- 1Department of Laser and Photonics, Faculty of Physics, University of Kashan, Kashan, Iran
- 2Institute of Nanoscience and Nanotechnology, University of Kashan, Kashan, Iran
- 3Department of Food Hygiene and Quality Control, Faculty of Veterinary Medicine, Science and Research Branch, Islamic Azad University, Tehran, Iran
- 4Research Center for Biochemistry and Nutrition in Metabolic Diseases, Kashan University of Medical Sciences, Kashan, Iran
- 5Anatomical Sciences Research Center, Institute for Basic Sciences, Kashan University of Medical Sciences, Kashan, Iran
Introduction: Nanoemulsion and nanoencapsulation are attractive novel methods that can be used for incorporating active plant extracts in food preparations and pharmaceutical formulations. In the current study, we aimed to investigate the anticancer and antibacterial effects of hydroethanolic extracts of Nettle (NE), Wormwood (WE), and the combination of the two plants (CNWE), as well as their nanoemulsion forms (NN, NW, CNNW) and nanoencapsulation forms (CN, CW, and CCNW).
Methods: The morphology and structure of the nanoemulsion and nanoencapsulation preparations were assessed utilizing dynamic light scattering (DLS) along with transmission electron microscopy (TEM). The antibacterial activity of the prepared formulations were assessed by determining minimum inhibitory concentration (MIC), zone of inhibition diameter, minimum bactericidal concentration (MBC), along with biofilm growth inhibition against Salmonaella typhimurium and Klebsiella. pneumoniae. The anticancer activity was evaluated via a MTT assay in the colon cancer cell line (HCT116).
Results: The nanoemulsion and nanoencapsulation particle size varied between 10 and 50 nm and 60 and 110 nm, respectively. The MIC values were between 11.25 and 95 µg/mL along with MBC values between 11.25 and 190 µg/mL. The highest inhibition of biofilm formation was observed with CCNW against K. pneumoniae (∼78.5%) and S. typhimurium (∼73%). In descending order, the inhibition of biofilm formation was CCNW > CW > CN > CNNW > NN > NW > CNWE > NE > WE against the tested bacteria. The IC50 values for NE, WE, CNWE, NN, NW, CNNW, CN, CW, and CCNW were determined as 250, 170, 560, 380, 312, 370, 250, 420, and 700 µg/mL, respectively. Exposure to a high concentration of NW resulted in a significantly lower HCT116 viability compared to other groups. Taken together, CNNW, and CCNW showed the highest antibacterial and anticancer activitiy.
Discussion: Nanoemulsion and nanoencapsulation were effective ways to increase the antibacterial and anticancer activity of the extracts and could be used in the food and pharmaceutical industries.
Introduction
There is a long history of using plants to treat diseases, and many traditional medical therapies have their roots in the use of medicinal plants. Today, medicinal plants are attractive for many researchers due to their low side effects, variety of effective ingredients, strong anti-inflammatory and antioxidant activity, and their ability to prevent tumor growth (Chaleshtori et al., 2015; Wu et al., 2023). In this regard, in recent decades, plants have become globally significant sources of new anticancer drugs and antibiotics (Kaur et al., 2023; Shehabeldine et al., 2023).
Nettle (Urtica dioica) is a widespread perennial herbaceous flowering plant found across the globe (Bhusal et al., 2022). This plant, belonging to the Urticaceae family, is a valuable source of minerals, amino acids, carotenoids, vitamins, flavonoids, tannins, sterols, and polysaccharides. It is important as a medicinal plant and is often recommended for inclusion in the human diet (Bhusal et al., 2022; Dağlıoğlu et al., 2023a). Numerous reports have shown the beneficial effects of Nettle (seeds and leaves) in treating various diseases, including diabetes, eczema, hepatitis, anemia, rheumatism, and prostate cancer. Additionally, the plant leaves have been used for treating high blood pressure as well as arthritis (Bhusal et al., 2022; Teixeira et al., 2023).
Wormwood, also known as Artemisia absinthium, belongs to Asteraceae family (Sohail et al., 2023). This plant can easily grow in various climate conditions in regions of Iran, including around Tehran (Damavand), Azerbaijan, Khorasan, and Gilan. It is cultivated and used in traditional Iranian medicine and around the world to treat liver failure, sore throat, ear infection, constipation, chronic diarrhea, wound healing, chronic fever, and can also serve as an antidote to insect bites (Mehrabani et al., 2016; Akram et al., 2023). Alpha and beta thujone are monoterpenes, which are the main components of the Wormwood plant, but are considered to be poisonous. Many pharmacological studies have reported that thujone is toxic, despite its use as a pain reliever and anthelmintic. High consumption of thujone can lead to nervous disorders, and it has been shown to exert its toxic effects via cannabinoid receptors (Pal and Dey, 2023).
Nanoemulsions are attractive formulations due to the small size of the droplets, long-term physical stability (no observable aggregation, bi-phase formation or precipitation) and transparency. They require less surfactant, promote higher absorption of antimicrobial compounds by microorganisms, leading to reduced consumption, which is advantageous. The production of nanoemulsions for microencapsulation and controlling the release of beneficial compounds, such as various drugs, dyes, essences, or vitamins, is an example of the ways nanotechnology is being used in the food industry (Naseema et al., 2021).
The technique of encapsulating aromatic compounds aims to protect them against light, heat, and oxygen, while reducing their volatility. This technique is used in the industry for creating capsules that allow controlled release in the digestive system at a specific time, speed, and location. Nanocapsules can also offer protection against other ingredients in food (English et al., 2023).
An earlier investigation revealed that the antibacterial activity of Nettle is more effective against Gram-negative bacteria like Pseudomonas fragi and Campylobacter jejuni strains, compared to other bacteria like Escherichia coli, Shewanella putrefaciens, Listeria innocua, etc. (Elez Garofulić, 2021). Another investigation revealed that a hot methanolic extract of A. absinthium leaves (HMEL) could actively inhibit the proliferation of breast cancer cells MCF-7, with an IC50 value of 80.96 ± 3.94 μg/mL. HMEL showed a moderate spectrum of antibacterial activity against both Gram-positive and Gram-negative bacteria (Sultan, Zuwaiel et al., 2020).
Nevertheless, so far no studies have been reported on the use of nanoemulsion and nanoencapsulation of Nettle and Wormwood to provide anticancer and antimicrobial activity. Therefore, we aimed to investigate the anticancer and antibacterial activity of NE, WE, and the CNWE hydroethanolic extracts, as well as their nanoemulsion forms (NN, NW, CNNW) and nanoencapsulation forms (CN, CW, and CCNW).
Materials and methods
Plant material and chemicals
The Nettle and Wormwood plants were obtained from an herbal market in Kashan, Iran. Ethanol, Tween 20, Tween 80, tetrazolium bromide solution (MTT), polyethylene glycol, phosphotungstic acid, crystal violet, L-glutamine, starch and chitosan were obtained from Sigma Aldrich Chemical Co. (St. Louis, MO, United States). Dimethyl sulfoxide (DMSO) was provided by Merck, Darmstadt, Germany. Nutrient agar (NA), Mueller Hinton agar (MHA) and broth (MHB) as well as brain heart infusion (BHI) were purchased from HiMedia, Mumbai, India. Fetal bovine serum (FBS) and penicillin/streptomycin were obtained from Hyclone, Logan, UT, United States. Gentamicin (10 µg) was purchased from Padtan Teb Co., Iran. Colon cancer cell line (HCT 116) was purchased from the Pasteur Institute of Iran. S. enterica subsp. enterica serovar typhimurium (ATCC 14028) and K. pneumoniae (ATCC 10031) were obtained from the Department of Clinical Medical Microbiology at Kashan University of Medical Sciences.
Extraction using hydroethanolic solvent
The seeds, bark, and leaves were carefully cleaned to remove any dust, followed by drying the leaves under shade for 2 weeks. The dried leaves were then powdered using a mixer grinder, and the powder was macerated with 80% ethanol to produce the extract (Rafieian, 2010; Bonilla and Sobral, 2016). Briefly, 400 mL of 80% ethanol was combined with 100 g of each powder and allowed to settle at ambient temperature (22°C ± 2°C) for 8 h. The resulting Nettle and Wormwood extracts (NE and WE) were collected, filtered, concentrated, and stored in the refrigerator.
Nanoemulsion and nanoencapsulation preparations of Nettle (NE and CN) and Wormwood (WE and CW)
The nanoemulsion and nanoencapsulation formulations were prepared using Nettle and Wormwood extracts, with Tween 20 and Tween 80 as emulsifiers, polyethylene glycol as an auxiliary solvent, and distilled water. To achieve a stable nanoemulsion, the ratio of emulsifier to extract was varied from 0.1 to 2. The results showed that a ratio of 0.6 produced the most stable emulsion. The preparation of all nanoemulsions was carried out using an ultrasonic wave generator probe (Heshler, Germany) for a duration of 30 min. Chitosan and modified starch (nanostarch) were dissolved in 1% (v/v) acetic acid overnight on a magnetic stirrer (Pouya Electric, Iran) at room temperature, at a ratio of 8.5:1.5% (w/v). The previously created nanoemulsion was added dropwise to this solution to create a nanoencapsulation, which was utilized in the subsequent analysis.
Particle size measurement
Transmission electron microscopy (TEM) analysis was conducted with a JEM-100CXII microscope from Hitachi Co. Ltd., Japan (Souza et al., 2016). To examine the structure of the nanoemulsion and nanoencapsulation, a droplet of each sample was deposited onto a carbon-coated copper wire and subsequently stained with a 2 wt% solution of phosphotungstic acid for 2 min. The stained droplets were allowed to air-dry for 10 min and subsequently examined with TEM at an acceleration voltage of 200 kV. The dynamic light scattering (DLS) method using a Zetasizer Nano ZS, model ZEN3500 from Malvern Instruments, United Kingdom, was employed for particle size analysis of the nanoemulsion and nanoencapsulation, (Souza et al., 2016). Each sample underwent the following preparation and analysis. A 100-fold dilution was prepared by adding 10 µL of sample to 990 µL of deionized water. The resulting mixture was then transferred to a disposable plastic cuvette for analysis. The analysis was conducted in triplicate at 25°C.
Analysis of antimicrobial activity
Two Gram-negative bacteria were employed in this investigation: S. typhimurium (ATCC 14028) and K. pneumoniae (ATCC 10031). Initially, each bacterium was grown in NA for 18 h at 37°C. Next, an inoculum was prepared by adjusting the concentration to 0.5 MacFarland units in sterile saline (∼1 × 108 CFU/mL) (Sharafati-Chaleshtori et al., 2010; Dinkov et al., 2016).
The MIC and MBC were determined by the microdilution method (Ali et al., 2021). A 10% (v/v) DMSO solution diluted in MHB was prepared as the growth media. In each row of a 96-well plate, the final two wells were designated as growth control and sterility control, respectively. Into each well, 5 µL of 0.5 McFarland suspension and 95 µL of MHB, were added. Next 100 µL of serial 2-fold dilutions of NE, WE, CNWE, NN, NW, CNNW, CN, CW, and CCNW were transferred to each well. The 96-well plates were placed in a shaking incubator (Noor Sanat Tajhiz Ferdows, Iran) and incubated for 20 h at 37°C. After that, the wells were checked for turbidity to measure bacterial growth. The plate containing the lowest concentration of NE, WE, CNWE, NN, NW, CNNW, CN, CW, and CCNW that resulted in no turbidity (indicating bacterial growth inhibition) was taken as the MIC. Additionally, the MBC was taken as the lowest concentration at which the MHA showed no discernible growth.
The disc diffusion test was conducted following the guidelines outlined in the CLSI M02-A11 document (CLSI, 2015). Briefly, a prepared inoculum of the mentioned bacteria (∼1 × 108 CFU/mL) was spread onto solid MHA plates. Sterile Whatman paper N.1 discs with a diameter of 6 mm were used to add various concentrations of NE, WE, CNWE, NN, NW, CNNW, CN, CW, and CCNW, which were diluted in 10% (w/v) DMSO and incubated for 24-h at 37°C. A millimeter-scale ruler was used for measuring the width of the inhibition zones (IZ). For this technique, DMSO 10% and gentamicin (10 µg) were used as negative and positive controls, respectively. The equation below was used to calculate the IZ diameter (Muchtaromah et al., 2020):
Antibiofilm activity
The bacterial biofilm formation capacity, as well as the anti-biofilm activity of NE, WE, CNWE, NN, NW, CNNW, CN, CW, and CCNW, were evaluated using a microtiter plate biofilm formation assay (Hassanshahian et al., 2020). To begin with, a bacterial suspension was prepared at a concentration of 106 CFU/mL. This suspension was used to inoculate BHI media at sub-lethal concentrations (0.5, 1, and 2 MICs) of the above preparations. The plates were then incubated for a 24-h at 37°C without agitation, allowing the cells to adhere to each well surface. After incubation, the cells were removed by flipping the plate over and emptying out the contents. Subsequently, the supernatant was carefully aspirated from each well, leaving the intact biofilm behind. The remaining biofilm in each well was stained with 1% crystal violet solution. The wells were twice rinsed with 250 µL of sterile water to remove any remaining crystal violet after 30-min incubation at 37°C. The plates were air-dried at 37°C for 1 h. To dissolve the stained biofilm, 200 µL of ethanol (95%) was added, and the optical density (OD) was measured at 570 nm (OD 570 sample). To minimize background effects, stained wells with BHI medium (without any bacterial cells or samples) were employed as a negative control. Similarly, wells containing only bacterial cells (without any samples) served as a positive control. The biofilm inhibition percentage for each antibacterial agent was determined using the following equation:
MTT assay for measuring anticancer activity
The MTT assay was carried out on HCT 116 cells to determine the anticancer activity of NE, WE, CNWE, NN, NW, CNNW, CN, CW, and CCNW (Zengin et al., 2019). HCT 116 cells were cultivated in a medium containing 10% heat-inactivated FBS, 1% penicillin-streptomycin solution (10,000 units of penicillin and 10 mg of streptomycin in 0.9% NaCl), plus 2 mM L-glutamine. The cells were kept at 37°C in a humidified environment with 5% CO2. The HCT 116 cells were sub-cultured in 75 cm2 cell culture flasks, and the culture medium was replenished every 3 days. To assess the cytotoxic effects of NE, WE, CNWE, NN, NW, CNNW, CN, CW, and CCNW, using the MTT test, several dilutions between 0 and 1,000 μg/mL were evaluated. In 96-well plates, the HCT 116 cells were seeded at a density of 1 × 104 cells per well and incubated until they reached 90%–95% confluency. Each well was treated with 100 μL of medium containing the desired concentrations of NE, WE, CNWE, NN, NW, CNNW, CN, CW, and CCNW, and incubated for 24 h. Then each well received 20 μL of MTT working solution (5 mg/mL), and cells were incubated for another 4 h. Following the incubation period, the culture media was aspirated and 200 μL of DMSO was added to every well. The absorbance was measured with an ELISA reader (Stat Fax 3200, United States) at a wavelength of 570 nm. The equation below was used to calculate the percentage of viable cells:
A linear regression equation was used to determine the IC50 value, which reflects the concentration where the cell viability decreased by 50%. The equation used for this calculation was Y = Mx + C, where Y represents 50 (the desired viability percentage), and the values of M and C were obtained from the viability plot.
Statistical analysis
The values were reported as mean ± standard deviation (SD), and each experiment in this study was carried out in triplicate. Statistical analysis was performed utilizing SPSS software (version 21, Chicago, IL, United States). The least significant differences (LSD) and One-way ANOVA test were employed to identify the statistically significant differences with p-value of 0.05 or less.
Results
The TEM and DLS characterization of the prepared nanoemulsion and nanoencapsulation is shown in Figure 1 and Figure 2. The results obtained from DLS analysis, which provided droplet size measurement, were further confirmed by the TEM images. The TEM micrographs (Figure 1) show droplets with diameters ranging from 10 to 30 nm, 20–40 nm, and 30–50 nm for NN (Nettle nanoemulsion), NW (Wormwood nanoemulsion), and CNNW (combination of Nettle and Wormwood nanoemulsion), respectively. The droplet sizes for CN (nanoencapsulated Nettle), CW (nanoencapsulated Wormwood), and CCNW (combination of nanoencapsulated Nettle and Wormwood) were 60–100 nm, 70–110 nm, and 90–110 nm, respectively.
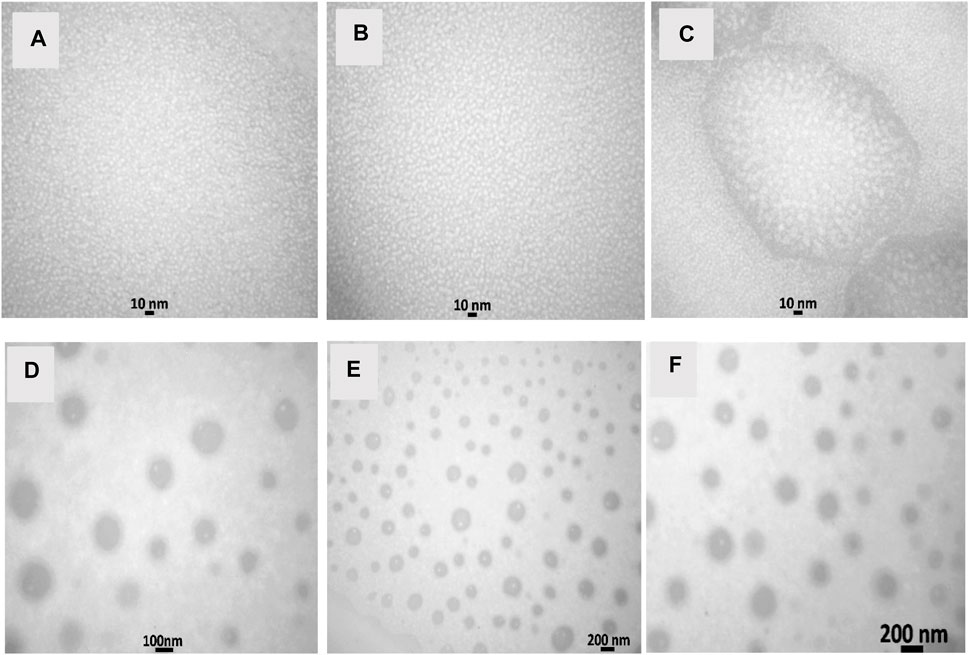
FIGURE 1. Transmission electron micrographs. (A) NN: Nettle nanoemulsion; (B) NW: Wormwood nanoemulsion; (C) CNNW: Combination of Nettle and Wormwood nanoemulsion; (D) CN: nanoencapsulated Nettle; (E) CW: nanoencapsulated Wormwood; (F) CCNW: combination of nanoencapsulated Nettle and Wormwood.
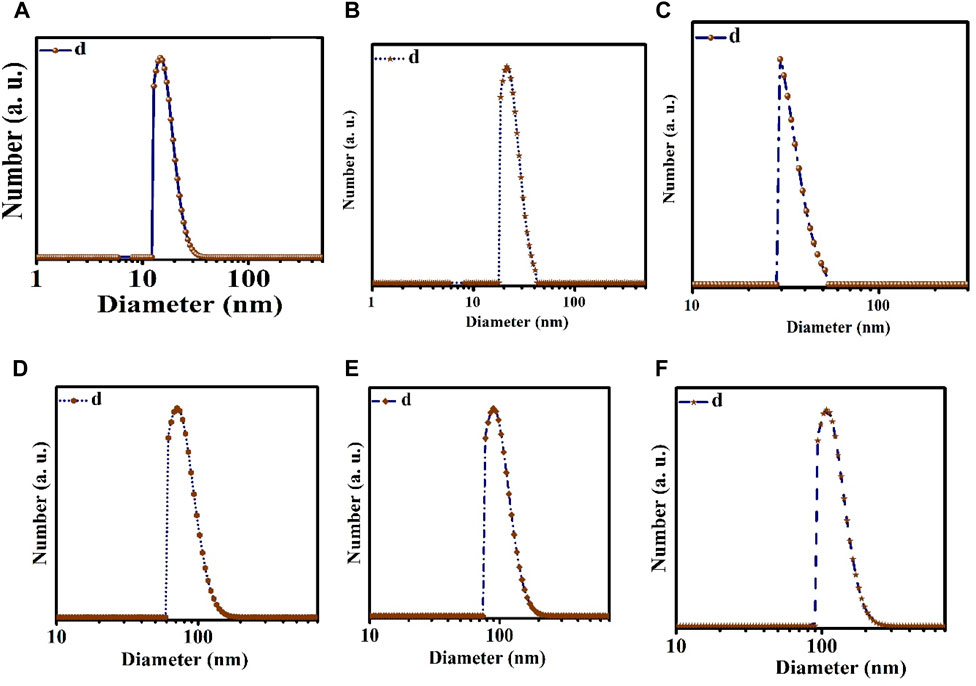
FIGURE 2. Dynamic light scattering (DLS). (A) NN: Nettle nanoemulsion; (B) NW: Wormwood nanoemulsion; (C) CNNW: Combination of Nettle and Wormwood nanoemulsion; (D) CN: nanoencapsulated Nettle; (E) CW: nanoencapsulated Wormwood; (F) CCNW: combination of nanoencapsulated Nettle and Wormwood.
The IZ, MIC, and MBC values for antibacterial activity of NE, WE, CNWE, NN, NW, CNNW, CN, CW, and CCNW are shown in Tables 1, 2. CNNW and CCNW, at high concentrations, showed significantly larger IZ values against the two bacterial strains compared to other treatments (p < 0.05). The investigated bacterial strains were resistant to at least some of the pure herbal extracts. At the concentration 380 μg/mL, S. typhimurium was found to be more susceptible to CCNW and CNNW compared to K. pneumoniae (p < 0.05). In addition, CW, CN, NW, CNNW and CCNW, at 380 and 190 μg/mL concentrations, showed significantly larger IZ values against the two tested bacterial strains compared to gentamicin 10 µg as a control group (p < 0.05). Overall, the MIC and MBC values of NN were generally lower compared to the other treatments. The studied bacteria had MIC values of all agents between 11.25 and 95 μg/mL along with MBC values between 11.25 and 190 μg/mL. The inhibition percentage of biofilm formation is summarized for NE, WE, CNWE, NN, NW, CNNW, CN, CW, and CCNW against S. typhimurium and K. pneumoniae in Figure 1. All of the investigated sub-inhibitory concentrations (0.5 MIC) reduced the biofilm formation of both bacteria. However, all the concentrations of CN, CW, and CCNW showed more noticeable effects on K. pneumoniae and S. typhimurium compared to the other treatments (p < 0.05). At a concentration 2 MIC of all agents, K. pneumoniae was found to be more susceptible to inhibition of biofilm formation compared to S. typhimurium (p < 0.05). The maximum inhibitory effects of CCNW were observed on K. pneumoniae (
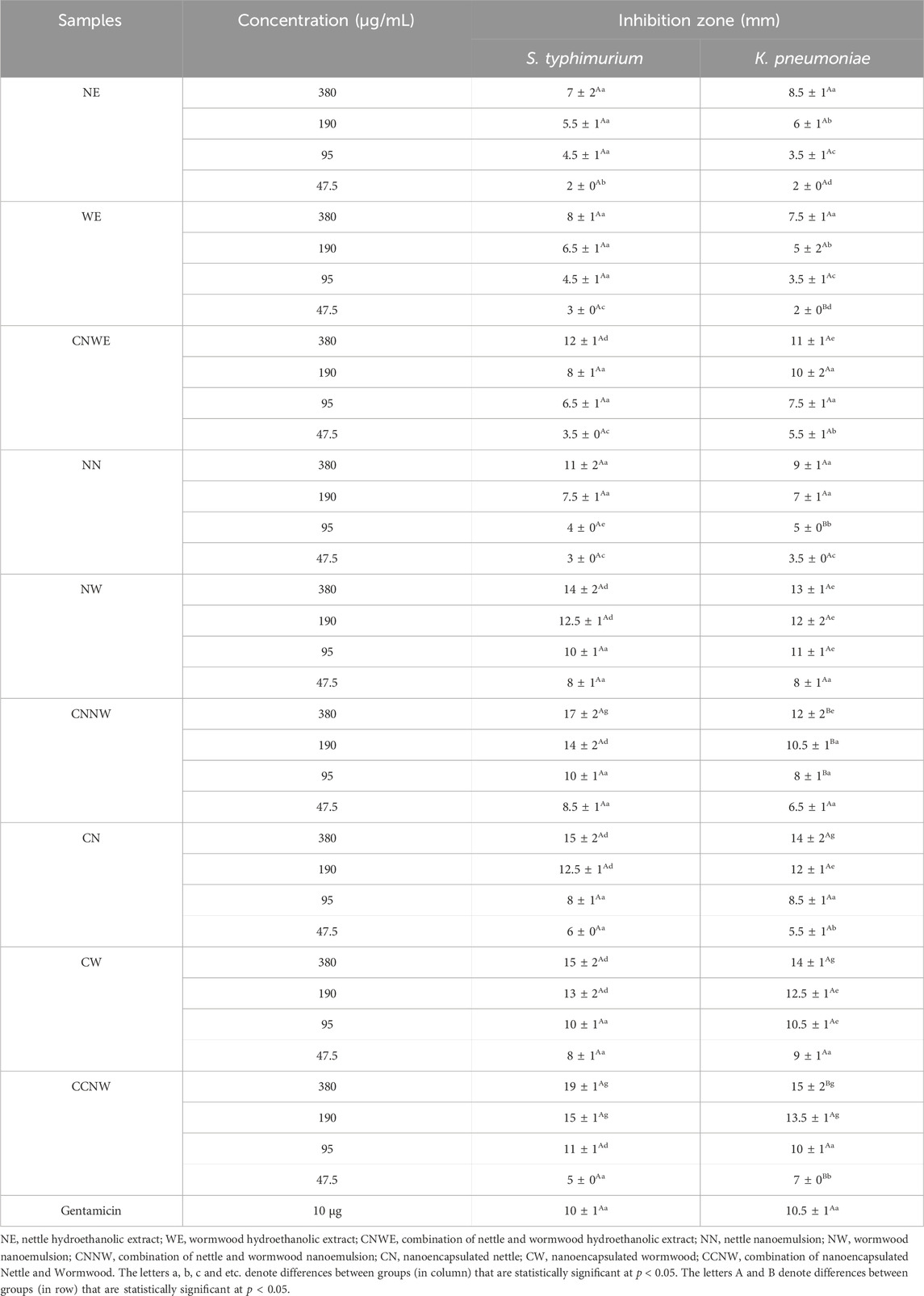
TABLE 1. Antibacterial activity using disc diffusion method of hydroethanolic extract, nanoemulsion and nanoencapsulation of Nettle and Wormwood.
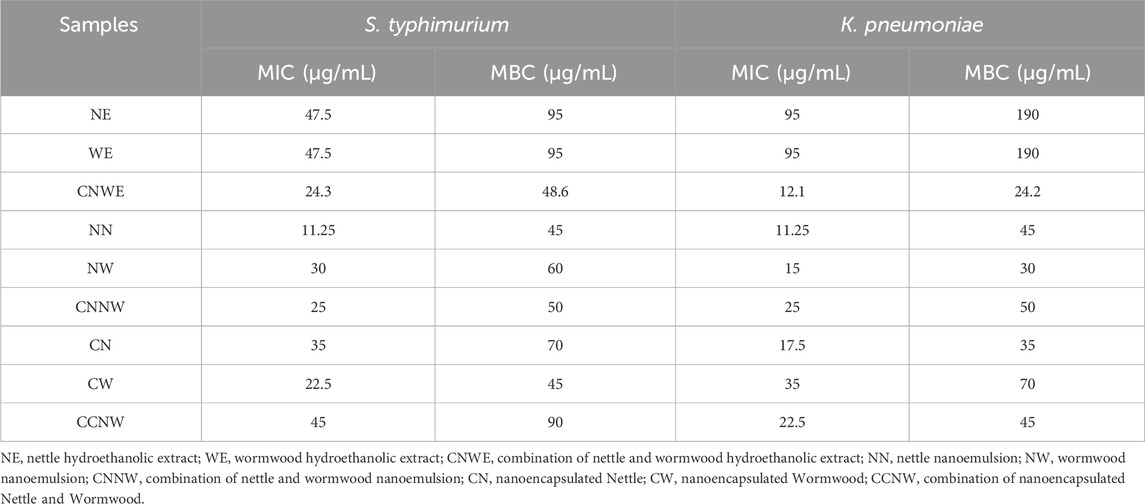
TABLE 2. Minimum inhibitory concentration (MIC) and minimum bactericidal concentration (MBC) of hydroethanolic extract, nanoemulsions and nanoencapsulations of Nettle and Wormwood against two bacteria.
Figure 3 shows the results of cell viability of HCT 116 cells following a 24-h exposure to the tested agents. The findings show that all the agents, including NE, WE, CNWE, NN, NW, CNNW, CN, CW, and CCNW, resulted in a substantial decrease in cell viability within the 24-h timeframe. The IC50 values of NE, WE, CNWE, NN, NW, CNNW, CN, CW, and CCNW were determined to be 250, 170, 560, 380, 312, 370, 250, 420, and 700 μg/mL, respectively. Cell viability assays indicated that exposure to a high concentration of NW resulted in a significantly lower cell viability compared to other groups (p < 0.05). However, as compared to other groups, the most pronounced drop in cell viability was seen with 500 μg/mL concentration of WE (p < 0.05). Figure 4 shows a dose dependent cytotoxic effect with all concentration of agents against HCT 116 (p < 0.05).
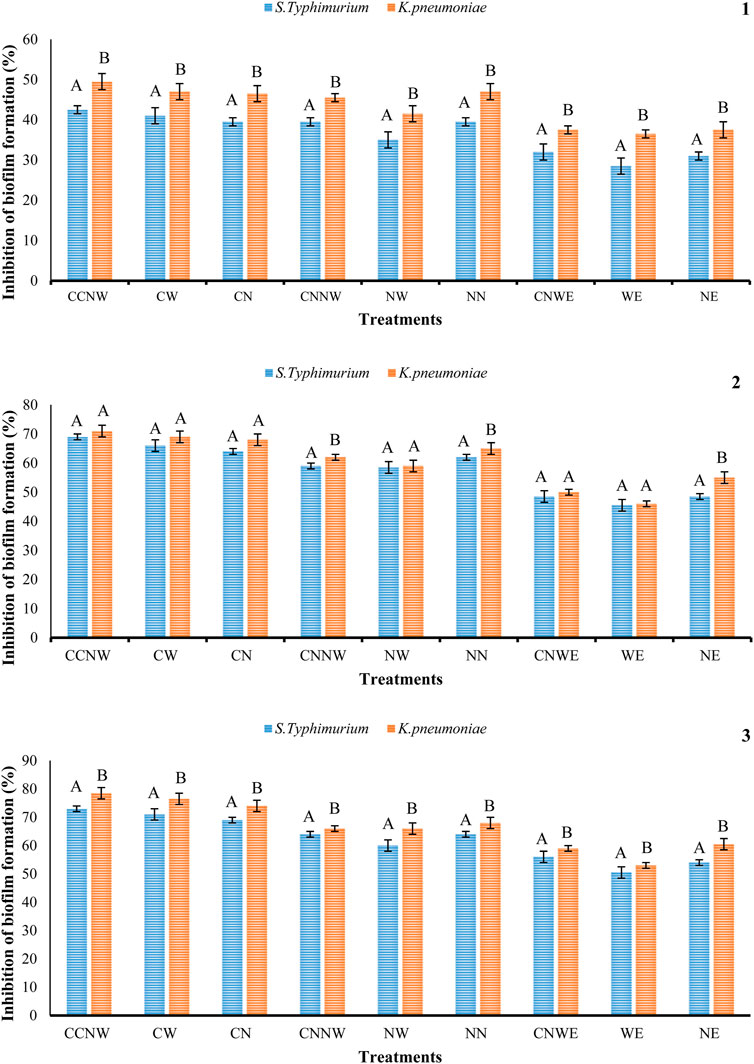
FIGURE 3. Percentage inhibition of S. typhimurium, and K. pneumonia biofilm formation with the various treatments. NE: Nettle hydroethanolic extract, WE: Wormwood hydroethanolic extract, CNWE: Combination of Nettle and Wormwood hydroethanolic extract, NN: Nettle nanoemulsion, NW: Wormwood nanoemulsion, CNNW: Combination of Nettle and Wormwood nanoemulsion, CN: nanoencapsulated Nettle, CW: nanoencapsulated Wormwood, CCNW: combination of nanoencapsulated Nettle and Wormwood. The letters A and B denote differences between groups that are statistically significant at p < 0.05. 1: biofilm formation at ½ MIC concentration, 2: biofilm formation at MIC concentration, 3: biofilm formation at 2 MIC concentration.
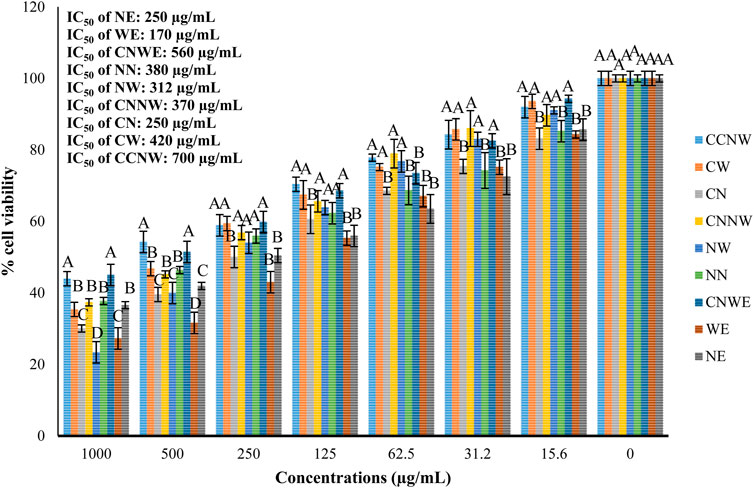
FIGURE 4. Effect of various treatments on the viability of colon cancer cell line (HCT 116). NE: Nettle hydroethanolic extract, WE: Wormwood hydroethanolic extract, CNWE: Combination of Nettle and Wormwood hydroethanolic extract, NN: Nettle nanoemulsion, NW: Wormwood nanoemulsion, CNNW: Combination of Nettle and Wormwood nanoemulsion, CN: nanoencapsulated Nettle, CW: nanoencapsulated Wormwood, CCNW: combination of nanoencapsulated Nettle and Wormwood. The letters A and B denote differences between groups that are statistically significant at p < 0.05.
Discussion
In recent years, plant extracts have been investigated as additives in the food industry, as well as for therapeutic applications due to their rich content of bioactive compounds, i.e., polyphenols and carotenoids that possess antioxidant and antimicrobial activity (Chaleshtori et al., 2015; Proestos, 2020). The secondary metabolites of different plants, including essential oils, extracts, and their nanoemulsion and nanoencapsulation forms, have been widely investigated for their biological actions. As a result, they have undergone extensive testing and are now used in many different applications, including pharmacology, medical sciences, pharmaceutical botany, clinical microbiology, food preservation and phytopathology (Dinda et al., 2016; Sharafati Chaleshtori and Sharafati Chaleshtori, 2017).
Earlier research reported that Nettle-mediated biosynthesis of silver nanoparticles (AgNPs) showed potent antibacterial activity against pathogenic microorganisms. Furthermore, the anticancer effect of the AgNPs was assessed by determining the IC50 dose using the XTT assay against MCF-7 breast cancer cells with an identified IC50 value of 0.243 ± 0.014 μg/mL (Dağlıoğlu et al., 2023b). Hashem and Salem (2022) reported that biosynthesized selenium nanoparticles (SeNPs) from an aqueous extract of U. dioica leaves indicated potent antibacterial activity against both Gram-negative and Gram-positive bacteria, along with multi-cellular and unicellular fungi. The MIC of the SeNPs against E. coli, Pseudomonas aeruginosa, B. subtilis, as well as Staphylococcus aureus were 250, 31.25, and 500 μg/mL, respectively, whilst the MIC values against C. albicans, A. fumigatus, A. niger, and A. flavus were 62.5, 15.62, 31.25, and 7.81 μg/mL, respectively. Moreover, the cytotoxicity of SeNPs on the normal Vero cell line CCL-81, showed an IC50 value of 173.2 μg/mL (Hashem and Salem, 2022). Our results showed that NE, NN, and CN exhibited antibacterial activity against S. typhimurium and K. pneumoniae. The MIC and MBC values for these agents ranged from 11.25 to 95 μg/mL. Additionally, the cytotoxicity of NE, NN, and CN on HCT 116 cells using the MTT assay showed IC50 values of 250, 380, and 250 μg/mL, respectively.
Evaluating the combined impact of Nettle and Wormwood extracts on pathogenic bacteria was a crucial focus of this study. Previous studies have demonstrated that the simultaneous utilization of various herbal extracts has a stronger antimicrobial effect than the use of a single extract. Zarei Yazdeli et al. (2021), demonstrated the potent combination of methanolic extracts from Dracocephalum kotschyi and Trachyspermum ammi in effectively inhibiting the growth of P. aeruginosa, Shigella dysenteriae, E. coli, and S. aureus through a synergistic antimicrobial effect (Zarei Yazdeli et al., 2021). Our results, specifically for CNWE, align with previous research. When multiple antimicrobial agents are used together, they can have a synergistic, antagonistic, or indifferent effect on microbial populations compared to their individual effects. One theory to explain the synergy is that one substance aids in binding another substance to its target site by altering the structure of the cell (Acharjee et al., 2023).
Many hundreds of plant polyphenols have been isolated with diverse molecular structures, typically featuring an aromatic ring with one or multiple hydroxyl groups plus other substituents. These compounds possess beneficial properties like antimicrobial, antioxidant, anticancer, and anti-inflammatory effects (Leri et al., 2020). Plant polyphenols could act as antimicrobial agents against antibiotic-resistant pathogens. These compounds can negatively interfere with various structural and biochemical attributes of microbes, disrupting the normal functioning of cell membranes, cytoplasmic contents, proteins as well as enzymes. As a result, the microbial metabolism is disturbed, leading to failure to grow or to outright cell death (Prakash et al., 2018).
Nanoparticles possess distinct physicochemical characteristics, including high reactivity, a huge surface area to mass ratio, ultrasmall and controlled size, along with a structure that can be functionalized (Onyeaka et al., 2022). Some nanomaterials are used as carrier agents, such as chitosan, cellulose, dextran, starch, cyclodextrin and alginate, but these also possess antimicrobial activity. They achieve this by disrupting membrane potential, generating reactive oxygen species, along with altering metabolic reactions (Kujur et al., 2017; Chen et al., 2023). Nanoemulsions, due to their tiny size and large surface area per unit volume, allow efficient transport through porin proteins in the outer membrane. This enables the effective delivery of herbal components (essential oils or extracts) because the hydrophilic groups of the emulsified molecules are exposed during the process (Barzegar et al., 2023). Furthermore, nanoencapsulation offers a way to control stability, solubility, bioavailability, and promote the release of bioactive components. Numerous encapsulation techniques for natural bioactive components have been demonstrated to be efficient methods to enhance their absorption both in vitro and in vivo (Mukurumbira et al., 2022; Reis et al., 2022).
One study demonstrated that the combined hydroethanolic extracts of A. absinthium and C. paradisi exhibited antibacterial activity against E. coli with a zone of inhibition of 5.6 ± 0.06 mm. However, this treatment did not show any antibacterial activity against S. aureus, K. pneumoniae, or S. enterica. The MTT findings showed that while neither plant extract had an affected the Vero cell line, they had a cytotoxic effect on the Huh-7 cell line (Ali et al., 2021). Sofi et al. (2022) reported that A. absinthium leaf methanolic extract displayed the highest activity against E. faecalis (20 ± 0.7 mm) as well as E. coli (18 ± 0.8 mm), followed by P. aeruginosa (16 ± 0.6 mm), C. albicans (14 ± 0.9 mm), and S. aureus (13 ± 0.8 mm), with MIC values of 128, 128, 128, 256, and 256 μg/mL, respectively. Moreover, this extract exhibited substantial (p ≤ 0.05) cytotoxicity against the A549 cancer cell line (human lung cancer) with an IC50 value of 36.8 μg/mL (Sofi et al., 2022). In agreement with above studies, we observed a significant antibacterial activity against S. typhimurium and K. pneumonia especially for the nanoencapsulation form of WE, Moreover anticancer activity again HCT 116 was observed for the nanoemulsion form of WE (cell viability = 23.37% for 1,000 μg/mL concentration; p < 0.05). A previous study showed that the leaf and stem ethanolic extracts of A. absinthium and A. annua showed significant antibacterial activity against carbapenem-resistant Klebsiella spp., extended-spectrum β-lactamases E. coli (ESBL) and ESBL Klebsiella spp. (Bordean et al., 2023). In our study, the highest antibacterial activity (MIC = 47.5 μg/mL, MBC = 95 μg/mL, IZ = 8 ± 1 mm and 50.5% inhibition of biofilm at 2 MIC concentration) was recorded for WE against S. typhimurium. However, we found that the nanoemulsion, and encapsulation forms of WE had significantly higher antibacterial and anticancer activity than WE alone (p < 0.05). Overall, the antibacterial activity of CCNW against the two tested bacteria was higher than the other agents.
Several compounds, including lommen, vanillic acid, chlorogenic acid and epichatechin have been found in various species of the genus Asteraceae. Recent studies have shown that chlorogenic acid attacks the outer membrane of bacteria, disrupts it, depletes the intracellular potential, and releases macromolecules from the cytoplasm, all leading to cell death (Mamatova et al., 2019). Vanillic acid was found to cause changes in the intracellular ATP concentration, intracellular pH, and membrane potential (Qian et al., 2020). Flavonoids have several hydroxyl groups and show a pronounced tendency to bind to proteins. The inhibition of the binding of KpDnaB (K. pneumoniae DnaB helicase) to dNTPs (deoxyribonucleoside triphosphates) by flavonols in K. pneumoniae, could possibly explain their antibacterial activity (Chen and Huang, 2011).
It is possible that all the extracts, nanoemulsion, and encapsulation forms led to the accumulation of lipid peroxidase (LPO) products accompanied by a decrease in intracellular enzymatic and non-enzymatic antioxidants, resulting in increased LPO-induced apoptosis. Moreover, these agents may induce mitochondrial changes in HCT 116 cells, leading to the translocation of cytochrome C to the cytosol along with caspase activation, which would subsequently trigger apoptosis (Thakur and Devaraj, 2020). Our findings are consistent with some of the previously mentioned studies, even though there are differences in bacterial strains, cancer cell lines, testing methods, and the use of nanoemulsion and encapsulation forms of the hydroethanolic extracts.
Conclusion
In conclusion, our finding confirmed that the combined extracts from both plants, as well as the combined extract in nanoemulsion and nanoencapsulation forms, showed the most pronounced antibacterial activity against K. pneumoniae and S. typhimurium, as well as the highest anticancer activitiy against HCT 116 colon cancer cells. The limitations of the study were that the cell viability assays for bacteria and HCT 116 cells were not sufficiently sensitive and the time points evaluated were insufficient to assess alterations in biofilm growth for bacteria, and subtle pH variations may have affected the results. Further validation of these results will be required using other methods, such as monitoring gene expression in cell lines (biofilm-related genes and colon cancer genes) and eventually carrying out studies in animal models.
Data availability statement
The raw data supporting the conclusion of this article will be made available by the authors, without undue reservation.
Author contributions
ZR: Methodology, Project administration, Supervision, Validation, Writing–review and editing. MK: Formal Analysis, Investigation, Methodology, Project administration, Writing–review and editing. IS: Methodology, Validation, Writing–original draft, Writing–review and editing. HM: Investigation, Methodology, Writing–review and editing. MN: Methodology, Validation, Writing–original draft. RS: Investigation, Methodology, Project administration, Software, Supervision, Validation, Writing–review and editing, Writing–original draft.
Funding
The author(s) declare that no financial support was received for the research, authorship, and/or publication of this article.
Acknowledgments
The authors acknowledge support through the Research Center for Biochemistry and Nutrition in Metabolic Diseases, Kashan University of Medical Sciences, Kashan, Iran.
Conflict of interest
The authors declare that the research was conducted in the absence of any commercial or financial relationships that could be construed as a potential conflict of interest.
Publisher’s note
All claims expressed in this article are solely those of the authors and do not necessarily represent those of their affiliated organizations, or those of the publisher, the editors and the reviewers. Any product that may be evaluated in this article, or claim that may be made by its manufacturer, is not guaranteed or endorsed by the publisher.
References
Acharjee, M., Zerin, N., Ishma, T., and Mahmud, M. R. (2023). In-vitro anti-bacterial activity of medicinal plants against Urinary Tract Infection (UTI) causing bacteria along with their synergistic effects with commercially available antibiotics. New Microbes New Infect. 51, 101076. doi:10.1016/j.nmni.2022.101076
Akram, M., Zainab, R., Daniyal, M., Rasul, S., and Munir, N. (2023). Phytochemistry and pharmacology of Artemisia absinthium linn: a multipurpose medicinal plant. Plant Biotechnol. Persa 5 (1), 0.
Ali, M., Iqbal, R., Safdar, M., Murtaza, S., Mustafa, G., Sajjad, M., et al. (2021). Antioxidant and antibacterial activities of Artemisia absinthium and Citrus paradisi extracts repress viability of aggressive liver cancer cell line. Mol. Biol. Rep. 48 (12), 7703–7710. doi:10.1007/s11033-021-06777-0
Barzegar, F., Nabizadeh, S., Kamankesh, M., Ghasemi, J. B., and Mohammadi, A. (2023). Recent advances in natural product-based nanoemulsions as promising substitutes for hazardous synthetic food additives: a new revolution in food processing. Food Bioprocess Technol., 1–22. doi:10.1007/s11947-023-03162-6
Bhusal, K. K., Magar, S. K., Thapa, R., Lamsal, A., Bhandari, S., Maharjan, R., et al. (2022). Nutritional and pharmacological importance of stinging nettle (Urtica dioica L.): a review. Heliyon 8 (6), e09717. doi:10.1016/j.heliyon.2022.e09717
Bonilla, J., and Sobral, P. J. (2016). Investigation of the physicochemical, antimicrobial and antioxidant properties of gelatin-chitosan edible film mixed with plant ethanolic extracts. Food Biosci. 16, 17–25. doi:10.1016/j.fbio.2016.07.003
Bordean, M.-E., Ungur, R. A., Toc, D. A., Borda, I. M., Marțiș, G. S., Pop, C. R., et al. (2023). Antibacterial and phytochemical screening of Artemisia species. Antioxidants 12 (3), 596. doi:10.3390/antiox12030596
Chaleshtori, R. S., Kopaei, M. R., and Salehi, E. (2015). Bioactivity of Apium petroselinum and Portulaca oleracea essential oils as natural preservatives. Jundishapur J. Microbiol. 8 (3), e20128. doi:10.5812/jjm.20128
Chen, C.-C., and Huang, C.-Y. (2011). Inhibition of Klebsiella pneumoniae DnaB helicase by the flavonol galangin. Protein J. 30, 59–65. doi:10.1007/s10930-010-9302-0
Chen, J., Guo, Y., Zhang, X., Liu, J., Gong, P., Su, Z., et al. (2023). Emerging nanoparticles in food: sources, application, and safety. J. Agric. Food Chem. 71 (8), 3564–3582. doi:10.1021/acs.jafc.2c06740
Clsi, W. L. (2015). M02-A11: performance standards for antimicrobial disk susceptibility tests; Approved Standard. CLSI Clin. Laboratory Stand. Inst. 32 (1).
Dağlıoğlu, Y., Öztürk, B. Y., and Khatami, M. (2023a). Apoptotic, cytotoxic, antioxidant, and antibacterial activities of biosynthesized silver nanoparticles from nettle leaf. Microsc. Res. Tech. 86 (6), 669–685. doi:10.1002/jemt.24306
Dağlıoğlu, Y., Öztürk, B. Y., and Khatami, M. (2023b). Apoptotic, cytotoxic, antioxidant, and antibacterial activities of biosynthesized silver nanoparticles from nettle leaf. Microsc. Res. Tech. 86 (6), 669–685. doi:10.1002/jemt.24306
Dinda, B., Kyriakopoulos, A. M., Dinda, S., Zoumpourlis, V., Thomaidis, N. S., Velegraki, A., et al. (2016). Cornus mas L.(cornelian cherry), an important European and Asian traditional food and medicine: ethnomedicine, phytochemistry and pharmacology for its commercial utilization in drug industry. J. Ethnopharmacol. 193, 670–690. doi:10.1016/j.jep.2016.09.042
Dinkov, D., Stratev, D., Balkanska, R., Sergelidis, D., and Vashin, I. (2016). Reduction effect of royal jelly and rape honey alone and in combination against methicillin-resistant Staphylococcus aureus (MRSA) strains. J. Bacteriol. Virology 46 (1), 36–43. doi:10.4167/jbv.2016.46.1.36
Elez Garofulić, I., Malin, V., Repajić, M., Zorić, Z., Pedisić, S., Sterniša, M., et al. (2021). Phenolic profile, antioxidant capacity and antimicrobial activity of nettle leaves extracts obtained by advanced extraction techniques. Molecules 26 (20), 6153. doi:10.3390/molecules26206153
English, M., Okagu, O. D., Stephens, K., Goertzen, A., and Udenigwe, C. C. (2023). Flavour encapsulation: a comparative analysis of relevant techniques, physiochemical characterisation, stability, and food applications. Front. Nutr. 10, 1019211. doi:10.3389/fnut.2023.1019211
Hashem, A. H., and Salem, S. S. (2022). Green and ecofriendly biosynthesis of selenium nanoparticles using Urtica dioica (stinging nettle) leaf extract: antimicrobial and anticancer activity. Biotechnol. J. 17 (2), 2100432. doi:10.1002/biot.202100432
Hassanshahian, M., Saadatfar, A., and Masoumipour, F. (2020). Formulation and characterization of nanoemulsion from Alhagi maurorum essential oil and study of its antimicrobial, antibiofilm, and plasmid curing activity against antibiotic-resistant pathogenic bacteria. J. Environ. Health Sci. Eng. 18, 1015–1027. doi:10.1007/s40201-020-00523-7
Kaur, M., Prasher, D., Sharma, A., Ghosh, D., and Sharma, R. (2023). Natural sunlight driven photocatalytic dye degradation by biogenically synthesized tin oxide (SnO2) nanostructures using Tinospora crispa stem extract and its anticancer and antibacterial applications. Environ. Sci. Pollut. Res. 30 (13), 38869–38885. doi:10.1007/s11356-022-25028-8
Kujur, A., Kiran, S., Dubey, N., and Prakash, B. (2017). Microencapsulation of Gaultheria procumbens essential oil using chitosan-cinnamic acid microgel: improvement of antimicrobial activity, stability and mode of action. LWT 86, 132–138. doi:10.1016/j.lwt.2017.07.054
Leri, M., Scuto, M., Ontario, M. L., Calabrese, V., Calabrese, E. J., Bucciantini, M., et al. (2020). Healthy effects of plant polyphenols: molecular mechanisms. Int. J. Mol. Sci. 21 (4), 1250. doi:10.3390/ijms21041250
Mamatova, A. S., Korona-Glowniak, I., Skalicka-Woźniak, K., Józefczyk, A., Wojtanowski, K. K., Baj, T., et al. (2019). Phytochemical composition of wormwood (Artemisia gmelinii) extracts in respect of their antimicrobial activity. BMC complementary Altern. Med. 19 (1), 288–8. doi:10.1186/s12906-019-2719-x
Mehrabani, M., Rameshk, M., and Raeiszadeh, M. (2016). Afsantin (Artemisia absinthium L.) from the perspective of modern and traditional Persian medicine. J. Islamic Iran. Traditional Med. 6 (4), 305–313.
Muchtaromah, B., Safitri, E. S., Fitriasari, P. D., and Istiwandhani, J. (2020). “Antibacterial activities of Curcuma mangga Val. extract in some solvents to Staphylococcus aureus and Escherichia coli,” in AIP Conference Proceedings (RS, Brazil: AIP Publishing).
Mukurumbira, A. R., Shellie, R. A., Keast, R., Palombo, E. A., and Jadhav, S. R. (2022). Encapsulation of essential oils and their application in antimicrobial active packaging. Food control. 136, 108883. doi:10.1016/j.foodcont.2022.108883
Naseema, A., Kovooru, L., Behera, A. K., Kumar, K. P., and Srivastava, P. (2021). A critical review of synthesis procedures, applications and future potential of nanoemulsions. Adv. Colloid Interface Sci. 287, 102318. doi:10.1016/j.cis.2020.102318
Onyeaka, H., Passaretti, P., Miri, T., and Al-Sharify, Z. T. (2022). The safety of nanomaterials in food production and packaging. Curr. Res. Food Sci. 5, 763–774. doi:10.1016/j.crfs.2022.04.005
Pal, S., and Dey, A. (2023). Thujone in daily life-a review on natural sources of Thujone, its side effects and reduction mechanism of Thujone toxicity. Biolife 11 (1), 21–31.
Prakash, B., Kujur, A., Yadav, A., Kumar, A., Singh, P. P., and Dubey, N. (2018). Nanoencapsulation: an efficient technology to boost the antimicrobial potential of plant essential oils in food system. Food control. 89, 1–11. doi:10.1016/j.foodcont.2018.01.018
Proestos, C. (2020). The benefits of plant extracts for human health. Foods 9 (11), 1653. doi:10.3390/foods9111653
Qian, W., Yang, M., Wang, T., Sun, Z., Liu, M., Zhang, J., et al. (2020). Antibacterial mechanism of vanillic acid on physiological, morphological, and biofilm properties of carbapenem-resistant Enterobacter hormaechei. J. food Prot. 83 (4), 576–583. doi:10.4315/jfp-19-469
Rafieian, M. (2010). Antibacterial effects of ethanolic extract of walnut leaves (Juglans regia) on propionibacterium acnes. J. Adv. Med. Biomed. Res. 18 (71), 42–49.
Reis, D. R., Ambrosi, A., and Luccio, M. D. (2022). Encapsulated essential oils: a perspective in food preservation. Future Foods 5, 100126. doi:10.1016/j.fufo.2022.100126
Sharafati Chaleshtori, F., and Sharafati Chaleshtori, R. (2017). Antimicrobial activity of chitosan incorporated with lemon and oregano essential oils on broiler breast meat during refrigerated storage. Nutr. Food Sci. 47 (3), 306–317. doi:10.1108/nfs-08-2016-0123
Sharafati-Chaleshtori, R., Sharafati-Chaleshtori, F., Rafieian-kopaei, M., Drees, F., and Ashrafi, K. (2010). Comparison of the antibacterial effect of ethanolic walnut (juglans regia) leaf extract with chlorhexidine mouth rinse on Streptococcus mutans and sanguinis. J. Iran. Dent. Assoc. 22 (4), 211–217.
Shehabeldine, A. M., Doghish, A. S., El-Dakroury, W. A., Hassanin, M. M., Al-Askar, A. A., AbdElgawad, H., et al. (2023). Antimicrobial, antibiofilm, and anticancer activities of syzygium aromaticum essential oil nanoemulsion. Molecules 28 (15), 5812. doi:10.3390/molecules28155812
Sofi, M. A., Nanda, A., Sofi, M. A., Sheikh, T., and Rather, G. A. (2022). In vitro evaluation of antimicrobial and anticancer potential of Artemisia absinthium growing in Kashmir Himalayas. J. Appl. Nat. Sci. 14 (3), 895–902. doi:10.31018/jans.v14i3.3619
Sohail, J., Zubair, M., Hussain, K., Faisal, M., Ismail, M., Haider, I., et al. (2023). Pharmacological activities of Artemisia absinthium and control of hepatic cancer by expression regulation of TGFβ1 and MYC genes. Plos one 18 (4), e0284244. doi:10.1371/journal.pone.0284244
Souza, T. G., Ciminelli, V. S., and Mohallem, N. D. S. (2016). “A comparison of TEM and DLS methods to characterize size distribution of ceramic nanoparticles,” in Journal of Physics: Conference Series, Moscow, Russian, 20-21 October 2020 (Bristol, UK: IOP Publishing).
Sultan, M. H., Zuwaiel, A. A., Moni, S. S., Alshahrani, S., Alqahtani, S. S., Madkhali, O., et al. (2020). Bioactive principles and potentiality of hot methanolic extract of the leaves from Artemisia absinthium L "in vitro cytotoxicity against human MCF-7 breast cancer cells, antibacterial study and wound healing activity. Curr. Pharm. Biotechnol. 21 (15), 1711–1721. doi:10.2174/1389201021666200928150519
Teixeira, J., Nunes, P., Outor-Monteiro, D., Mourão, J. L., Alves, A., and Pinheiro, V. (2023). Effects of Urtica urens in the feed of broilers on performances, digestibility, carcass characteristics and blood parameters. Anim. (Basel) 13 (13), 2092. doi:10.3390/ani13132092
Thakur, R. S., and Devaraj, E. (2020). <i>Lagerstroemia speciosa</i> (L.) Pers. triggers oxidative stress mediated apoptosis via intrinsic mitochondrial pathway in HepG2 cells. Environ. Toxicol. 35 (11), 1225–1233. doi:10.1002/tox.22987
Wu, S., Wang, C., Bai, D., Chen, N., Hu, J., and Zhang, J. (2023). Perspectives of international multi-center clinical trials on traditional Chinese herbal medicine. Front. Pharmacol. 14, 1195364. doi:10.3389/fphar.2023.1195364
Zarei Yazdeli, M., Ghazaei, C., Tasallot Maraghi, E., Bakhshi, A., and Shukohifar, M. (2021). Evaluation of antibacterial synergism of methanolic extract of Dracocephalum kotschyi and Trachyspermum ammi. Malays J. Med. Sci. 28 (6), 64–75. doi:10.21315/mjms2021.28.6.7
Keywords: Urtica dioica, Artemisia absinthium, antibacterial activity, anticancer activity, hydroethanolic extract, nanoemulsion, nanoencapsulation
Citation: Rahmani Z, Karimi M, Saffari I, Mirzaei H, Nejati M and Sharafati Chaleshtori R (2024) Nanoemulsion and nanoencapsulation of a hydroethanolic extract of Nettle (Urtica dioica) and Wormwood (Artemisia absinthium): comparison of antibacterial and anticancer activity. Front. Chem. 12:1266573. doi: 10.3389/fchem.2024.1266573
Received: 25 July 2023; Accepted: 08 January 2024;
Published: 16 January 2024.
Edited by:
Baskaran Stephen Inbaraj, Fu Jen Catholic University, TaiwanReviewed by:
Mansuri M. Tosif, Lovely Professional University, IndiaJyoti Singh, Lovely Professional University, India
Luqman Jameel Rather, Southwest University, China
Copyright © 2024 Rahmani, Karimi, Saffari, Mirzaei, Nejati and Sharafati Chaleshtori. This is an open-access article distributed under the terms of the Creative Commons Attribution License (CC BY). The use, distribution or reproduction in other forums is permitted, provided the original author(s) and the copyright owner(s) are credited and that the original publication in this journal is cited, in accordance with accepted academic practice. No use, distribution or reproduction is permitted which does not comply with these terms.
*Correspondence: Merat Karimi, merat.karimi123@gmail.com; Iman Saffari, imansaffari9@gmail.com; Reza Sharafati Chaleshtori, sharafati.reza@gmail.com