- 1Hoffmann Institute of Advanced Materials, Postdoctoral Innovation Practice Base, Shenzhen Polytechnic University, Shenzhen, China
- 2Jiangsu Provincial Engineering Research Center of Low Dimensional Physics and New Energy, School of Science, Key Laboratory for Organic Electronics and Information Displays, Institute of Advanced Materials (IAM), Jiangsu National Synergistic Innovation Center for Advanced Materials (SICAM), Nanjing University of Posts and Telecommunications, Nanjing, China
The remarkable optoelectronic properties of organometal halide perovskite solar cells have captivated significant attention in the energy sector. Nevertheless, the instability of 3D perovskites, despite their extensive study and attainment of high-power conversion efficiency, remains a substantial obstacle in advancing PSCs for practical applications and eventual commercialization. To tackle this issue, researchers have devised mixed-dimensional perovskite structures combining 1D and 3D components. This innovative approach entails incorporating stable 1D perovskites into 3D perovskite matrices, yielding a significant improvement in long-term stability against various challenges, including moisture, continuous illumination, and thermal stress. Notably, the incorporation of 1D perovskite yields a multitude of advantages. Firstly, it efficiently passivates defects, thereby improving the overall device quality. Secondly, it retards ion migration, a pivotal factor in degradation, thus further bolstering stability. Lastly, the inclusion of 1D perovskite facilitates charge transport, ultimately resulting in an elevated device efficiency. In this succinct review, we thoroughly encapsulate the recent progress in PSCs utilizing 1D/3D mixed-dimensional architectures. These advancements encompass both stacked bilayer configurations of 1D/3D structures and mixed monolayer structures of 1D/3D. Additionally, we tackle critical challenges that must be surmounted and offer insights into the prospects for further advancements in this domain.
1 Introduction
The recent rapid development of research on organometal halide perovskite solar cells (PSCs) has made them become one of the most promising photoelectric materials to alleviate the energy crisis and global climate issues due to their economical cost, facilitated large-scale fabrication and, most of all, excellent photoelectric properties such as high absorption coefficient, long carrier diffusion length and broad-range absorption (Bai et al., 2019; Jeong et al., 2020; Bu et al., 2021; Jeong et al., 2021; Min et al., 2021; Yoo et al., 2021; Li et al., 2022a; Li et al., 2022b; Wang et al., 2022b; Ni et al., 2022). In the past decade, the power conversion efficiency (PCE) of PSCs has significantly improved to 26.1% (Best, 2023), which is comparable to commercial solar cells (Kojima et al., 2009; Min et al., 2021). However, despite their considerable potential in next-generation solar cells, further improvement of PSCs for practical application still faces several challenges, especially the unreliable stability in complex and harsh working environments due to the intrinsic structure of 3D perovskites that are extensively studied, making them sensitive when exposed to UV light, moisture, heat, and oxygen (Wang et al., 2016; Wang et al., 2017; Heo et al., 2019; Yang et al., 2020b; Nazir et al., 2022). To address this critical issue, the introduction of one-dimensional (1D) perovskite, constantly named as perovskitoid (Milić et al., 2019), has been regarded as an ideal alternative due to their unique characteristics such as structural diversity, multifunctional properties, and extraordinary environmental stability.
Generally, the chemical formula of 3D organometal halide perovskites is ABX3, in which A represents small cations such as methylammonium cations (MA+), Formamidine cations (FA+) and cesium cations (Cs+), B stands for bivalent metal cations (e.g., Pb2+, Sn2+) and X is halide anion (e.g., Cl−, Br−, I−), the typical structure of 3D perovskite can be described as the [BX6]4- octahedra surrounded by A-site cations are corner-sharing with each adjacent one in all three dimensions to form octahedral framework, therefore 3D perovskites are classic bulk materials (Lin et al., 2018; Chu et al., 2021; Yao et al., 2021). It is worth noting that in order to maintain stable bulk structure, the radius of the A-site cations is strictly limited according to tolerance factor (Li et al., 2016; Bartel et al., 2019). By comparison, the utilization of large cations occupying A-site in 1D perovskites expands the lattice configuration, in the meantime the adjacent [BX6]4- octahedra may not only be corner-sharing, but also be edge-sharing or face-sharing to form linear perovskite chains with “shoulder to shoulder” arrangement (Stoumpos et al., 2017; Zhou et al., 2017; Hong et al., 2018). The intrinsic high formation and low self-doping energies can remarkably suppress the occurrence of Schottky/Frenkel defects, thus inhibiting the ion migration. In addition, the presence of large hydrophobic cations efficiently prevents the lattice structure from moisture invasion, resulting in superior long-term stability of 1D perovskites (Fu et al., 2019; Kim and Seok, 2020; Paek et al., 2020). Nevertheless, the application of pure 1D perovskites in photovoltaic field suffers from a series of limitations, such as wide optical bandgap, high exciton binding energy caused by natural quantum wells, poor charge conductivity and low film quality (Koh et al., 2016; Yuan and Huang, 2016; Qian et al., 2017; Yang et al., 2018; Yang et al., 2022; Chen et al., 2023a; Zheng et al., 2023) Therefore, the 1D/3D mixed-dimensional perovskites have been extensively studied to combine the excellent photoelectric performance of 3D perovskites and the reliable long-term stability of 1D perovskites.
In this review, we summarize the recent progress on 1D/3D mixed-dimensional PSCs. We systematically classify them into 1D/3D stacked bilayers and 1D/3D mixed-dimensional layers according to the distribution of 1D perovskites. In particular, various functional effects or mechanisms owing to the incorporation of diverse 1D perovskite are stated. Finally, we carry on a brief summary and point out some perspectives toward further improving stability and efficiency of mixed-dimensional PSCs.
2 1D perovskite
Typically, rigid and bulk organic cations are incorporated within the perovskite structure to serve as spacers, prompting the assembly of [BX6]4− octahedra into a chain arrangement to form the 1D perovskite structure. The bulk organic cations that occupy the [BX6]4− octahedral chain predominantly encompass aromatic amines, pyridinium, and so on (Chen et al., 2023b; Zhao et al., 2023). In this mini review, Figure 1 illustrates a range of A-site cations capable of inducing the formation of 1D perovskite structures. Attributed to the distinctive structure of 1D perovskite, where octahedral chains are isolated within rigid ammonium groups, carriers encounter confinement within the chain (Ge et al., 2022; Gao et al., 2023). This confinement results in elevated exciton binding energy and notable quantum confinement effects, which might not appear suitable for PSC applications. However, numerous reports have illustrated that when integrated into 1D/3D heterojunctions, these 1D perovskites can effectively function as passivation layers, thereby reducing interface defect density (Zhan et al., 2021a; Liu et al., 2023). Simultaneously, the inorganic [BX6]4− octahedral chain is enveloped by rigid organic cations in 1D perovskites, resulting in a theoretically more environmentally stable structure than 3D perovskites (Zhu et al., 2022). This inherent stability is beneficial for enhancing the relative stability of PSC devices. The application of 1D/3D heterojunction structures in PSCs has been extensively explored and will be discussed in intricate detail below.
3 1D/3D stacked bilayer
The bilayer multidimensional PSCs which contain pristine 3D perovskites layer and 1D perovskites passivation layer fabricated by posttreatment on top of 3D layer are considered to be an effective strategy. In 2019, Gao’s group (Gao et al., 2019) modified 3D perovskite by depositing thiazole ammonium (TA) through a gas-pump drying-assisted two-step process. More important, the introduction of a 1D capping layer effectively passivates 3D perovskite films, leading to enhanced charge transport, prolonged carrier lifetime, and prevention of iodide ion migration within the 3D perovskite. Moreover, the resultant device exhibited significant efficiency and superior environmental stability (Figure 2A). Elsenety et al. (2020) applied a 1D (CH3)3SPbI3 extra top layer via spin-coating onto the perovskite film (Figure 2B). This additional layer functions as a barrier, effectively hindering ionic migration and charge carrier recombination. Consequently, this barrier layer significantly enhances the stability of non-sealed devices, demonstrating improved performance under both ambient conditions and light-induced stress. Xu et al. (2020) address both the phase stability and energy efficiency concerns of FA-based PSCs by treating the perovskite surface with pyrrolidinium hydroiodide (PyI) salts. This treatment results in the formation of a 1D perovskite structure (PyPbI3) stacked onto the original 3D perovskite, revealing that the temperature-dependent phase transition activation barrier is heightened upon the creation of the 1D/3D structure via in-situ XRD measurements (Figure 2C). This innovative 1D/3D bilayer configuration holds the potential to be employed in PSCs to bolster both phase stability and photovoltaic performance. Yang et al. (2020a) introduce an innovative approach involving the in situ cross-linking of polymerizable propargylammonium (PA+) onto the surfaces and grain boundaries of 3D perovskite films to the formation of a 1D/3D perovskite heterostructure (Figure 2D). This passivation technique brings about notable enhancements in both interfacial carrier transport and the alleviation of residual tensile strain within the perovskite films. Consequently, the resulting devices achieve excellent photovoltaic performance. More recently, Chen et al. (2022a) effectively establishes a robust interface for perovskite films by employing 1D/3D mixed-dimensional engineering. Furthermore, the 1D capping layer acts as a diffusion barrier, curtailing ion migration and safeguarding the vulnerable 3D perovskite from moisture-induced degradation. The photovoltaic device achieves a high PCE and improved longterm stability (Figure 2E).
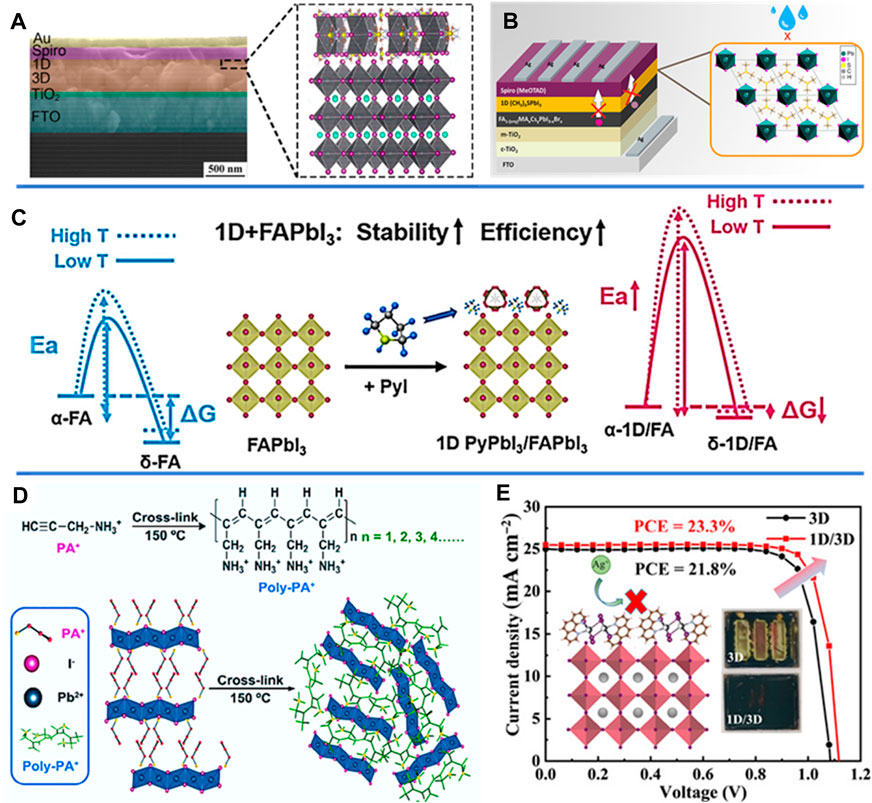
FIGURE 2. (A) Schematic illustration of crystal structure of TA+ based 1D/3D stacked perovskite. (B) Schematic diagram of device incorporated with 1D (CH3)3SPbI3. (C) The thermodynamic and kinetic analysis of 3D perovskite and 1D/3D perovskite phase transition process. (D) Schematic illustration of the cross-linking progress of PA+ in 1D perovskitoid. (E) J-V curves and apparent images of control and phen modified 1D/3D devices.
Wang et al. (2022a) employed 4-chlorobenzamidine hydrochloride (CBAH) as a spacer to facilitate the development of an orientationally crystallized nanorod-like 1D perovskite layer atop the 3D perovskite surface. Further structural analyses reveal the coexistence of both 1D and 3D hybrid perovskite lattices within the nanorod-like passivation layer via SEM-EDS and TEM (Figures 3A–C). This coexistence effectively governs crystallization and morphology, consequently aiding in the enhancement of charge extraction and the suppression of charge recombination. Notably, the unencapsulated devices demonstrate elevated PCE, heightened thermal, moisture, and illumination stability. Zhang et al. (2023) confirmed ChI’s reaction with CsPbI3 to form a novel 1D ChPbI3 crystal phase, creating a 1D/3D heterostructure with CsPbI3, more effectively mitigating defects and substantially enhancing lifetime (Figure 3D). Remarkably, the 1D ChPbI3 suppressed δ-CsPbI3 deficiencies during formation, significantly bolstering CsPbI3 film stability. The final device achieved a certified record PCE of 18.05% for HTM-free inorganic C-PSCs. Meng et al. (2023) introduced the ionic liquid 1-ethyl-3-methylimidazolium iodide ([EMIM]I) to the 3D perovskite surface, reducing iodine vacancy defects and modulates band energy alignment, resulting in a substantial enhancement of the open circuit voltage (Voc) (Figure 3E). Consequently, the device achieves a high PCE and remarkable environmental and thermal stability. Chen et al. (2022b) introduced a novel type of benzimidazolium salt, N,N′-dialkylbenzimidazolium iodide to form 1D capping layer-topped 1D/3D structure (Figure 3F). This conformal interface modulation not only effectively stabilizes FACs-based perovskite films by impeding lateral and vertical iodide diffusion within devices or modules, thus ensuring excellent operational and environmental stability, but also establishes a proficient charge transport pathway through a well-designed 1D crystal structure.
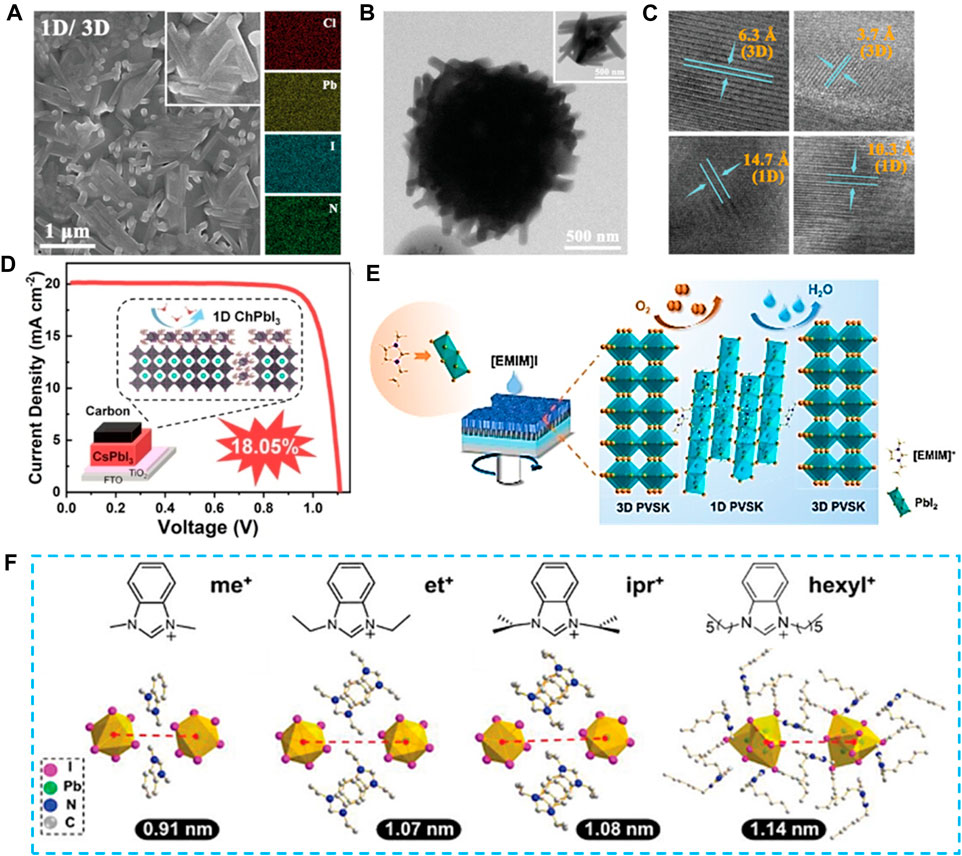
FIGURE 3. (A) Top-surface SEM and EDS images of the CBAH-treated perovskite films. (B,C) High-resolution TEM images of the CBAH-treated perovskite film. (D) ChPbI3 was formed via ChI treatment of CsPbI3 surface, creating a 1D/3D heterostructure that substantially enhances photovoltaic performance. (E) The ionic liquid [EMIM]I was introduced at the 3D perovskite surface to enhance device photovoltaic performance. (F) Crystal structures and corresponding front-view crystal structures of 1D perovskites.
4 1D/3D mixed monolayer
Apart from the 1D/3D stacked bilayer structure mentioned above, the mixed-dimensional perovskite monolayer is another typical structure of 1D/3D perovskite heterojunction, in which the 1D perovskitoid can be regarded as a specific additive to reduce bulk defect density, block ion migration, and prevent the collapse of 3D perovskite induced by moisture. The most common method to introduce 1D perovskitoid is mixing target ligands in perovskite precursor solutions; hence, it is constantly capable of regulating morphology by effecting the crystallization process of perovskite. Early as in 2016, Bi et al. (2016) incorporated 1,1,1-trifluoro-ethyl ammonium iodide (FEAI) into MAPbI3 to simultaneously enhance the stability and efficiency. Moreover, the apparent defects which widely distributed on the surface of MAPbI3 has been obviously reduced after the introducing of FEAI via SEM (Figure 4A). The optimal device exhibited a PCE of 18% and long-term stability (Figure 4B). Fan et al. (2018) introduced 2-(1H-pyrazol-1-yl)pyridine (PZPY) into 3D perovskites, enabling the creation of 1D/3D hybrid perovskite materials. The 1D/3D hetero-structural exhibit the remarkable ability to significantly extend photoluminescence decay lifetime and suppress charge carrier recombination. Crucially, the fabricated 1D/3D PSCs showcase a thermodynamic self-healing capacity, attributed to the flexible 1D perovskite’s ability to obstruct the ion-migration pathways of A-site ions. Zhang et al. (2021) demonstrate the formation of 1D/3D mixed perovskites by integrating room temperature 1D ferroelectric TMIMPbI3 with 3D perovskite, displaying notable ferroelectric properties. HR-TEM analysis confirmed that the incorporation of the 1D perovskite into the precursor results in the formation of a 1D/3D mixed perovskite film. The 1D phase is uniformly dispersed throughout the film without noticeable phase separation, underscoring that the 1D/3D mixed perovskite represents an integral entity with a shared PbI6 octahedra backbone (Figure 4C). Yu et al. (2021) introduced hydrazinium cation (HA+: NH2NH3+) into perovskite layer. The robust hydrogen bonding of HA+ elevates perovskite crystallinity and grain size (Figures 4D, E). This engenders a 1D/3D hybrid dimension structure, bolstering the photovoltaic performance of PSCs. Kong et al. (2021) utilize 2-diethylaminoethylchloride cations (DEAEC+) as templates to induce a 1D@3D perovskite structure (Figure 4F), resulting in smoother surface texture, extended charge-carrier lifetime, reduced residual tensile strain, and minimized surface-defect density in the perovskite film. This approach yields highly efficient and stable 1D@3D perovskite solar cells (PSCs) with remarkable reproducibility. Zhan et al. (2021a) investigated a novel 1D/3D perovskite material through the introduction of a bulk benzimidazole cation (Bn+) organic salt. Bn+ induces controlled crystalline growth of 3D perovskite with preferred orientation while creating spatially distributed 1D BnPbI3 perovskite within the 3D perovskite film. The 1D/3D perovskite effectively curbs electro-strictive responses and imbalanced charge carrier extraction, cultivating an inherently stable lattice with fortified ionic bonds and reduced excess charge carriers. Consequently, ion migration tendencies within the p-i-n 1D/3D PSC are significantly mitigated under operational conditions. Wei et al. (2022) introduced a functional ionic liquid, 1-ethyl-3-methylimidazolium trifluoroacetate (EMIMTFA), where EMIM+ interact with PbI2 to establish a stable 1D perovskite, and anions TFA− passivate the perovskite surface. EMIM+ cations are distributed not only on the top surface and within the bulk phase but also accumulate at the buried interface of the 3D perovskite film, resulting in a multi-level distribution. This distribution effectively passivates distinct defect states, notably reducing non-radiative recombination.
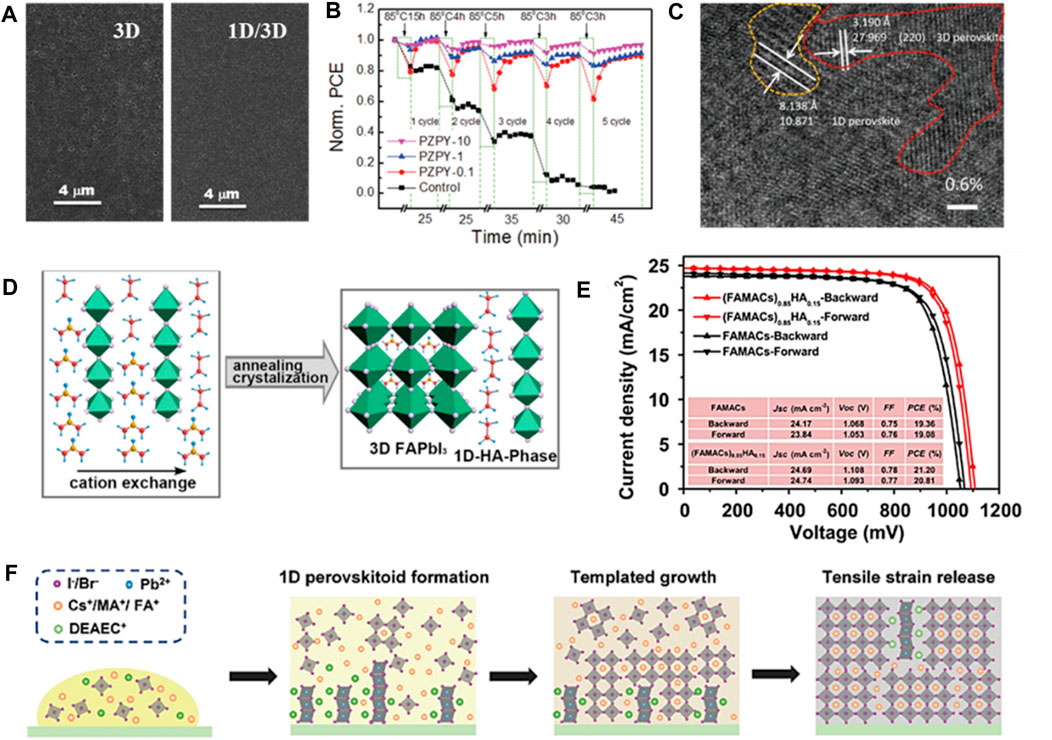
FIGURE 4. 1D/3D mixed monolayer structure. (A) SEM images of pristine and mixed dimensional perovskite films. (B) The long-term stability of the unencapsulated control and 1D/3D hybrid PSCs during temperature cycling. (C) HRTEM image of 1D/3D heterostructure perovskite film. (D) Schematic illustration of cation exchange process between HA+ and FA+ and (E) J-V curves of control and HA modified devices. (F) Schematic diagram of the template-induced crystallization evolution process of the 1D/3D perovskite film introduced with DEAECCl.
Long et al. (2021) introduced aminoquinoline to create a new 1D perovskite with closely arranged distorted octahedra and orderly distributed quinoline (Aq) organic groups (Figure 5A). Incorporating this 1D AqPbI3(II) perovskite into perovskite improves carrier transport and hydrophobicity due to its unique structure and lattice-matching heterojunction. The resulting cell increased Voc, PCE, and stability. Chen et al. (2023c) developed a cross-linkable monomer [5-(1,2-dithiolan-3-yl)] pentanehydrazide hydroiodide (TA-NI) with specific building blocks, including dynamic covalent disulfide bonds, H-bonds, and ammonium functional groups (Figure 5B). This cross-linked TA-NI possesses elastomeric properties and can autonomously self-heal at room temperature. It also forms cross-links during perovskite film growth, attaching to grain boundaries and repairing mechanical-stress-induced cracks in the perovskite film. Furthermore, the ammonium functional group can interact with PbI64− to create a 1D perovskite, which reduces residual tensile strain in the perovskite, mitigates lattice mismatch, and inhibits ion migration. Wang et al. (2023) introduced an IL called 1-(3-cyanopropyl)-3-methylimidazolium chloride (CPMIMCl) to induce an in-plane preferred orientation of 1D perovskite in 3D perovskite thin films through a sequential deposition process. The crystal structure of 1D perovskite is shown in Figure 5C. This in-plane-oriented 1D CPMIMPbI3 resides at grain boundaries within 3D perovskite, resulting in high-quality films with larger grain sizes and enhanced defect passivation. Consequently, the devices exhibit enhanced photovoltaic performance. We summarize all PSCs based on 1D/3D mixed-dimensional structure in Table 1.
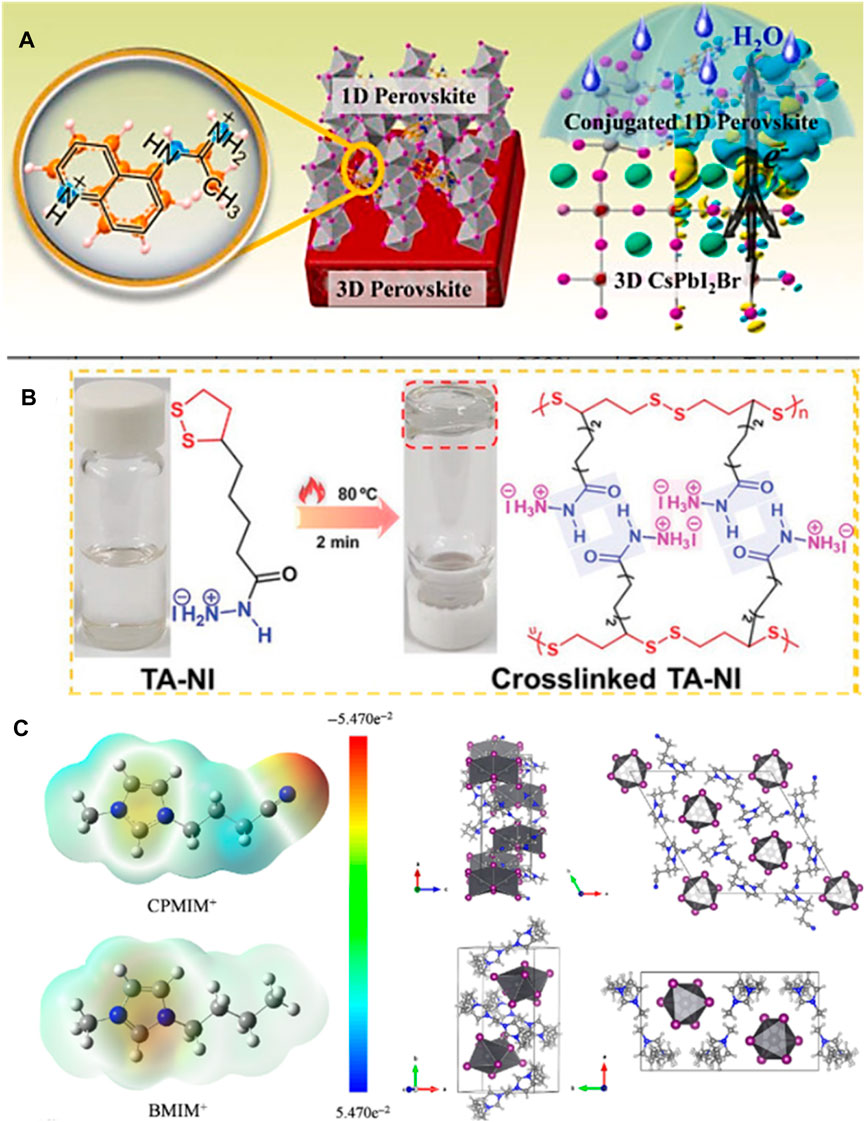
FIGURE 5. (A) A-site quinoline organic groups and 1D–3D heterojunction. (B) TA-NI before and after cross-linking: the state in solvent and chemical structures. (C) ESP distribution maps and structural arrangements of ions CPMIM+ and BMIM+.
5 Conclusion and outlooks
The excellent photoelectric properties of 3D perovskite and reliable stability of 1D perovskite have attracted tremendous attention on researches of 1D/3D mixed-dimensional perovskite. In the past few years, numerous precious outbreaks have been achieved already, in this review we divide they into two typical structures according to the distribution of 1D perovskite and briefly state the recent research progress of each one. Overall, the beneficial improvements of the introduction of 1D perovskite can be summarized as reduced defects, inhibited ion migration, promoted charge transport and, most of all, enhanced long-term stability. As mentioned previously in this review, the impressive high PCE of devices based on 1D/3D mixed-dimensional perovskite can be realized by applying reasonable and appropriate strategies, and most of them only get small losses of initial efficiency after aging under complex conditions for long period. In addition, the incorporation of 1D perovskite may leads to various extra functional effects or special configurations due to the diversity of additional ligands, such as the cross-linked structure, thermodynamic self-healing ability, ferroelectricity and so on.
Even though great improvements have been achieved in this field, substantial issues that severely restrict the further developments and potential applications of 1D/3D mixed-dimensional PSCs need to be addressed urgently. The major problem is the unsatisfied device efficiency compared to that of the state-of-the-art PSCs (26.1%). This is mainly attributed to the intrinsic poor and anisotropic charge conductivity of 1D perovskite, hence the further exploration for new ligand that possess the capability of forming 1D perovskite and better natural characteristics is essential. Secondly, most researches are focus on small-area films and devices, which are definitely far away from available practical applications. Even if appreciable PCE of small-area devices have been realized, it is not convincing for preparation of large-area devices considering the difficulty of fabricating large area films. Therefore, the exploration on preparation methods toward high quality large-area films is essential to meet the requirements in practical applications. Finally, the low-cost advantage of PSCs is always weakened by the high-cost of electron or hole transmission layer materials and electrode materials, developing budget alternative materials and feasible strategy to reduce the total cost is necessary. Apart from these three problems stated above, there are numerous challenges that the application of PSCs needs to face, nonetheless, we still consider 1D/3D mixed-dimensional strategy as prospective method to improve the efficiency and stability of PSCs simultaneously, thus leading to the large-scale application of PSCs in the future.
Author contributions
XZ: Writing–original draft. XL: Writing–review and editing. FW: Writing–review and editing. YX: Writing–review and editing. HL: Writing–review and editing. HH: Writing–review and editing.
Funding
The authors declare financial support was received for the research, authorship, and/or publication of this article. HH thanks the support from Scientific Research Startup Fund for Shenzhen High-Caliber Personnel of Shenzhen Polytechnic (No. 6022310038k).
Conflict of interest
The authors declare that the research was conducted in the absence of any commercial or financial relationships that could be construed as a potential conflict of interest.
Publisher’s note
All claims expressed in this article are solely those of the authors and do not necessarily represent those of their affiliated organizations, or those of the publisher, the editors and the reviewers. Any product that may be evaluated in this article, or claim that may be made by its manufacturer, is not guaranteed or endorsed by the publisher.
References
Bai, S., Da, P., Li, C., Wang, Z., Yuan, Z., Fu, F., et al. (2019). Planar perovskite solar cells with long-term stability using ionic liquid additives. Nature 571, 245–250. doi:10.1038/s41586-019-1357-2
Bartel, C. J., Sutton, C., Goldsmith, B. R., Ouyang, R., Musgrave, C. B., Ghiringhelli, L. M., et al. (2019). New tolerance factor to predict the stability of perovskite oxides and halides. Sci. Adv. 5, eaav0693. doi:10.1126/sciadv.aav0693
Best (2023). NREL. Best research-cell efficiency chart. Available at: www.nrel.gov/best-research-cell-efficiencies. https://www.nrel.gov/pv/assets/pdfs/best-research-cell-efficiencies.
Bi, D., Gao, P., Scopelliti, R., Oveisi, E., Luo, J., Grätzel, M., et al. (2016). High-performance perovskite solar cells with enhanced environmental stability based on amphiphile-modified CH3NH3PbI3. Adv. Mat. 28, 2910–2915. doi:10.1002/adma.201505255
Bu, T., Li, J., Li, H., Tian, C., Su, J., Tong, G., et al. (2021). Lead halide-templated crystallization of methylamine-free perovskite for efficient photovoltaic modules. Science 372, 1327–1332. doi:10.1126/science.abh1035
Chen, Q., Deng, K., Shen, Y., and Li, L. (2022a). Stable one dimensional (1D)/three dimensional (3D) perovskite solar cell with an efficiency exceeding 23%. InfoMat 4, 1–9. doi:10.1002/inf2.12303
Chen, R., Shen, H., Chang, Q., Tang, Z., Nie, S., Chen, B., et al. (2022b). Conformal imidazolium 1D perovskite capping layer stabilized 3D perovskite films for efficient solar modules. Adv. Sci. 9, e2204017. doi:10.1002/advs.202204017
Chen, Y., Liu, B., Zhou, Q., Ma, D., Han, X., He, D., et al. (2023a). Critical role of 1D materials in realizing efficient and stable perovskite solar cells. J. Mat. Chem. A 11, 18592–18604. doi:10.1039/D3TA03174H
Chen, Y., Liu, B., Zhou, Q., Ma, D., Han, X., He, D., et al. (2023b). Critical role of 1D materials in realizing efficient and stable perovskite solar cells. J. Mat. Chem. A 11, 18592–18604. doi:10.1039/D3TA03174H
Chen, Z., Cheng, Q., Chen, H., Wu, Y., Ding, J., Wu, X., et al. (2023c). Perovskite grain-boundary manipulation using room-temperature dynamic self-healing “ligaments” for developing highly stable flexible perovskite solar cells with 23.8% efficiency. Adv. Mater 35, e2300513. doi:10.1002/adma.202300513
Chu, Z., Chu, X., Zhao, Y., Ye, Q., Jiang, J., Zhang, X., et al. (2021). Emerging low-dimensional crystal structure of metal halide perovskite optoelectronic materials and devices. Small Struct. 2, 2000133. doi:10.1002/sstr.202000133
Elsenety, M. M., Antoniadou, M., Balis, N., Kaltzoglou, A., Sygellou, L., Stergiou, A., et al. (2020). Stability improvement and performance reproducibility enhancement of perovskite solar cells following (FA/MA/Cs)PbI3- xBrx/(ch3)3sPbI3 dimensionality engineering. ACS Appl. Energy Mat. 3, 2465–2477. doi:10.1021/acsaem.9b02117
Fan, J., Ma, Y., Zhang, C., Liu, C., Li, W., Schropp, R. E. I., et al. (2018). Thermodynamically self-healing 1D–3D hybrid perovskite solar cells. Adv. Energy Mater 8. doi:10.1002/aenm.201703421
Fu, W., Liu, H., Shi, X., Zuo, L., Li, X., and Jen, A. K.-Y. (2019). Tailoring the functionality of organic spacer cations for efficient and stable quasi-2D perovskite solar cells. Adv. Funct. Mat. 29, 1900221. doi:10.1002/adfm.201900221
Gao, L., Hu, P., and Frank) Liu, S. (2023). Low-dimensional perovskite modified 3D structures for higher-performance solar cells. J. Energy Chem. 81, 389–403. doi:10.1016/j.jechem.2023.01.061
Gao, L., Spanopoulos, I., Ke, W., Huang, S., Hadar, I., Chen, L., et al. (2019). Improved environmental stability and solar cell efficiency of (MA,FA)PbI3 perovskite using a wide-band-gap 1D thiazolium lead iodide capping layer strategy. ACS Energy Lett. 4, 1763–1769. doi:10.1021/acsenergylett.9b00930
Ge, C., Lu, J.-F., Singh, M., Ng, A., Yu, W., Lin, H., et al. (2022). Mixed dimensional perovskites heterostructure for highly efficient and stable perovskite solar cells. Sol. RRL 6. doi:10.1002/solr.202100879
Heo, S., Seo, G., Lee, Y., Seol, M., Kim, S. H., Yun, D. J., et al. (2019). Origins of high performance and degradation in the mixed perovskite solar cells. Adv. Mat. 31, e1805438–5. doi:10.1002/adma.201805438
Hong, K., Le, Q. V., Kim, S. Y., and Jang, H. W. (2018). Low-dimensional halide perovskites: review and issues. J. Mat. Chem. C 6, 2189–2209. doi:10.1039/C7TC05658C
Jeong, J., Kim, M., Seo, J., Lu, H., Ahlawat, P., Mishra, A., et al. (2021). Pseudo-halide anion engineering for α-FAPbI3 perovskite solar cells. Nature 592, 381–385. doi:10.1038/s41586-021-03406-5
Jeong, M., Choi, I. W., Go, E. M., Cho, Y., Kim, M., Lee, B., et al. (2020). Stable perovskite solar cells with efficiency exceeding 24.8% and 0.3-V voltage loss. Science 369, 1615–1620. doi:10.1126/science.abb7167
Kim, B., and Seok, S.Il (2020). Molecular aspects of organic cations affecting the humidity stability of perovskites. Energy Environ. Sci. 13, 805–820. doi:10.1039/C9EE03473K
Koh, T. M., Shanmugam, V., Schlipf, J., Oesinghaus, L., Müller-Buschbaum, P., Ramakrishnan, N., et al. (2016). Nanostructuring mixed-dimensional perovskites: a route toward tunable, efficient photovoltaics. Adv. Mat. 28, 3653–3661. doi:10.1002/adma.201506141
Kojima, A., Teshima, K., Shirai, Y., and Miyasaka, T. (2009). Organometal halide perovskites as visible-light sensitizers for photovoltaic cells. J. Am. Chem. Soc. 131, 6050–6051. doi:10.1021/ja809598r
Kong, T., Xie, H., Zhang, Y., Song, J., Li, Y., Lim, E. L., et al. (2021). Perovskitoid-Templated formation of a 1D@3D perovskite structure toward highly efficient and stable perovskite solar cells. Adv. Energy Mat. 11, 2101018. doi:10.1002/aenm.202101018
Li, M., Zhou, J., Tan, L., Li, H., Liu, Y., Jiang, C., et al. (2022a). Multifunctional succinate additive for flexible perovskite solar cells with more than 23% power-conversion efficiency. Innov 3, 100310. doi:10.1016/j.xinn.2022.100310
Li, Z., Li, B., Wu, X., Sheppard, S. A., Zhang, S., Gao, D., et al. (2022b). Organometallic-functionalized interfaces for highly efficient inverted perovskite solar cells. Science 376, 416–420. doi:10.1126/science.abm8566
Li, Z., Yang, M., Park, J.-S., Wei, S.-H., Berry, J. J., and Zhu, K. (2016). Stabilizing perovskite structures by tuning tolerance factor: formation of formamidinium and cesium lead iodide solid-state alloys. Chem. Mat. 28, 284–292. doi:10.1021/acs.chemmater.5b04107
Lin, H., Zhou, C., Tian, Y., Siegrist, T., and Ma, B. (2018). Low-dimensional organometal halide perovskites. ACS Energy Lett. 3, 54–62. doi:10.1021/acsenergylett.7b00926
Liu, Y., Yuan, S., Zheng, H., Wu, M., Zhang, S., Lan, J., et al. (2023). Structurally dimensional engineering in perovskite photovoltaics. Adv. Energy Mat. 13. doi:10.1002/aenm.202300188
Long, Y., Xian, Y., Yuan, S., Liu, K., Sun, M., Guo, Y., et al. (2021). π-π conjugate structure enabling the channel construction of carrier-facilitated transport in 1D–3D multidimensional CsPbI2Br solar cells with high stability. Nano Energy 89, 106340. doi:10.1016/j.nanoen.2021.106340
Meng, Y., Zhang, W., Zhang, X., Deng, Y., Zhang, H., Zeng, G., et al. (2023). Self-assembled 1D/3D perovskite heterostructure for stable all-air-processed perovskite solar cells with improved open-circuit voltage. ChemSusChem 16, e202300257. doi:10.1002/cssc.202300257
Milić, J. V., Im, J., Kubicki, D. J., Ummadisingu, A., Seo, J., Li, Y., et al. (2019). Supramolecular engineering for formamidinium-based layered 2D perovskite solar cells: structural complexity and dynamics revealed by solid-state NMR spectroscopy. Adv. Energy Mat. 9, 1900284. doi:10.1002/aenm.201900284
Min, H., Lee, D. Y., Kim, J., Kim, G., Lee, K. S., Kim, J., et al. (2021). Perovskite solar cells with atomically coherent interlayers on SnO2 electrodes. Nature 598, 444–450. doi:10.1038/s41586-021-03964-8
Nazir, G., Lee, S., Lee, J., Rehman, A., Lee, J., Seok, S.Il, et al. (2022). Stabilization of perovskite solar cells: recent developments and future perspectives. Adv. Mat. 34, 2204380. doi:10.1002/adma.202204380
Ni, Z., Xu, S., Jiao, H., Gu, H., Fei, C., and Huang, J. (2022). High grain boundary recombination velocity in polycrystalline metal halide perovskites. Sci. Adv. 8, eabq8345. doi:10.1126/sciadv.abq8345
Paek, S., Roldán-Carmona, C., Cho, K. T., Franckevičius, M., Kim, H., Kanda, H., et al. (2020). Molecular design and operational stability: toward stable 3D/2D perovskite interlayers. Adv. Sci. 7, 2001014. doi:10.1002/advs.202001014
Qian, J., Guo, Q., Liu, L., Xu, B., and Tian, W. (2017). A theoretical study of hybrid lead iodide perovskite homologous semiconductors with 0D, 1D, 2D and 3D structures. J. Mat. Chem. A 5, 16786–16795. doi:10.1039/C7TA04008C
Stoumpos, C. C., Mao, L., Malliakas, C. D., and Kanatzidis, M. G. (2017). Structure–band gap relationships in hexagonal polytypes and low-dimensional structures of hybrid tin iodide perovskites. Inorg. Chem. 56, 56–73. doi:10.1021/acs.inorgchem.6b02764
Wang, D., Wright, M., Elumalai, N. K., and Uddin, A. (2016). Stability of perovskite solar cells. Sol. Energy Mat. Sol. Cells 147, 255–275. doi:10.1016/j.solmat.2015.12.025
Wang, F., Duan, D., Zhou, K., Xue, Y. Z. B., Liang, X., Zhou, X., et al. (2023). Ionic liquid engineering enabled in-plane orientated 1D perovskite nanorods for efficient mixed-dimensional perovskite photovoltaics. InfoMat 5. doi:10.1002/inf2.12459
Wang, J., Liu, L., Chen, S., Qi, L., Zhao, M., Zhao, C., et al. (2022a). Growth of 1D nanorod perovskite for surface passivation in FAPbI3 perovskite solar cells. Small 18, e2104100. doi:10.1002/smll.202104100
Wang, Q., Chen, B., Liu, Y., Deng, Y., Bai, Y., Dong, Q., et al. (2017). Scaling behavior of moisture-induced grain degradation in polycrystalline hybrid perovskite thin films. Energy Environ. Sci. 10, 516–522. doi:10.1039/c6ee02941h
Wang, T., Zhang, Y., Kong, W., Qiao, L., Peng, B., Shen, Z., et al. (2022b). Transporting holes stably under iodide invasion in efficient perovskite solar cells. Science 377, 1227–1232. doi:10.1126/science.abq6235
Wei, N., Chen, Y., Wang, X., Miao, Y., Qin, Z., Liu, X., et al. (2022). Multi-level passivation of MAPbI3 perovskite for efficient and stable photovoltaics. Adv. Funct. Mat. 32, 1–7. doi:10.1002/adfm.202108944
Xu, A. F., Liu, N., Xie, F., Song, T., Ma, Y., Zhang, P., et al. (2020). Promoting thermodynamic and kinetic stabilities of fa-based perovskite by an in situ bilayer structure. Nano Lett. 20, 3864–3871. doi:10.1021/acs.nanolett.0c00988
Yang, J., He, T., Li, M., Li, G., Liu, H., Xu, J., et al. (2022). π-Conjugated carbazole cations enable wet-stable quasi-2D perovskite photovoltaics. ACS Energy Lett. 7, 4451–4458. doi:10.1021/acsenergylett.2c02219
Yang, N., Zhu, C., Chen, Y., Zai, H., Wang, C., Wang, X., et al. (2020a). An: in situ cross-linked 1D/3D perovskite heterostructure improves the stability of hybrid perovskite solar cells for over 3000 h operation. Energy Environ. Sci. 13, 4344–4352. doi:10.1039/d0ee01736a
Yang, R., Li, R., Cao, Y., Wei, Y., Miao, Y., Tan, W. L., et al. (2018). Oriented quasi-2D perovskites for high performance optoelectronic devices. Adv. Mat. 30, 1804771. doi:10.1002/adma.201804771
Yang, Z., Babu, B. H., Wu, S., Liu, T., Fang, S., Xiong, Z., et al. (2020b). Review on practical interface engineering of perovskite solar cells: from efficiency to stability. Sol. RRL 4, 1900257. doi:10.1002/solr.201900257
Yao, D., Hoang, M. T., and Wang, H. (2021). Low-dimensional-networked perovskites with A-site-cation engineering for optoelectronic devices. Small Methods 5, 2001147. doi:10.1002/smtd.202001147
Yoo, J. J., Seo, G., Chua, M. R., Park, T. G., Lu, Y., Rotermund, F., et al. (2021). Efficient perovskite solar cells via improved carrier management. Nature 590, 587–593. doi:10.1038/s41586-021-03285-w
Yu, S., Liu, H., Wang, S., Zhu, H., Dong, X., and Li, X. (2021). Hydrazinium cation mixed FAPbI3-based perovskite with 1D/3D hybrid dimension structure for efficient and stable solar cells. Chem. Eng. J. 403, 125724. doi:10.1016/j.cej.2020.125724
Yuan, Y., and Huang, J. (2016). Ion migration in organometal trihalide perovskite and its impact on photovoltaic efficiency and stability. Acc. Chem. Res. 49, 286–293. doi:10.1021/acs.accounts.5b00420
Zhan, Y., Yang, F., Chen, W., Chen, H., Shen, Y., Li, Y., et al. (2021a). Elastic lattice and excess charge carrier manipulation in 1D–3D perovskite solar cells for exceptionally long-term operational stability. Adv. Mat. 33, e2105170. doi:10.1002/adma.202105170
Zhang, H., Shi, Z., Hu, L., Tang, Y. Y., Qin, Z., Liao, W. Q., et al. (2021). Highly efficient 1D/3D ferroelectric perovskite solar cell. Adv. Funct. Mat. 31. doi:10.1002/adfm.202100205
Zhang, J., Zhang, G., Su, P., Huang, R., Lin, J., Wang, W., et al. (2023). 1D choline-PbI 3 -based heterostructure boosts efficiency and stability of CsPbI 3 perovskite solar cells. Angew. Chem. Int. Ed. 62, e202303486. doi:10.1002/anie.202303486
Zhao, Y., Xiang, H., Ran, R., Zhou, W., Wang, W., and Shao, Z. (2023). Beyond two-dimension: one- and zero-dimensional halide perovskites as new-generation passivators for high-performance perovskite solar cells. J. Energy Chem. 83, 189–208. doi:10.1016/j.jechem.2023.04.025
Zheng, H., Ghosh, A., Swamynadhan, M. J., Wang, G., Zhang, Q., Wu, X., et al. (2023). Electron spin decoherence dynamics in magnetic manganese hybrid organic–inorganic crystals: the effect of lattice dimensionality. J. Am. Chem. Soc. 145, 18549–18559. doi:10.1021/jacs.3c05503
Zhou, C., Tian, Y., Wang, M., Rose, A., Besara, T., Doyle, N. K., et al. (2017). Low-dimensional organic tin bromide perovskites and their photoinduced structural transformation. Angew. Chem. Int. Ed. 56, 9018–9022. doi:10.1002/anie.201702825
Keywords: long-chain organic molecules, 1D/3D mixed-dimensional perovskite, perovskite solar cell (PSC), long-term stability, low dimensional perovskite
Citation: Zhou X, Wang T, Liang X, Wang F, Xu Y, Lin H, Hu R and Hu H (2024) Long-chain organic molecules enable mixed dimensional perovskite photovoltaics: a brief view. Front. Chem. 11:1341935. doi: 10.3389/fchem.2023.1341935
Received: 21 November 2023; Accepted: 29 December 2023;
Published: 11 January 2024.
Edited by:
Guangshe Li, Jilin University, ChinaReviewed by:
Jien Yang, Henan Normal University, ChinaCopyright © 2024 Zhou, Wang, Liang, Wang, Xu, Lin, Hu and Hu. This is an open-access article distributed under the terms of the Creative Commons Attribution License (CC BY). The use, distribution or reproduction in other forums is permitted, provided the original author(s) and the copyright owner(s) are credited and that the original publication in this journal is cited, in accordance with accepted academic practice. No use, distribution or reproduction is permitted which does not comply with these terms.
*Correspondence: Hanlin Hu, hanlinhu@szpu.edu.cn; Yan Xu, xycuba@163.com
†These authors have contributed equally to this work