cm−1): 3053 (=C–H, stretching vibration sp2), 2956 (C–H, stretching vibration sp3), 1648 (C=O, stretching vibration), 1619 (C=C, stretching vibration sp2), 1593, 1468 (C=C, Ar stretching vibration sp2), 1230 (C–O, stretching vibration). 1H-NMR (400 MHz, DMSO): δ (ppm) 0.96 (s, 3H), 1.12 (s, 3H), 2.25 (d, 1H, J = 16 Hz), 2.30 (d, 1H, J = 16.3 Hz), 2.56 (s, 2H), 5.70 (s, 1H), 7.07 (t, 1H, J = 7.5 Hz), 7.18 (t, 2H, J = 8 Hz), 7.31–7.45 (m, 5H), 7.77 (d, 1H, J = 8.3 Hz), 7.79 (d, 1H, J = 6.3 Hz), 8.02 (d, 1H J = 8.3 Hz). 13C-NMR (100 MHz, DMSO) δ (ppm) 28.3, 33.0, 33.9, 40.7, 51.1, 117.4, 119.5, 124.1, 125.4, 127.1, 127.7, 128.1, 128.3, 129.0, 129.2, 130.4, 132.8, 142.4, 153.9, 164.4, 197.7.
2.4.2 9,9-Dimethyl-12-(4-chlorophenyl)-8,9,10,12-tetrahydrobenzo[a]xanthen-11-one
White solid; M. P.: 181°C–183 °C. FT-IR (KBr, cm−1): 3068 (=C–H, stretching vibration sp2), 2958 (C–H, stretching vibration sp3), 1652 (C=O, stretching vibration), 1621 (C=C, stretching vibration sp2), 1594, 1479 (C=C, Ar stretching vibration sp2), 1225 (C–O, stretching vibration). 1H-NMR (400 MHz, DMSO): δ (ppm) 0.97 (s, 3H), 1.08 (s, 3H), 2.28 (d, 1H, J = 16.5 Hz), 2.35 (d, 1H, J = 16.1 Hz), 2.64 (s, 2H), 5.73 (s, 1H), 7.36 (d, 1H, J = 9.1), 7.38–7.48 (m, 2H), 7.54 (d, 2H, J = 8.9 Hz), 7.84–7.87 (m, 3H), 8.10 (d, 2H, J = 8.5 Hz). 13C-NMR (100 MHz, DMSO) δ (ppm) 28.3, 33.5, 34.1, 40.7, 51.6, 118.1, 120.0, 123.9, 124.3, 125.6, 127.7, 128.2, 129.3, 129.4, 130.7, 132.8, 147.2, 147.5, 154.3, 164.2, 197.5.
3 Results and discussion
Figure 1 illustrates the preparation method for PE/IL-WO4=. Initially, the coacervation technique was used to immobilize the [MOIm] [Br] into/onto polyethylene to create PE/IL. To synthesize the PE/IL-WO4= composite, the PE/IL material was subsequently treated with Na2WO4.
FT-IR analysis was used to confirm the structure of all prepared materials. Figure 2A shows the characteristic peaks of PE, including the methylene (-CH2-) groups stretching vibration at 2920 and 2819 cm−1, the C-H deformation at 1453 cm−1, and the CH2 rocking stretching vibration at 715 cm−1. The peaks that appeared at 1635 and 1530 cm−1 are attributed to the C=N and C=C bonds of ionic liquid moieties, respectively, confirming the successful incorporation/immobilization of ILs into/onto the polymer framework (Figures 2B, C) (Kargar and Elhamifar, 2020). The band of O-W-O bonds appeared at 828 cm−1 proving the successful immobilization of WO4= on PE/IL composite (Dkhilalli et al., 2018) (Figure 2C).
The PXRD of PE, PE/IL, and PE/IL-WO4= are shown in Figure 3. As seen, all samples exhibit the typical orthorhombic unit cell structure with the two crystal planes of (110) and (200) at angles of 21.6° and 24.0°, respectively. Additionally, the relatively low-intensity peaks at 2θ of 30.2° and 36.5°, are attributed to the (210) and (020) crystal planes, respectively. These findings are in good agreement with the PXRD pattern of high-density polyethylene (Inci and Wagener, 2011; Chouit et al., 2014), proving that the crystalline structure of PE is maintained throughout the modification procedure. This indicates that the incorporation of IL in the polymer matrix did not affect its original crystalline structure.
Moreover, from the EDX analysis, the successful incorporation/immobilization of IL-WO4= complex into/onto the PE network was confirmed by the presence of C, N, O, Br, and W elements (Figure 4), which is in accordance with the FT-IR results.
In addition, the distribution of the above-mentioned elements was studied by using the EDX mapping analysis (Figure 5), indicating a uniform distribution for all elements throughout the material framework.
TG analysis was performed to determine the thermal stability of PE/IL-WO4= composite. The TG curve of the designed catalyst showed two weight losses (Figure 6). The first weight loss, approximately 2%, occurred below 190°C, and resulted from alcoholic solvents and water evaporation. The main weight loss (94%), observed between 400°C and 510°C, is attributed to the decomposition of polyethylene and ionic liquid moieties. These findings demonstrate that the PE/IL-WO4= composite is very thermally stable.
SEM analysis indicates that the PE/IL-WO4= particles have a flower-like morphology with uniform size distribution (Figure 7).
After PE/IL-WO4= was successfully characterized, its catalytic activity was evaluated in the synthesis of tetrahydrobenzo[a]xanthen-11-ones. For this, the condensation between aldehyde (1 mmol), 2-naphthol (1 mmol), and dimedone was considered as a reaction model. To achieve the optimal conditions, the effect of catalyst loadings, solvents, and reaction temperature were investigated (Table 1). It was found that among different solvents of toluene, ethanol, and water, in EtOH the highest product yield is obtained (Table 1, entries 1–3). The amount of catalyst had an impact on the reaction as well, and the highest yield resulted from using 0.10 mol% of PE/IL-WO4= (Table 1, entry 3). The study also demonstrated that the rate of the reaction is affected by temperature, in which the best result was obtained at 78°C (Table 1, entries 6, 7). Accordingly, the use of 0.10 mol% of the PE/IL-WO4= catalyst and EtOH solvent under reflux conditions (78°C) was selected as the optimum conditions. The activity of PE/IL and PE materials was subsequently compared to that of PE/IL-WO4=. No conversion was observed with these W-free materials, confirming that the reaction is actually catalyzed via supported tungsten species (Table 1, entry 3 versus entries 9 and 10). In addition, to elucidate the role of the imidazolium-based IL, the reaction was carried out in the presence of the unsupported WO4= (Table 1, entry 11). Attractively, this latter case showed a significantly lower catalytic activity compared to the PE/IL-WO4=. These observations clearly indicate that the ionic liquid moieties prevent the aggregation of WO4= species and therefore improve their stability and catalytic activity under applied conditions (Wang et al., 2016).
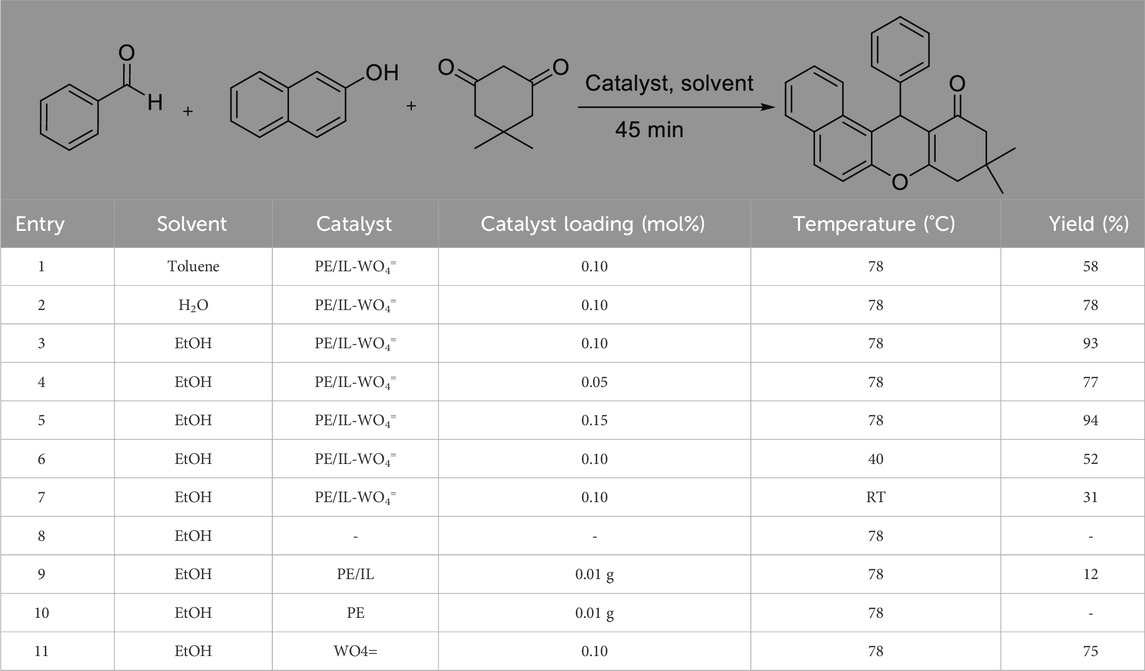
TABLE 1. Effect of catalyst loading, temperature, and solvents in the preparation of tetrahydrobenzo [a]xanthen-11-ones.
Following the optimization of the experimental conditions (Table 1, entry 3, a range of aldehydes were employed as substrate. The tetrahydrobenzo[a]xanthen-11-ones were obtained in high yields using all types of aldehydes, as indicated in Table 2. It is noteworthy that the influence of electronic characteristics and substituent positions on this process was negligible, and the PE/IL-WO4= catalyst can catalyze this procedure effectively.
In the next study, the recoverability and reusability of PE/IL-WO4= were investigated under optimal conditions. For this, the condensation between benzaldehyde, dimedone, and 2-naphthol was used as a test model. After completing the reaction, the PE/IL-WO4= catalyst was separated via filtration and reused under identical conditions as the initial run. These steps were repeated and it was found that PE/IL-WO4= can be recovered and reapplied at least seven times without losing its activity (Figure 8).
The SEM image of the recovered catalyst also showed no significant change in the catalyst morphology after seven recovery times confirming the high stability of the structure of the designed material during the applied conditions (Figure 9).
The PXRD of the recovered PE/IL-WO4= also showed four peaks at 2θ = 22.1°, 24.7°, 30.8°, and 36.9°, which are in good agreement with the PXRD pattern of the fresh catalyst. This analysis also confirms the high stability of the crystalline structure of PE after seven times of recovery and reuse (Figure 10).
In the subsequent study, a leaching test was conducted under applied conditions. To do this, once the reaction had reached approximately 50% completion, the PE/IL-WO4= catalyst was separated, and the progress of the filtrate was monitored. After 120 min, no progress in the reaction was observed. Moreover, the atomic absorption analysis showed that the amount of W in the aforementioned filtrate is lower than 1 ppm. These results confirm no leaching and high stability of supported W sites and also the heterogeneous nature of the designed catalyst.
A plausible mechanism for the synthesis of tetrahydrobenzo[a]xanthen-11-ones using the PE/IL-WO4= catalyst is outlined in Figure 11. At the first step, a Knoevenagel condensation between W-activated aldehyde (1) and 2-naphthol gives intermediate 2. Then, intermediate 3 is formed via Michael-type addition between intermediate 2 and the enol form of dimedone. Finally, intermediate 3 undergoes an intramolecular cyclization followed by tautomerization in the presence of W-sites to give the desired product 5 with a high yield (Ardeshirfard and Elhamifar, 2023).
Next, the efficacy of the PE/IL-WO4= catalyst in the synthesis of tetrahydrobenzo[a]xanthen-11-ones was compared to that of previous catalysts (Table 3). Overall, the results showed that the designed catalyst is better than the other catalysts in terms of temperature, catalyst loading, and number of recycling cycles. This better performance can be attributed to its highly lipophilic backbone, the incorporation of ILs into the material network to prevent leaching of the catalytic active site, and the bifunctional properties (both Lewis acidic and Lewis basic sites) of the supported tungstate. Moreover, PE, with its outstanding properties such as high thermal and chemical resistance, chemical inertness, and cost-effectiveness, provides distinct advantages over other supports.
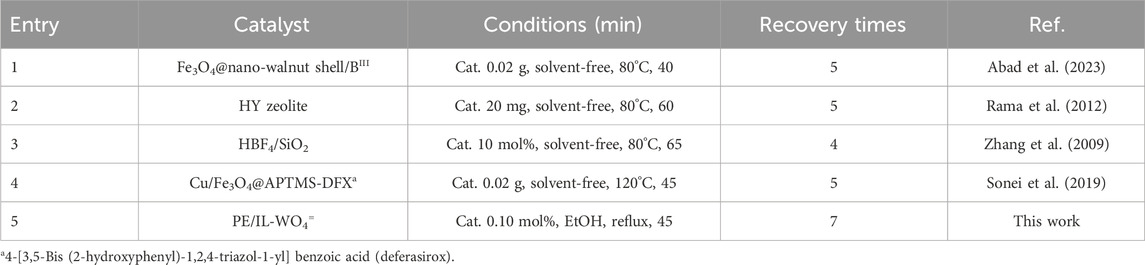
TABLE 3. Comparative study of the performance of the present catalyst with that of previous catalysts.
4 Conclusion
In conclusion, a new composite consisting of high-density polyethylene (PE) and ionic liquid (IL)-WO4= complex was synthesized, characterized and its catalytic application was investigated. The successful immobilization and great stability of the IL-WO4= complex into/onto the polyethylene framework were confirmed through the utilization of FT-IR, TGA, SEM, PXRD, and EDX techniques. The tetrahydrobenzo[a]xanthen-11-one products were effectively prepared under green conditions using the PE/IL-WO4= catalyst, resulting in high yields. With no appreciable decrease in efficiency, the PE/IL-WO4= catalyst was recovered and reused at least seven times. The leaching test and also the atomic absorption analysis showed high stability and no leaching of catalytic active WO4= species during reaction conditions. Moreover, the SEM and PXRD analyses confirmed the high durability of the structure of the designed catalyst under applied conditions. In light of these findings, future investigations on PE/IL-WO4= are warranted to advance its applicability and understanding. As an example, the application of this catalyst in other catalytic processes such as coupling and oxidation reactions is underway in our laboratory. Moreover, both PE/IL and PE/IL-WO4= can also be used as efficient adsorbents for the removal of pollutants from water.
Data availability statement
The original contributions presented in the study are included in the article/Supplementary material, further inquiries can be directed to the corresponding author.
Author contributions
FM: Formal Analysis, Investigation, Writing–original draft. DwE: Conceptualization, Supervision, Visualization, Writing–review and editing. SK: Formal Analysis, Writing–original draft. DvE: Formal Analysis, Resources, Writing–original draft.
Funding
The author(s) declare that no financial support was received for the research, authorship, and/or publication of this article.
Acknowledgments
The authors acknowledge Yasouj University and Iran National Science Foundation (INSF) for supporting this work.
Conflict of interest
The authors declare that the research was conducted in the absence of any commercial or financial relationships that could be construed as a potential conflict of interest.
Publisher’s note
All claims expressed in this article are solely those of the authors and do not necessarily represent those of their affiliated organizations, or those of the publisher, the editors and the reviewers. Any product that may be evaluated in this article, or claim that may be made by its manufacturer, is not guaranteed or endorsed by the publisher.
References
Abad, S. S. S., Mirjalili, B. B. F., and Bamoniri, A. (2023). Fe3O4@ nano-walnut shell/BIII as a new natural based catalyst for synthesis of tetrahydrobenzo [a] xanthene-11-one derivatives. Polycycl. Aromat. Compd. 43, 7979–7991. doi:10.1080/10406638.2022.2144907
Ardeshirfard, H., and Elhamifar, D. (2023). Magnetic cobalt oxide supported organosilica-sulfonic acid as a powerful nanocatalyst for the synthesis of tetrahydrobenzo[a]xanthen-11-ones. Sci. Rep. 13, 14134. doi:10.1038/s41598-023-41234-x
Aziz, S. T., and Islam, R. U. (2018). Polymer-supported Cu–nanoparticle as an efficient and recyclable catalyst for oxidative homocoupling of terminal alkynes. Catal. Lett. 148, 205–213. doi:10.1007/s10562-017-2237-8
Bahrami, K., Khodaei, M. M., and Roostaei, M. (2014). The preparation and characterization of boehmite nanoparticles-TAPC: a tailored and reusable nanocatalyst for the synthesis of 12-aryl-8, 9, 10, 12-tetrahydrobenzo [a] xanthen-11-ones. New J. Chem. 38, 5515–5520. doi:10.1039/c4nj01128g
Chandrashekhar, V. G., Senthamarai, T., Kadam, R. G., Malina, O., Kašlík, J., Zbořil, R., et al. (2022). Silica-supported Fe/Fe–O nanoparticles for the catalytic hydrogenation of nitriles to amines in the presence of aluminium additives. Nat. Catal. 5, 20–29. doi:10.1038/s41929-021-00722-x
Chellapandi, T., and Madhumitha, G. (2022). Montmorillonite clay-based heterogenous catalyst for the synthesis of nitrogen heterocycle organic moieties: a review. Mol. Divers 26, 2311–2339. doi:10.1007/s11030-021-10322-3
Chen, X., Liu, D., Yang, C., Shi, L., and Li, F. (2023). Hexaazatrinaphthalene-based covalent triazine framework-supported rhodium (III) complex: a recyclable heterogeneous catalyst for the reductive amination of ketones to primary amines. Inorg. Chem. 62, 9360–9368. doi:10.1021/acs.inorgchem.3c00301
Chibale, K., Visser, M., van Schalkwyk, D., Smith, P. J., Saravanamuthu, A., and Fairlamb, A. H. (2003). Exploring the potential of xanthene derivatives as trypanothione reductase inhibitors and chloroquine potentiating agents. Tetrahedron 59, 2289–2296. doi:10.1016/s0040-4020(03)00240-0
Chouit, F., Guellati, O., Boukhezar, S., Harat, A., Guerioune, M., and Badi, N. (2014). Synthesis and characterization of HDPE/N-MWNT nanocomposite films. Nanoscale Res. Lett. 9, 288. doi:10.1186/1556-276x-9-288
Clot-Almenara, L., Rodríguez-Escrich, C., Osorio-Planes, L., and Pericas, M. A. (2016). Polystyrene-supported TRIP: a highly recyclable catalyst for batch and flow enantioselective allylation of aldehydes. ACS Catal. 6, 7647–7651. doi:10.1021/acscatal.6b02621
Das, B., Laxminarayana, K., Krishnaiah, M., and Srinivas, Y. (2007). An efficient and convenient protocol for the synthesis of novel 12-aryl- or 12-Alkyl-8,9,10,12-tetrahydrobenzo[a]xanthen-11-one derivatives. Synlett 2007, 3107–3112. doi:10.1055/s-2007-990922
Das, T. K., Ganguly, S., Remanan, S., Ghosh, S., and Das, N. C. (2020). Mussel-inspired Ag/poly(norepinephrine)/MnO2 heterogeneous nanocatalyst for efficient reduction of 4-nitrophenol and 4-nitroaniline: an alternative approach. Res. Chem. Intermed. 46, 3629–3650. doi:10.1007/s11164-020-04165-0
Das, T. K., Jesionek, M., Çelik, Y., and Poater, A. (2023). Catalytic polymer nanocomposites for environmental remediation of wastewater. Sci. Total Environ. 901, 165772. doi:10.1016/j.scitotenv.2023.165772
Das, T. K., Remanan, S., Ghosh, S., Ghosh, S. K., and Das, N. C. (2021). Efficient synthesis of catalytic active silver nanoparticles illuminated cerium oxide nanotube: a mussel inspired approach. Environ. Nanotechnol. Monit. Manag. 15, 100411. doi:10.1016/j.enmm.2020.100411
Dkhilalli, F., Megdiche, S., Guidara, K., Rasheed, M., Barillé, R., and Megdiche, M. (2018). AC conductivity evolution in bulk and grain boundary response of sodium tungstate Na 2 WO 4. Ionics 24, 169–180. doi:10.1007/s11581-017-2193-8
Franco, F., Rettenmaier, C., Jeon, H. S., and Cuenya, B. R. (2020). Transition metal-based catalysts for the electrochemical CO 2 reduction: from atoms and molecules to nanostructured materials. Chem. Soc. Rev. 49, 6884–6946. doi:10.1039/d0cs00835d
Fusini, G., Rizzo, F., Angelici, G., Pitzalis, E., Evangelisti, C., and Carpita, A. (2020). Polyvinylpyridine-supported palladium nanoparticles: an efficient catalyst for Suzuki–Miyaura coupling reactions. Catalysts 10, 330. doi:10.3390/catal10030330
Gao, W., Tang, X., Yi, H., Jiang, S., Yu, Q., Xie, X., et al. (2023). Mesoporous molecular sieve-based materials for catalytic oxidation of VOC: a review. J. Environ. Sci. 125, 112–134. doi:10.1016/j.jes.2021.11.014
Gao, Y., Cheng, L., Gao, R., Hu, G., and Zhao, J. (2021). Deep desulfurization of fuels using supported ionic liquid-polyoxometalate hybrid as catalyst: a comparison of different types of ionic liquids. J. Hazard Mater 401, 123267. doi:10.1016/j.jhazmat.2020.123267
Goetjen, T. A., Liu, J., Wu, Y., Sui, J., Zhang, X., Hupp, J. T., et al. (2020). Metal–organic framework (MOF) materials as polymerization catalysts: a review and recent advances. Chem. Commun. 56, 10409–10418. doi:10.1039/d0cc03790g
Gokmen, M. T., and Du Prez, F. E. (2012). Porous polymer particles—a comprehensive guide to synthesis, characterization, functionalization and applications. Prog. Polym. Sci. 37, 365–405. doi:10.1016/j.progpolymsci.2011.07.006
Goswami, S., Thorat, P., Dhone, S., and Bhusare, S. (2011). Phenylboronic acid-catalyzed synthesis of 9, 9-dimethyl-12-phenyl-9, 10-dihydro-8h-benzo [a] xanthen-11 (12h)-one derivatives. J. Chem. Pharm. Res. 3, 632–635.
Hobbs, C., Yang, Y.-C., Ling, J., Nicola, S., Su, H.-L., Bazzi, H. S., et al. (2011). Thermomorphic polyethylene-supported olefin metathesis catalysts. Org. Lett. 13, 3904–3907. doi:10.1021/ol2014329
Inci, B., and Wagener, K. B. (2011). Decreasing the alkyl branch frequency in precision polyethylene: pushing the limits toward longer run lengths. J. Am. Chem. Soc. 133, 11872–11875. doi:10.1021/ja2040046
Jain, A., Kumari, A., Saha, S. K., Shukla, K., Chauhan, A., Metre, R. K., et al. (2023). Recyclable polymer supported DMAP catalyzed cascade synthesis of α-pyrones. J. Org. Chem. 88, 11346–11351. doi:10.1021/acs.joc.3c01052
Janardhan, B., Vijaya, L., and Rajitha, B. (2012). An efficient synthesis of 12-aryl-8, 9, 10, 12-tetrahydrobenzo [a] xanthen-11-ones using (4-sulfobutyl) tris (4-sulfophenyl) phosphonium hydrogen sulphate as catalyst under neat conditions. J. Chem. Pharm. Res. 4, 519–525.
Jiang, H., Zhang, W., Kang, X., Cao, Z., Chen, X., Liu, Y., et al. (2020). Topology-based functionalization of robust chiral Zr-based metal–organic frameworks for catalytic enantioselective hydrogenation. J. Am. Chem. Soc. 142, 9642–9652. doi:10.1021/jacs.0c00637
Jiang, L., Shi, X.-L., Lv, Y., Gong, H., Liu, S., Du, M., et al. (2022). Acid–base bifunctional catalysis by a heteropolyacid and amines on the polyetheretherketone fiber for cleaner acceleration of the one-pot tandem reactions. J. Ind. Eng. Chem. 113, 439–449. doi:10.1016/j.jiec.2022.06.019
Jiang, W., Xu, J., Sun, W., and Li, Y. (2021). CuSO4 nanoparticles loaded onto poly (toluenesulfonic acid-formaldehyde)/polyethyleneimine composites: an efficient retrievable catalyst for A3/decarboxylative A3 reactions. Appl. Organomet. Chem. 35, e6167. doi:10.1002/aoc.6167
Kargar, S., and Elhamifar, D. (2020). Ionic liquid-containing polyethylene supported palladium: a green, highly efficient and stable catalyst for Suzuki reaction. Mater Today Chem. 17, 100318. doi:10.1016/j.mtchem.2020.100318
Kargar, S., Elhamifar, D., and Elhamifar, D. (2022). Ionic liquid/high-density polyethylene composite supported molybdenum complex: a powerful, highly stable and easy recoverable catalyst. J. Polym. Res. 29, 484. doi:10.1007/s10965-022-03324-8
Karimi, B., Tavakolian, M., Akbari, M., and Mansouri, F. (2018). Ionic liquids in asymmetric synthesis: an overall view from reaction media to supported ionic liquid catalysis. ChemCatChem 10, 3173–3205. doi:10.1002/cctc.201701919
Karimi, N., Oskooie, H. A., Heravi, M. M., and Tahershamsi, L. (2010). Caro's acid–silica gel–catalyzed one-pot synthesis of 12-aryl-8, 9, 10, 12-tetrahydrobenzo [a] xanthen-11-ones. Synth. Communications® 41, 307–312. doi:10.1080/00397910903537372
Khazaei, A., Zolfigol, M. A., Moosavi-Zare, A. R., Zare, A., Khojasteh, M., Asgari, Z., et al. (2012). Organocatalyst trityl chloride efficiently promoted the solvent-free synthesis of 12-aryl-8, 9, 10, 12-tetrahydrobenzo [a]-xanthen-11-ones by in situ formation of carbocationic system in neutral media. Catal. Commun. 20, 54–57. doi:10.1016/j.catcom.2012.01.001
Khodakarami, M., and Bagheri, M. (2021). Recent advances in synthesis and application of polymer nanocomposites for water and wastewater treatment. J. Clean. Prod. 296, 126404. doi:10.1016/j.jclepro.2021.126404
Lin, X.-Y., Qi, M.-Y., Tang, Z.-R., and Xu, Y.-J. (2022). Photochemical dehydrogenation of N-heterocycles over MOF-supported CdS nanoparticles with nickel modification. Appl. Catal. B 317, 121708. doi:10.1016/j.apcatb.2022.121708
Liu, K., Wang, L., Fu, T., Zhang, H., Lu, C., Tong, Z., et al. (2023). Oxygen-functionalized Ti3C2 MXene/exfoliated montmorillonite supported S-scheme BiOBr/Bi2MoO6 heterostructures for efficient photocatalytic quinolone antibiotics degradation. Chem. Eng. J. 457, 141271. doi:10.1016/j.cej.2023.141271
Mirjalili, B., Bamoniri, A., and Zamani, L. (2012). Nano-TiCl4/SiO2: an efficient and reusable catalyst for the synthesis of tetrahydrobenzo [a] xanthenes-11-ones. Lett. Org. Chem. 9, 338–343. doi:10.2174/157017812801264700
Mohammadi, R., Eidi, E., Ghavami, M., and Kassaee, M. Z. (2014). Chitosan synergistically enhanced by successive Fe3O4 and silver nanoparticles as a novel green catalyst in one-pot, three-component synthesis of tetrahydrobenzo [α] xanthene-11-ones. J. Mol. Catal. A Chem. 393, 309–316. doi:10.1016/j.molcata.2014.06.005
Mohebbi, A., and Farajzadeh, M. A. (2020). Chemical synthesis–free and facile preparation of magnetized polyethylene composite and its application as an efficient magnetic sorbent for some pesticides. J. Chromatogr. A 1625, 461340. doi:10.1016/j.chroma.2020.461340
Mondal, J., Nandi, M., Modak, A., and Bhaumik, A. (2012). Functionalized mesoporous materials as efficient organocatalysts for the syntheses of xanthenes. J. Mol. Catal. A Chem. 363-364, 254–264. doi:10.1016/j.molcata.2012.06.017
Moosavi-Zare, A. R., Zolfigol, M. A., Zarei, M., Zare, A., and Khakyzadeh, V. (2013). Preparation, characterization and application of ionic liquid sulfonic acid functionalized pyridinium chloride as an efficient catalyst for the solvent-free synthesis of 12-aryl-8, 9, 10, 12-tetrahydrobenzo [a]-xanthen-11-ones. J. Mol. Liq. 186, 63–69. doi:10.1016/j.molliq.2013.05.009
Mossmann, A., Dotto, G. L., Hotza, D., Jahn, S. L., and Foletto, E. L. (2019). Preparation of polyethylene–supported zero–valent iron buoyant catalyst and its performance for Ponceau 4R decolorization by photo–Fenton process. J. Environ. Chem. Eng. 7, 102963. doi:10.1016/j.jece.2019.102963
Nandi, G. C., Samai, S., Kumar, R., and Singh, M. S. (2009). An efficient one-pot synthesis of tetrahydrobenzo[a]xanthene-11-one and diazabenzo[a]anthracene-9,11-dione derivatives under solvent free condition. Tetrahedron 65, 7129–7134. doi:10.1016/j.tet.2009.06.024
Nandi, M., Mondal, J., Sarkar, K., Yamauchi, Y., and Bhaumik, A. (2011). Highly ordered acid functionalized SBA-15: a novel organocatalyst for the preparation of xanthenes. Chem. Commun. 47, 6677–6679. doi:10.1039/c1cc11007a
Ni, B., and Headley, A. D. (2010). Ionic-Liquid-Supported (ILS) catalysts for asymmetric organic synthesis. Chem. Eur. J. 16, 4426–4436. doi:10.1002/chem.200902747
Oozeerally, R., Burnett, D. L., Chamberlain, T. W., Walton, R. I., and Degirmenci, V. (2018). Exceptionally efficient and recyclable heterogeneous metal–organic framework catalyst for glucose isomerization in water. ChemCatChem 10, 706–709. doi:10.1002/cctc.201701825
Oskooie, H. A., Heravi, M. M., Karimi, N., and Kohansal, G. (2011). Cu/SiO2-catalyzed one-pot synthesis of 12-aryl-8, 9, 10, 12-tetrahydrobenzo [α] xanthen-11-ones under solvent-free conditions. Synth. Commun. 41, 2763–2768. doi:10.1080/00397911.2010.515353
Peron, D. V., Barrios, A. J., Taschin, A., Dugulan, I., Marini, C., Gorni, G., et al. (2021). Active phases for high temperature Fischer-Tropsch synthesis in the silica supported iron catalysts promoted with antimony and tin. Appl. Catal. B 292, 120141. doi:10.1016/j.apcatb.2021.120141
Pribyl, J., Benicewicz, B., Bell, M., Wagener, K., Ning, X., Schadler, L., et al. (2019). Polyethylene grafted silica nanoparticles prepared via surface-initiated ROMP. ACS Macro Lett. 8, 228–232. doi:10.1021/acsmacrolett.8b00956
Rama, V., Kanagaraj, K., and Pitchumani, K. (2012). A multicomponent, solvent-free, one-pot synthesis of benzoxanthenones catalyzed by HY zeolite: their anti-microbial and cell imaging studies. Tetrahedron Lett. 53, 1018–1024. doi:10.1016/j.tetlet.2011.10.143
Ray, S., Bhaumik, A., Pramanik, M., Butcher, R. J., Yildirim, S. O., and Mukhopadhyay, C. (2014). Binary conjugate Brønsted–Lewis acid supported on mesoporous silica nanoparticles for the domino addition/elimination/addition and addition/elimination/addition/cyclization cascade. Catal. Commun. 43, 173–178. doi:10.1016/j.catcom.2013.10.012
Rimaz, S., Kosari, M., Zarinejad, M., and Ramakrishna, S. (2022). A comprehensive review on sustainability-motivated applications of SAPO-34 molecular sieve. J. Mater Sci. 57, 848–886. doi:10.1007/s10853-021-06643-1
Saito, Y., and Kobayashi, S. (2020). Development of robust heterogeneous chiral rhodium catalysts utilizing acid–base and electrostatic interactions for efficient continuous-flow asymmetric hydrogenations. J. Am. Chem. Soc. 142, 16546–16551. doi:10.1021/jacs.0c08109
Sarkar, B., Tayyab, M., Kim, N., and Habib, M. S. (2019). Optimal production delivery policies for supplier and manufacturer in a constrained closed-loop supply chain for returnable transport packaging through metaheuristic approach. Comput. Ind. Eng. 135, 987–1003. doi:10.1016/j.cie.2019.05.035
Sharma, S., Sudhakara, P., Misra, S., and Singh, J. (2020). A comprehensive review of current developments on the waste-reinforced polymer-matrix composites for automotive, sports goods and construction applications: materials, processes and properties. Mater Today Proc. 33, 1671–1679. doi:10.1016/j.matpr.2020.06.523
Shi, X.-L., Lv, Y., Zhang, T., Hu, Q., Shi, K., Zhang, W., et al. (2023). Polyetheretherketone fiber-supported TBD as an efficient fibrous superbase catalyst for organic conversions in continuous-flow processing. J. Catal. 418, 110–120. doi:10.1016/j.jcat.2023.01.010
Shi, X.-L., Zhang, M., Li, Y., and Zhang, W. (2013). Polypropylene fiber supported ionic liquids for the conversion of fructose to 5-hydroxymethylfurfural under mild conditions. Green Chem. 15, 3438–3445. doi:10.1039/c3gc41565a
Soliman, H. A., and Khatab, T. K. (2018). New approach for tetrachlorosilane promoted One-Pot, condensation reaction for Tetrahydrobenzo [a] Xanthene-11-Ones with docking validation as Aurora Kinase Inhibitor. Silicon 10, 229–233. doi:10.1007/s12633-016-9421-0
Sonei, S., Gholizadeh, M., and Taghavi, F. (2019). Cu (II) anchored on modified magnetic nanoparticles: as a green and efficient recyclable nano catalyst for one pot synthesis of 12-Aryl-8, 9, 10, 12tetrahydrobenzo [a] xanthene-11-oneʺ. Polycycl. Aromat. Compd. 40, 1127–1142. doi:10.1080/10406638.2018.1531431
Szymczyk-Ziółkowska, P., Łabowska, M. B., Detyna, J., Michalak, I., and Gruber, P. (2020). A review of fabrication polymer scaffolds for biomedical applications using additive manufacturing techniques. Biocybern. Biomed. Eng. 40, 624–638. doi:10.1016/j.bbe.2020.01.015
Tabatabaeian, K., Khorshidi, A., Mamaghani, M., Dadashi, A., and Jalali, M. K. (2011). One-pot synthesis of tetrahydrobenzo[a]xanthen-11-one derivatives catalyzed by ruthenium chloride hydrate as a homogeneous catalyst. Can. J. Chem. 89, 623–627. doi:10.1139/v11-042
Taheri, K., Elhamifar, D., Kargar, S., and Zarnegaryan, A. (2023). Graphene oxide supported ionic liquid/Fe complex: a robust and highly stable nanocatalyst. RSC Adv. 13, 16067–16077. doi:10.1039/d3ra01438j
Wan, Y.-J., Li, G., Yao, Y.-M., Zeng, X.-L., Zhu, P.-L., and Sun, R. (2020). Recent advances in polymer-based electronic packaging materials. Compos Commun. 19, 154–167. doi:10.1016/j.coco.2020.03.011
Wang, G., Yuan, S., Wu, Z., Liu, W., Zhan, H., Liang, Y., et al. (2019). Ultra-low-loading palladium nanoparticles stabilized on nanocrystalline Polyaniline (Pd@ PANI): a efficient, green, and recyclable catalyst for the reduction of nitroarenes. Appl. Organomet. Chem. 33, e5159. doi:10.1002/aoc.5159
Wang, H., Liu, Z., Wu, Y., Yao, Z., Zhao, W., Duan, W., et al. (2016). Preparation of highly dispersed W/Al2O3 hydrodesulfurization catalysts via a microwave hydrothermal method: effect of oxalic acid. Arab. J. Chem. 9, 18–24. doi:10.1016/j.arabjc.2014.11.023
Xie, W., and Li, J. (2023). Magnetic solid catalysts for sustainable and cleaner biodiesel production: a comprehensive review. Renew. Sust. Energ Rev. 171, 113017. doi:10.1016/j.rser.2022.113017
Xie, W., and Wang, H. (2021). Grafting copolymerization of dual acidic ionic liquid on core-shell structured magnetic silica: a magnetically recyclable Brönsted acid catalyst for biodiesel production by one-pot transformation of low-quality oils. Fuel 283, 118893. doi:10.1016/j.fuel.2020.118893
Xu, S., Song, K., Li, T., and Tan, B. (2015). Palladium catalyst coordinated in knitting N-heterocyclic carbene porous polymers for efficient Suzuki–Miyaura coupling reactions. J. Mater Chem. A 3, 1272–1278. doi:10.1039/c4ta05265j
Xue, Q., Bai, J., Han, C., Chen, P., Jiang, J.-X., and Chen, Y. (2018). Au nanowires@ Pd-polyethylenimine nanohybrids as highly active and methanol-tolerant electrocatalysts toward oxygen reduction reaction in alkaline media. ACS Catal. 8, 11287–11295. doi:10.1021/acscatal.8b03447
Zaki, E. G., Mohmed, D., Hussein, M. F., El-Zayat, M. M., Soliman, F. S., and Aman, D. (2021). Assessment of polyethylene/Zn-ionic as a diesel fuel sulfur adsorbent: gamma radiation effect and response surface methodology. Environ. Sci. Pollut. Res. 28, 52993–53009. doi:10.1007/s11356-021-14501-5
Zhang, G., Li, Q., Allahyarov, E., Li, Y., and Zhu, L. (2021). Challenges and opportunities of polymer nanodielectrics for capacitive energy storage. ACS Appl. Mater Interfaces 13, 37939–37960. doi:10.1021/acsami.1c04991
Zhang, J., Lei, Z., Luo, S., Jin, Y., Qiu, L., and Zhang, W. (2020). Malleable and recyclable conductive mwcnt-vitrimer composite for flexible electronics. ACS Appl. Nano Mater 3, 4845–4850. doi:10.1021/acsanm.0c00902
Zhang, Z.-H., Wang, H.-J., Ren, X.-Q., and Zhang, Y.-Y. (2009). A facile and efficient method for synthesis of xanthone derivatives catalyzed by HBF 4/SiO 2 under solvent-free conditions. Monatsh fur Chem. 140, 1481–1483. doi:10.1007/s00706-009-0204-9
Keywords: polyethylene, tetrahydrobenzo[a]xanthen-11-ones, ionic liquid, nanocomposite, catalyst
Citation: Mousavi F, Elhamifar D, Kargar S and Elhamifar D (2024) Ionic liquid containing high-density polyethylene supported tungstate: a novel, efficient, and highly recoverable catalyst. Front. Chem. 12:1346108. doi: 10.3389/fchem.2024.1346108
Received: 28 November 2023; Accepted: 06 February 2024;
Published: 29 February 2024.
Edited by:
Zhen Ma, Fudan University, ChinaReviewed by:
Asim Bhaumik, Indian Association for the Cultivation of Science (IACS), IndiaTushar Kanti Das, Silesian University of Technology, Poland
Jian He, Jishou University, China
Copyright © 2024 Mousavi, Elhamifar, Kargar and Elhamifar. This is an open-access article distributed under the terms of the Creative Commons Attribution License (CC BY). The use, distribution or reproduction in other forums is permitted, provided the original author(s) and the copyright owner(s) are credited and that the original publication in this journal is cited, in accordance with accepted academic practice. No use, distribution or reproduction is permitted which does not comply with these terms.
*Correspondence: Dawood Elhamifar, ZC5lbGhhbWlmYXJAeXUuYWMuaXI=