- 1Pharmaceutical Sciences Research Center, School of Pharmacy, Shiraz University of Medical Sciences, Shiraz, Iran
- 2Department of Pharmaceutics, School of Pharmacy, Shiraz University of Medical Sciences, Shiraz, Iran
Hydrogels are hydrophilic, three-dimensional, cross-linked polymers that absorb significant amounts of biological fluids or water. Hydrogels possess several favorable properties, including flexibility, stimulus-responsiveness, versatility, and structural composition. They can be categorized according to their sources, synthesis route, response to stimulus, and application. Controlling the cross-link density matrix and the hydrogels’ attraction to water while they’re swelling makes it easy to change their porous structure, which makes them ideal for drug delivery. Hydrogel in drug delivery can be achieved by various routes involving injectable, oral, buccal, vaginal, ocular, and transdermal administration routes. The hydrogel market is expected to grow from its 2019 valuation of USD 22.1 billion to USD 31.4 billion by 2027. Commercial hydrogels are helpful for various drug delivery applications, such as transdermal patches with controlled release characteristics, stimuli-responsive hydrogels for oral administration, and localized delivery via parenteral means. Here, we are mainly focused on the commercial hydrogel products used for drug delivery based on the described route of administration.
1 Introduction
From scientific laboratories to clinical applications, the rise of commercial hydrogels has received significant attention in the field of biological advancements. The term “hydrogel” was first used by Van n Bemmelen in 1894. Later, in 1960, Lim and Wichterle demonstrated that hydrogels composed of poly (2-hydroxyethyl methacrylate) had potential applications as a filler after enucleation of the eye, fabrication of contact lenses, drug carriers, and arteries. As researchers delve deeper into the remarkable properties of these hydrophilic networks, their potential to revolutionize diverse biological applications becomes increasingly evident (van Bemmelen, 1894; Wichterle and LÍM, 1960; Peppas et al., 2006).
Hydrogels are complex networks of hydrophilic polymers that form three-dimensional (3-D) structures, enabling them to absorb and maintain significant volumes of biological fluids or water while preserving the integrity of their structure (Morteza et al., 2016). This balance relies on various factors, such as the selection of polymer types, the response to pH changes, cross-link density, and the behavior displayed in biological environments (Ullah et al., 2015). Functional polymer gels can swell because they have hydrophilic functional groups attached to polymer chains and cross-links between the chains (Douglas, 2018; Tanpichai et al., 2022). Hydrophilic functional groups, such as sulfates, carboxylic acids, hydroxyl groups, and amides, enable hydrogels to retain water and exhibit swelling behavior (Kesharwani et al., 2021).
Hydrogels offer remarkable versatility and are utilized in different subjects owing to their unique structures and compatibility with varying conditions of use (Morteza et al., 2016). Hydrogels show outstanding biomimetic properties because of multifunctional qualities, such as flexibility, softness, nontoxicity, biocompatibility, and biodegradability. These properties enable numerous biomedical, pharmaceutical, and other biomedical applications (Caló and Khutoryanskiy, 2015; Ahmad et al., 2019; Volpi et al., 2022). The particular physical characteristics of hydrogels have generated considerable investigation into their prospective uses in drug delivery applications. These biocompatible hydrogels have very advantageous physical characteristics, such as elasticity, that provide controlled release and long-term protection for the encapsulated entities (Gull et al., 2020). The porous structure of hydrogels can be easily modified by controlling the density of cross-links within the gel matrix and the hydrogels’ affinity for the external aqueous environment during swelling (Hoare and Kohane, 2008). The rapid diffusion of drug molecules into and out of swollen hydrogels, which involves drug entrapment and release, enables the utilization of cross-linked polymer networks in a hydrated or dehydrated state as effective carriers for drug delivery via various routes such as vaginal, ocular, oral, buccal, and parenteral administration (Erol et al., 2019).
The use of hydrogels in drug delivery systems has seen an increase in recent years. The current valuation of the hydrogel market stands at USD 22.1 billion as of 2019, with projected growth to reach USD 31.4 Billion by the year 2027. This growth is anticipated at a compound annual growth rate (CAGR) of 6.7% from 2020 to 2027. A rise in the utilization of hydrogel products stands as a significant stimulant for expanding the hydrogel market over the next few years (Cascone and Lamberti, 2020). Commercial hydrogels can be obtained for diverse drug delivery applications, including localized delivery through the parenteral route, stimuli-responsive hydrogels for oral delivery, and controlled release properties for transdermal patches (Shi and Li, 2005; Sharpe et al., 2014a; Zielińska et al., 2022).
Research on hydrogel has been ongoing for many years, leading to the development of products that have found numerous applications in the drug delivery system. Therapeutic drugs are now loaded into polymer-based carriers, and the delivery and release of drug molecules is a topic of great interest in many medical fields (Roointan et al., 2018; Farjadian et al., 2019a; Farjadian et al., 2020; Farzanfar et al., 2021; Ghasemi et al., 2022a; Bahmani et al., 2022). The carriers facilitate the transportation of drugs to the specific target location (Entezar-Almahdi et al., 2021; Ghasemi et al., 2022b). Numerous studies have been published on commercial hydrogels for biomedical applications, specifically wound dressings, contact lenses, and cosmetics (Cascone and Lamberti, 2020). Following our previous reports on pharmaceutical applications of precious advanced materials (Farjadian et al., 2019b; Farjadian et al., 2019c; Ahmadi et al., 2020; Entezar-Almahdi et al., 2020; Hoseini-Ghahfarokhi et al., 2020; Zarkesh et al., 2021; Hejabi et al., 2022), in this paper we will discuss various commercial hydrogel products used for drug delivery, focusing on different routes of administration such as injectable, oral, buccal, ophthalmic, vaginal, and transdermal routes (Figure 1). Each section contains a table with an overview of the products on the market, their manufacturer, and their main ingredients.
2 Classification of hydrogel products
Hydrogels are cross-linked networks of hydrophilic polymeric chains. Hydrogels absorb about 70%–99% of their weight in water and can be used to formulate semi-solid delivery systems for labile and hydrophilic drugs (Narayanaswamy and Torchilin, 2020). Hydrogels can be classified in multiple ways (Figure 2). Based on their source, hydrogels are categorized as follows: natural hydrogels are biodegradable and biocompatible; synthetic hydrogels are non-toxic and compatible; and hybrid hydrogels, which combine synthetic and natural polymers for use in biomedical applications, are available (Malpure et al., 2018). Based on the synthesis route, hydrogels can be categorized into homopolymers, copolymers, and multipolymers. Hydrogels can exhibit different charge characteristics on the bound groups, leading to their classification as cationic, anionic, ampholytic, or electrically neutral, depending on the ionic charges present (Mahinroosta et al., 2018). Hydrogels, as complex polymeric structures, can display swelling behavior in response to various external stimuli. These stimuli can include alterations in pH, temperature, electromagnetic radiation, ionic strength, and other similar factors (Farjadian et al., 2023). The swelling of a polymeric chain occurs due to the hydration of hydrophilic and polar groups. The polar moieties expand, exposing hydrophobic groups that interact with water molecules. The polymeric network absorbs more water through an osmotic force, leading to infinite dilution (Holback et al., 2011). Hydrogels, according to the type of cross-linking, can be classified into two distinct categories: chemically cross-linked networks, characterized by the presence of permanent junctions, and physical networks, characterized by the formation of temporary junctions through polymer chain entanglements or physical interactions (Malpure et al., 2018). From the initial studies on the pharmaceutical applications of hydrogels, an extensive range of drug delivery systems were designed to prolong therapeutic efficacy and accomplish targeted delivery to particular tissues or organs. Hydrogels are divided into four groups concerning the mechanism controlling the drug release: 1) swelling-controlled, 2) diffusion-controlled, 3) chemically-controlled, and 4) environment-responsive systems (Mohite and Adhav, 2017).
3 Drug delivery commercial product
Hydrogels are frequently utilized as drug delivery vehicles. The commercial hydrogel-based drug delivery products are discussed in the following section according to the administration route.
3.1 Injectable drug delivery product
There has been a growing focus on injectable hydrogels compared to conventional gels in recent years. This is mainly due to their minimally invasive nature during surgery and ability to change shape in real time (Tan and Marra, 2010). Injectable hydrogels can be implanted through a minimally invasive procedure, significantly reducing patient discomfort and pain and decreasing healing time. Furthermore, this approach is cost-effective and applicable to hard-to-reach tissue sites (Rizzo and Kehr, 2021). Injectable hydrogels with multiple functions can be utilized to treat cancer, diabetes, and gene therapy by enabling the effective delivery of numerous pharmaceuticals and other substances, including proteins (Huang and Brazel, 2001). The main types of injectable hydrogels are synthesized by chemical or physical cross-linking.
Physically cross-linked hydrogels, developed by non-covalent secondary forces, are preferable for a sustained delivery system. In contrast, injectable hydrogels created by chemical cross-linking offer superior long-term stability and mechanical qualities but may cause adverse effects due to toxic crosslinkers (Yu and Ding, 2008; Mathew et al., 2018). Novel types of hydrogels, like dual cross-linking hydrogels, nanocomposite hydrogels, and slide-ring hydrogels, are developed to enhance mechanical properties (Figure 3) (Katsuno et al., 2013; Zhao et al., 2016).
Temperature-responsive hydrogels are as attractive as physical gels because they can be customized for specific purposes. These materials possess a biologically compatible in situ setting process, allowing for the incorporation of cells, bioactive compounds, and even intricate 3-D structures (Han et al., 2018). Hydrogel has been extensively investigated for localized therapy, but few have been commercialized because of its complicated delivery system, difficulties in large-scale production, failure to pass clinical trials, and related side effects (Mishra and Singh, 2021). Table 1 contains a list of several commercial products.
3.2 Oral drug delivery product
The oral route is a popular drug delivery method owing to its numerous benefits, including sustained and controlled drug delivery, ease of administration, feasibility of solid formulation, and high level of patient compliance (Homayun et al., 2019). The gastrointestinal tract (GIT) has a complex structure and functions that notably impact the release, dissolution, and absorption of orally administered dosage forms. This impact is primarily attributed to factors such as enzyme content, bile salts, acidity, and the mucosal absorptive surface of the GIT (Shah et al., 2017). Hydrogels are employed in smart oral delivery systems for hydrophobic biological molecules, facilitating site-specific dispersion of medicinal compounds within the intricate GIT. Traditional methods encounter obstacles such as limited permeability of the epithelial membrane and denaturation of drugs (Carr et al., 2010). Stimuli-responsive hydrogels are vital in oral delivery as they can respond to environmental changes. These hydrogels can be triggered by various physical and chemical stimuli, like pH, light, ionic strength, solvent composition, temperature, and electric field (Peppas et al., 2000; Farjadian et al., 2022). Drug delivery systems can be designed to administer therapeutic substances to specific organs in a regulated and predictable manner (Gull et al., 2019a). In the complicated environment of the GI tract, hydrogels protect therapeutic agents and allow targeted delivery by taking advantage of basic physiological changes (Figure 4) (Sharpe et al., 2014b). There have been studies of controlled release systems used in various pH ranges within the body, including the oral, intestines, gastric, and periodontal areas (Gull et al., 2019b). Hydrogels were extensively studied for their potential application in oral drug delivery of insulin, trying to solve the challenges associated with parenteral insulin administration. However, despite numerous advances in this field, progress has been limited, and there is now no commercially successful oral insulin product available for human use (Chaturvedi et al., 2013). Biopolymers such as alginate, hydroxypropyl cellulose, soybean protein, pectin, and cellulose acetate have been the subject of significant investigation in the field of GIT drug delivery (Vashist et al., 2014). Researchers are now working on the development of cost-effective and efficient drug delivery methods utilizing hydrogel materials. Numerous hydrogel systems have been designed to achieve controlled drug release through the utilization of various mechanisms that arise during the administration of medications through the oral route. Despite the challenges encountered by oral drug delivery, these systems have been designed to successfully solve these obstacles (Farrukh et al., 2023). Some examples of these commercial products are included in Table 2.
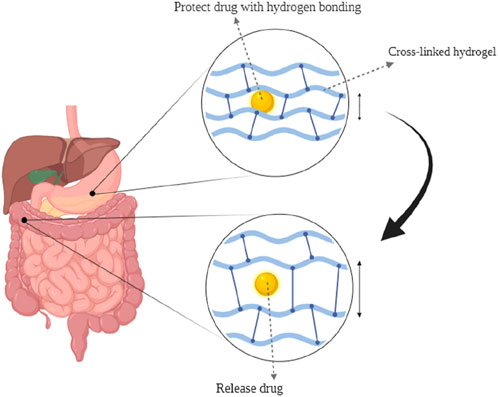
FIGURE 4. (1) preserving the drug in a low-pH environment. (2) Hydrogen bonds between polymer chains cause the carrier to become complex.
3.3 Buccal drug delivery product
The buccal mucosa is highly accessible and characterized by a wide area of smooth muscle and generally static mucosa, making it an ideal site for administering retentive dosage forms. The internal jugular vein bypasses the hepatic first-pass metabolic process to allow direct entry of medicines into the systemic circulation, resulting in increased bioavailability. Additional benefits include minimal enzymatic activity, compatibility with medications or excipients that cause only minor and reversible mucosal damage or irritation, administration without pain, and convenient drug withdrawal (Sudhakar et al., 2006). Drug administration through the buccal route provides a viable alternative for drug delivery to the systemic circulation. In the study of Hu et al., a mucoadhesive film inspired by mussels and containing polydopamine (DOPA) nanoparticles has been shown to have more significant advantages for transporting drugs over the mucosal barrier, as well as increased drug bioavailability and therapeutic efficacy in models of oral mucositis (Hu et al., 2021). The synthesis of PVA-DOPA polymers involved the modification of poly (vinyl alcohol) (PVA) using the mussel adhesive protein DOPA. Then, different PLGA (poly (D,L-lactide-co-glycolide) nanoparticles were integrated into the PVA-DOPA film to create a combined buccal drug delivery system with dexamethasone (Dex) known as the PVA-DOPA@NPs-Dex film (Figure 5). The nanoparticles are slowly released from the film, then move through the mucus layer and pass through the epithelium. This results in sustained drug release and increased therapeutic efficacy in the treatment of oral mucositis.
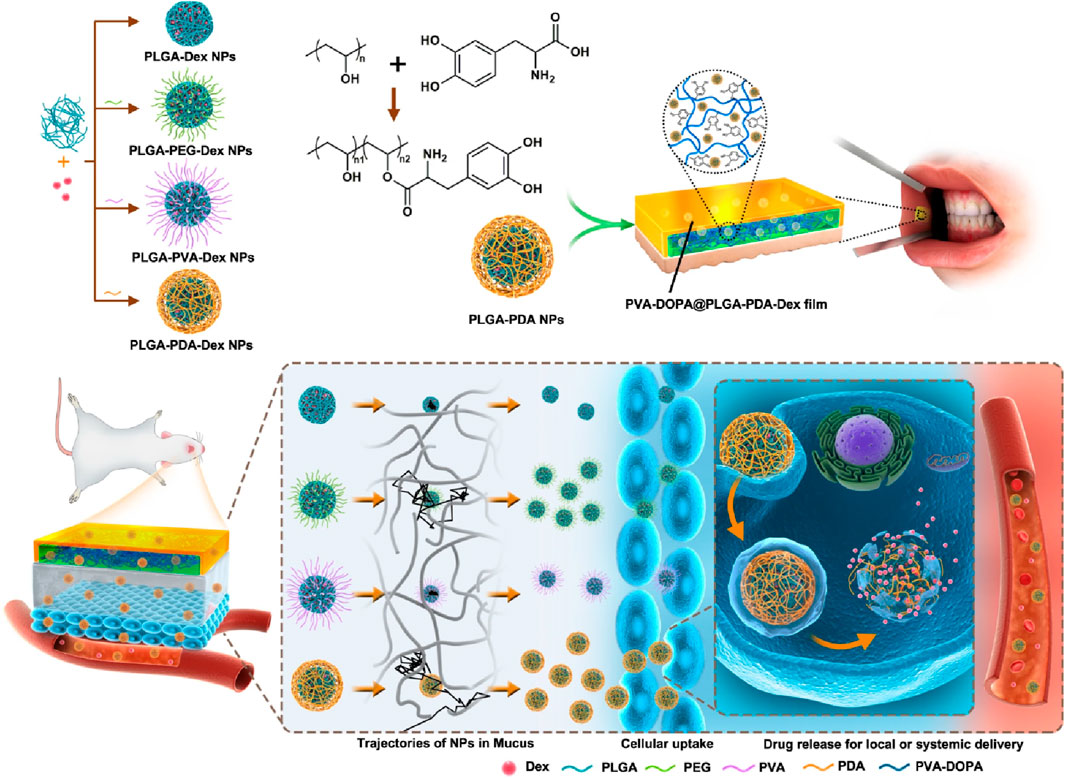
FIGURE 5. The synthesis and biomedical application of PVA-DOPA@NPs-Dex mucoadhesive film enhances mucoadhesion for buccal drug delivery. PVA: poly (vinyl alcohol), DOPA: 3,4-dihydroxy-D-phenylalanine, NPs: nanoparticles, Dex: dexamethasone. Adopted from (Hu et al., 2021).
Various commercial buccal medication administration dosage forms are available on the market, including buccal tablets, sprays, mucoadhesive formulations, sublingual lozenges, chewing gums, films, and solutions (Nagai and Machida, 1993). There are numerous disadvantages associated with buccal drug delivery. Firstly, the buccal mucosa acts like a barrier that may limit the permeability of certain drugs. Secondly, the presence of saliva can potentially dilute the drug, thereby affecting its absorption. Lastly, the oral cavity experiences a variable environment due to factors such as food consumption and other daily activities. To address this challenge, many strategies can be employed for buccal drug delivery, including physical penetration enhancers like iontophoresis, chemical penetration enhancers like surfactants, and formulation technologies like polymeric and mucoadhesive dosage forms (Wanasathop et al., 2021). Their bioadhesive qualities significantly influence the choice of hydrogel delivery methods. When a hydrogel has high adhesion to the epithelium, it can extend the system’s retention at a target site, delivering enough drug doses for the intended therapeutic effect. This is especially crucial for buccal delivery (Peppas and Sahlin, 1996). Specific polymers, like poly (acrylic acid) and chitosan, have been observed to possess mucoadhesive properties. Poly (acrylic acid) can establish hydrogen bonds with the mucosa, whereas chitosan, which carries a positive charge, can engage in electrostatic interactions with negatively charged surfaces of tissues and cells (Li and Mooney, 2016). Hydrogel carriers for drug delivery contain the potential to control the release rate of drugs based on the hydration state. This hydration level determines the hydrogel’s swelling ability (Narayanaswamy and Torchilin, 2020). In the field of drug delivery, a variety of material-based hydrogels have been commercially applied. Some commonly used hydrogels include hydroxyethyl cellulose, hydroxypropyl cellulose, polyvinyl alcohol, polyacrylic resins, carboxymethyl cellulose, and hydroxypropyl methylcellulose (Cascone and Lamberti, 2020). Some of these commercial products are listed in Table 3.
3.4 Ocular drug delivery product
The human eye is a complex, spherical organ. The structure can be anatomically separated into two compartments, namely, the anterior and posterior segments. Ophthalmic drugs administered to the anterior section of the eye encounter challenges from dynamic and static barriers. The main barriers are the blood-queous barrier, corneal epithelium, corneal stroma, lymph flow, conjunctival blood flow, and tear drainage. These factors play a critical role in the formulation and development of ophthalmic therapies (Torres-Luna et al., 2020). Drug contact with ocular surface tissues is brief, often lasting between 1 and 2 min. This is due to the continuous generation of tears, which range from 0.5 to 2.2 μL per minute, as well as the turnover of tears (Sánchez-López et al., 2017). Hydrogels possess the capability to effectively resist the rapid blinking and flushing actions of tears, thereby extending the duration of drug presence on the eye surface. This prolonged drug resident time facilitates enhanced drug efficacy regarding localized therapeutic action on mucosal surfaces or more profound penetration into eye tissues. Hydrogels can extend the duration of drug presence on the ocular surface and maintain a controlled release of drugs within the intraocular tissues, including the vitreous cavity and aqueous humor (Cooper and Yang, 2019). The versatility and modifiability of hydrogels make them ideal for efficiently transporting medications to the eye, as they may be designed to take advantage of the specific function and environment for which they are developed (Torres-Luna et al., 2020; MohammadSadeghi et al., 2021). Figure 6 illustrates the diverse range of potential applications of hydrogels in therapeutic ophthalmic.
Hydrogels can augment medication retention on the ocular surface by elevating solution viscosity, facilitating their swelling in water or aqueous solvents. Chitosan, hydroxypropyl cellulose, dextran, hydroxypropyl methylcellulose, and poly (acrylic acid) derivatives, including polycarbophil and carbomer 934, have been identified in studies that are most appropriate for bioadhesive polymers for ocular medication administration (Fathi et al., 2015). Table 4 is a compilation of several commercial products.
3.5 Vaginal drug delivery product
The vagina is an essential reproductive organ made of a 7.50-cm-long muscular canal located between the urethra, rectum, and bladder. The vaginal membrane is composed of three different layers, including the muscular coat, epithelial layer, and tunica adventitia. The thickness of the vaginal epithelium is dependent upon various factors, including age, hormonal activity, and life phases. The vaginal branch of the uterine artery is responsible for providing blood circulation to the vagina (Valenta, 2005). The vaginal route of drug administration is thought to be highly advantageous owing to its extensive blood flow, capacity to elude the first-pass effect, and significant permeability to many pharmacological compounds, including peptides and proteins (Aka-Any-Grah et al., 2010). A variety of pharmacological groups, including antimicrobials, labor inducers, sexual hormones, and spermicides, have been delivered via the vaginal mucosa. The majority of these drugs are employed for localized conditions, while a few of them can achieve serum concentrations that are adequate for producing systemic effects (Alexander et al., 2004). Vaginal drug delivery methods use natural or synthetic polymers to facilitate drug interaction with the target site and achieve controlled, reproducible, and predictable drug release. Various systems are presently being utilized or under examination (Osmałek et al., 2021). The term “mucoadhesion” pertains to when a substance with biological origin is bound to the mucosa surface for a prolonged period due to interfacial forces (Das Neves and Bahia, 2006). The mucoadhesive system possesses the capacity to regulate the rate of drug release from the vaginal canal and prolong its residence time. Bioadhesion has the potential to enhance the level of contact and extend the residence time of dosage forms across different administration routes. These formulations have the potential to include pharmaceutical substances or function in combination with moisturizing agents to serve as a means of managing vaginal dryness (de Araujo Pereira and Bruschi, 2012). A variety of bioadhesive polymers were evaluated to establish a vaginal delivery system. These polymers included sodium alginate, polycarbophil, guar gum and xanthan, Carbopol®, sodium carboxymethylcellulose, hydroxypropyl methylcellulose, and hydroxypropyl cellulose (Veiga et al., 2018). Mucoadhesive vaginal drug delivery systems include gels, tablets, films, emulsion-type systems, and suppositories, but gels are the primary mucoadhesive vaginal drug delivery methods in current usage. Vaginal formulations are characterized by their ease of manufacturing, comfort, and efficacy in establishing intimate contact with the vaginal mucosa. These compounds contain a significant amount of water and exhibit rheological qualities, hence offering hydrating and lubricating effects, particularly in cases of dry vaginal mucosa. The utilization of mucoadhesive polymers has been shown to enhance the duration of contact, reduce formulation loss, and prolong the therapeutic impact (Das Neves and Bahia, 2006; de Araujo Pereira and Bruschi, 2012). Table 5 presents a comprehensive overview of several proprietary vaginal compositions now accessible in the marketplace.
3.6 Transdermal drug delivery product
The skin, which is the largest and most external organ within the human body, covers an approximate surface area of 1.8 square meters and constitutes approximately 20% of the whole body weight of an average individual (Brown and Williams, 2019). The utilization of the skin as a way of drug delivery presents advantages such as sustained release, greater compliance, and reduced rates of adverse effects in comparison to oral and parenteral administration methods. The transdermal delivery method is considered ideal due to its large surface area, minimal potential for enzyme-dependent degradation, and extended duration of application (Akbarian et al., 2022; Dahri et al., 2023). The stratum corneum offers an important challenge in the transdermal administration of active substances due to its role as the initial barrier of the skin. This barrier restricts drug absorption and limits the range of medications that can be effectively given (Al-Japairai et al., 2020). Polymers are of significant importance in the formulation of skin preparations since they act as a matrix for delivering the active ingredient to the desired application site or target organ. This intricate system distinguishes between active and inactive components, achieving the desired result. Polymers possess diverse applications, serving as gelating agents in gel systems, consistency excipients in emulsions and creams, matrix materials in patches, and skin adhesives in transdermal systems. Their diverse range of applications makes them useful in numerous formulations for skin preparations (Valenta and Auner, 2004). Hydrogels, which are derived from hydrophilic polymers, exhibit favorable characteristics for drug delivery purposes owing to their capacity to hold large amounts of water, thus enhancing skin elasticity and moisturization (Viyoch et al., 2005). Hydrogel-based patches offer the capacity to deliver drugs in a controlled manner over a specified duration, rendering them more favorable compared to conventional methods.
Additionally, these systems possess the advantage of being quickly packaged and transported while also enabling specific drug delivery to the intended site (Boriwanwattanarak et al., 2008). The Scopolamine patch, which represents the first marketed transdermal delivery patch, presents a revolutionary approach to preventing the effects of motion sickness (Prausnitz et al., 2012). Transdermal patches are available in various types, such as single-layer, multi-layer, reservoir, matrix, and vapor patches. In a single-layer patch, the adhesive layer contains and releases the drug. The multi-layer patch consists of two distinct layers designed for immediate and controlled drug release, which are effectively separated by a membrane. The reservoir transdermal system includes different layers, including a drug layer and a liquid compartment containing a drug suspension or solution, separated by an adhesive layer. The release rate follows zero-order kinetics (Pastore et al., 2015). The matrix system consists of a partially overlaid adhesive layer and a semi-solid matrix, which includes a drug solution or suspension. The novel-type vapor patch is designed to deliver essential oils continuously for over 6 h. Its primary purposes include decongestion and enhancing sleep quality (Lee et al., 2001). Hydrogels are utilized for transdermal delivery in patches or creams, as they effectively enhance drug permeation by promoting skin hydration.
Moreover, hydrogels are well-suited for topical applications. Furthermore, investigations have been conducted on their potential to improve the stability and efficacy of transdermal delivery systems such as liposomes, micelles, and nanoparticles (Kong et al., 2016). Table 6 presents examples of commercially available transdermal hydrogels for drug delivery.
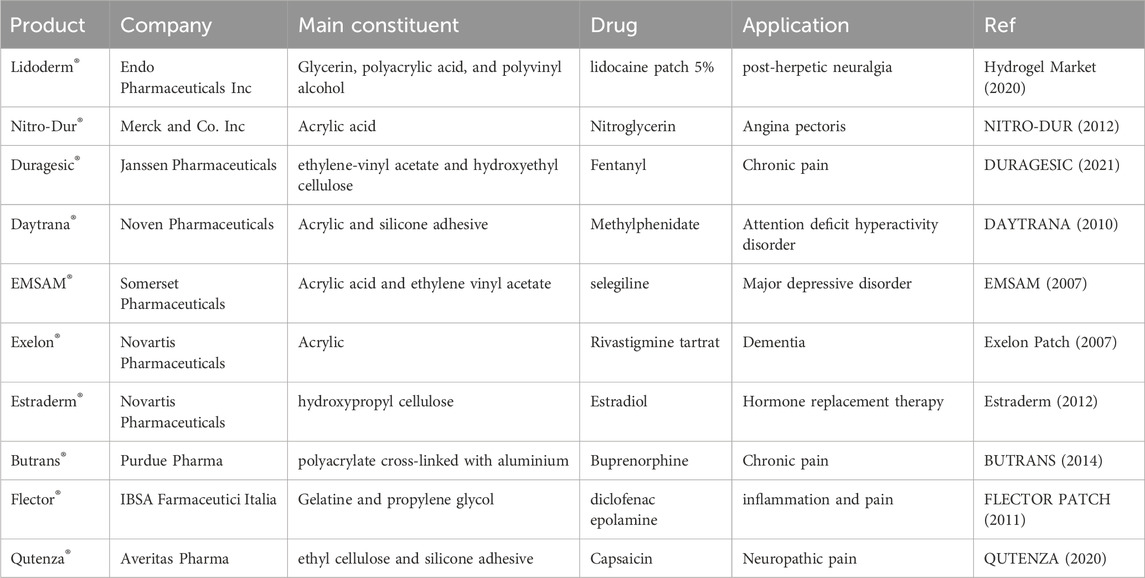
TABLE 6. Some of the transdermal commercial hydrogels [data from the Food and Drug Administration (FDA)].
4 Advantages and disadvantages
Hydrogels provide numerous advantages that make them ideal for biomedical applications, particularly drug delivery. These benefits include sustained action, decreasing administration doses, ease of modification, reduced side effects, drug targeting capabilities to specific locations, and the ability to respond to stimuli. Nevertheless, hydrogels exhibit several disadvantages, including hypoxia, dehydration, as well as limited mechanical strength, challenging manipulability, and high cost.
Oxygen is a vital factor in the existence of cellular organisms. Some hydrogels can induce hypoxia as a state of lack of oxygen. This phenomenon can be considered as a limitation or advantage of hydrogel application in therapy. Hypoxia-induced hydrogels in surrounding tissues can stimulate the invasion of blood vessels and activate hypoxia-regulated pathways to regenerate tissue in neovascularization (Park and Gerecht, 2014). One drawback of hydrogel utilization is dehydration, which can cause stiffening and locking their dynamic behavior. This can be observed in thermos-responsive hydrogel after reaching LCST and can be considered as a limiting factor in their application in therapy. (Zhang et al., 2023). Hydrogels have low mechanical strength due to two characteristics: high solution and low friction between chains (Lin et al., 2022). This problem has arisen by designing composite structures by integrating heterogeneous elements such as silica in the hydrogel structure (Xu et al., 2022). Overall, the advantages of hydrogels exceed their disadvantages in the field of drug delivery. Considering positive factors, hydrogel commercial administration in drug delivery will be vast.
5 Conclusion and future perspectives
Drug carriers represent revolutionary delivery systems within the science of medicine. Numerous studies have shown various polymers employed in carrier synthesis. Among these, hydrogel-based systems received significant attention due to their cost-effectiveness, ease of production, and remarkable capacity to carry diverse drug types. Utilizing cross-linked polymeric networks for enhancing therapeutic efficacy presents a novel way for drug delivery applications. Still, despite the numerous capabilities and advantages of hydrogels in the field of drug delivery, there remains an essential requirement for further research and development to efficiently and swiftly introduce more hydrogel-based formulations to the market. Novel features of hydrogels will continue to play a crucial function in drug delivery and enable the development of a vast array of drugs, peptides, proteins, and delivery systems.
Commercial hydrogel products for drug delivery based on different routes of administration represent a rapidly growing market with significant potential. Continued research and development in hydrogels hold promising prospects for the future. Advancements in drug delivery and polymer-based carrier systems will facilitate targeted and efficient transportation of therapeutic drugs to specific locations within the body. Continued research, technological innovations, and collaborative efforts can fully realize the potential of hydrogel drug delivery in improving therapeutic outcomes.
Author contributions
AR: Investigation, Writing–original draft. FF: Investigation, Writing–original draft, Conceptualization.
Funding
The author(s) declare that no financial support was received for the research, authorship, and/or publication of this article.
Conflict of interest
The authors declare that the research was conducted in the absence of any commercial or financial relationships that could be construed as a potential conflict of interest.
Publisher’s note
All claims expressed in this article are solely those of the authors and do not necessarily represent those of their affiliated organizations, or those of the publisher, the editors and the reviewers. Any product that may be evaluated in this article, or claim that may be made by its manufacturer, is not guaranteed or endorsed by the publisher.
References
Acarturk, F. (2009). Mucoadhesive vaginal drug delivery systems. Recent Pat. drug Deliv. formulation 3 (3), 193–205. doi:10.2174/187221109789105658
Ahmad, S., Ahmad, M., Manzoor, K., Purwar, R., and Ikram, S. (2019). A review on latest innovations in natural gums based hydrogels: preparations and applications. Int. J. Biol. Macromol. 136, 870–890. doi:10.1016/j.ijbiomac.2019.06.113
Ahmadi, S., Rabiee, N., Bagherzadeh, M., Elmi, F., Fatahi, Y., Farjadian, F., et al. (2020). Stimulus-responsive sequential release systems for drug and gene delivery. Nano today 34, 100914. doi:10.1016/j.nantod.2020.100914
Aka-Any-Grah, A., Bouchemal, K., Koffi, A., Agnely, F., Zhang, M., Djabourov, M., et al. (2010). Formulation of mucoadhesive vaginal hydrogels insensitive to dilution with vaginal fluids. Eur. J. Pharm. Biopharm. 76 (2), 296–303. doi:10.1016/j.ejpb.2010.07.004
Akbarian, M., Chen, S. H., Kianpour, M., Farjadian, F., Tayebi, L., and Uversky, V. N. (2022). A review on biofilms and the currently available antibiofilm approaches: matrix-destabilizing hydrolases and anti-bacterial peptides as promising candidates for the food industries. Int. J. Biol. Macromol. 219, 1163–1179. doi:10.1016/j.ijbiomac.2022.08.192
Alexander, N. J., Baker, E., Kaptein, M., Karck, U., Miller, L., and Zampaglione, E. (2004). Why consider vaginal drug administration? Fertil. Steril. 82 (1), 1–12. doi:10.1016/j.fertnstert.2004.01.025
Al-Japairai, K. A. S., Mahmood, S., Hamed Almurisi, S., Reddy Venugopal, J., Rebhi Hilles, A., Azmana, M., et al. (2020). Current trends in polymer microneedle for transdermal drug delivery. Int. J. Pharm. 587, 119673. doi:10.1016/j.ijpharm.2020.119673
Al-Kinani, A. A., Zidan, G., Elsaid, N., Seyfoddin, A., Alani, A. W., and Alany, R. G. (2018). Ophthalmic gels: past, present and future. Adv. Drug Deliv. Rev. 126, 113–126. doi:10.1016/j.addr.2017.12.017
Aswathy, S., Narendrakumar, U., and Manjubala, I. (2020). Commercial hydrogels for biomedical applications. Heliyon 6 (4), e03719. doi:10.1016/j.heliyon.2020.e03719
Bahmani, M., Akbarian, M., Tayebi, L., and Farjadian, F. (2022). The inhibitory effect of curcumin loaded poly (vinyl caprolactam) nanohydrogel on insulin fibrillation. Process Biochem. 117, 209–218. doi:10.1016/j.procbio.2022.04.009
Bahmanpour, A., Mollazadeh-Bajestani, M., Moztarzadeh, F., and Sepahvandi, A. (2023). Hydrogel-based formulations for drug delivery to the posterior segment of the eye. Eye 7, 038–050. doi:10.29328/journal.abse.1001024
Boriwanwattanarak, P., Ingkaninan, K., Khorana, N., and Viyoch, J. (2008). Development of curcuminoids hydrogel patch using chitosan from various sources as controlled-release matrix. Int. J. Cosmet. Sci. 30 (3), 205–218. doi:10.1111/j.1468-2494.2008.00437.x
Brache, V., Croxatto, H., Kumar, N., Sitruk-Ware, R., Cochón, L., Schiappacasse, V., et al. (2009). Effect of sexual intercourse on the absorption of levonorgestrel after vaginal administration of 0.75 mg in Carraguard® gel: a randomized, cross-over, pharmacokinetic study. Contraception 79 (2), 150–154. doi:10.1016/j.contraception.2008.08.010
Brown, M. B., and Williams, A. C. (2019). The art and science of dermal formulation development. Florida, United States: CRC Press.
BUTRANS (2014). BUTRANS (buprenorphine) for transdermal administration. Available from: https://www.accessdata.fda.gov/drugsatfda_docs/label/2014/021306s015s019lbl.pdf.
Caló, E., and Khutoryanskiy, V. V. (2015). Biomedical applications of hydrogels: a review of patents and commercial products. Eur. Polym. J. 65, 252–267. doi:10.1016/j.eurpolymj.2014.11.024
Carr, D. A., Gómez-Burgaz, M., Boudes, M. C., and Peppas, N. A. (2010). Complexation hydrogels for the oral delivery of growth hormone and salmon calcitonin. Industrial Eng. Chem. Res. 49 (23), 11991–11995. doi:10.1021/ie1008025
Cascone, S., and Lamberti, G. (2020). Hydrogel-based commercial products for biomedical applications: a review. Int. J. Pharm. 573, 118803. doi:10.1016/j.ijpharm.2019.118803
Chaturvedi, K., Ganguly, K., Nadagouda, M. N., and Aminabhavi, T. M. (2013). Polymeric hydrogels for oral insulin delivery. J. Control. Release 165 (2), 129–138. doi:10.1016/j.jconrel.2012.11.005
Chowhan, A., and Giri, T. K. (2020). Polysaccharide as renewable responsive biopolymer for in situ gel in the delivery of drug through ocular route. Int. J. Biol. Macromol. 150, 559–572. doi:10.1016/j.ijbiomac.2020.02.097
Cooper, R. C., and Yang, H. (2019). Hydrogel-based ocular drug delivery systems: emerging fabrication strategies, applications, and bench-to-bedside manufacturing considerations. J. Control. Release 306, 29–39. doi:10.1016/j.jconrel.2019.05.034
Coskun, M., Tutkunkardas, M. D., and Zoroglu, S. (2009). OROS methylphenidate-induced skin eruptions. J. Child Adolesc. Psychopharmacol. 19 (5), 593–594. doi:10.1089/cap.2009.0009
Dahri, M., Beheshtizadeh, N., Seyedpour, N., Nakhostin-Ansari, A., Aghajani, F., Seyedpour, S., et al. (2023). Biomaterial-based delivery platforms for transdermal immunotherapy. Biomed. Pharmacother. 165, 115048. doi:10.1016/j.biopha.2023.115048
Das Neves, J., and Bahia, M. F. (2006). Gels as vaginal drug delivery systems. Int. J. Pharm. 318 (1-2), 1–14. doi:10.1016/j.ijpharm.2006.03.012
DAYTRANA (2010). DAYTRANA (methylphenidate transdermal system). Available from: https://www.accessdata.fda.gov/drugsatfda_docs/label/2010/021514s009s010lbl.pdf.
de Araujo Pereira, R. R., and Bruschi, M. L. (2012). Vaginal mucoadhesive drug delivery systems. Drug Dev. industrial Pharm. 38 (6), 643–652. doi:10.3109/03639045.2011.623355
Douglas, J. F. (2018). Weak and strong gels and the emergence of the amorphous solid state. Gels 4 (1), 19. doi:10.3390/gels4010019
DURAGESIC (2021). DURAGESIC (fentanyl transdermal). Available from: https://www.accessdata.fda.gov/drugsatfda_docs/label/2021/019813s081lbl.pdf.
EMSAM (2007). EMSAM(SELEGILINE TRANSDERMAL SYSTEM). Available from: https://www.accessdata.fda.gov/drugsatfda_docs/label/2007/021336s002lbl.pdf.
Entezar-Almahdi, E., Heidari, R., Ghasemi, S., Mohammadi-Samani, S., and Farjadian, F. (2021). Integrin receptor mediated pH-responsive nano-hydrogel based on histidine-modified poly (aminoethyl methacrylamide) as targeted cisplatin delivery system. J. Drug Deliv. Sci. Technol. 62, 102402. doi:10.1016/j.jddst.2021.102402
Entezar-Almahdi, E., Mohammadi-Samani, S., Tayebi, L., and Farjadian, F. (2020). Recent advances in designing 5-fluorouracil delivery systems: a stepping stone in the safe treatment of colorectal cancer. Int. J. nanomedicine 15, 5445–5458. doi:10.2147/ijn.s257700
Erol, O., Pantula, A., Liu, W., and Gracias, D. H. (2019). Transformer hydrogels: a review. Adv. Mater. Technol. 4 (4), 1900043. doi:10.1002/admt.201900043
Estraderm (2012). Estraderm(estradiol transdermal system). Available from: https://www.accessdata.fda.gov/drugsatfda_docs/label/2012/019081s042lbl.pdf.
Exelon Patch (2007). Exelon Patch (rivastigmine transdermal system). Available from: https://www.accessdata.fda.gov/drugsatfda_docs/label/2007/022083lbl.pdf.
Fang, G., Yang, X., Wang, Q., Zhang, A., and Tang, B. (2021). Hydrogels-based ophthalmic drug delivery systems for treatment of ocular diseases. Mater. Sci. Eng. C 127, 112212. doi:10.1016/j.msec.2021.112212
Farjadian, F., Akbarian, M., Hoseini-Ghahfarokhi, M., Moghoofei, M., and Doroudian, M. (2022). Physically stimulus-responsive nanoparticles for therapy and diagnosis. Front. Chem. 10, 952675. doi:10.3389/fchem.2022.952675
Farjadian, F., Mohseni, F., Raeisi, A., Sabet, R., and Daneshamouz, S. (2023). Smart stimuli responsive hydrogels; fascinating platform for therapeutic applications. J. Mazandaran Univ. Med. Sci. 33 (228), 161–190.
Farjadian, F., Ghasemi, A., Gohari, O., Roointan, A., Karimi, M., and Hamblin, M. R. (2019b). Nanopharmaceuticals and nanomedicines currently on the market: challenges and opportunities. Nanomedicine 14 (1), 93–126. doi:10.2217/nnm-2018-0120
Farjadian, F., Ghasemi, S., Andami, Z., and Tamami, B. (2020). Thermo-responsive nanocarrier based on poly (N-isopropylacrylamide) serving as a smart doxorubicin delivery system. Iran. Polym. J. 29, 197–207. doi:10.1007/s13726-020-00785-w
Farjadian, F., Rezaeifard, S., Naeimi, M., Ghasemi, S., Mohammadi-Samani, S., Welland, M. E., et al. (2019a). Temperature and pH-responsive nano-hydrogel drug delivery system based on lysine-modified poly (vinylcaprolactam). Int. J. nanomedicine 14, 6901–6915. doi:10.2147/ijn.s214467
Farjadian, F., Roointan, A., Mohammadi-Samani, S., and Hosseini, M. (2019c). Mesoporous silica nanoparticles: synthesis, pharmaceutical applications, biodistribution, and biosafety assessment. Chem. Eng. J. 359, 684–705. doi:10.1016/j.cej.2018.11.156
Farrukh, F., Zaman, M., and Kashif Rehman, M. U. (2023). Pharmaceutical and biomedical applications of hydrogels.
Farzanfar, J., Farjadian, F., Roointan, A., Mohammadi-Samani, S., and Tayebi, L. (2021). Assessment of pH responsive delivery of methotrexate based on PHEMA-st-PEG-DA nanohydrogels. Macromol. Res. 29, 54–61. doi:10.1007/s13233-021-9007-6
Fathi, M., Barar, J., Aghanejad, A., and Omidi, Y. (2015). Hydrogels for ocular drug delivery and tissue engineering. BioImpacts BI 5 (4), 159–164. doi:10.15171/bi.2015.31
FLECTOR PATCH (2011). FLECTOR PATCH (diclofenac epolamine). Available from: https://www.accessdata.fda.gov/drugsatfda_docs/label/2011/021234s005lbl.pdf.
Ghasemi, S., Ahmadi, L., and Farjadian, F. (2022a). Thermo-responsive PNIPAAm-b-PLA amphiphilic block copolymer micelle as nanoplatform for docetaxel drug release. J. Mater. Sci. 57 (36), 17433–17447. doi:10.1007/s10853-022-07711-w
Ghasemi, S., Owrang, M., Javaheri, F., and Farjadian, F. (2022b). Spermine modified PNIPAAm nano-hydrogel serving as thermo-responsive system for delivery of cisplatin. Macromol. Res. 30 (5), 314–324. doi:10.1007/s13233-022-0035-7
Gok, B., McGirt, M. J., Sciubba, D. M., Garces-Ambrossi, G., Nelson, C., Noggle, J., et al. (2009). ADJUVANT TREATMENT WITH LOCALLY DELIVERED ONCOGEL DELAYS THE ONSET OF PARESIS AFTER SURGICAL RESECTION OF EXPERIMENTAL SPINAL COLUMN METASTASIS. Neurosurgery 65 (1), 193–200. doi:10.1227/01.neu.0000345948.54008.82
Gull, N., Khan, S. M., Butt, M. T. Z., Zia, S., Khalid, S., Islam, A., et al. (2019a). Hybrid cross-linked hydrogels as a technology platform for in vitro release of cephradine. Polym. Adv. Technol. 30 (9), 2414–2424. doi:10.1002/pat.4688
Gull, N., Khan, S. M., Butt, O. M., Islam, A., Shah, A., Jabeen, S., et al. (2020). Inflammation targeted chitosan-based hydrogel for controlled release of diclofenac sodium. Int. J. Biol. Macromol. 162, 175–187. doi:10.1016/j.ijbiomac.2020.06.133
Gull, N., Khan, S. M., Zahid Butt, M. T., Khalid, S., Shafiq, M., Islam, A., et al. (2019b). In vitro study of chitosan-based multi-responsive hydrogels as drug release vehicles: a preclinical study. RSC Adv. 9 (53), 31078–31091. doi:10.1039/c9ra05025f
Guo, J., Feng, Z., Liu, X., Wang, C., Huang, P., Zhang, J., et al. (2020). An injectable thermosensitive hydrogel self-supported by nanoparticles of PEGylated amino-modified PCL for enhanced local tumor chemotherapy. Soft Matter 16 (24), 5750–5758. doi:10.1039/d0sm00147c
Han, D., Lu, Z., Chester, S. A., and Lee, H. (2018). Micro 3D printing of a temperature-responsive hydrogel using projection micro-stereolithography. Sci. Rep. 8 (1), 1963. doi:10.1038/s41598-018-20385-2
Hejabi, F., Abbaszadeh, M. S., Taji, S., O’Neill, A., Farjadian, F., and Doroudian, M. (2022). Nanocarriers: a novel strategy for the delivery of CRISPR/Cas systems. Front. Chem. 10, 957572. doi:10.3389/fchem.2022.957572
Hoare, T. R., and Kohane, D. S. (2008). Hydrogels in drug delivery: progress and challenges. Polymer 49 (8), 1993–2007. doi:10.1016/j.polymer.2008.01.027
Hobzova, R., Hampejsova, Z., Cerna, T., Hrabeta, J., Venclikova, K., Jedelska, J., et al. (2019). Poly (d, l-lactide)/polyethylene glycol micro/nanofiber mats as paclitaxel-eluting carriers: preparation and characterization of fibers, in vitro drug release, antiangiogenic activity and tumor recurrence prevention. Mater. Sci. Eng. C 98, 982–993. doi:10.1016/j.msec.2019.01.046
Holback, H., Yeo, Y., and Park, K. (2011). “Hydrogel swelling behavior and its biomedical applications,” in Biomedical hydrogels (Amsterdam, Netherlands: Elsevier), 3–24.
Homayun, B., Lin, X., and Choi, H.-J. (2019). Challenges and recent progress in oral drug delivery systems for biopharmaceuticals. Pharmaceutics 11 (3), 129. doi:10.3390/pharmaceutics11030129
Hoseini-Ghahfarokhi, M., Mirkiani, S., Mozaffari, N., Abdolahi Sadatlu, M. A., Ghasemi, A., Abbaspour, S., et al. (2020). Applications of graphene and graphene oxide in smart drug/gene delivery: is the world still flat? Int. J. Nanomedicine 15, 9469–9496. doi:10.2147/ijn.s265876
Hu, S., Pei, X., Duan, L., Zhu, Z., Liu, Y., Chen, J., et al. (2021). A mussel-inspired film for adhesion to wet buccal tissue and efficient buccal drug delivery. Nat. Commun. 12 (1), 1689. doi:10.1038/s41467-021-21989-5
Huang, X., and Brazel, C. S. (2001). On the importance and mechanisms of burst release in matrix-controlled drug delivery systems. J. Control. release 73 (2-3), 121–136. doi:10.1016/s0168-3659(01)00248-6
Hydrogel Market (2020). Hydrogel market outlook-2027. Available from: https://www.alliedmarketresearch.com/hydrogel-market.
Jacob, S., Nair, A. B., Shah, J., Sreeharsha, N., Gupta, S., and Shinu, P. (2021). Emerging role of hydrogels in drug delivery systems, tissue engineering and wound management. Pharmaceutics 13 (3), 357. doi:10.3390/pharmaceutics13030357
Jain, A., Kunduru, K. R., Basu, A., Mizrahi, B., Domb, A. J., and Khan, W. (2016). Injectable formulations of poly (lactic acid) and its copolymers in clinical use. Adv. drug Deliv. Rev. 107, 213–227. doi:10.1016/j.addr.2016.07.002
Jindal, S., Awasthi, R., Goyal, K., and Kulkarni, G. T. (2022). Hydrogels for localized drug delivery: a special emphasis on dermatologic applications. Dermatol. Ther. 35 (11), e15830. doi:10.1111/dth.15830
Kalhori, R. P., Ehsani, S., Daneshgar, F., Ashtarian, H., and Rezaei, M. (2016). Different nursing care methods for prevention of keratopathy among intensive care unit patients. Glob. J. health Sci. 8 (7), 212. doi:10.5539/gjhs.v8n7p212
Katsuno, C., Konda, A., Urayama, K., Takigawa, T., Kidowaki, M., and Ito, K. (2013). Pressure-responsive polymer membranes of slide-ring gels with movable cross-links. Adv. Mater. 25 (33), 4636–4640. doi:10.1002/adma.201301252
Kesharwani, P., Bisht, A., Alexander, A., Dave, V., and Sharma, S. (2021). Biomedical applications of hydrogels in drug delivery system: an update. J. Drug Deliv. Sci. Technol. 66, 102914. doi:10.1016/j.jddst.2021.102914
Kong, B. J., Kim, A., and Park, S. N. (2016). Properties and in vitro drug release of hyaluronic acid-hydroxyethyl cellulose hydrogels for transdermal delivery of isoliquiritigenin. Carbohydr. Polym. 147, 473–481. doi:10.1016/j.carbpol.2016.04.021
Kropp, M., Morawa, K. M., Mihov, G., Salz, A., Harmening, N., Franken, A., et al. (2014). Biocompatibility of poly (ester amide)(PEA) microfibrils in ocular tissues. Polymers 6 (1), 243–260. doi:10.3390/polym6010243
Lee, W.-R., Shen, S. C., Lai, H. H., Hu, C. H., and Fang, J. Y. (2001). Transdermal drug delivery enhanced and controlled by erbium: YAG laser: a comparative study of lipophilic and hydrophilic drugs. J. Control. release 75 (1-2), 155–166. doi:10.1016/s0168-3659(01)00391-1
Li, J., and Mooney, D. J. (2016). Designing hydrogels for controlled drug delivery. Nat. Rev. Mater. 1 (12), 16071. doi:10.1038/natrevmats.2016.71
Lin, X., Zhao, X., Xu, C., Wang, L., and Xia, Y. (2022). Progress in the mechanical enhancement of hydrogels: fabrication strategies and underlying mechanisms. J. Polym. Sci. 60 (17), 2525–2542. doi:10.1002/pol.20220154
Mahinroosta, M., Jomeh Farsangi, Z., Allahverdi, A., and Shakoori, Z. (2018). Hydrogels as intelligent materials: a brief review of synthesis, properties and applications. Mater. Today Chem. 8, 42–55. doi:10.1016/j.mtchem.2018.02.004
Malpure, P. S., Patil, S. S., More, Y. M., and Nikam, P. P. (2018). A review on-hydrogel. Am. J. PharmTech Res. 8 (3), 41–61. doi:10.46624/ajptr.2018.v8.i3.005
Mathew, A. P., Uthaman, S., Cho, K. H., Cho, C. S., and Park, I. K. (2018). Injectable hydrogels for delivering biotherapeutic molecules. Int. J. Biol. Macromol. 110, 17–29. doi:10.1016/j.ijbiomac.2017.11.113
Mishra, B., and Singh, J. (2021). “Hydrogel-based drug delivery systems for cancer therapy,” in Advanced drug delivery systems in the management of cancer (Amsterdam, Netherlands: Elsevier), 63–74.
Mishra, D., Gade, S., Glover, K., Sheshala, R., and Singh, T. R. R. (2023). Vitreous humor: composition, characteristics and implication on intravitreal drug delivery. Curr. eye Res. 48 (2), 208–218. doi:10.1080/02713683.2022.2119254
MohammadSadeghi, A., Farjadian, F., and Alipour, S. (2021). Sustained release of linezolid in ocular insert based on lipophilic modified structure of sodium alginate. Iran. J. basic Med. Sci. 24 (3), 331–340. doi:10.22038/ijbms.2021.49866.11385
Mohite, P. B., and Adhav, S. (2017). A hydrogels: methods of preparation and applications. Int. J. Adv. Pharm. 6 (3), 79–85. doi:10.7439/ijap.v6i3.3972
Morteza, B., Naimeh, M., and Mehdi, M. (2016). “An introduction to hydrogels and some recent applications,” in Emerging concepts in analysis and applications of hydrogels. Editor M. Sutapa Biswas (Rijeka: IntechOpen). Ch. 2.
Mu, X., Tobyn, M. J., and Staniforth, J. N. (2003). Development and evaluation of bio-dissolution systems capable of detecting the food effect on a polysaccharide-based matrix system. J. Control. release 93 (3), 309–318. doi:10.1016/j.jconrel.2003.08.013
Nagai, T., and Machida, Y. (1993). Buccal delivery systems using hydrogels. Adv. Drug Deliv. Rev. 11 (1), 179–191. doi:10.1016/0169-409x(93)90032-y
Narayanaswamy, R., and Torchilin, V. P. (2020). Hydrogels and their applications in targeted drug delivery. Road Nanomedicine Precis. Med., 1117–1150. doi:10.1201/9781003027058-18
NITRO-DUR (2012). NITRO-DUR(nitroglycerin). Available from: https://www.accessdata.fda.gov/drugsatfda_docs/label/2014/020145s027lbl.pdf.
Nyamweya, N. N. (2021). Applications of polymer blends in drug delivery. Future J. Pharm. Sci. 7, 18–15. doi:10.1186/s43094-020-00167-2
Organization, W. H. (2016). Quality of medicines: quality of misoprostol products. WHO Drug Inf. 30 (1), 35–39.
Osmałek, T., Froelich, A., Jadach, B., Tatarek, A., Gadziński, P., Falana, A., et al. (2021). Recent advances in polymer-based vaginal drug delivery systems. Pharmaceutics 13 (6), 884. doi:10.3390/pharmaceutics13060884
Park, K. M., and Gerecht, S. (2014). Hypoxia-inducible hydrogels. Nat. Commun. 5 (1), 4075. doi:10.1038/ncomms5075
Pastore, M. N., Kalia, Y. N., Horstmann, M., and Roberts, M. S. (2015). Transdermal patches: history, development and pharmacology. Br. J. Pharmacol. 172 (9), 2179–2209. doi:10.1111/bph.13059
Peppas, N. A., Bures, P., Leobandung, W. S., and Ichikawa, H. (2000). Hydrogels in pharmaceutical formulations. Eur. J. Pharm. Biopharm. 50 (1), 27–46. doi:10.1016/S0939-6411(00)00090-4
Peppas, N. A., Hilt, J., Khademhosseini, A., and Langer, R. (2006). Hydrogels in biology and medicine: from molecular principles to bionanotechnology. Adv. Mater. 18 (11), 1345–1360. doi:10.1002/adma.200501612
Peppas, N. A., and Sahlin, J. J. (1996). Hydrogels as mucoadhesive and bioadhesive materials: a review. Biomaterials 17 (16), 1553–1561. doi:10.1016/0142-9612(95)00307-x
Perez-Marrero, R., and Tyler, R. C. (2004). A subcutaneous delivery system for the extended release of leuprolide acetate for the treatment of prostate cancer. Expert Opin. Pharmacother. 5, 447–457. (Print)). doi:10.1517/14656566.5.2.447
Prausnitz, M. R., Peter, M. E., Thomas, J. F., Matthias, S., Jui-Chen, T., Gopinathan, K., et al. (2012). Skin barrier and transdermal drug delivery. Dermatology 3, 2065–2073.
QUTENZA (2020). QUTENZA(capsaicin). Available from: https://www.accessdata.fda.gov/drugsatfda_docs/label/2020/022395s019lbl.pdf.
Raymond, E. G., Lien Chen, P., and Luoto, J. (2004). Contraceptive effectiveness and safety of five nonoxynol-9 spermicides: a randomized trial. Obstetrics Gynecol. 103 (3), 430–439. doi:10.1097/01.aog.0000113620.18395.0b
Rizzo, F., and Kehr, N. S. (2021). Recent advances in injectable hydrogels for controlled and local drug delivery. Adv. Healthc. Mater. 10 (1), 2001341. doi:10.1002/adhm.202001341
Roointan, A., Farzanfar, J., Mohammadi-Samani, S., Behzad-Behbahani, A., and Farjadian, F. (2018). Smart pH responsive drug delivery system based on poly (HEMA-co-DMAEMA) nanohydrogel. Int. J. Pharm. 552 (1-2), 301–311. doi:10.1016/j.ijpharm.2018.10.001
Sánchez-López, E., Espina, M., Doktorovova, S., Souto, E., and García, M. (2017). Lipid nanoparticles (SLN, NLC): overcoming the anatomical and physiological barriers of the eye–Part II-Ocular drug-loaded lipid nanoparticles. Eur. J. Pharm. Biopharm. 110, 58–69. doi:10.1016/j.ejpb.2016.10.013
Schulz, K., Oberdieck, U., Backensfeld, T., and Weitschies, W. (2013). Changes in recovery due to drug product matrix ageing as a source of mass imbalances. J. Pharm. Biomed. analysis 74, 117–125. doi:10.1016/j.jpba.2012.10.014
Shah, H. P., Prajapati, S. T., and Patel, C. (2017). Gastroretentive drug delivery systems: from conception to commercial success. J. Crit. Rev. 4 (2), 10. doi:10.22159/jcr.2017v4i2.16717
Sharpe, L. A., Daily, A. M., Horava, S. D., and Peppas, N. A. (2014a). Therapeutic applications of hydrogels in oral drug delivery. Expert Opin. drug Deliv. 11 (6), 901–915. doi:10.1517/17425247.2014.902047
Sharpe, L. A., Daily, A. M., Horava, S. D., and Peppas, N. A. (2014b). Therapeutic applications of hydrogels in oral drug delivery. Expert Opin. Drug Deliv. 11, 901–915. (Electronic)). doi:10.1517/17425247.2014.902047
Shi, Y., and Li, L. (2005). Current advances in sustained-release systems for parenteral drug delivery. Expert Opin. drug Deliv. 2 (6), 1039–1058. doi:10.1517/17425247.2.6.1039
Spierer, O., and O’Brien, T. P. (2015). Endothelial keratoplasty combined with cataract surgery or alone using polyethylene glycol hydrogel sealant for closure of corneal incisions. J. Cataract Refract. Surg. 41 (3), 492–496. doi:10.1016/j.jcrs.2015.02.002
Sudhakar, Y., Kuotsu, K., and Bandyopadhyay, A. (2006). Buccal bioadhesive drug delivery—a promising option for orally less efficient drugs. J. Control. release 114 (1), 15–40. doi:10.1016/j.jconrel.2006.04.012
Tan, H., and Marra, K. G. (2010). Injectable, biodegradable hydrogels for tissue engineering applications. Materials 3 (3), 1746–1767. doi:10.3390/ma3031746
Tanpichai, S., Phoothong, F., and Boonmahitthisud, A. (2022). Superabsorbent cellulose-based hydrogels cross-liked with borax. Sci. Rep. 12 (1), 8920. doi:10.1038/s41598-022-12688-2
Tomczak, M., Chomicka, A., and Dmitriew, A. (2021). Zespół suchego oka w okresie pooperacyjnym po zabiegach laserowej korekcji wzroku. OphthaTherapy 8 (1), 66–70. doi:10.24292/01.ot.310321.s
Torres-Luna, C., Fan, X., Domszy, R., Hu, N., Wang, N. S., and Yang, A. (2020). Hydrogel-based ocular drug delivery systems for hydrophobic drugs. Eur. J. Pharm. Sci. 154, 105503. doi:10.1016/j.ejps.2020.105503
Ullah, F., Othman, M. B. H., Javed, F., Ahmad, Z., and Akil, H. M. (2015). Classification, processing and application of hydrogels: a review. Mater. Sci. Eng. C 57, 414–433. doi:10.1016/j.msec.2015.07.053
Valenta, C. (2005). The use of mucoadhesive polymers in vaginal delivery. Adv. Drug Deliv. Rev. 57, 1692–1712. (0169-409X (Print)). doi:10.1016/j.addr.2005.07.004
Valenta, C., and Auner, B. G. (2004). The use of polymers for dermal and transdermal delivery. Eur. J. Pharm. Biopharm. 58 (2), 279–289. doi:10.1016/j.ejpb.2004.02.017
van Bemmelen, J. M. (1894). Das Hydrogel und das krystallinische Hydrat des Kupferoxyds. Z. für Anorg. Chem. 5 (1), 466–483. doi:10.1002/zaac.18940050156
Vashist, A., Gupta, Y. K., and Ahmad, S. (2014). Recent advances in hydrogel based drug delivery systems for the human body. J. Mater. Chem. B 2 (2), 147–166. doi:10.1039/c3tb21016b
Veiga, M.-D., Roberto, R.-C., Araceli, M.-I., Fernando, N.-P., and Raúl, C.-L. (2018). Polymer gels in vaginal drug delivery systems. Polym. Gels Synthesis Charact., 197–246.
Viyoch, J., Sudedmark, T., Srema, W., and Suwongkrua, W. (2005). Development of hydrogel patch for controlled release of alpha-hydroxy acid contained in tamarind fruit pulp extract. Int. J. Cosmet. Sci. 27 (2), 89–99. doi:10.1111/j.1467-2494.2004.00250.x
Volpi, M., Paradiso, A., Costantini, M., and Świȩszkowski, W. (2022). Hydrogel-based fiber biofabrication techniques for skeletal muscle tissue engineering. ACS Biomaterials Sci. Eng. 8 (2), 379–405. doi:10.1021/acsbiomaterials.1c01145
Wanasathop, A., Patel, P. B., Choi, H. A., and Li, S. K. (2021). Permeability of buccal mucosa. Pharmaceutics 13 (11), 1814. doi:10.3390/pharmaceutics13111814
Wasilewska, K., and Winnicka, K. (2019). Ethylcellulose–a pharmaceutical excipient with multidirectional application in drug dosage forms development. Materials 12 (20), 3386. doi:10.3390/ma12203386
Wichterle, O., and Lím, D. (1960). Hydrophilic gels for biological use. Nature 185 (4706), 117–118. doi:10.1038/185117a0
Wu, Y., Liu, Y., Li, X., Kebebe, D., Zhang, B., Ren, J., et al. (2019). Research progress of in-situ gelling ophthalmic drug delivery system. Asian J. Pharm. Sci. 14 (1), 1–15. doi:10.1016/j.ajps.2018.04.008
Xu, P., Shang, Z., Yao, M., and Li, X. (2022). Mechanistic insight into improving strength and stability of hydrogels via nano-silica. J. Mol. Liq. 357, 119094. doi:10.1016/j.molliq.2022.119094
Yu, L., and Ding, J. (2008). Injectable hydrogels as unique biomedical materials. Chem. Soc. Rev. 37 (8), 1473–1481. doi:10.1039/b713009k
Zarkesh, K., Entezar-Almahdi, E., Ghasemiyeh, P., Akbarian, M., Bahmani, M., Roudaki, S., et al. (2021). Drug-based therapeutic strategies for COVID-19-infected patients and their challenges. Future Microbiol. 16 (18), 1415–1451. doi:10.2217/fmb-2021-0116
Zhang, M., Lee, Y., Zheng, Z., Khan, M. T. A., Lyu, X., Byun, J., et al. (2023). Micro- and nanofabrication of dynamic hydrogels with multichannel information. Nat. Commun. 14 (1), 8208. doi:10.1038/s41467-023-43921-9
Zhao, D., Huang, J., Zhong, Y., Li, K., Zhang, L., and Cai, J. (2016). High-strength and high-toughness double-cross-linked cellulose hydrogels: a new strategy using sequential chemical and physical cross-linking. Adv. Funct. Mater. 26 (34), 6279–6287. doi:10.1002/adfm.201601645
Keywords: hydrogel, drug delivery, commercial products, routes of administration, buccal, transdermal, vaginal, oral
Citation: Raeisi A and Farjadian F (2024) Commercial hydrogel product for drug delivery based on route of administration. Front. Chem. 12:1336717. doi: 10.3389/fchem.2024.1336717
Received: 11 November 2023; Accepted: 13 February 2024;
Published: 27 February 2024.
Edited by:
Renyun Zhang, Mid Sweden University, SwedenReviewed by:
Preeti Gupta, Leibniz Institute for Solid State and Materials Research Dresden (IFW Dresden), GermanyNafisa Gull, University of the Punjab, Pakistan
Copyright © 2024 Raeisi and Farjadian. This is an open-access article distributed under the terms of the Creative Commons Attribution License (CC BY). The use, distribution or reproduction in other forums is permitted, provided the original author(s) and the copyright owner(s) are credited and that the original publication in this journal is cited, in accordance with accepted academic practice. No use, distribution or reproduction is permitted which does not comply with these terms.
*Correspondence: Fatemeh Farjadian, ZmFyamFkaWFuX2ZAc3Vtcy5hYy5pcg==