- 1A School of Pharmacy, Anhui University of Chinese Medicine, Hefei, China
- 2College of Traditional Chinese Medicine, Bozhou University, Bozhou, China
- 3MOE-Anhui Joint Collaborative Innovation Center for Quality Improvement of Anhui Genuine Chinese Medicinal Materials, Hefei, China
- 4Anhui Province Rural Revitalization Collaborative Technology Service Center, Hefei, China
- 5Anhui Province Modern Chinese Medicine Industry Common Technology Research Center, Heifei, China
- 6Anhui Province Key Laboratory of Pharmaceutical Preparation Technology and Application, Heifei, Anhui, China
Introduction: Ginger (Zingiber officinale Roce.) is a widely consumed food item and a prominent traditional Chinese medicinal herb. The intrinsic quality of ginger may differ due to variations in its origin and processing techniques. To evaluate the quality of ginger, a straightforward and efficient discriminatory approach has been devised, utilizing 6-gingerol, 8-gingerol, and 10-gingerol as benchmarks.
Methods: In order to categorize ginger samples according to their cultivated origins with different longitude and latitude (Shandong, Anhui, and Yunnan provinces in China) and processing methods (liquid nitrogen pulverization, ultra-micro grinding, and mortar grinding), similarity analysis (SA), hierarchical cluster analysis (HCA), and principal component analysis (PCA) were employed. Furthermore, there was a quantitative determination of the significant marker compounds gingerols, which has considerable impact on maintaining quality control and distinguishing ginger products accurately. Moreover, discrimination analysis (DA) was utilized to further distinguish and classify samples with unknown membership degrees based on the eigenvalues, with the aim of achieving optimal discrimination between groups.
Results: The findings obtained from the high-performance liquid chromatography (HPLC) data revealed that the levels of various gingerols present in all samples exhibited significant variations. The study confirmed that the quality of ginger was primarily influenced by its origin and processing method, with the former being the dominant factor. Notably, the sample obtained from Anhui province and subjected to liquid nitrogen pulverization demonstrated the highest content of gingerols.
Conclusion: The results obtained from the analysis of SA, HCA, PCA, and DA were consistent and could be employed to evaluate the quality of ginger. As such, the combination of HPLC fingerprints and chemo metric techniques provided a dependable approach for comprehensively assessing the quality and processing of ginger.
Highlights
Discriminant analysis of ginger source by 6-gingerol, 8-gingerol, 10 gingerol.
Different processing methods can be employed to address the problem of ginger’s loss of active ingredients during utilization or storage.
The fingerprint spectrum of ginger derived from different production areas was established through the use of HPLC and similarity software.
1 Introduction
Ginger (Zingiber officinale Roce.) is the rhizome of the ginger family and belongs to the perennial herb (Shukla and Singh, 2007) Ginger originates in Asia. Presently, this plant is commonly cultivated in tropical regions around the world as a spice and dietary supplement (Peng et al., 2017). Additionally, ginger is highly sought after in the perfume industry due to its abundance of volatile essential oils (Marak et al., 2019). As a result of the copious amounts of vitamins contained within ginger, it is commonly utilized as a flavoring component in candies and beverages (Sangwan et al., 2014). As per the research findings, the pungent taste of ginger is a result of the presence of shogaols and gingerols. Moreover, the unique flavor of ginger can be attributed to the volatility and pungency of its essential oil (Yamaguchi et al., 2010). Simultaneously, ginger is also a well-known traditional Chinese medicine (TCM) that has been widely utilized in clinical settings due to its medicinal and nutritional properties. As a medicine-food homology, ginger possesses various health benefits such as bacteriostasis, detoxification, anti-inflammatory properties, hypolipidemia, anti-oxidant properties, and tumor suppression (Shanmugam et al., 2011; Funk et al., 2016; Kim et al., 2018; Mahomoodally et al., 2021). The advantageous health properties of ginger have been attributed to an array of functional components, including gingerol, shogaol, phenolic elements, purine compounds, ginger oil, volatile oils, active polysaccharides, and glycoproteins (Levita et al., 2018). The primary constituent of ginger is gingerol, which comprises 6-gingerol, 8-gingerol, and 10-gingerol, with 6-gingerol constituting over 75% of the total composition (Liu et al., 2019). Gingerols convert into their corresponding shogaols after being subjected to long-term storage or heat treatment, resulting in the manifestation of high levels of antioxidant activity (Ballester et al., 2022). Nevertheless, the constituents and composition of ginger were substantially influenced by elements such as localities, climates, and soil environments (Gaur et al., 2016). These factors directly affect the unique flavor of ginger and its medical and health effects. The specific conditions of cultivation and various processing methods are crucial factors in determining the active constituents that define its distinct characteristics (Chen et al., 2019). In light of the consistent increase in the utilization and consumption of ginger, it is of utmost importance to establish an appropriate methodology for the development of quality control measures. The Chinese Pharmacopoeia (China Pharmacopoeia Committee, 2020) is identified 6-gingerol as the marker compound for evaluating the quality of ginger. However, ginger is composed of a multitude of intricate constituents beyond this singular compound. Consequently, relying solely on one component as the standard for quality evaluation is inadequate. It is therefore imperative to include more effective ingredients in the evaluation process, rather than relying on a single compound.
The utilization of chemometric methodologies, particularly principal component analysis (PCA) and hierarchical cluster analysis (HCA), has facilitated the systematic acquisition of chemical profiles that have a broad range of applications in the classification and identification of medicinal compounds. These techniques have been extensively employed in the analysis of the chemical composition of herbal medicines (Guo et al., 2018). Furthermore, the application of high-performance liquid chromatography (HPLC) in combination with multivariate statistical methods has been utilized to discriminate and classify various constituents (Miao et al., 2019). Chromatographic fingerprinting was considered as a reliable method to evaluate the quality of traditional Chinese medicine (Cui et al., 2016). In addition, the TCM fingerprint is internationally recognized for the evaluation and quality control of TCM and related products.
To summarize, the constituents of gingerols involved in processing have the potential to greatly affect the ultimate innate excellence of ginger. The established methods for evaluating quality do not suffice in meeting the needs of industry progress and quality appraisal. The present study employs a variety of chemometric methods, such as SA, HCA, PCA, and DA, to analyze the HPLC fingerprint profiles of ginger, with a particular emphasis on the active substances of 6, 8, and 10-gingerols, in order to evaluate the quality of ginger. Furthermore, standard samples were utilized to determine the contents of markers. Through the integration of stoichiometric techniques and fingerprint analysis, the influence of the production area and processing processes on the intrinsic quality of ginger was systematically examined.
2 Materials and methods
2.1 Chemicals
Ginger reference substance: 6-gingerol (lot number: 111833–201806, purity >99.9%) was purchased from National Institutes for Food and Drug Control, 8-gingerol (lot number: CHB 180305, purity >98%) and 10-gingerol (lot number: CHB 180311, purity >98%) were purchased from Chengdu Chroma-Biotech Co., Ltd. Their structures were shown in Figure 1. The deionized water was generated by a laboratory water purification system (HITECH Instruments CO., Ltd). The acetic acid was of analytical grade purchased from Shanghai Runjie Chemical Reagent Co. Ltd. Other chemicals, like methanol (lot number: 34860-4L-R) and acetonitrile (lot number: 134752), were HPLC grade and obtained by Sigma-Aldrich Shanghai Co, Ltd. and Beijing J&K Scientific Ltd., respectively.
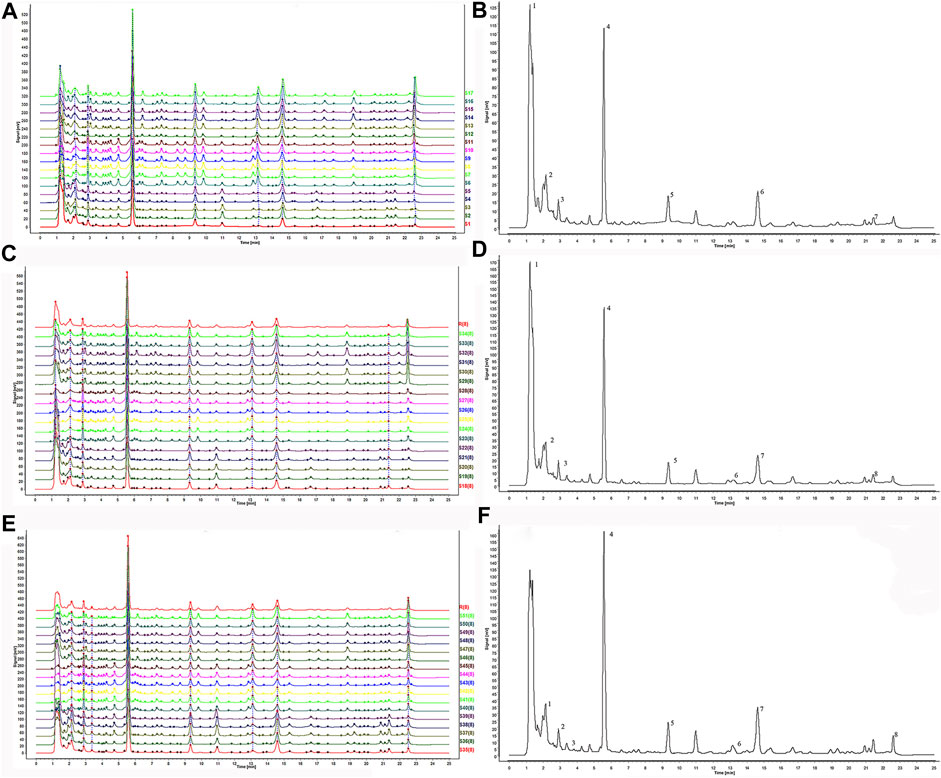
FIGURE 1. The HPLC fingerprints of Zingiber officinale Roce. and the characteristic spectrogram for the chromatographic fingerprint of Zingiber officinale Roce. (A) The fingerprints of superfine crush pre-treated ginger. (B) The reference standard fingerprint of superfine crush pre-treated ginger. (C) The fingerprints of grinding pretreatment ginger. (D) The reference standard fingerprints of grinding pretreatment ginger. (E) The fingerprints of liquid nitrogen freeze mill pre-treated ginger. (F) The reference standard fingerprints of liquid nitrogen freeze mill pre-treated ginger.
2.2 Ginger samples
Three kinds of ginger samples were collected from the south (Jietou town, Qujing city, Yunnan province), middle (Tianmen Town, Tongling City, Anhui Province) and north (Tancheng County, Linyi City, Shandong Province) of China, respectively (Table 1). In our work, 5 batches of ginger from Shandong, 6 batches of ginger from Anhui and 6 batches of ginger from Yunnan were used in the experiment. Professor Xie Dongmei has authenticated all samples from the Department of Pharmacy of Anhui University of Chinese medicine (Anhui, China). They were identified as the dried rhizome of ginger and complied with official Chinese pharmacopeia (China Pharmacopoeia Committee, 2020).
2.3 Sample preparation
First of all, scissors were used to cut haphazardly selected ginger from every origin into thin strips. Then strips were pulverized to powder samples through the following three methods (Table 1). 1) Strips were chilled into liquid nitrogen, then pulverized with a magnetically driven impactor to let it turn into a fine powder. This experiment was performed by the SPEX SamplePrep’s Freezer/Mills. The instrument condition was as follows: run time, 1 min; cycles, 2; rate, 8 cps. 2) Microchips were put into the ST-528 Ultra-micro Crusher (Ruian saite electromechanical Co., Ltd.) and removed the fine powder that flies into the upper container. 3) Ginger was placed into the mortar and ground it manually in one direction. According to the requirements of Chinese Pharmacopoeia 2015, powders passed through 50 mesh sieves were selected. All of the steps were performed at 25°C.
The scale was used in one out of 10,000 g of precision. The ginger powder was precisely weighted about 0.25 g and placed in a core-shaped flask. Moreover, the powder was added with 75% chromatogram grade methanol (20 mL). The total weight of the weighing samples and container were weighed and written down. After 40 min of ultrasonic treatment, with 30°C water in the power of 300 W and the frequency was 40 kHz. After ultrasonic, the ginger extracting solution was naturally cooled. The whole weight was weighed again to make up the lost weight with 75% chromatogram grade methanol. Finally, the solution was filtrated through a 0.22 μm microporous filter membrane and the filtration was taken as the test solution. All solutions and powders were placed in a light-proof sealed plastic bag, numbered and stored in the refrigerator at −20°C.
2.4 Apparatus and chromatographic conditions and optimization
The Waters Acquity UPLC™ system was equipped with an auto-sample, a binary pump, a degasser, the 4-channel solvent degasser integrated into the pump module, UV-Vis variable wavelength detector, thermostated column compartment, and connected to Waters Empower 2 software. A reverse-phase column (C18 analytical column, 4.6 mm × 150 mm, 5 μm, WondaCract ODS-2, Japan) was carried out in the analysis. The best separation effect was obtained in the chromatogram.
By comparing different mobile phase compositions and different mobile phase proportions and gradient conditions, the optimal choice was finally determined. The mobile phase was composed of water containing 0.1% (v/v) acetic acid (A) and acetonitrile (B). The gradient program for the HPLC was as follows: 0–15 min, 51%–70% B; 15–20 min, 70%–90% B; 20–21 min, 90%–51% B; 21–24 min, 51% B. The column and the auto-sampler temperature were maintained at 25°C. The sample injection volume was 20 μL. The UV spectrum was performed and peak absorbance was detected at 280 nm.
2.5 Chemometrics analysis
2.5.1 Similarity analysis
Chromatograms can make representative of all samples and have the characteristics of completeness and ambiguity. By analyzing the mutual mode of the chromatogram, the sample could be well-identified and analyzed. The contents of several chemical components were different. It is necessary to find possible quality markers to identify and analyze different samples (Tan et al., 2018). Ginger, in the same pretreatment, was categorized into a team. The HPLC chromatograms of the ginger were exported as CDF files. They imported them into a software named Similarity Evaluation System for Chromatographic Fingerprint of Traditional Chinese Medicine. The software was validated and supplied by the Chinese Pharmacopoeia Committee (Version 2012.130723; Beijing, China) and analyzed the data under optimal conditions. The software calculates the mean values and generates mock chromatograms which were used as representative standard chromatograms for the ginger samples. Correlation coefficients and similarities between the means were calculated for each mock chromatogram.
2.5.2 Hierarchical cluster analysis
Hierarchical cluster analysis (HCA) was a multivariate analysis technique that categorized samples to show the degree of correlation between a large number of samples (L et al., 2011). Each object was the same as the other objects in a group but differed from the other objects regarding predetermined selection criteria (Kong et al., 2009). Based on the subjective comparison, HCA was used to evaluate the chromatographic peaks areas of active ingredients.
2.5.3 Principal component analysis
Principal component analysis (PCA) can remove redundant information, reduce multidimensional data to lower dimensions, and highlight hidden features (Liu et al., 2016; Chen et al., 2017; Guo et al., 2018; Ghisoni et al., 2019). Here, SIMCA software was used to evaluate and select the similarity evaluations, get more accurate and detailed fingerprint information. By importing pre-processed fingerprints spectrum data to the SIMCA system, quality control and ginger evaluation from different origins were established.
2.5.4 Discriminant analysis
To further trace the origin of ginger and realize the source identification of ginger. Discriminant analysis (DA) was a standard method to identify the sample type in multivariate statistics. Fisher discrimination was used in Statistical Product and Service Solutions (SPSS).
3 Results and discussion
3.1 Establishment of HPLC fingerprint
The proportion of the acetonitrile phase was decreased to make the peak time earlier. Under the conditions reported in the literature, acetic acid was added to the water phase. The degree of sample separation was tested at different concentrations of the acetonitrile and water phase. Finally, acetonitrile and 0.1% acetic acid were selected as the mobile phase. Gradient elution displayed a better resolution and made each component peak well separated from adjacent peaks.
The similarity evaluation system had excellent resolution and large areas in the HPLC fingerprints, which could be regarded as the reference chromatogram to identify ginger. According to the fingerprints analysis, seven common peaks were demarcated from superfine samples (Figure 1A). Eight peaks were determined from grinding and liquid nitrogen samples of 17 batches of ginger, respectively (Figures 1C, E). In the three treatments, the common peaks obtained all contain 6-gingerol, 8-gingerol, and 10-gingerol.
3.2 Similarity analysis
The system separately compared all the 17 batches of each team (Figures 1A, C, E) to generate a reference chromatogram. The median method was utilized to compare the similarities between the entire and reference chromatogram. Differences in correlation coefficients indicate the variation of the fingerprint and quality of these samples (Zhong et al., 2015; Li et al., 2016). It indicated that it is necessary to identify all the possible quality markers to distinguish and analyze different samples. In this study, the correlation coefficient close to 1.0 indicated that the samples were entirely similar by multivariate analyzing score and loading.
Table 1 showed correlation coefficients of 17 samples of ginger with different pretreatments, respectively. As shown in the similarity of grinding samples (Supplementary Table S1A), S6 and S7, S6 and S8, S6 and S9, S6, and S10 showed a high degree of similarity. S4 and S6, S4 and S7, S4 and S8, S4 and S9, S4, and S10 had low similarity (about 0.42). In Figure 1B, 7 related peaks may affect the quality of ginger. Peaks 2, 4, 5, 6, and 7 with the highest content as characteristic peaks were significant for quality control of ginger fingerprints. Meanwhile, similar characteristic peaks were obtained in the grinding samples. In Supplementary Table S1B, S23 and S24, S23, and S25, S23, and S27 correlated the grinding samples. There were eight peaks with the highest content in S23, which might affect the quality of samples. In contrast, the correlation between S20 and S23, S20 and S24, S20 and S25, and S20 and S27 were lower than other batches. Apparently, the contents of peak 4 in S20 were significantly higher than that of S23, 24, 25, and 27. Meanwhile, the content of peaks 5 and 7 of S20 was markedly higher than that of S23, S24, S25, and S27. As the control spectrum of grinding pretreatment samples shows (Figure 1D), peaks 4, 5, 7, and other characteristic peaks had crucial implications for quality control. Meanwhile, liquid nitrogen samples (Supplementary Table S1C) showed that S50 and S46, S50 and S48, S50, and S49, S50, and S51 had higher correlations. On the contrary, correlations between S35 and S42, S35, and S43, S35, and S44, S35, and S45 were lower than other batches. The content of peaks 2, 4, and 5 in S50 was lower than in other samples. The contents of peaks 2, 5, and 7 in S35 were quite different from those in S42, S43, and S45. It was observed that peaks 2, 4, 5, and 7 could significantly affect the quality of gingers (Figure 1F).
The total content of the main component had an essential effect on the intrinsic quality of ginger through the analysis of similarity data. Meanwhile, some small elements also showed significant differences in different samples. It was noted that other origin areas and processing methods influenced the quality of ginger samples.
3.3 Hierarchical cluster analysis
The dendrogram plot defined three distinctive clusters. These results showed that HCA could effectively distinguish samples from the same origin with different extraction methods and classify them into three quality clusters based on morphological characteristics.
As shown in the scoring chart of ultramicro comminuted sample (Figure 2A), it could be classified into three separate groups. S6-S11 clustered into one category. The S13, S14, S16, S17 from Yunnan province were close to each other, they were regarded as one group. The profile of S12 and S15 were on the brink of S1-S5 samples. Therefore, S1-S5, S12, S15 were tended to be grouped into the same cluster. These inspired that the samples from Shandong and Yunnan province had similarities in chemical composition. Figures 2B, C showed the data of liquid nitrogen and grinding samples divided into three clusters based on different origins. According to the origins, 17 batches of samples were divided into three clusters strictly. This indicates that they differ in the content and distribution of the main chemical components. In Figure 3B, these were the compounds that separated S29-S34 as group 1. Group 2 was comprised of peak 18–22 and the samples were all collected from Shandong province. When it comes to the other six samples, S23-S28 from Anhui province was clustered in group 3. As shown in liquid nitrogen freezer mill pre-treated samples (Figure 2C), group 1 consisted of S35-S39 collected from Shandong province. Group 2 included samples S40-S45 collected from Anhui province. The extra six samples, S46-S51 from Anhui province, were clustered in group 3.
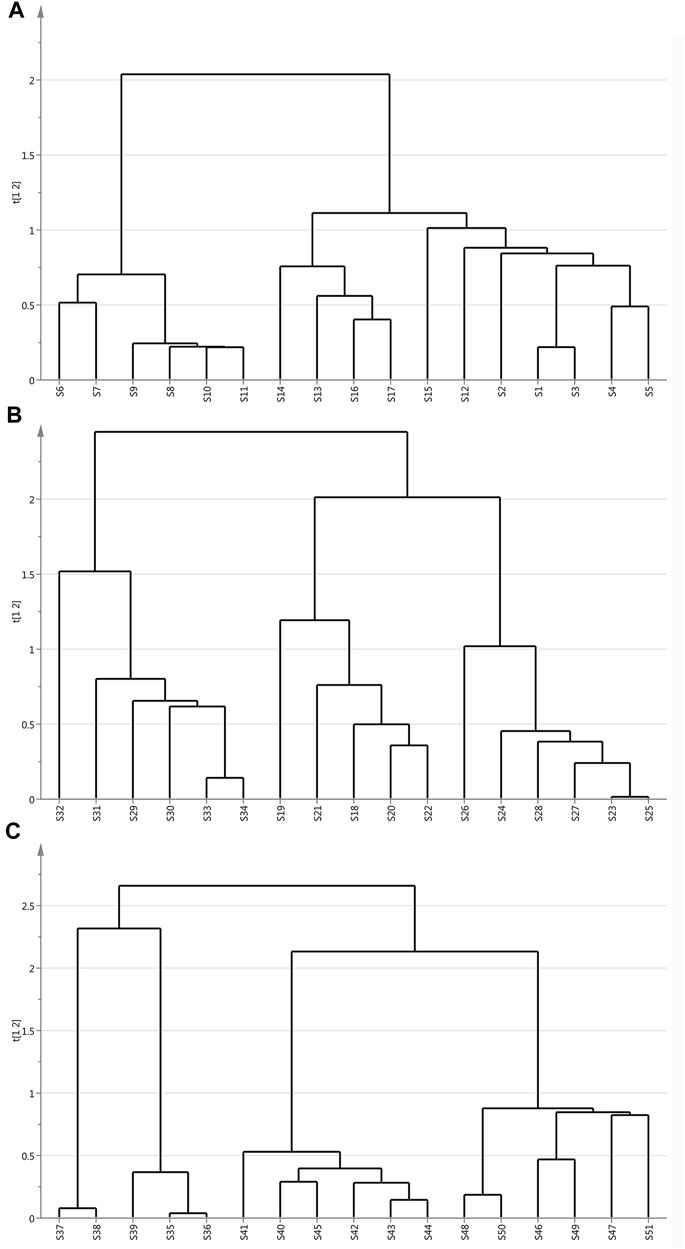
FIGURE 2. Dendrogram of ginger samples with different extraction methods: (A) Superfine crush pre-treated ginger. (B) Grinding pretreatment ginger. (C) Liquid nitrogen freeze mill pre-treated ginger.
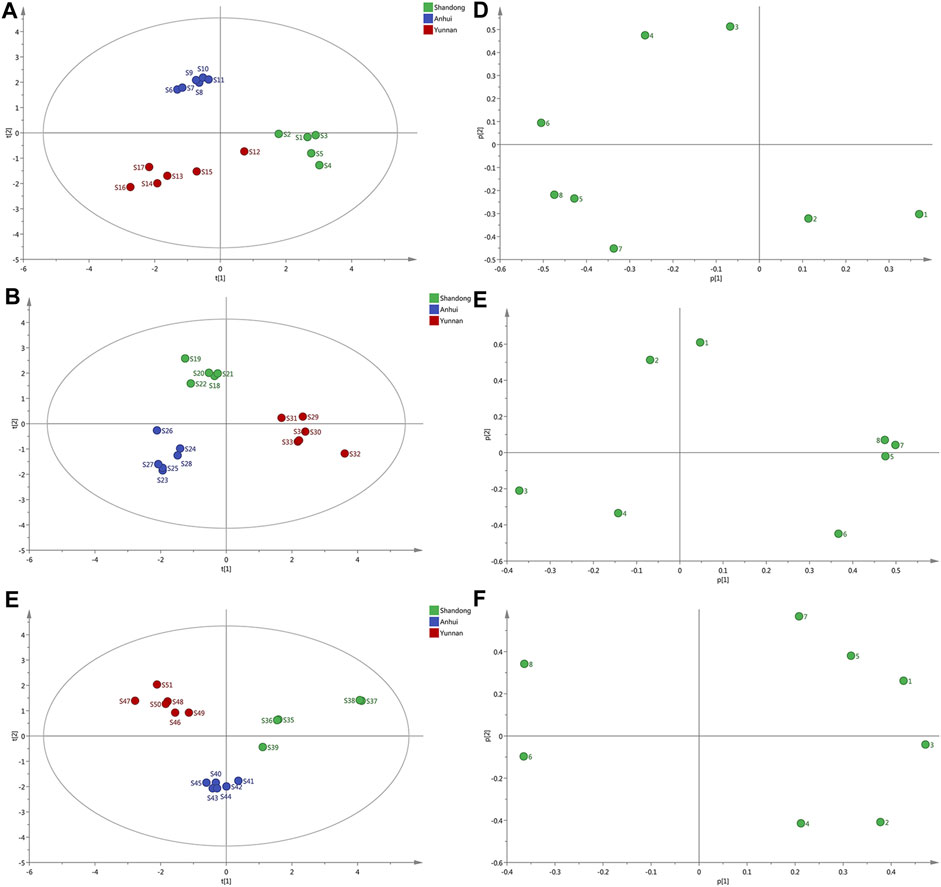
FIGURE 3. PCA score scatter plots and loading scatter plots for the samples. (A–C) The score scatter. Plots of ginger with different extraction methods from Shandong, Anhui, Yunnan, respectively. (D–F) The loadings plot of ginger with varying forms of extraction from Shandong, Anhui, Yunnan, respectively.
3.4 Principal component analysis
In Figure 3A, the samples could be visually classified into three separate groups according to different origins. S1-S5 were prone to be classified into one group. S6-S11 seemed to be grouped into the same cluster. What is more, S12-S17 were in the same group. The profile of S12 was closed to that of S1-S5 samples. To compare the result which obtained from both techniques, the PCA and HCA showed comparable results. Most samples with the same origin were aggregated into the same clusters. As shown in the PCA score plot (Figure 3B), the samples could be visually classified into three separate groups. S18-S22 (Shandong province) were in the same domain. S23-S28 (Anhui province) was also in the same domain. In comparison, the other six samples (S29-S34 from Yunnan province) were in the same domain. The dendrogram (Figure 3C) visibly showed that all samples were divided into three clusters. Five samples (S35-S39) from Anhui were classified into the same group. Indeed, samples from Anhui province (S40-S45) were clustered into the same group. The rest of the samples (S46-S51) formed a group. The results were consistent with their native place. It shows that the ginger from Anhui and the other two origins (Shandong and Yunnan province) were divided into three main categories.
The results of the principal component analysis were presented in the form of a score. The load focuses on multivariate changes that affect the differences between samples. Therefore, common peak areas of various resolved components were analyzed, and a data matrix of elements for samples was established (Kong et al., 2009). The peak areas of 8 components in each sample were the first and second PCs (PC1, PC2) for visually this new data matrix.
The loadings plot of ginger with different extraction was illustrated (Figure 3D–F). Analysis of the loading plot of PC1 against PC2 (Figure 3D) revealed that peaks contributing to PCA influenced the cluster in top-down order. Peaks 3, 4, and 6 that mainly contributed to PC2 classified S6-S11 as the same group. In summary, since PC1 contributing fewer peaks than PC2, samples on the left side of Figure 3D were presumed higher quality. Accordingly, ginger collected from Anhui province had the highest quality. The loadings scores indicated that the peaks 3, 4, 5, 6, 7 might be the main influencing factors for the discrimination of different samples. Analysis of ginger loading plots of PC1 against PC2 (Figure 3E) revealed that peaks explained by PC1, peaks 5, 7, and 8 influenced the cluster in top-down order. Owing to the higher intensities of peaks 7, 8, 5, Yunnan samples were characterized primarily by positive values of PC1. From the loading plot, peak 1, 2, 4, 5, 7, 8 had the most substantial influence on the quality of geographical differences and various processing techniques. From the loading plot of liquid nitrogen (Figure 3F), peaks 1, 3, 5, and 7 had an enormous impact on geographic difference quality. Besides, peak 2 and peak 4 also affect quality evaluation.
By determining the contents of those chemical marker compounds, the quality of gingers from various sources could be measured. The consistent result was obtained from SA, PCA, and HCA, implying that PCA was an efficient complement for HCA and SA. In consideration of our existing standard and the currently contained standards produced in the laboratory, 6-gingerol, 8-gingerol, 10-gingerol (peak 4, 5, 6 in superfine samples; peak 4, 5, 7 in grinding and liquid nitrogen samples) were selected as the natural substance to study the effect of producing area on the quality of ginger. Meanwhile, gingerols exhibited major medicinal activity as the main component of ginger.
3.5 Method validations of quantitative analysis
3.5.1 Linearity
As shown in Figures 4A, B, methanol was used to prepare the mixed standard of 3 main ingredients at each concentration level to establish the calibration curves, ensuring that the concentration of samples was within the linear range. Table 2 demonstrated that the established calibration curves had a favorable direct relationship within the test ranges. The correlation coefficients were more excellent than 0.9990. Their structures are shown in Figure 4C.
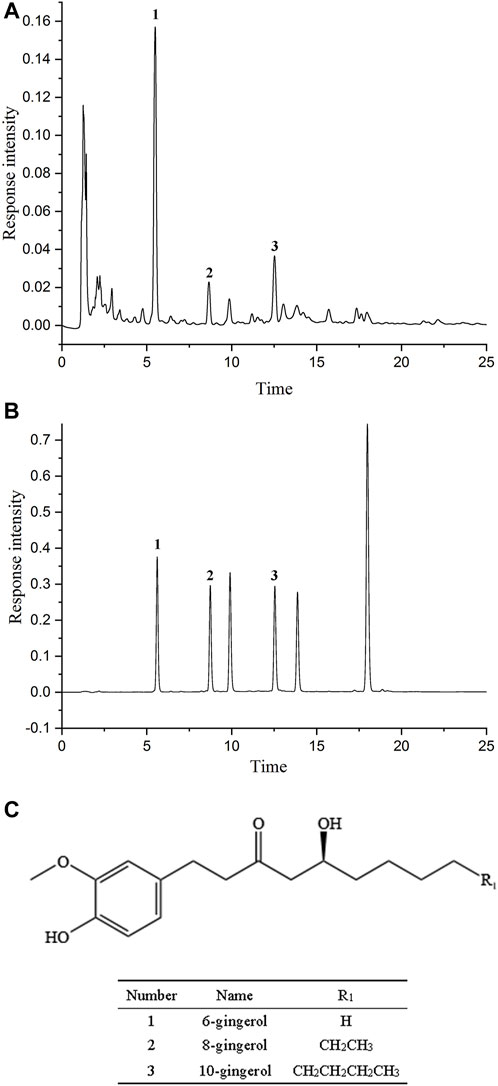
FIGURE 4. The chromatographic fingerprint and chemical structure of gingerols. (A) The chromatographic fingerprint of Zingiber officinale Roce. (B) The HPLC chromatogram of gingerols composition. (1) 6-gingerol; (2) 8-gingerol; (3) 10-gingerol. (C) The chemical structure of gingerols.
3.5.2 Precision, repeatability, and stability
The standard sample precision was inspected six times of injection in succession. Six consecutive doses of the sample were performed for accurate inspection. Reproducibility was evaluated by comparing the peak areas of three independently prepared samples. The reproducibility assessed by comparing the peak area of three individually designed samples can be viewed in Supplementary Table S2. The methodology validation that the relative standard deviation (RSD) for precision, repeatability. The stability coefficient was lower than 3.37. All these results showed that the HPLC method is accurate and valid.
3.6 Contents analyses of the three primary active ingredients
The relative peak area (RPA) and relative retention time (RRT) was represented as the reference peaks. The contents of three primary active ingredients of ginger in Supplementary Table S3. It illustrated that the content of gingerols was significantly varied in each sample, especially the samples from different sources. The internal quality relationship should be evaluated to conquer the suitable origin and extraction method. The content of the 6-gingerol was the most abundant component. The sequence of the total contents of the three compounds in diverse samples was 6-gingerol >8-gingerol >10-gingerol. They were primary index ingredients as well as potentially active compounds in ginger. Consequently, the quality control approach in Chinese Pharmacopoeia using only 6-gingerol for quantification was improper. It was suggested that other gingerols (8-gingerol and 10-gingerol) also should be quantified. Hence, we used 6-gingerol as well as 8-gingerol and 10-gingerol as the quality marker in this paper.
The samples collected from Anhui province had the highest total contents of three components. It indicated that the ginger from the Anhui province might have the most excellent exceptional quality. Moreover, the samples crushed by low-temperature liquid nitrogen, the content of gingerols, were significantly higher than other pre-processing crushing methods. Liquid nitrogen processing might provide the usable method to get the highest gingerols contents of ginger.
3.7 Discriminant analysis
Based on Fisher’s linear discriminant function coefficients, classification discriminant models of samples were established from three origins: Shandong, Anhui, and Yunnan provinces (X1: 6-gingerol, X2: 8-gingerol, X3: 10-gingerol,):
In superfine samples:
In grinding samples:
In liquid nitrogen samples:
When discriminating against the samples type, the samples were plugged into the three classification functions, respectively. Then classify them into the class corresponding to the most considerable numerical value as the substantial value. X represents the variable. Meanwhile, the regression estimate method was used for discriminant analysis. The actual result was shown in Table 3, 82.4%, 100%, and 88.2% of the original grouped cases were correctly classified. It showed that this method was practical and feasible. The discriminant analysis could achieve the prediction and identification of the origin of gingers. So as to, discriminant analysis had an absolute value for the promotion and application.
4 Discussion
Interestingly, a discriminant analysis of the region of origin of ginger found that the retention of active ingredients varied among different processing methods. In order to investigate potential factors, the ginger was ground using liquid nitrogen freezing, resulting in the smallest particle size. Additionally, the use of lower temperatures helped to preserve the stability of the phenolic components. Simultaneously, the elevated temperature involved in the ultra-fine grinding procedure, coupled with the excessive powder produced during the grinding and crushing stages, has the potential to diminish the concentration of active constituents. The gingerols may convert into shogaols upon exposure to high temperatures during the drying or frying process. Consequently, the anti-oxidant and antimicrobial properties of gingerols are enhanced. In addition, it has been established that practical processing methods have a substantial influence on the gingerol content. The results of the current study suggest that the utilization of liquid nitrogen freezing is a superior process for achieving the highest recovery of gingerol from ginger (Chen et al., 2019). The application of ultrasonic pressure waves and cavitation effectively disrupted the cells and cell walls, leading to the breakdown of the ginger material. Furthermore, the utilization of ultrasound pretreatment has the added advantage of preserving the natural color of dried ginger slices. These techniques have been shown to be effective in enhancing the concentration of bioactive compounds such as gingerols oil, derivatives, and antioxidants, while also inactivating harmful enzymes like polyphenol oxidase and peroxidase (Osae et al., 2019). The temperature was an essential factor to be considered for determining the optimal extraction condition (Hsieh et al., 2020). The source of ginger is identified, and the advantages and disadvantages of ginger quality are evaluated through this method. The liquid nitrogen freezing and crushing method and ultrasonic extraction method retain the unique flavor of ginger to make a relevant diet. The evaluation method for ginger will serve as a potential and valuable reference for evaluating the quality, extraction methods, and development of food or other related traditional Chinese medicines. It is necessary to acknowledge the limitations of this work, for example, the number of ginger samples is small and all compounds in ginger were not acknowledged through the HPLC. In our future work, we intend to collect more ginger samples and identify more compounds of ginger by mass spectrometry.
5 Conclusion
In this work, a fast and straightforward HPLC fingerprint of ginger was developed. The results of chemometric techniques such as SA, HCA, PCA, and DA were suitable for analyzing fingerprint data, establishing quality control, and evaluating ginger. Characteristic fingerprint peaks were identified, and gingerol components could be used as markers and quantitatively determined. Based on the analysis, the disparity of samples from different origins was the dominant factor in quality differences and geographical distance. The physicochemical properties of ginger are greatly influenced by the processing, fermentation, and drying methods employed. In essence, Anhui serves as the most suitable source of ginger, while the optimal processing technique involves the use of liquid nitrogen to freeze and crush the ginger. These findings provide valuable insights for the future utilization of ginger. For scholars conducting research on food and drug quality control of traditional Chinese medicine, this series of development strategies can offer constructive suggestions and ideas.
Data availability statement
The original contributions presented in the study are included in the article/Supplementary Material, further inquiries can be directed to the corresponding authors.
Author contributions
YL: Writing–original draft, Writing–review and editing, Conceptualization, Investigation. JW: Writing–original draft, Writing–review and editing, Data curation, Methodology, Validation. CZ: Investigation, Software, Writing–review and editing. JZ: Conceptualization, Supervision, Writing–review and editing. WL: Methodology, Writing–review and editing. YL: Data curation, Writing–review and editing. WY: Formal Analysis, Visualization, Writing–review and editing. HY: Project administration, Validation, Writing–review and editing. CP: Funding acquisition, Visualization, Writing–review and editing.
Funding
The author(s) declare financial support was received for the research, authorship, and/or publication of this article. Anhui University Collaborative Innovation Project (Grant No. GXXT-2019-043), Anhui University Collaborative Innovation Project (Grant No. GXXT-2022-075), Anhui University Collaborative Innovation Project (Grant No. GXXT-2020-025).
Conflict of interest
The authors declare that the research was conducted in the absence of any commercial or financial relationships that could be construed as a potential conflict of interest.
Publisher’s note
All claims expressed in this article are solely those of the authors and do not necessarily represent those of their affiliated organizations, or those of the publisher, the editors and the reviewers. Any product that may be evaluated in this article, or claim that may be made by its manufacturer, is not guaranteed or endorsed by the publisher.
Supplementary material
The Supplementary Material for this article can be found online at: https://www.frontiersin.org/articles/10.3389/fchem.2023.1296712/full#supplementary-material
Abbreviations
HPLC, high-performance liquid chromatography; SA, similarity analysis; PCA, principal component analysis; HCA, hierarchical cluster analysis; DA, discriminant analysis; SPSS, statistical product and service solutions; RPA, relative peak area; RRT, relative retention time.
References
Ballester, P., Cerdá, B., Arcusa, R., Marhuenda, J., Yamedjeu, K., and Zafrilla, P. (2022). Effect of ginger on inflammatory diseases. Mol. (Basel, Switz. 27 (21), 7223. doi:10.3390/molecules27217223
Chen, G. T., Yuan, B., Wang, H. X., Qi, G. H., and Cheng, S. J. (2019). Characterization and antioxidant activity of polysaccharides obtained from ginger pomace using two different extraction processes. Int. J. Biol. Macromol. 139, 801–809. doi:10.1016/j.ijbiomac.2019.08.048
Chen, Q., An, X., Li, H., Zhu, Y., Su, J., and Cui, L. (2017). Do manure-borne or indigenous soil microorganisms influence the spread of antibiotic resistance genes in manured soil? Soil Biol. Biochem. 114, 229–237. doi:10.1016/j.soilbio.2017.07.022
Cui, L., Zhang, Y., Shao, W., and Gao, D. (2016). Analysis of the HPLC fingerprint and QAMS from Pyrrosia species. Industrial Crops Prod. 85, 29–37. doi:10.1016/j.indcrop.2016.02.043
Funk, J. L., Frye, J. B., Oyarzo, J. N., Chen, J., Zhang, H., and Timmermann, B. N. (2016). Anti-inflammatory effects of the essential oils of ginger (zingiber officinale roscoe) in experimental rheumatoid arthritis. PharmaNutrition 4 (3), 123–131. doi:10.1016/j.phanu.2016.02.004
Gaur, M., Das, A., Sahoo, R. K., Mohanty, S., Joshi, R. K., and Subudhi, E. (2016). Comparative transcriptome analysis of ginger variety Suprabha from two different agro-climatic zones of Odisha. Genomics data 9, 42–43. doi:10.1016/j.gdata.2016.06.014
Ghisoni, S., Lucini, L., Rocchetti, G., Chiodelli, G., Farinelli, D., Tombesi, S., et al. (2019). Untargeted metabolomics with multivariate analysis to discriminate hazelnut (Corylus avellana L.) cultivars and their geographical origin. J. Sci. Food Agric. 100 (2), 500–508. doi:10.1002/jsfa.9998
Guo, H., Zhang, Z., Yao, Y., Liu, J., Chang, R., Liu, Z., et al. (2018). A new strategy for statistical analysis-based fingerprint establishment: application to quality assessment of Semen sojae praeparatum. Food Chem. 258, 189–198. doi:10.1016/j.foodchem.2018.03.067
Hsieh, Y. H., Li, Y., Pan, Z., Chen, Z., Lu, J., Yuan, J., et al. (2020). Ultrasonication-assisted synthesis of alcohol-based deep eutectic solvents for extraction of active compounds from ginger. Ultrason. sonochemistry 63, 104915. doi:10.1016/j.ultsonch.2019.104915
Kim, S., Lee, M. S., Jung, S., Son, H. Y., Park, S., Kang, B., et al. (2018). Ginger extract ameliorates obesity and inflammation via regulating MicroRNA-21/132 expression and AMPK activation in white adipose tissue. Nutrients 10 (11), 1567. doi:10.3390/nu10111567
Kong, W. J., Zhao, Y. L., Xiao, X. H., Jin, C., and Li, Z. L. (2009). Quantitative and chemical fingerprint analysis for quality control of rhizoma Coptidischinensis based on UPLC-PAD combined with chemometrics methods. Phytomedicine Int. J. phytotherapy Phytopharm. 16 (10), 950–959. doi:10.1016/j.phymed.2009.03.016
Levita, J., Syafitri, D. M., Supu, R. D., Mutakin, M., Megantara, S., Febrianti, M., et al. (2018). Pharmacokinetics of 10-gingerol and 6-shogaol in the plasma of healthy subjects treated with red ginger (Zingiber officinale var. Rubrum) suspension. Biomed. Rep. 9 (6), 474–482. doi:10.3892/br.2018.1163
Li, Y., Hong, Y., Han, Y., Wang, Y., and Xia, L. (2016). Chemical characterization and antioxidant activities comparison in fresh, dried, stir-frying and carbonized ginger. J. Chromatogr. B, Anal. Technol. Biomed. life Sci. 1011, 223–232. doi:10.1016/j.jchromb.2016.01.009
Liu, W., Wang, D., Liu, J., Li, D., and Yin, D. (2016). Quality evaluation of potentilla fruticosa L. By high performance liquid chromatography fingerprinting associated with chemometric methods. PloS one 11 (2), e0149197. doi:10.1371/journal.pone.0149197
Liu, X. J., Hu, J., Li, Z. Y., Qin, X. M., Zhang, L. Z., and Guo, X. Q. (2011). Species classification and quality assessment of Chaihu (Radix Bupleuri) based on high-performance liquid chromatographic fingerprint and combined chemometrics methods. Archives pharmacal Res. 34 (6), 961–969. doi:10.1007/s12272-011-0613-2
Liu, Y., Liu, J., and Zhang, Y. (2019). Research progress on chemical constituents of zingiber officinale roscoe. BioMed Res. Int. 2019, 1–21. doi:10.1155/2019/5370823
Mahomoodally, M. F., Aumeeruddy, M. Z., Rengasamy, K. R. R., Roshan, S., Hammad, S., Pandohee, J., et al. (2021). Ginger and its active compounds in cancer therapy: from folk uses to nano-therapeutic applications. Seminars cancer Biol. 69, 140–149. doi:10.1016/j.semcancer.2019.08.009
Marak, N. R., Malemnganbi, C. C., Marak, C. R., and Mishra, L. K. (2019). Functional and antioxidant properties of cookies incorporated with foxtail millet and ginger powder. J. food Sci. Technol. 56 (11), 5087–5096. doi:10.1007/s13197-019-03981-6
Miao, L. L., Zhou, Q. M., Peng, C., Meng, C. W., Wang, X. Y., and Xiong, L. (2019). Discrimination of the geographical origin of the lateral roots of aconitum carmichaelii using the fingerprint, multicomponent quantification, and chemometric methods. Mol. (Basel, Switz. 24 (22), 4124. doi:10.3390/molecules24224124
Osae, R., Zhou, C., Xu, B., Tchabo, W., Tahir, H. E., Mustapha, A. T., et al. (2019). Effects of ultrasound, osmotic dehydration, and osmosonication pretreatments on bioactive compounds, chemical characterization, enzyme inactivation, color, and antioxidant activity of dried ginger slices. J. food Biochem. 43 (5), e12832. doi:10.1111/jfbc.12832
Peng, L. Q., Cao, J., Du, L. J., Zhang, Q. D., Xu, J. J., Chen, Y. B., et al. (2017). Rapid ultrasonic and microwave-assisted micellar extraction of zingiberone, shogaol and gingerols from gingers using biosurfactants. J. Chromatogr. A 1515, 37–44. doi:10.1016/j.chroma.2017.07.092
Sangwan, A., Kawatra, A., and Sehgal, S. (2014). Nutritional composition of ginger powder prepared using various drying methods. J. food Sci. Technol. 51 (9), 2260–2262. doi:10.1007/s13197-012-0703-2
Shanmugam, K. R., Mallikarjuna, K., Kesireddy, N., and Sathyavelu Reddy, K. (2011). Neuroprotective effect of ginger on anti-oxidant enzymes in streptozotocin-induced diabetic rats. Food Chem. Toxicol. Int. J. Publ. Br. Industrial Biol. Res. Assoc. 49 (4), 893–897. doi:10.1016/j.fct.2010.12.013
Shukla, Y., and Singh, M. (2007). Cancer preventive properties of ginger: a brief review. Food Chem. Toxicol. Int. J. Publ. Br. Industrial Biol. Res. Assoc. 45 (5), 683–690. doi:10.1016/j.fct.2006.11.002
Tan, J., Zheng, M., Duan, S., Zeng, Y., Zhang, Z., Cui, Q., et al. (2018). Chemical profiling and screening of the marker components in the fruit of Cassia fistula by HPLC and UHPLC/LTQ-Orbitrap MSn with chemometrics. Mol. (Basel, Switz. 23 (7), 1501. doi:10.3390/molecules23071501
Yamaguchi, K., Kato, T., Noma, S., Igura, N., and Shimoda, M. (2010). The effects of high hydrostatic pressure treatment on the flavor and color of grated ginger. Biosci. Biotechnol. Biochem. 74 (10), 1981–1986. doi:10.1271/bbb.90712
Zhong, J. S., Wan, J. Z., Ding, W. J., Wu, X. F., and Xie, Z. Y. (2015). Multi-responses extraction optimization combined with high-performance liquid chromatography-diode array detection-electrospray ionization-tandem mass spectrometry and chemometrics techniques for the fingerprint analysis of Aloe barbadensis Miller. J. Pharm. Biomed. analysis 107, 131–140. doi:10.1016/j.jpba.2014.12.032
Keywords: HPLC, high-performance liquid chromatography, SA, similarity analysis, PCA, principal component analysis, HCA, hierarchical cluster analysis, DA, discriminant analysis, SPSS, statistical product and service solutions, RPA, relative peak area, RRT, relative retention time
Citation: Wang J, Liu Y, Zuo C, Zhang J, Liang W, Liu Y, Yu W, Yu H and Peng C (2023) Different origins and processing methods affect the intrinsic quality of ginger: a novel approach to evaluating ginger quality. Front. Chem. 11:1296712. doi: 10.3389/fchem.2023.1296712
Received: 19 September 2023; Accepted: 31 October 2023;
Published: 10 November 2023.
Edited by:
David Kitts, University of British Columbia, CanadaReviewed by:
Tushar Dhanani, Florida Agricultural and Mechanical University, United StatesIsmail Yener, Dicle University, Türkiye
Copyright © 2023 Wang, Liu, Zuo, Zhang, Liang, Liu, Yu, Yu and Peng. This is an open-access article distributed under the terms of the Creative Commons Attribution License (CC BY). The use, distribution or reproduction in other forums is permitted, provided the original author(s) and the copyright owner(s) are credited and that the original publication in this journal is cited, in accordance with accepted academic practice. No use, distribution or reproduction is permitted which does not comply with these terms.
*Correspondence: Can Peng, cGVuZ2NhbkBhaHRjbS5lZHUuY24=; Hao Yu, eWh6XzEyMzBAMTYzLmNvbQ==
†These authors have contributed equally to this work