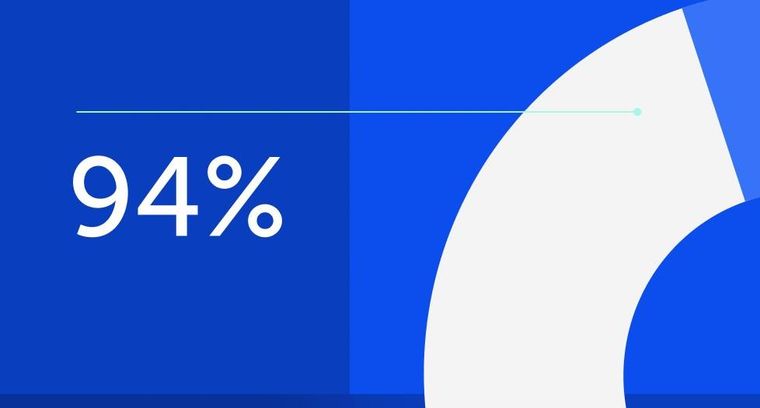
94% of researchers rate our articles as excellent or good
Learn more about the work of our research integrity team to safeguard the quality of each article we publish.
Find out more
ORIGINAL RESEARCH article
Front. Chem., 08 December 2023
Sec. Medicinal and Pharmaceutical Chemistry
Volume 11 - 2023 | https://doi.org/10.3389/fchem.2023.1287317
This article is part of the Research TopicMedicinal Chemistry of Active Pharmaceutical Ingredients of Drug ProductsView all 8 articles
Innovative approaches are urgently required to treat divestating bacterial diseases in the face of rising bacterial resistance rates. The current investigative work focused on hydro-distilling Tasmanian blue gum (Eucalyptus globulus) to isolate the essential oil, which was then tested for bioactivity, antioxidant capacity, and antibacterial activity using in-vitro and in silico assays. The antioxidant activity was avualated against DPPH and FRAP. With results of 69.63 RSA (%) (µL/L AAE) at a concentration of 5 mL/L and 51.56 (µL/L AAE) at concentration of 90 ppm in the 2,20-diphenyl-1-picryl hydrazyl (DPPH) and ferric reducing antioxidant power (FRAP) assays, respectively, the extracted oil indicated considerable antioxidant activity. The extracted oil demonstrated powerful antibacterial activity in in-vitro tests against both Gram-positive and Gram-negative bacterial strains, including Bordetella bronchiseptica (21 mm), Staphylococcus epidermidis (19 mm), and Staphylococcus aureus (19 mm), with significant minimal inhibitory (MIC) and minimum bactericidal (MIB) concentrations. Additionally, GC-MS analysis of the oil from E. globulus identified several low-molecular-weight compounds, including Eucalyptol, γ-Terpinene, Shisool acetate, 1,3-trans,5-cis-Octatriene, 2,6-Dimethyl-1,3,5,7-octatetraene, E,E, Cyclohexene, 1-methyl-4-(1-methylethylidene), Benzene, 1-methyl-4-(1-methylethenyl), Butanoic acid, 3-methyl-, 3-methylbutyl ester, and 1,3,8-p-Menthatriene. Several other compounds were also identified, including Fenchol, 2-Methyl-trans-3a,4,7,7a-tetrahydroindane, (E,E,E)-2,4,6-Octatriene, 1,2,3,6-Tetrahydrobenzylalcohol, acetate, Alloaromadendrene, Phenol, 2-ethyl-4,5-dimethyl, Phenol, 2-methyl-5-(1-methylethyl)-, p-Cymen-7-ol, 1,5,5-Trimethyl-6-methylene-cyclohexene, 1,3-Cyclohexadiene, 1-methyl-4-(1-methylethyl)-, 2,6-Octadien-1-ol, 3,7-dimethyl-, acetate, (Z), and more. The bioactive potential of Eucalyptus globulus essential oil against 1AJ6 and 1R4U was highlighted by molecular docking analyses, suggesting its utility as a natural source of antioxidant and antibacterial compounds with the potential to replace chemical disinfectants in a variety of applications.
Traditional Herbal medicine has always been the cheapest and easiest way to treat people in primary healthcare systems in places with few resources. Local people have been using plants as medicine for a long time. Fossils showed that people have been using plants as medicine since the Middle Paleolithic era, which gives them an edge here (often hundreds or thousands of years) (Rafat et al., 2010). Since the beginning, people have used plants as medicine to treat illnesses in the ensuing years (Prakash and Gupta, 2005). Indigenous healthcare systems worldwide use medicinal plants as essential to their care (Farnsworth, 1990). Most things sold as “traditional herbal remedies” have been around for a long time, and many herbal medicines on the market today are the same way. Even though modern medicine and these old-fashioned methods can work together, herbal treatments have often stayed popular (Vishwakarma et al., 2013). In the past few years, it has become more common to use knowledge from traditional medicine to study plants (Jones et al., 2006).
Several secondary metabolites produced by medicinal plants have potent antibacterial properties, which have long been used in traditional medicine. According to research, some herbs have been shown to help with skin cleaning. Processed extracts of these plants are often used to scrub the skin. Eucalyptus robusta and Aloe barbadensis are two of the most often utilized plants for disinfection in the home. For respiratory infections, Andrographis paniculata, Berberis vulgaris, and Cinnamomum verum are among the most often utilized herbs as antimicrobial and antiseptics (Dixit and Mittal, 2013). Various germ-killing plants were tested in research on herbal hand washes and sanitizers Tests of polyherbal handwashes produced and tested to fight Putida vulgaris and other skin illnesses discovered that they were all ineffective against these bacteria (Saad et al., 2011). There have been reports of bacteriostatic effects on hands and inanimate objects for a variety of plant mixtures. Using herbal hand sanitizer, Coleus vettiveroides, Coriandrum sativum and Citrus limon, Vetiveria zizaniides, and Azadirachta indica extracts were shown to be safe, effective, and had high antibacterial and cooling properties. Compared to the reference product, the herbal hand soap proved to be efficient and safe against E. coli, Pseudomonas aeuroginosa, Staphylococcus aureus, Bacillus subtilis, Saccharomyces cerevisie, and Candida albicans (Kadapure et al., 2022). Herbal toothpowder was also found to be as effective as commercial brands including Colgate and Pepsodent (Vohra et al., 2012). Plants that are aromatic and medicinal produce secondary compounds such as terpenoids, alcoholic compounds (such as geraniol and menthol), aldehydes (like citral and carvone camphor), acidic compounds (like benzoic acid, cinnamic acid, and myristic acid), aldehydes (like citral and carvone camphor), and ketonic bodies (such as thymol) that are used in aromatherapy (e.g., ascaridole, anethole) (Koul et al., 2008).
Since ancient times, the Eucalyptus tree has been used as an ethnomedicinal plant and is currently one of the most frequently planted genera in the Myrtaceae. This is a fast-growing tree that will thrive in any environment. Flu, fever, sore throat, respiratory tract infections, urinary tract infections, and bronchial infections may be treated with eucalyptus trees. The species’ anti-cancer properties have also been shown (Damjanović-Vratnica et al., 2011). As a result, Eucalyptus spp. Essential oil is widely used all over the globe. Since this achievement, the utilization of these chemicals as raw materials in the production of food, medicine, and cosmetics has expanded significantly (Fabiyi et al., 2020). A large variety of foods and drinks include eucalyptol, which is utilized in flavoring, scenting, and cosmetics as well, as well (Harkat-Madouri et al., 2015). Oil from eucalyptus leaves may be used to treat a variety of ailments. This ingredient might be used in dental care products due to its anti-inflammatory and antifungal properties, as well as its insecticide and acaricidal actions (Vigo et al., 2004). The Widespread usage of essential oil of medicinal plants as food and beverage flavorings, medicinal perfumes, and other product scenting agents attests to the versatility of their aroma distributed globally (Clark, 1996).
The World Health Organization (WHO) strategically incorporates the use of plant medicines in accordance with established conventional medical practices. The cultivation and processing of medicinal plants, utilizing established agro-industrial techniques, are of utmost significance in the manufacturing of herbal medicines. It is worth noting that modern pharmaceuticals frequently utilize components derived from medicinal plants (Newman and Cragg, 2007). Considering the increasing prevalence of bacterial resistance, there is a need for innovative interventions, despite the availability of established and well-validated methods for mitigating bacterial infections. Within this particular context, herbal medicine presents itself as a promising avenue for addressing pathogenic bacteria, showcasing potential opportunities for commercial utilization.
Natural goods are far more important than synthetic equivalents because of their inherent biological compatibility, lower toxicity, and wider range of bioactivity. Additionally, they support sustainability issues, provide a variety of solutions across different industries, and meet the market’s rising desire for natural and organic goods (Yadav et al., 2022). Furthermore, the in silico methodology is a cutting-edge and environmentally friendly way to assess the biological potential of bioactive substances derived from plants. It eliminates the need for time-consuming lab studies by using computational algorithms to predict pharmacological qualities quickly and affordably. This eco-conscious methodology is a useful tool for rapidly investigating bioactive compounds in plant-based medicine and other sectors because it not only speeds up drug discovery but also helps with compound design optimization (Aldossari et al., 2023; Yusuf, 2023).
Taking into consideration the existing challenges, the objective of this study is to utilize the inherent properties of medicinal plants to effectively combat bacterial infections. This research aims to isolate essential oils with therapeutic potential, specifically focusing on Eucalyptus globulus. The study aims to utilize the antimicrobial properties of these essential oils to make a valuable contribution to the advancement of alternative disinfectants and antiseptic agents. The investigation focuses on enhancing the interaction between alcohol and phenolic compounds, taking advantage of their volatility and reactivity, to produce effective formulations with minimal contact time. The objective of this study is to develop a new methodology based on the utilization of indigenous medicinal plants, with the aim of providing a potential solution for combating bacterial pathogens.
Fresh leaves of E. globulus were collated from Islamabad, Pakistan. The plant was identified by Dr. Hassan Javed (Department of Plant Science) at Quaid I Azam University (QAU), Islamabad, and given a voucher specimen NCBI:txid34317. The sample was washed with DdH2O to eradicate the contaminations, then the fresh and washed leaves were cut into pieces (Chahomchuen et al., 2020).
To prepare leaves solution for oil extraction, 50 g fresh leaves were thoroughly mixed with 100 mL DdH2O in a 300 mL volumetric flask. Then the prepared solution was hydro-distilled for 3 h in triplicate using a Clevenger-type device, following the procedure prescribed in the European Pharmacopoeia (Pharmacopoeia (2005). To prepare for further analysis, the oils were dried up over anhydrous sodium sulfate, separated, and stored at −4°C. The volume of basic oil divided by the weight of the leaves (v/w) was used to calculate the percentage of fundamental oil substance present in the leaves.
The chemical analysis and identification of bioactive compounds present in crude essential of E. globulus was done by using GCMS-QP 2010 Plus, Shimadzu, Japan working with electron ionization mode at 70 eV. Mass units were checked from 35 to 500 AMU. A DB-5 MS (30 m 0.25 mm id, 0.25 mm film thickness) fine segment. GC examination conditions and injector and indicator temperatures were the same as they were in this phase of the experiment as well (Stein et al., 2001), i.e., The oven’s temperature was set to rise from 50°C to 150°C at a rate of 3°C per minute, hold isothermal for 10 min, and then rise to 300°C at a rate of 10°C per minute. Peak area normalization was used to express the relative percentage of the chemical components in crude extracts as a percentage.
The preserved standard bacterial cultures of Cronobacter sakazakii (ATCC#29544), Pseudomonas aeruginosa (ATCC#10145), Listeria monocytogenes (ATCC#13932), Bordetella bronchiseptica (ATCC#4617,) Staphylococcus epidermidis (ATCC# 12228), and S. aureus (ATTC#9144), were collected from pathology laboratory of a Biogen life sciences pharma Islamabad, Pakistan. The bacterial strains used were freeze-dried on Petri dish plates.
A nutritional media for juvenile culture was prepared by dissolving 6 g of nutrient broth in 100 mL of distilled water for the development of bacterial inoculation. When the pH was adjusted to 7.0, then autoclaved, and then put onto Petri plates (20 mL) in the laminar flow cabinet, the solution was ready for use.
A laminar flow cabinet was used to disinfect and label Petri dishes before C.r sakazakii (ATCC#29544), was introduced into one of the plates. All of the same procedures were used for the five bacteria, P. aeruginosa (ATCC#10145), L. monocytogenes (ATCC#13932), B. bronchiseptica (ATCC#4617,) S. epidermidis (ATCC# 12228) and S. aureus (ATTC#9144). It was then incubated at 37°C for 24 h on all Petri plates. On Petri plates, the bacteria were now active and ready to multiply.
For evaluating the in-vitro antimicrobial activity of the essential oils, a range of pathogenic Gram-negative and Gram-positive bacterial strains were selected from the American Type Culture Collection (ATCC). To initiate the experiment, all microbial cultures were streaked on agar slants and incubated at 3°C for 24 h. Subsequently, they were transferred to the broth for overnight growth.
A nutrient agar solution containing 6.3 g was dissolved in 120 mL of distilled water (DW). Subsequently, the agar solution and sterile Petri dishes underwent autoclaving at 121°C for 15 min. Post-sterilization, 40 mL of the agar solution was dispensed into each Petri dish and allowed to solidify for 20 min. Following solidification, the intended bacterial strain was inoculated onto each Petri dish utilizing the streak plate technique. After streaking, two wells were generated using a Cork borer and systematically labeled. Subsequently, 30 µL of Erythromycin (1 mg/mL) (used as the positive control) and 30 µL of the plant oil extract solution (1 mg/mL) were introduced into the respective wells. The Petri dishes were then incubated at 37°C for 24 h. The entire procedural operation occurred within a laminar flow cabinet under aseptic laboratory conditions (Magaldi et al., 2004).
The assessment of MICs and MBCs for E. globulus Essential Oils (EOs) were carried out employing the microdilution technique within 96-well microplates (Newman and Cragg, 2007). The experimental procedures were conducted employing Mueller-Hinton (MH) broth as the growth medium. Microbial cell suspensions were rendered at a concentration of 10^6 colony-forming units per milliliter (cfu/mL) by three-fold dilution with 0.5% sodium chloride solution (0.5% NaCl). This dilution process achieved an optical density of 0.1 at 600 nm, as determined by a NanoDrop 2000c Spectrophotometer (Thermo Scientific, United States). Subsequently, the bacterial solution (100 µL) was added to each well of a 96-well plate.
Employing two-fold serial dilutions, a sequence of oil concentrations spanning from 4% to 0.625% was introduced to the subsequent wells, resulting in a final well volume of 100 µL. The negative control wells received 100 µL of MH broth, while the positive control wells contained 100 µL of bacterial inoculum devoid of the oil. The microplates were then incubated at 37°C for 24 h. After incubation, the optical density (OD) was quantified at 595 nm utilizing a microplate reader (iMark Microplate Reader, Bio-RAD, United States).
The determination of MIC was predicated on the lowest dilution displaying observable growth. In each well, 5 µL of a resazurin indicator solution was introduced. After a 30-min incubation at 37°C, the MIC assay outcomes were corroborated through color alteration. The presence of viable bacteria induced a discernible pinkish hue in the resazurin indicator. The MBC, signifying the concentration at which microbial growth was entirely inhibited and color alteration halted, was determined. The MBC was substantiated by applying 5 µL from the well corresponding to the MIC or higher concentrations onto MH agar plates. Following a 24-h incubation at 37°C, the absence of any growth on the agar corroborated the MBC value.
By measuring the percentage decrease in bacterial count over some time while in the presence of extract at its MIC, time–kill tests may be used to assess the antibacterial activity of EOs. The effectiveness of EOs against strains was determined by quantifying the decrease in CFU per milliliter during 24 h, as reported by Song et al. (2006). Quantification using this technique should not be more than 102 CFU.
Measuring the ability of essential oils to search 2,20 -diphenyl-1-picryl hydrazyl (DPPH) stable fundamental was the key to determining their activity as an antioxidant. The assay was carried out spectrophotometrically according to the instructions provided by Harkat-Madouri et al. (2015); Chahomchuen et al. (2020), Shimada et al. (1992); Akolade et al. (2012). Ascorbic acid was used as standard solution and RSA (%) (µL/L AAE) was calculated the following formula.
Where Abs STD is absorbance of standard and Abs SPL is absorbance of sample.
The method of EFabiyi et al. (2020) followed for the reduction ability of crucial oils were accessed using this process. Samples were compered with ascorbic standared caruve to find out AAE of the extracted oil using the following formula.
The molecular docking methodology used in this study involved several software and tools. First, the crystal structures of the antioxidant and antimicrobial target proteins were obtained from the RCSB protein data bank. The PDB IDs of the proteins were 1AJ6 (National Center for Biotechnology Information, 1998) and 1R4U (RCSB, 2004). The decision to utilize the crystal structures of proteins (PDB IDs: 1AJ6 and 1R4U) for molecular docking in the study is considered due to their availability, potential functional relevance, and diversity of target representation. While the exact roles of these proteins in the metabolism of the selected bacteria were not explicitly mentioned, their selection is stem from factors such as known biological functions, structural characteristics, possible implications for human health, and the potential for uncovering novel interactions. These proteins also share binding site similarities with metabolic enzymes, facilitating insights into broader cellular mechanisms. Technical feasibility and compatibility with available software tools have further influenced their choice of docking targets. To prepare the protein structures for docking, we used BIOVIA’s Discovery Studio Visualizer (Morris et al., 2009) and Autodock tools (Visualizer, 2005; Bilal et al., 2022). This involved removing heteroatoms, co-crystal ligands, and solvent molecules, and optimizing the protein for docking. Next, the ligands were designed and drawn in ChemDraw Ultra (Channar et al., 2022), and their energy was minimized using Chem3D Pro (Ferreira et al., 2015). The ligands were then converted to pdbqt files using OpenBabel GUI (Zentgraf et al., 2007). In AutoDock, the grid box was built using a size 60 × 60 × 60 Å, pointing for 1AJ6 and 1R4U receptors, X = 60.02, Y = −7.77, Z = 34.74 and X = 44.35, Y = 40.91, Z = 30.47 coordinates, respectively, with a grid point spacing of 0.375 Å for each. The centre of the grid box was adjusted to cover the active pocket. To perform the docking, we used Autodock vina (Yusuf et al., 2008; Morris et al., 2009; Trott and Olson, 2010; Eberhardt et al., 2021). The ligands were docked into the active site of the protein, and the resulting ligand-protein interactions were analyzed using BIOVIA’s Discovery Studio Visualizer.
With the help of Microsoft Excel 2013, the mean value and standard deviations were determined. Analysis of variance (Ahmadov et al., 2020) was used to analyze the data by determining the differences between means for implication at P 0.05 using SPSS’s Duncan’s numerous range test (version 16.0) (Khan et al., 2019).
Fresh leaves of E. globulus were collated from Islamabad. The plant was identified with the help of a taxonomist at QAU, Islamabad. The samples were washed with DdH2O to eradicate the contamination, and then the fresh and washed leaves were cut into pieces.
Essential oils were by using a Clevenger-type device, following the procedure prescribed in the European Pharmacopoeia, according to the protocol (Yadav et al., 2022). To prepare for further analysis, the oils were dried up over anhydrous sodium sulfate, separated, and stored at - 4°C. The volume of basic oil divided by the weight of the leaves (v/w) was used to calculate the percentage of fundamental oil substance present in the leavesi.e., 1 mL/100 g leaves (Figure 1).
The constituents of the yellow volatile oil of E. globulus were detected by GC-MS Chromatogram, and the compounds in the oil extract were characterized based on GC-MS evidence with different retention times (Supplementary Figure S1). The analysis of complex mixtures such as crude oil identified some low molecular weight compounds Eucalyptol, Gamma.-Terpinene, Shisool acetate, 1,3-trans,5-cis-Octatriene, 2,6-Dimethyl-1,3,5,7-octatetraene, E, E, Cyclohexene, 1-methyl-4-(1-methylethylidene)-, Benzene, 1-methyl-4-(1-methylethenyl)- Butanoic acid, 3-methyl-, 3-methylbutyl ester, 1,3,8-p-Menthatriene shown in (Table 1). In addition to that several other compounds were also found, i.e., Fenchol 2-Methyl-trans-3a,4,7,7a-tetrahydroindane, (E,E,E)-2,4,6-Octatriene, 1,2,3,6-Tetrahydrobenzylalcohol, acetate, Alloaromadendrene,Phenol, 2-ethyl-4,5-dimethy,Phenol, 2-methyl-5-(1-methylethyl)-,p-Cymen-7-ol,1,5,5-Trimethyl-6-methylene-cyclohexene, 1,3-Cyclohexadiene, 1-methyl-4-(1-methylethyl)-,2,6-Octadien-1-ol, 3,7-dimethyl-, acetate, (Z) etc. The large compound fragments compared to small compounds gave a taller appearance of peaks at different m/z ratios for 28 min (Figures 2–7). The small peaks could be caused by bioactive compounds that are present in small amounts or by major compounds that have broken down. Due to these bio-active components, in the last several years, the application of Eucalyptus oil has become a significant field of health- and medical-related study. Earlier research was also documented by Sharma, and Kaur, (Pharmacopoeia, 2005).
According to Sumitha & Thoppil, (2016), analyzing the chemical composition of leaf essential oil was accomplished using gas chromatography-mass spectrometry (GC/MS). GC/MS research revealed that O. gratissimum essential oil consists of 51 distinct components. 4-(5-methyl-1-azabicyclo (2, 2) octan-2), and 1-(2, 5-dimethoxyphenyl)-propyl-benzene were the most common compounds (5.53 percent). 24 h after inhaling the essential oil, the oil had an LC50 value of 26.10 parts per million, and an LC90 value of 82.83% of that value (Stein et al., 2001).
In another study, Panyajai, et al., 2020 studied that The Algerian wild plant Elaeoselinum thapsioides had its leaves and stem hydro-distilled to get an essential oil. This oil was analyzed by GC-MS for the first time. 45 compounds were found, which made up 93.8 percent of the total oil. The oil had a high amount of hydrocarbon derivatives of monoterpenes (75.9 percent). Myrcene made up 61% of the essential oil, followed by germacrene D (10.3%), -pinene (6.5%), and -pinene (2.9%).
Antibacterial activities of extracted oil of E. globulus were investigated against various pathogenic bacteria, such as C. sakazakii (ATCC#29544), P. aeruginosa (ATCC#10145), L. monocytogenes (ATCC#13932), B. bronchiseptica (ATCC#4617) S. epidermidis (ATCC# 12228) and S. aureus (ATTC#9144) using the well diffusion method. Extracted oil of E. globulus showed antibacterial activity against both Gram-positive and negative bacteria. The diameter of inhibition zones (mm) around each well with extracted oil of E. globulus is represented in (Figures 8A, B). The antimicrobial activity of extracted oil of E. globulus was found against (Figure 9A) (A) (Cronobactersakazakii (Zone of inhibition 17 mm), (B) P. aeruginosa (18 mm), (C) L. monocytogenes (Zone of inhibition 16 mm), (Figure 9B), (A) B. bronchiseptica (21 mm), (B) S. epidermidis (Zone of inhibition 19 mm), and (C) S. aureus (Zone of inhibition 19 mm), pathogenic bacterial strains.
FIGURE 8. (A) Inhibition zone of extracted oil of E. globulus against Gram-negative (Std) positive control (Spl). (B) Inhibition zone of extracted oil of E. globulus against Gram-positive (Std) positive control (Spl).
Many researchers have investigated the antibacterial properties of essential oils and different polarity of crude plant extracts against a variety of bacterial strains. (Magaldi et al., 2004; Song et al., 2006). They found that the essential oils had a strong antibacterial effect on the bacterial strains they were tested against. The primary chemical components are responsible for the plant essential oil’s considerable biological actions. This research work shows great antibacterial activities against both Gram-negative and Gram positive similar to as reported in previous literature.
Bacterial growth was measured when exposed to Eos at the minimum inhibitory concentration (MIC) (Figure 9). In fact, after just 1 h of incubation, bacterial growth was decreased when Eos was present at concentrations comparable to their MIC. This significant impact may be attributable to carvacrol’s ability to depolarize and weaken the membrane that surrounds the cytoplasm. These tissues are a vital biological target for EO components because of their hydrophobic nature. Some antimicrobials, like thyme, can kill microbes by destroying their whole cell membranes. Also, carvacrol and thymol are structural isomers in that they vary in the position of a hydroxyl group on the phenolic ring. The hydroxyl group makes them more hydrophilic, which may facilitate their solubility in the microbial membrane and subsequent alterations to the membrane.
MICs and MBCs were determined for the five reference strains listed in (Table 2) to assess the antibacterial activity of the E. globulus oil extract. The minimum inhibitory concentration (MIC) for C. sakazakii (ATCC#29544), was 0.4% (v/v), whereas the minimum inhibitory concentration (MBC) was 3% (v/v) (Table 2). The minimal inhibitory concentration (MIC) and minimum inhibitory concentration (MBC) values for P. aeruginosa (ATCC#10145, L. monocytogenes (ATCC#13932), B. bronchiseptica (ATCC#4617) S.s epidermidis (ATCC# 12228) and S. aureus (ATTC#9144) were all 2% (v/v) and 4% (v/v), respectively. These findings suggested that oil extracted from E. citriodora might be antibacterial against the microorganisms used in the study. This was discovered by examining the cytotoxic and antibacterial effects on fibroblast cell lines (MIC, MBC and agar disc diffusion technique). As with NLP-subcytotoxic ZMEO, the results were better in comparison to MIC/MBC values for multidrug-resistant bacteria and the chlorhexidine dosages examined (Shimada et al., 1992). Our result also was comparable to the above literature.
TABLE 2. Minimal inhibitory concentrations (MIC) and minimum bactericidal concentrations (MBC) for the antibacterial activity of E. globulus oil.
In the present study antioxidant activities of essential oil extracted from Eucalyptus globulus were estimated by DPPH. Polyphenols have an ability that is called as H-ion donating ability due to which a toxic and reactive oxygen species could be converted to nontoxic one. For determining the antioxidant activity, the reaction with DPPH was hoped to be quick in action due to its rapid switching of labile hydrogen to radicals. There was formation of purple colored solution and absorbance was taken at 517 nm. Standard of a curve of Ascorbic acid. Antioxidants were present in the Eucalyptus extracts that faded the dark colour of the solution. Technique used for extraction was maceration and DPPH assay final calculations were shown as ascorbic acid equivalent of Eucalyptus extracts. Methanolic extract was used for testing Eucalyptus is scavenging activity (Figure 10).
The FRAP method is the sharp, less difficult, cheap, renewable, and highly achievable method. Antioxidant contents have a significantly high reducing power due to which they can easily and effectively reduce ferric to ferrous ions. As the concentration of extract is increased this reducing power will also increase. In the present research work,a regular increase in the reduction potential was observed as the concentration of the tested extract increased. The reduction potential of the oil extracted from E. globulus was measured over the concentrations ranging from 50 to 90 ppm showing 24.26 to 51.56 AAE (µL/L) shown in (Figure 11). Antioxidants may reduce compounds because they may stabilize radicals by giving up electrons or hydrogen atoms to make radicals less reactive. Osawa et al. reported that E. cinerea, E. cosmophylla, E. globulus, E. perriniana and E. viminalis have antioxidant properties due to the presence of 𝛽-diketone and ellagic acid. In another study, Singhat al., 2012 analysis of essential oil by gaschromatography–mass spectrometry (GC–MS) revealed the presence of 43 components constituting 99.2% of the oil of E. citriodora. This research work also shows antioxidant activities similar to previous literature.
Eucalyptus globulus oil is utilized in pharmaceutical preparations as an anti-diabetic, antioxidative, antiviral, antibacterial, antifungal, antitumor, antihistaminic, anticancer cytochrome p450 inhibitor and hepatoprotective agent, as well as in goods like cough sweets, lozenges, ointments, toothpaste, and inhalants. Extracted oil of E. globulus, its potential health benefits because safe and effective, extracted oil of E. globulus product can be widely used as a medicinal plant using GC-MS technique as a rapid, simple, low cost for low molecular weight organic analysis.
Table 3 provides information on the ligands that bind to the antioxidant protein with the code 1AJ6, along with their binding scores (in Kcal/mol) and the residues in the binding pocket of the protein that interact with the ligands, along with the distance (Fabiyi et al., 2020) between the ligand and the interacting residues. The table lists 18 ligands, numbered from 1 to 18. For each ligand, the chemical name, binding score, and interacting residues with their distances are listed. The interacting residues are listed using their three-letter amino acid code followed by the position number in the protein sequence in parentheses. The distance between the ligand and the residue is given in angstroms (Å).
TABLE 3. Ligand Codes with Chemical names, Molecular docking scores and binding interactions with distance for 1AJ6 and 1R4U.
Upon analyzing the ligand binding scores and the interaction residues of the antioxidant protein 1AJ6, we can compare the different ligands’ binding affinities to the protein as shown in Table 3 and Supplementary Table S5. Eucalyptol has a binding score of −4.8 kcal/mol and interacts with GLY119, IIE94, and ALA100 residues. Gamma-Terpinene has the highest binding affinity with a score of −5.6 kcal/mol and interacts with Asn46, Glu50, Asp73, Ile78, Met95, Val120, and Thr165 residues. The 1,3-trans,5-cis-Octatriene has a binding score of −4.1 kcal/mol and interacts with VAL43, MET95, ALA47, VAL71, VAL167, VAL120, and ILE78 residues. 2,6-Dimethyl-1,3,5,7-octatetraene, E,E has a binding score of −4.2 kcal/mol and interacts with VAL43, ALA47, VAL120, VAL167, and ILE78 residues. Cyclohexene, 1-methyl-4-(1-methylethylidene)- has a binding score of −6.0 kcal/mol and interacts with ILE78, VAL43, VAL120, VAL167, GLU50, THR165, and ASN46 residues. Benzene, 1-methyl-4-(1-methylethenyl)- has a binding score of −5.5 kcal/mol and interacts with ASN46, VAL43, VAL120, VAL167, ILE78, THR165, MET95, and GLU50 residues. Butanoic acid, 3-methyl-, 3-methylbutyl ester has a binding score of −5.8 kcal/mol and interacts with THR165, ILE78, MET95, VAL120, LEU132, and VAL167 residues. 1,3,8-p-Menthatriene has a binding score of −5.5 kcal/mol and interacts with ASN46, VAL43, VAL120, VAL167, ILE78, and MET95 residues. Fenchol has a binding score of −4.5 kcal/mol and interacts with ILE94 and ALA100 residues (E,E,E)-2,4,6-Octatriene has a binding score of −4.5 kcal/mol and interacts with ALA47, ILE78, VAL43, VAL71, and VAL167 residues. 1,2,3,6-Tetrahydrobenzylalcohol, acetate has a binding score of −5.8 kcal/mol and interacts with GLY77, THR165, and ALA47 residues. Alloaromadendrene has the highest binding affinity with a score of −6.2 kcal/mol and interacts with Arg190 and PHE41 residues. Phenol, 2-ethyl-4,5-dimethy has a binding score of −5.7 kcal/mol and interacts with ASP73, THR165, ALA47, VAL43, VAL71, VAL167, and ILE78 residues. Phenol, 2-methyl-5-(1-methylethyl)- has a binding score of −6.1 kcal/mol and interacts with ASN46, VAL43, VAL120, VAL167, ILE78, and THR165 residues. p-Cymen-7-ol has a binding score of −5.7 kcal/mol and interacts with VAL71, THR165, and ALA47 residues. 1,5,5-Trimethyl-6-methylene-cyclohexene has a binding score of −4.5kval.mol, indicating a relatively strong binding. The amino acid residues involved in the interaction are ILE94 and ALA100, which are both hydrophobic amino acids, and the distance between the ligand and these residues is 5.42 and 4.32 Å, respectively. 1,3-Cyclohexadiene, 1-methyl-4-(1-methylethyl)-has a higher binding score of −5.6, indicating an even stronger binding affinity. The amino acid residues involved in the interaction are ASN46, VAL43, VAL120, VAL167, and ILE78, which are all hydrophobic residues, and the distances range from 3.64 to 5.07 Å. Lastly, 2,6-Octadien-1-ol, 3,7-dimethyl-, acetate, (Z), the binding score is −5.0, indicating strong binding. The amino acid residues involved in the interaction are ARG76, ILE78, VAL120, VAL43, and VAL167, which are also hydrophobic residues, and the distances range from 2.72 to 5.39 Å. Among the analyzed ligands, Alloaromadendrene showed the highest binding affinity of −6.9 kcal/mol, surpassing the native ligand novobiocin’s binding score of −6.4 kcal/mol against the antimicrobial protein 1R4U.
The results of the molecular docking analysis of 18 ligands against the anti-microbial protein 1R4U were obtained, and the binding scores and the interacting residues and distance in the binding pocket were recorded and presented in Table 3 and shown in Supplementary Table S6. The analysis revealed that the ligand Eucalyptol has the highest binding score of −5.1 kcal/mol, and it interacts with the residues AGR105, TRP208, AGR105, and AGR128, with distances of 2.74, 3.91, 5.05, and 5.26 Å, respectively. Gamma.-Terpinene had the second highest binding score of −5.0 kcal/mol, and it interacts with the residues CYS103, PRO76, CYS103, TYR30, PRO76, and ARG128, with distances of 3.90, 4.38, 4.47, 4.86, 5.38, and 5.33 Å, respectively. 1,3-trans,5-cis-Octatriene had a binding score of −4.3 kcal/mol and interacted with the residues VAL73, PRO76, PRO76, CYS103, CYS103, MET32, MET32, CYS103, and TYR30, with distances of 5.28, 5.01, 4.26, 4.05, 4.22, 4.72, 3.59, 3.83, and 4.86 Å, respectively. 2,6-Dimethyl-1,3,5,7-octatetraene, E,E had a binding score of −4.8 kcal/mol and interacted with the residues CYS103, TYR30, TYR30, with distances of 3.77, 5.09, and 5.17 Å, respectively. Cyclohexene, 1-methyl-4-(1-methylethylidene)- had a binding score of −5.4 kcal/mol and interacted with the residues CYS103 and TYR30, with distances of 5.10 Å and 4.83 Å, respectively. Benzene, 1-methyl-4-(1-methylethenyl)- had a binding score of −5.0 kcal/mol and interacted with the residues TRP208, TRP208, ARG105, ARG128, CYS103, ARG105, ARG128, TRP208, TRP208, TRP208, and ARG128, with distances of 5.24, 4.02, 4.52, 4.28, 4.67, 3.98, 4.35, 5.07, 4.72, 4.91, and 5.41 Å, respectively. Butanoic acid, 3-methyl-, 3-methylbutyl ester had a binding score of −4.9 kcal/mol and interacted with the residues TRP106, THR107, CYS103, and TYR30, with distances of 1.95, 2.84, 3.89, and 5.14 Å, respectively. 1,3,8-p-Menthatriene exhibited a binding affinity of −5.0 kcal/mol and was found to interact with key residues such as ARG105, ARG128, CYS103, and TRP208. Ligand 9, Fenchol, had a slightly higher binding affinity of −5.2 kcal/mol and interacted with HIS256 and ARG176. Ligand 10, (E,E,E)-2,4,6-Octatriene, showed a binding affinity of −3.8 kcal/mol and interacted with key residues including CYS103, ARG105, MET32, and VAL73.1,2,3,6-Tetrahydrobenzylalcohol, acetate, exhibited the highest binding affinity among all the ligands analyzed, with a value of −5.3 kcal/mol. It interacted with key residues such as TRP106, MET32, PRO76, CYS103, and TYR30. Ligand 12, Alloaromadendrene, showed a binding affinity of −6.9 kcal/mol and interacted with TRP208, CYS103, and TYR30. Phenol, 2-ethyl-4,5-dimethy, exhibited a binding affinity of −5.5 kcal/mol and interacted with key residues such as GLU31, TYR30, PRO76, and CYS103. Ligand 14, Phenol, 2-methyl-5-(1-methylethyl)-, showed a binding affinity of −5.6 kcal/mol and interacted with GLU31, HIS104, CYS103, and TYR30. Ligand 15, p-Cymen-7-ol, exhibited a binding affinity of −5.2 kcal/mol and interacted with key residues such as VAL29, TRP106, TYR30, and CYS103.1,5,5-Trimethyl-6-methylene-cyclohexene showed a binding affinity of −5.1 kcal/mol and interacted with key residues including PRO76, CYS103, and TYR30. Ligand 17, 1,3-Cyclohexadiene, 1-methyl-4-(1-methylethyl)-, exhibited a binding affinity of −5.0 kcal/mol and interacted with key residues such as CYS103, PRO76, TYR30, and ARG128. Finally, 2,6-Octadien-1-ol, 3,7-dimethyl-, acetate, (Z), exhibited a binding affinity of −5.2 kcal/mol and interacted with key residues such as GLU31, ARG105, ARG128, TRP208, and ARG105. Comparatively, the native ligand oxonic acid displayed a binding score of −4.8 kcal/mol, while the ligands Alloaromadendrene and Phenol, 2-methyl-5-(1-methyl ethyl)- exhibited notably stronger binding affinities of −6.9 and −5.6 kcal/mol, respectively, against the anti-microbial protein 1R4U.
The findings of this study underscore the unexplored potential of E. globulus leaves as a rich source of polyphenolic compounds endowed with notable antioxidant and antibacterial attributes. The essential oil derived from this plant emerges as a promising resource with potential health benefits, presenting a valuable avenue for combating various illnesses. A leading contribution of this study resides in the delineation of methodologies to formulate locally available and economically feasible disinfectants utilizing indigenous Pakistani flora. The integration of E. globulus aligns with the heightened demand for disinfectants amidst the COVID-19 pandemic, signifying its pertinence and timeliness. Furthermore, the molecular docking analysis augments the comprehension of intricate protein-ligand interactions, thereby furnishing a foundation for innovative therapeutic development. This study’s multifaceted insights offer a valuable reference for pharmacognosy or natural product researchers seeking to unearth novel therapeutic prospects from alternative perspectives. In summation, the present study’s outcomes substantiate E. globulus latent benefits, bridge the gap between traditional knowledge and contemporary healthcare needs, and facilitate a deeper exploration of potential therapeutic applications within the natural plant product domain.
The original contributions presented in the study are included in the article/Supplementary Material, further inquiries can be directed to the corresponding authors.
SN: Writing–original draft, Writing–review and editing. IK: Data curation, Writing–original draft. SM: Investigation, Writing–original draft. AH: Conceptualization, Writing–original draft. MU: Data curation, Writing–original draft. TS: Writing–original draft, Writing–review and editing. FS: Formal Analysis, Writing–original draft. AS: Investigation, Writing–original draft. AM: Supervision, Writing–original draft. MB: Supervision, Writing–original draft.
The author(s) declare financial support was received for the research, authorship, and/or publication of this article. This work is financially supported by the Researchers Supporting Project number (RSP-2023R437), King Saud University, Riyadh, Saudi Arabia, IUCN Policy statement. The collection of plant material complies with relevant institutional, national, and international guidelines and legislation.
The authors declare that the research was conducted in the absence of any commercial or financial relationships that could be construed as a potential conflict of interest.
All claims expressed in this article are solely those of the authors and do not necessarily represent those of their affiliated organizations, or those of the publisher, the editors and the reviewers. Any product that may be evaluated in this article, or claim that may be made by its manufacturer, is not guaranteed or endorsed by the publisher.
The Supplementary Material for this article can be found online at: https://www.frontiersin.org/articles/10.3389/fchem.2023.1287317/full#supplementary-material
Ahmadov, I. S., Bandaliyeva, A. A., Nasibova, A. N., Hasanova, F. V., and Khalilov, R. I. (2020). The synthesis of the silver nanodrugs in the medicinal plant Baikal skullcap (Scutellaria Baicalensis Georgi) and their antioxidant, antibacterial activity. Adv. Biol. Earth Sci. 5 (2), 103–118.
Akolade, J. O., Olajide, O. O., Afolayan, M. O., Akande, S. A., Idowu, D. I., and Orishadipe, A. T. (2012). Chemical composition, antioxidant and cytotoxic effects of Eucalyptus globulus grown in north-central Nigeria. J. Nat. Prod. Plant Resour. 2 (1), 1–8.
Aldossari, R. M., Ali, A., Rashid, S., Rehman, M. U., Bilal Ahmad, S., and Ahmad Malla, B. (2023). Insights on in-silico approaches for identifying potential bioactive inhibitors for TNF-α and IL-6 proteins associated with rheumatoid arthritis. Arabian J. Chem. 16 (11), 105200. doi:10.1016/j.arabjc.2023.105200
Bilal, M. S., Ejaz, S. A., Zargar, S., Akhtar, N., Wani, T. A., Riaz, N., et al. (2022). Computational investigation of 1, 3, 4 oxadiazole derivatives as lead inhibitors of VEGFR 2 in comparison with EGFR: density functional theory, molecular docking and molecular dynamics simulation studies. Biomolecules 12 (11), 1612. doi:10.3390/biom12111612
Chahomchuen, T., Insuan, O., and Insuan, W. (2020). Chemical profile of leaf essential oils from four Eucalyptus species from Thailand and their biological activities. Microchem. J. 158, 105248. doi:10.1016/j.microc.2020.105248
Channar, S. A., Channar, P. A., Saeed, A., Alsfouk, A. A., Ejaz, S. A., Ujan, R., et al. (2022). Exploring thiazole-linked thioureas using alkaline phosphatase assay, biochemical evaluation, computational analysis and structure–activity relationship (SAR) studies. Med. Chem. Res. 31 (10), 1792–1802. doi:10.1007/s00044-022-02945-4
Clark, A. M. (1996). Natural products as a resource for new drugs. Pharm. Res. 13 (8), 1133–1144. doi:10.1023/a:1016091631721
Damjanović-Vratnica, B., Đakov, T., Šuković, D., and Damjanović, J. (2011). Antimicrobial effect of essential oil isolated from Eucalyptus globulus Labill. from Montenegro. Czech J. Food Sci. 29 (3), 277–284. doi:10.17221/114/2009-cjfs
Dixit, P. K., and Mittal, S. (2013). An overview of herbal antimicrobial agents: boon for treatment of microbial infections. Int. J. Pharm. Res. Dev. 5 (6), 38–45.
Eberhardt, J., Santos-Martins, D., Tillack, A. F., and Forli, S. (2021). AutoDock Vina 1.2. 0: new docking methods, expanded force field, and python bindings. J. Chem. Inf. Model. 61 (8), 3891–3898. doi:10.1021/acs.jcim.1c00203
Fabiyi, O. A., Atolani, O., and Olatunji, G. A. (2020). Toxicity effect of Eucalyptus globulus on Pratylenchus spp. of Zea mays. Sarhad J. Agric. 36 (4). doi:10.17582/journal.sja/2020/36.4.1244.1253
Ferreira, L. G., dos Santos, R., Oliva, G., and Andricopulo, A. (2015). Molecular docking and structure-based drug design strategies. Molecules 20 (7), 13384–13421. doi:10.3390/molecules200713384
Harkat-Madouri, L., Asma, B., Madani, K., Bey-Ould Si Said, Z., Rigou, P., Grenier, D., et al. (2015). Chemical composition, antibacterial and antioxidant activities of essential oil of Eucalyptus globulus from Algeria. Industrial Crops Prod. 78, 148–153. doi:10.1016/j.indcrop.2015.10.015
Jones, W. P., Chin, Y.-W., and Kinghorn, A. D. (2006). The role of pharmacognosy in modern medicine and pharmacy. Curr. drug targets 7 (3), 247–264. doi:10.2174/138945006776054915
Kadapure, S. A., Kadapure, P., Anjali, C., Akansha, B., Sabera, B., Sanket, M., et al. (2022). Extraction of essential oil form sweet lime orange peel: a comparison study. Switzerland: Trans Tech Publ.
Khan, I., Ali, M., Aftab, M., Shakir, S., Qayyum, S., Haleem, K. S., et al. (2019). Mycoremediation: a treatment for heavy metal-polluted soil using indigenous metallotolerant fungi. Environ. Monit. Assess. 191 (10), 622–715. doi:10.1007/s10661-019-7781-9
Koul, O., Walia, S., and Dhaliwal, G. S. (2008). Essential oils as green pesticides: potential and constraints. Biopestic. Int. 4 (1), 63–84.
Magaldi, S., Mata-Essayag, S., Hartung de Capriles, C., Perez, C., Colella, M., Olaizola, C., et al. (2004). Well diffusion for antifungal susceptibility testing. Int. J. Infect. Dis. 8 (1), 39–45. doi:10.1016/j.ijid.2003.03.002
Morris, G. M., Huey, R., Lindstrom, W., Sanner, M. F., Belew, R. K., Goodsell, D. S., et al. (2009). AutoDock4 and AutoDockTools4: automated docking with selective receptor flexibility. J. Comput. Chem. 30 (16), 2785–2791. doi:10.1002/jcc.21256
National Center for Biotechnology Information (1998). NOVOBIOCIN-RESISTANT MUTANT (R136H) OF THE N-TERMINAL 24 KDA FRAGMENT OF DNA GYRASE B COMPLEXED WITH NOVOBIOCIN AT 2.3 ANGSTROMS RESOLUTION. Available from: https://www.rcsb.org/structure/1aj6.
Newman, D. J., and Cragg, G. M. (2007). Natural products as sources of new drugs over the last 25 years. J. Nat. Prod. 70 (3), 461–477. doi:10.1021/np068054v
Pharmacopoeia, E. (2005). Directorate for the quality of medicines of the council of europe (EDQM). Strasbourg: Council of Europe.
Prakash, P., and Gupta, N. (2005). Therapeutic uses of Ocimum sanctum Linn (Tulsi) with a note on eugenol and its pharmacological actions: a short review. Indian J. physiology Pharmacol. 49 (2), 125–131.
Rafat, A., Philip, K., and Muniandy, S. (2010). Antioxidant potential and phenolic content of ethanolic extract of selected Malaysian plants. Res. J. Biotechnol. 5, 1.
Saad, A. H., Gamil, S. N., Kadhim, R. B., and Samour, R. (2011). Formulation and evaluation of herbal handwash from Matricaria chamomilla flowers extracts. Int. J. Res. Ayur Pharm. 2, 1811–1813.
Shimada, K., Fujikawa, K., Yahara, K., and Nakamura, T. (1992). Antioxidative properties of xanthan on the autoxidation of soybean oil in cyclodextrin emulsion. J. Agric. food Chem. 40 (6), 945–948. doi:10.1021/jf00018a005
Song, J.-H., Kim, S. K., Chang, K. W., Han, S. K., Yi, H. K., and Jeon, J. G. (2006). In vitro inhibitory effects of Polygonum cuspidatum on bacterial viability and virulence factors of Streptococcus mutans and Streptococcus sobrinus. Archives Oral Biol. 51 (12), 1131–1140. doi:10.1016/j.archoralbio.2006.06.011
Stein, S., Mirokhin, Y., Tchekhovskoi, D., and Mallard, G. (2001). The NIST mass spectral search program for the NIST/EPA/NIH mass spectral library. Gaithersburg, MD, USA: NIST.
Trott, O., and Olson, A. J., (2010). AutoDock Vina: improving the speed and accuracy of docking with a new scoring function, efficient optimization, and multithreading. J. Comput. Chem. 31 (2), 455–461. doi:10.1002/jcc.21334
Vigo, E., Cepeda, A., Perez-Fernandez, R., and Gualillo, O. (2004). In-vitro anti-inflammatory effect of Eucalyptus globulus and Thymus vulgaris: nitric oxide inhibition in J774A. 1 murine macrophages. J. Pharm. Pharmacol. 56 (2), 257–263. doi:10.1211/0022357022665
Vishwakarma, A. P., Vishwe, A., Sahu, P., and Chaurasiya, A. (2013). Magical remedies of Terminalia arjuna (ROXB.). Int. J. Pharm. Archive 2 (8), 189–201.
Vohra, K., Sharma, M., Guarve, K., and Gobind, G. (2012). Evaluation of Herbal toothpowder and its comparison with various marketed toothpaste brands. Int. J. Green Herb. Chem. 1 (3), 271–276.
Yadav, M., Lal, K., Kumar, A., Kumar, A., and Kumar, D. (2022). Indole-chalcone linked 1, 2, 3-triazole hybrids: facile synthesis, antimicrobial evaluation and docking studies as potential antimicrobial agents. J. Mol. Struct. 1261, 132867. doi:10.1016/j.molstruc.2022.132867
Yusuf, D., Davis, A. M., Kleywegt, G. J., and Schmitt, S. (2008). An alternative method for the evaluation of docking performance: RSR vs RMSD. J. Chem. Inf. Model. 48 (7), 1411–1422. doi:10.1021/ci800084x
Yusuf, M. (2023). Insights into the in-silico research: current scenario, advantages, limits, and future perspectives. Life Silico 1 (1), 13–25.
Keywords: essential oils, Eucalyptus, disinfectants, GC-MS, molecular docking
Citation: Nasir Shah S, Khan I, Tul Muntaha S, Hayat A, Ur Rehman M, Ali Shah T, Siddique F, Salamatullah AM, Mekonnen AB and Bourhia M (2023) Bioactive, antioxidant and antimicrobial properties of chemically fingerprinted essential oils extracted from Eucalyptus globulus: in-vitro and in-silico investigations. Front. Chem. 11:1287317. doi: 10.3389/fchem.2023.1287317
Received: 01 September 2023; Accepted: 27 November 2023;
Published: 08 December 2023.
Edited by:
Shah Alam Khan, National University of Science and Technology, OmanCopyright © 2023 Nasir Shah, Khan, Tul Muntaha, Hayat, Ur Rehman, Ali Shah, Siddique, Salamatullah, Mekonnen and Bourhia. This is an open-access article distributed under the terms of the Creative Commons Attribution License (CC BY). The use, distribution or reproduction in other forums is permitted, provided the original author(s) and the copyright owner(s) are credited and that the original publication in this journal is cited, in accordance with accepted academic practice. No use, distribution or reproduction is permitted which does not comply with these terms.
*Correspondence: Ibrar Khan, YWJyYXJAYXVzdC5lZHUucGs=; Amare Bitew Mekonnen, YW1hcmViaXRldzhAZ21haWwuY29t
Disclaimer: All claims expressed in this article are solely those of the authors and do not necessarily represent those of their affiliated organizations, or those of the publisher, the editors and the reviewers. Any product that may be evaluated in this article or claim that may be made by its manufacturer is not guaranteed or endorsed by the publisher.
Research integrity at Frontiers
Learn more about the work of our research integrity team to safeguard the quality of each article we publish.