- 1Department of Chemistry, University College London (UCL), London, United Kingdom
- 2Institute for Materials Discovery, University College London (UCL), London, United Kingdom
- 3Xiamen Institute of Rare-earth Materials, Haixi Institutes, Chinese Academy of Sciences, Xiamen, China
- 4Center of Single-Molecule Sciences, College of Electronic Information and Optical Engineering, Nankai University, Tianjin, China
Resazurin (Alamar Blue, RZ) is a widely utilized fluorescent probe for biological sensing, whose fluorescent intensity can be modulated by changing its redox states; thereby, electrochemical reactivity of RZ is of significance when designing a sensing assay. Herein, we report novel two-way electrochemical reactivity modulation of RZ using host-guest complexation with rigid molecular containers cucurbit[n]uril (CBn, n = 7, 8). The complexation between CBn and RZ is confirmed by 1H NMR measurements and supported by computational simulation, and the binding constants are determined via UV-vis titration. Notably, the voltametric data highlights that the redox reactivity of RZ can be activated or deactivated upon encapsulation by CB8 or CB7, respectively. This two-way reactivity modulation is hypothesized to be mediated by the difference in cavity volume that favors or hinders the approach of water molecules to the encapsulated reaction center during the reduction process. Despite the similar cavity size to CB, molecular containers such as cyclodextrins (CDs) exhibit considerably weaker modulation effects. Our approach can potentially be applied to other redox processes that involve proton transfer, and open new possibilities in supramolecular electrochemistry.
1 Introduction
Resazurin (7-hydroxy-3H-phenoxazin-one-10-oxide, RZ), also known as Alamar Blue, is a redox-sensitive, non-toxic, and water-soluble dye that has found widespread use as a tracer for analyzing diverse biochemical and metabolic processes in biological system (O'Brien et al., 2000; Emter and Natsch, 2015; Präbst et al., 2017; Mishra et al., 2019). The versatility of RZ as a redox dye stems from its two-step reduction process. Initially, the purple and slightly fluorescent RZ undergoes an irreversible reduction, transforming into pink and highly fluorescent resorufin (RS). This step is then followed by a reversible reduction, converting RS into a colorless and non-fluorescent compound known as dihydro-resorufin (DHRS) (Ibáñez et al., 2020). The modulation of these reduction processes is beneficial for exploiting RZ as a sensing indicator; however, it remains largely unexplored in existing literature, highlighting its untapped potential and practical significance.
Cucurbitu[n]rils (CBn, n = 5–8) are a family of macrocyclic hosts synthesized through the condensation of affordable starting materials (Barrow et al., 2015; Lin et al., 2020). Their versatile properties offer a wide range of potential applications, including reactivity modulation (Lee et al., 2013; Moorthy et al., 2022; Moorthy et al., 2023), drug delivery (Walker et al., 2011), environmental monitoring (Chio et al., 2022), explosive detection (Chio et al., 2019) and biosensing (Chio et al., 2020; Chio et al., 2021; Davison et al., 2023). Notably, CB7 and CB8, are both water-soluble (with a solubility of >20 mM for CB7 and <0.1 mM for CB8, depending on the preparation method) and highly biocompatible, demonstrate promising applicability in aqueous-based scenarios, such as biological systems (Uhlenheuer et al., 2010) and electrochemical investigations (Boulas et al., 1998; Jeon et al., 2002; Gadde et al., 2009; Luo et al., 2019). The electrochemical behaviors of CB7- and CB8-encapsulated redox-active molecules, e.g., dimethyl viologen (MV2+), have been reported by Kim et al. (Jeon et al., 2002; Kim et al., 2002) and Kaifer et al. (Ong et al., 2002; Ling et al., 2007; Gadde and Kaifer, 2011). In particular, encapsulation of MV2+ within CB7 was observed to deactivate its reduction reaction due to the preferential stabilization of the dicationic charge by the electron-rich portals of CB7. In contrast, the reduction of MV2+ can be significantly activated upon CB8-encapsulation by forming stable 1:2 complexes of CB8•2 MV+•. However, this activation driven by dimerization relies on the formation of highly polarizable aromatic radical cations (e.g., MV+•) (Cai et al., 2021), which are relatively rare. Thus, precedent examples are limited to only a few viologen-based compounds.
Herein, we report the host-guest complexation of resazurin with CB7 and CB8 for the first time. UV-vis spectroscopy and NMR spectroscopy were used to determine the binding affinities of the inclusion complexes, while computational modelling based on density functional theory (DFT) was employed to investigate the binding modes. Notably, we discovered that the electrochemical reduction of resazurin can be either activated or deactivated through complexation with different cucurbituril homologues. Specifically, CB7 was found to deactivate the reduction process (ΔECV = −54 mV), whereas CB8 activated it (ΔECV = +37 mV), as confirmed by cyclic voltammetry (CV) and square wave voltammetry (SWV) measurements. DFT modelling provided insights into the underlying mechanism, suggesting that the variation in cavity volume facilitates or hinders the approach of water molecules to the encapsulated reaction center, i.e., the -NO group (Figure 1). Our supramolecular approach holds promising prospects for a broader range of redox-active host-guest complexes, where proton transfer is coupled with electron transfer during electrochemical reactions, and thus open new possibilities in supramolecular electrochemistry.
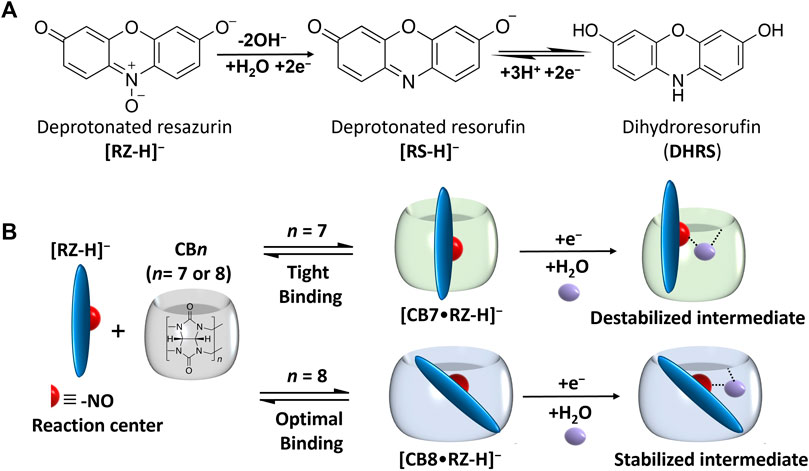
FIGURE 1. (A) Reduction process of deprotonated resazurin ([RZ-H]–) and corresponding chemical structures at pH 7.5 (B) Host-guest complexation process of [RZ-H]– with CB7 and CB8 and schematic illustration of corresponding intermediates formed by the first one-electron reduction process.
2 Materials and experimental methods
2.1 Materials
Resazurin, boric acid and phosphoric acid were purchased from Aladdin. Acetic acid and sodium hydroxide were bought from SCR. Resorufin was ordered from TCI. β-cyclodextrin (βCD), γ-cyclodextrin (γCD), deuterium oxide, deuterium chloride (20% in D2O) and 1-adamantylamine (AdNH2) were bought from Adamas. Cucurbit[7]uril (CB7) and cucurbit[8]uril (CB8) were synthesized and purified following the procedures reported before (Isaacs, 2009). All chemicals were of analytical grade and used without further treatment.
2.2 Electrochemical measurements
All electrochemical experiments were performed on Gamry Interface 1010E workstation. A glassy carbon working electrode (d = 3 mm), platinum plate counter electrode (6.5 mm × 6.5 mm) and leakless Ag/AgCl reference electrode (d = 5 mm) were inserted into a 50-mL single-compartment electrochemical cell. Prior to each electrochemical measurement, all samples were purged with nitrogen gas for more than 10 min to remove dissolved oxygen in solution, and the surface of glassy carbon working electrode was polished with a 0.05 μm alumina slurry on a felt polishing cloth. To eliminate the influences of pH on the electrochemical behaviors of RZ, which is pH-sensitive, pH values of all samples were adjusted again after introducing host molecules into the sample solution.
CV measurements were conducted by sweeping potential from 300 mV to −400 mV at a scan rate of 10 mV/s with a step size of 1 mV for 5 cycles. The SWV measurements were performed with initial potential of 300 mV, final potential of −400 mV, step size of 1 mV, pulse size of 25 mV and frequency of 5 Hz. Unless stated otherwise, all measurements were done with these parameters.
All samples for electrochemical measurements were prepared at concentration of 1 mM in Britton-Robinson (B-R) buffer at pH of 7. It should be noted that the [CB8•RZ-H]− complex formation might lead to a slight reduction in the solubility of both the host and guest molecules in the solution, resulting in the formation of a minor amount of precipitate. However, the precipitate was removed through filtration prior to the measurements. B-R buffer solution was prepared by adjusting the pH of a mixture of boric acid (0.04 M), phosphoric acid (0.04 M) and acetic acid (0.04 M) by NaOH (0.2 M). The pH of solution was checked by Mettler Toledo F20 pH meter.
2.3 1H NMR measurements
1H NMR spectra were collected using a Bruker Avance III 500 MHz spectrometer. Samples for 1H NMR measurements were prepared at 1 mM in D2O unless specified otherwise.
2.4 UV-vis titrations
UV-vis titrations for the determination of binding constants were conducted using an Agilent Cary 60 spectrophotometer. The guest molecule was maintained at a concentration of 4 μM throughout the titration, while the concentration of the host molecule was gradually increased. Water was used as the solvent.
2.5 Optimization of molecular models
Initial optimization of molecular models was done by MMFF94 in Chem3D, and the full optimization was performed at wB97XD/6-31G* and CPCM/wB97XD/6-31G* level of theory using Gaussian 09. Solvent effects were approximated by using CPCM implicit water model. Moreover, the van der Waals interactions, which are regarded as the major contributor for the stability of the host-guest complexes, was evaluated by employing the dispersion-corrected DFT functional wB97XD. Energy change in redox reactions and complexation-decomplexation processes were calculated by using the energy of optimized models. All DFT optimization was performed on the UCL Thomas high performance computing facility (Thomas@UCL).
3 Results and discussion
3.1 Electrochemical modulation
The effect of supramolecular complexation on the redox properties of [RZ-H]− was investigated using electrochemical techniques, including CV and SWV. It is well-established that RZ undergoes a two-step reduction process: first, through an irreversible two-electron reduction step (R1), yielding deprotonated resorufin ([RS-H]−), which can subsequently undergo a reversible two-electron reduction step (R2) to produce the neutral form of dihydro-resorufin (DHRS), as illustrated in Figure 1A. The cyclic voltammogram of free [RZ-H]− is shown by the black line in Figure 2A, consistent with previous literature reports (Wang et al., 1998). Specifically, the reduction peak potentials of free [RZ-H]− corresponding to R1 and R2 are observed at −5 mV and −141 mV vs. Ag/AgCl in CV, respectively.
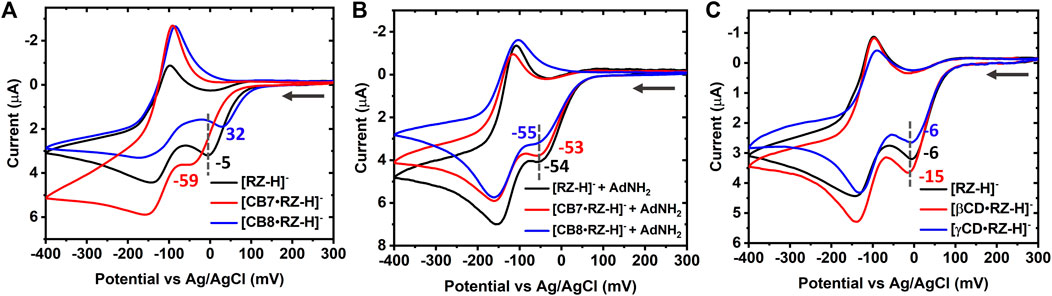
FIGURE 2. (A) Overlaid cyclic voltammograms of 1 mM [RZ-H]− alone (black line), and in the presence of equal molar CB7 (red line) or CB8 (after precipitate removing, blue line) and (B) control measurements in the presence of 3 mM of AdNH2. (C) Overlaid cyclic voltammograms of 1 mM [RZ-H]− alone (black line), and in the presence of equal molar βCD (red line) or γCD (blue line). Electrolyte: B-R buffer (pH 7) solution containing 0.04 M boric acid, 0.04 M phosphoric acid, 0.04 M acetic acid and 0.2 M sodium hydroxide; Potential range: from 300 mV to −400 mV. Scan rate: 10 mV/s. Working electrode: glassy carbon electrode (d = 3 mm).
Upon the introduction of 1 equivalent of CB7 or CB8, a noticeable increase in the peak-to-peak splitting between R2 and its corresponding anodic peak was observed (Figure 2A), suggesting a reduced diffusivity and a slower electrochemical kinetics within bulky inclusion complexes ([CB7•RZ-H]− and [CB8•RZ-H]−) compared to free [RZ-H]− molecules. Furthermore, host-guest complexation with CB7 or CB8 caused distortion in the cathodic waves and a significant increase in the anodic peak current, which can be attributed to slower mass transport kinetics associated with the bulky complexes (Liu et al., 2021). The host-guest complexation/dissociation processes, coupled to the electron-transfer step, have also been reported previously to contribute to the distortions observed in the cathodic wave (Saleh et al., 2008).
Notably, in the presence of 1 equivalent of CB7 or CB8, the irreversible reduction R1 exhibited opposite shifts with respect to free [RZ-H]−:
Although the reduction potential of RZ is known to be pH-dependent (Wang et al., 1998), the observed modulation of the potential cannot be attributed to a change in the pH of the media in the presence of CB. This is because the pH value of the samples was maintained at 7 throughout the electrochemical measurements using B-R buffer, which also served as the supporting electrolyte. Additionally, this modulation cannot be ascribed to the previously reported pKa shift effects of CB (Nau et al., 2011), as no such effect was observed for both [CB7•RZ-H]− and [CB8•RZ-H]− complexes (Supplementary Figure S2). These combined observations indicate that the modulation of the redox activity of [RZ-H]− induced by CB complexation does not occur via a change in the protonation state of [RZ-H]− and thus hinting that it may be caused by alterations in the microenvironment surrounding [RZ-H]− upon complexation with CB.
To investigate the above hypothesis and as a control experiment, 1-adamantylamine (AdNH2) was employed as a strong competitive guest to displace [RZ-H]– from the CB cavity. It is known that AdNH2 exhibits considerably high binding constants with CB7 and CB8 [Kbind = 4.23
3.2 Mechanism analysis
To gain further insights into the modulation mechanism, we conducted measurements using β-cyclodextrin (βCD) and γ-cyclodextrin (γCD) as supramolecular hosts. βCD and γCD are well-known analogues of CB7 and CB8, respectively, with comparable cavity volumes but different geometric structures and interacting functional groups. The formation of inclusion complexes of [RZ-H]− with hosts (CB7, CB8, βCD and γCD) has been studied with 1H NMR spectroscopy (Supplementary Figures S2–S6). The down-field shifted RZ signals in the presence of CB7, CB8, and βCD indicates the location of the terminal benzene groups of the guest near the portal region, whereas the middle part (without hydrogen) was included inside the cavity. Interestingly, unlike these three hosts, γCD has caused a significant up-field shift of the guest signals. Fitting of the data suggests a potential 2:1 (guest:host) ternary complexation. The chemical shift of free resazurin drops markedly to 6.23 ppm upon the addition of only 0.5 equiv. of γCD, which is expected to be above 6.30 ppm should it follow a fast-exchange 1:1 binding mechanism (the apparent chemical shift is determined by the content average of the free and complexed guest). The binding affinities of the complexes have been determined via UV-vis titrations and NMR titrations (Table 1; Supplementary Figures S7, S8). It is found that the binding affinities of resazurin with cucurbiturils (6.5 × 104 M−1 for [CB7•RZ-H]− and 7.9 × 104 M−1 for [CB7•RZ-H]− are generally an order of magnitude larger than their corresponding reference cyclodextrins 2.2×103 M−1 for βCD and ca. 106 ∼107 M−2 for γCD in the 2:1 complexatin form). Athough the complexaion degree of resazurin is expected to reach over 50% and 90%, respectively, for βCD and γCD in the electrochemical experimental conditions, as calculated from the binidng constants, the shifts observed in R1, as shown in Figure 2C and Supplementary Figure S1C, are still relatively small (<10 mV). This indicates that CDs only have minor modulation effects on the electrochemical reduction of [RZ-H]− compared with CBs. This may be attributed to the interacting and geometric differences between the two types of host molecules, including the molecular shape, constrictive binding effect, as well as the different dipole interactions at host portals.
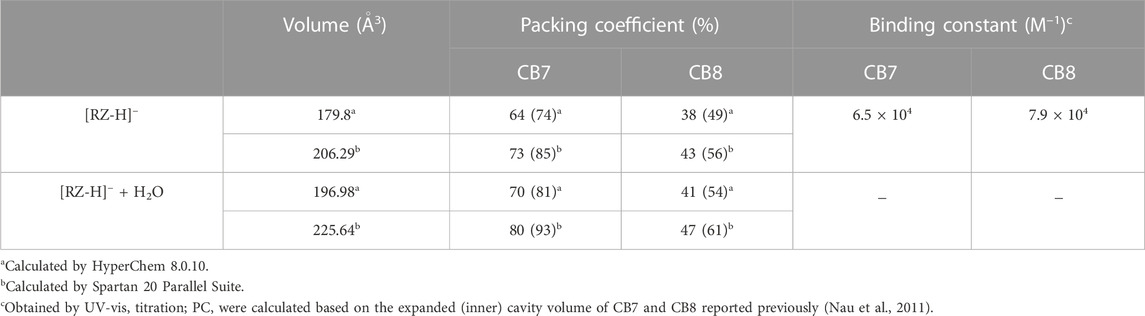
TABLE 1. Molecular volume, packing coefficients and binding constants of [CB7•RZ-H]− and [CB8•RZ-H]− with/without one H2O molecule included.
The irreversible reduction (R1) of [RZ-H]− is known to involve cascades of electron transfer and protonation of its -NO group, as illustrated in Figure 1A (Khazalpour and Nematollahi, 2014), and at pH 7, protonation of the -NO group is mediated by water molecules. As reported by the Nematollah et al., the ease of reduction process of [RZ-H]− can be remarkably affected by the ease of -NO group protonation, i.e., the reduction peak potential is shifted to a more positive position as the protonation of -NO is easier to occur at lower pH (Khazalpour and Nematollahi, 2014). Based on this understanding, we propose that the modulation of the electrochemical reactivity of [RZ-H]− is achieved by regulating the accessibility of water molecules to the reaction center (-NO group) for its protonation encapsulated within the CB cavity, as shown in Figure 1B. To support this hypothesis, we studied the binding geometries of the complexes using DFT calculations (Figure 3). The computational results show that the reaction center (the -NO group of [RZ-H]−) is deeply immersed in the host cavity for both CB7 and CB8. Therefore, the accessibility of water molecules to the confined space where the -NO group is located becomes a key factor for the reduction of [RZ-H]–, as shown in the energy-minimized models in Supplementary Figure S9.
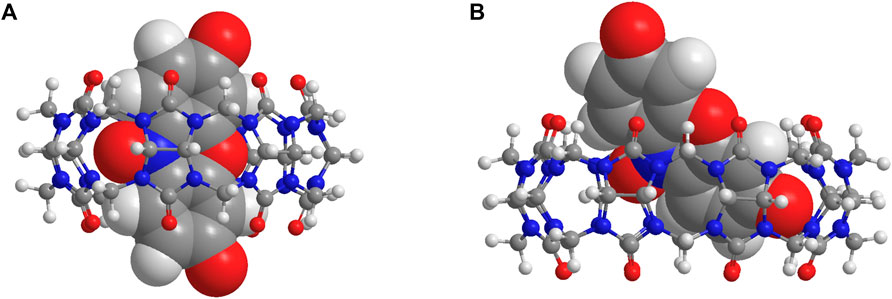
FIGURE 3. Energy-minimized molecular models of (A) [CB7•RZ-H]– and (B) [CB8•RZ-H]– at CPCM/wB97XD/6-31G* level of theory.
To evaluate the tightness of the binding of RZ in both host molecules, we calculated the van der Waals volume of [RZ-H]− using HyperChem software and Spartan software, and determined its packing coefficient (PC) within CB7 and CB8 (Table 1). The PC of [RZ-H]− in CB7, concerning both the expanded and inner cavity volume, appears to be larger than the optimum value of 55% as defined in Rebek’s rule (Mecozzi and Rebek, 1998). However, considering that [RZ-H]− resides only partially in the cavity rather than being fully encapsulated, as shown in Figure 3, the calculated PC values, which use the full volume of the guest molecules, are overestimates. In the case of CB8, the PC generally falls around 55%, even with a partial complexation pattern, indicating that the remaining void space inside the host cavity can remain certain moving freedom (e.g., vibration, rotation, even translation) for both host and guest molecules and even allows the entry of other small molecules, such as a water molecule. This is in contrast to CB7, where tight binding may restrict the accessibility of water molecules to approach the -NO group at the cavity center (Figure 3A).
As a consequence, the conversion from [RZ-H]− to [RS-H]− requires partial displacement of [RZ-H]− from the CB7 cavity for both [CB7•RZ-H]− and its one-electron reduced intermediate [CB7•RZ-H + e]2−. This can be observed in their optimized molecular models in Supplementary Figures S9A–S10B, respectively. The partial dissociation is expected to weaken the host-guest interactions, thus destabilizing the reaction intermediate during the proton transfer step. On the other hand, in the case of CB8, the larger cavity size ensures no significant change in the binding geometry before and after the introduction of a water molecule around the -NO group, both for [CB8•RZ-H]− (Supplementary Figure S9B) and [CB8•RZ-H + e]2− (Supplementary Figure S10D). This suggests the presence of pre-organization effects in [CB8•RZ-H]− and [CB8•RZ-H + e]2−, which effectively accommodate and stabilize the incoming water molecule during the proton transfer step. This pre-organization effect may also contribute to the decrease in entropic penalty upon encapsulation of a water molecule.
To gain semi-quantitative insights into the hypothesis, we calculated energy values of the optimized structures of the one-electron reduced intermediates (free [RZ-H + e]2−, [CB7•RZ-H + e]2− and [CB8•RZ-H + e]2−) interacting with a water molecule located at two different sites: the phenoxide group or the -NO group (Supplementary Figure S10; Supplementary Table S1). For free [RZ-H + e]2−, the system experiences a slight destabilization of +2.7 kcal mol–1 when the water molecule moves from the phenoxide group to the–NO group. Importantly, this energy difference increases to +6.8 kcal mol–1 in the case of [CB7•RZ-H + e]2−, indicating a decreased energetic favorability for the water molecule to approach the reaction center. Conversely, the energy difference reverses to −4.2 kcal mol–1 in the case of [CB8•RZ-H + e]2−, an energetically favorable approach of the water molecule, which supports our hypothesis. We acknowledge that the current DFT modelling results do not account for the entropic terms which can be important in these systems and can be a subject for further computational investigation.
4 Conclusion
In summary, we have successfully demonstrated the deactivation and activation of the electrochemical reactivity of resazurin through host-guest complexation with rigid molecular hosts CB7 and CB8, respectively. This modulation effect is attributed to the tightness of the binding, determined by the combined effects of the packing coefficient and constrictive binding, which control the accessibility of water molecules to the encapsulated reaction center during the proton transfer step. Notably, flexible host molecules βCD and γCD do not exhibit reactivity modulation, highlighting the unique role of CB’s well-defined rigid cavity and their structural features. These findings offer opportunities for fine-tuning the redox behavior of resazurin to accommodate a wider range of target molecules in biosensing applications. Furthermore, our generic approach can be extended to other redox-active processes involving proton transfer and is expected to inspire further understanding and design of nano-confined chemical systems.
Data availability statement
The original contributions presented in the study are included in the article/SupplementaryMaterial, further inquiries can be directed to the corresponding authors.
Author contributions
JL: Data curation, Formal Analysis, Investigation, Writing–original draft. S-HH: Formal Analysis, Investigation, Writing–review and editing. HL: Data curation, Formal Analysis, Writing–review and editing. T-CL: Conceptualization, Formal Analysis, Funding acquisition, Investigation, Supervision, Writing–original draft, Writing–review and editing, Project administration.
Funding
The author(s) declare financial support was received for the research, authorship, and/or publication of this article. This project is funded by EPSRC (Grant EP/P020194/1) and Leverhulme Trust Grant (RPG-2016-393) of T-CL. HL and T-CL are grateful to the Studentship funded by the A*STAR-UCL Research Attachment Programme through the EPSRC M3S CDT (EP/L015862/1).
Acknowledgments
T-CL, HL, and JL acknowledge the use of the UCL Thomas high performance computing facility (Thomas@UCL), and associated support services, in the completion of this work. We are grateful to the United Kingdom Materials and Molecular Modelling Hub for computational resources. JL is grateful to the Xiamen Institute of Rare-earth Materials and Huaqiao University for access to experimental equipment.
Conflict of interest
The authors declare that the research was conducted in the absence of any commercial or financial relationships that could be construed as a potential conflict of interest.
Publisher’s note
All claims expressed in this article are solely those of the authors and do not necessarily represent those of their affiliated organizations, or those of the publisher, the editors and the reviewers. Any product that may be evaluated in this article, or claim that may be made by its manufacturer, is not guaranteed or endorsed by the publisher.
Supplementary material
The Supplementary Material for this article can be found online at: https://www.frontiersin.org/articles/10.3389/fchem.2023.1295715/full#supplementary-material
References
Barrow, S. J., Kasera, S., Rowland, M. J., Del Barrio, J., and Scherman, O. A. (2015). Cucurbituril-based molecular recognition. Chem. Rev. 115, 12320–12406. doi:10.1021/acs.chemrev.5b00341
Boulas, P. L., Gómez-Kaifer, M., and Echegoyen, L. (1998). Electrochemistry of supramolecular systems. Angew. Chem. Int. Ed. 37, 216–247. doi:10.1002/(sici)1521-3773(19980216)37:3<216::aid-anie216>3.0.co;2-p
Cai, K., Zhang, L., Astumian, R. D., and Stoddart, J. F. (2021). Radical-pairing-induced molecular assembly and motion. Nat. Rev. Chem. 5, 447–465. doi:10.1038/s41570-021-00283-4
Chio, W.-I. K., Liu, J., Jones, T., Perumal, J., Dinish, U. S., Parkin, I. P., et al. (2021). SERS multiplexing of methylxanthine drug isomers via host–guest size matching and machine learning. J. Mater. Chem. C 9, 12624–12632. doi:10.1039/D1TC02004H
Chio, W.-I. K., Moorthy, S., Perumal, J., U. S, D., Parkin, I. P., Olivo, M., et al. (2020). Dual-triggered nanoaggregates of cucurbit[7]uril and gold nanoparticles for multi-spectroscopic quantification of creatinine in urinalysis. J. Mater. Chem. C 8, 7051–7058. doi:10.1039/D0TC00931H
Chio, W.-I. K., Xie, H., Zhang, Y., Lan, Y., and Lee, T.-C. (2022). SERS biosensors based on cucurbituril-mediated nanoaggregates for wastewater-based epidemiology. Trends Anal. Chem. 146, 116485. doi:10.1016/j.trac.2021.116485
Chio, W. K., Peveler, W. J., Assaf, K. I., Moorthy, S., Nau, W. M., Parkin, I. P., et al. (2019). Selective detection of nitroexplosives using molecular recognition within self-assembled plasmonic nanojunctions. J. Phys. Chem. C 123, 15769–15776. doi:10.1021/acs.jpcc.9b02363
Davison, G., Jones, T., Liu, J., Kim, J., Yin, Y., Kim, D., et al. (2023). Computer-aided design and Analysis of spectrally aligned hybrid plasmonic nanojunctions for SERS detection of nucleobases. Adv. Mater. Technol. 8, 2201400. doi:10.1002/admt.202201400
Emter, R., and Natsch, A. (2015). A fast Resazurin-based live viability assay is equivalent to the MTT-test in the KeratinoSens assay. Toxicol. Vitro. 29, 688–693. doi:10.1016/j.tiv.2015.02.003
Gadde, S., Batchelor, E. K., and Kaifer, A. E. (2009). Controlling the formation of cyanine dye H- and J-aggregates with cucurbituril hosts in the presence of anionic polyelectrolytes. Chemistry 15, 6025–6031. doi:10.1002/chem.200802546
Gadde, S., and Kaifer, A. (2011). Cucurbituril complexes of redox active guests. Curr. Org. Chem. 15, 27–38. doi:10.2174/138527211793797800
Ibáñez, D., Izquierdo-Bote, D., Pérez-Junquera, A., González-García, M. B., Hernández-Santos, D., and Fanjul-Bolado, P. (2020). Raman and fluorescence spectroelectrochemical monitoring of resazurin-resorufin fluorogenic system. Dyes Pigm 172, 107848. doi:10.1016/j.dyepig.2019.107848
Isaacs, L. (2009). Cucurbit [n] urils: from mechanism to structure and function. Chem. Comm. 6, 619–629. doi:10.1039/b814897j
Jeon, W. S., Kim, H.-J., Lee, C., and Kim, K. (2002). Control of the stoichiometry in host–guest complexation by redox chemistry of guests: inclusion of methylviologen in cucurbit [8] uril. Chem. Comm. 17, 1828–1829. doi:10.1039/b202082c
Khazalpour, S., and Nematollahi, D. (2014). Electrochemical study of Alamar Blue (resazurin) in aqueous solutions and room-temperature ionic liquid 1-butyl-3-methylimidazolium tetrafluoroborate at a glassy carbon electrode. RSC Adv. 4, 8431–8438. doi:10.1039/C3RA45800H
Kim, H.-J., Jeon, W. S., Ko, Y. H., and Kim, K. (2002). Inclusion of methylviologen in cucurbit [7] uril. Proc. Natl. Acad. Sci. U.S.A. 99, 5007–5011. doi:10.1073/pnas.062656699
Lee, T. C., Kalenius, E., Lazar, A. I., Assaf, K. I., Kuhnert, N., Grün, C. H., et al. (2013). Chemistry inside molecular containers in the gas phase. Nat. Chem. 5, 376–382. doi:10.1038/nchem.1618
Lin, R.-L., Liu, J.-X., Chen, K., and Redshaw, C. (2020). Supramolecular chemistry of substituted cucurbit[n]urils. Inorg. Chem. Front. 7, 3217–3246. doi:10.1039/D0QI00529K
Ling, Y., Mague, J. T., and Kaifer, A. E. (2007). Inclusion complexation of diquat and paraquat by the hosts cucurbit [7] uril and cucurbit [8] uril. Chem. Eur. J. 13, 7908–7914. doi:10.1002/chem.200700402
Liu, J., Lambert, H., Zhang, Y.-W., and Lee, T.-C. (2021). Rapid estimation of binding constants for cucurbit [8] uril ternary complexes using electrochemistry. Anal. Chem. 93, 4223–4230. doi:10.1021/acs.analchem.0c04887
Liu, S., Ruspic, C., Mukhopadhyay, P., Chakrabarti, S., Zavalij, P. Y., and Isaacs, L. (2005). The cucurbit [n] uril family: prime components for self-sorting systems. J. Am. Chem. Soc. 127, 15959–15967. doi:10.1021/ja055013x
Luo, H., Chen, L.-X., Ge, Q.-M., Liu, M., Tao, Z., Zhou, Y.-H., et al. (2019). Applications of macrocyclic compounds for electrochemical sensors to improve selectivity and sensitivity. J. Incl. Phenom. Macrocycl. Chem. 95, 171–198. doi:10.1007/s10847-019-00934-6
Mecozzi, S., and Rebek, J. (1998). The 55 % solution: a formula for molecular recognition in the liquid state. Chem. Eur. J. 4, 1016–1022. doi:10.1002/(SICI)1521-3765(19980615)4:6<1016::AID-CHEM1016>3.0.CO;2-B
Mishra, P., Singh, D., Mishra, K. P., Kaur, G., Dhull, N., Tomar, M., et al. (2019). Rapid antibiotic susceptibility testing by resazurin using thin film platinum as a bio-electrode. J. Microbiol. Methods. 162, 69–76. doi:10.1016/j.mimet.2019.05.009
Moorthy, S., Castillo Bonillo, A., Lambert, H., Kalenius, E., and Lee, T.-C. (2022). Modulating the reaction pathway of phenyl diazonium ions using host–guest complexation with cucurbit[7]uril. Chem. Comm. 58, 3617–3620. doi:10.1039/D1CC06982A
Moorthy, S., Lambert, H., Mohan, N., Schwarzlose, T., Nau, W. M., Kalenius, E., et al. (2023). Noncovalent modulation of chemoselectivity in the gas phase leads to a switchover in reaction type from heterolytic to homolytic to electrocyclic cleavage. Angew. Chem. Int. Ed. 62, e202303491. doi:10.1002/anie.202303491
Nau, W. M., Florea, M., and Assaf, K. I. (2011). Deep inside cucurbiturils: physical properties and volumes of their inner cavity determine the hydrophobic driving force for host–guest complexation. Isr. J. Chem. 51, 559–577. doi:10.1002/ijch.201100044
O'Brien, J., Wilson, I., Orton, T., and Pognan, F. (2000). Investigation of the Alamar Blue (resazurin) fluorescent dye for the assessment of mammalian cell cytotoxicity. Eur. J. Biochem. 267, 5421–5426. doi:10.1046/j.1432-1327.2000.01606.x
Ong, W., Gómez-Kaifer, M., and Kaifer, A. E. (2002). Cucurbit [7] uril: a very effective host for viologens and their cation radicals. Org. Lett. 4, 1791–1794. doi:10.1021/ol025869w
Präbst, K., Engelhardt, H., Ringgeler, S., and Hübner, H. (2017). Basic colorimetric proliferation assays: MTT, WST, and resazurin. Methods Mol. Biol. 1601, 1–17. doi:10.1007/978-1-4939-6960-9_1
Saleh, N., Koner, A. L., and Nau, W. M. (2008). Activation and stabilization of drugs by supramolecular pKa shifts: drug-delivery applications tailored for cucurbiturils. Angew. Chem. Int. Ed. 120, 5478–5481. doi:10.1002/ange.200801054
Uhlenheuer, D. A., Petkau, K., and Brunsveld, L. (2010). Combining supramolecular chemistry with biology. Chem. Soc. Rev. 39, 2817–2826. doi:10.1039/B820283B
Walker, S., Oun, R., McInnes, F. J., and Wheate, N. J. (2011). The potential of cucurbit[n]urils in drug delivery. Isr. J. Chem. 51, 616–624. doi:10.1002/ijch.201100033
Keywords: supramolecular electrochemistry, host-guest complexes, reactivity modulation, resazurin, cucurbituril
Citation: Liu J, He S-H, Lambert H and Lee T-C (2023) Modulation of redox reactivity of resazurin through host-guest complexation with Cucurbit[n]uril (n = 7, 8). Front. Chem. 11:1295715. doi: 10.3389/fchem.2023.1295715
Received: 17 September 2023; Accepted: 01 December 2023;
Published: 14 December 2023.
Edited by:
Kai Chen, Nanjing Tech University, ChinaReviewed by:
Jing-Xin Liu, Anhui University of Technology, ChinaAnthony Ivan Day, University of New South Wales, Australia
Copyright © 2023 Liu, He, Lambert and Lee. This is an open-access article distributed under the terms of the Creative Commons Attribution License (CC BY). The use, distribution or reproduction in other forums is permitted, provided the original author(s) and the copyright owner(s) are credited and that the original publication in this journal is cited, in accordance with accepted academic practice. No use, distribution or reproduction is permitted which does not comply with these terms.
*Correspondence: Tung-Chun Lee, tungchun.lee@ucl.ac.uk; Su-Hang He, shhe404@gmail.com