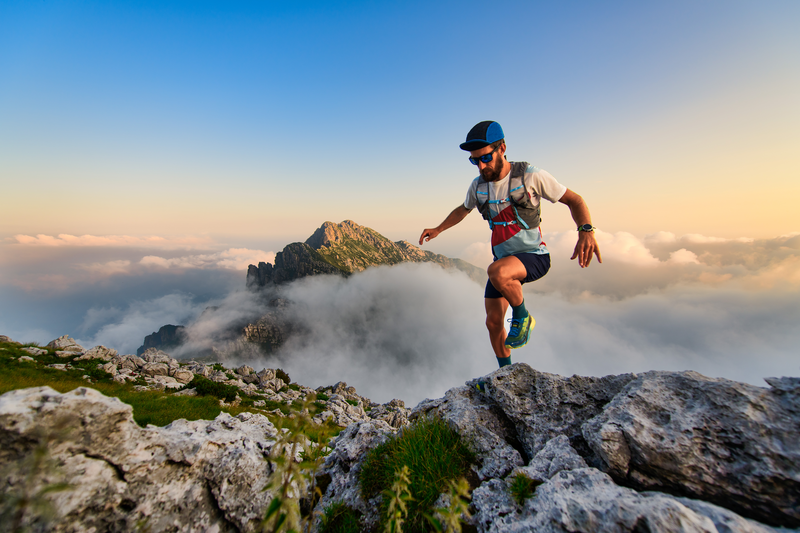
94% of researchers rate our articles as excellent or good
Learn more about the work of our research integrity team to safeguard the quality of each article we publish.
Find out more
PERSPECTIVE article
Front. Chem. , 09 October 2023
Sec. Electrochemistry
Volume 11 - 2023 | https://doi.org/10.3389/fchem.2023.1280816
This article is part of the Research Topic Functional Materials with Charge Transfer Properties and Their Application in Photoelectric Devices - Volume II View all 8 articles
Diketopyrrolopyrrole (DPP) and its derivatives, as electron deficient units, are widely used as building blocks in organic field-effect transistors, obtaining high performance. However, further modification of the DPP structure is crucial for the development of organic semiconductors. In this work, an FDPP is synthesized and characterized. The results show that FDPP exhibited not only a good planar core structure with a good conjugation system, but also strong aggregation in the solid state. As a consequence, FDPP presents p-type behavior with a hole mobility of ∼9.7 × 10−3 cm2 V−1 s−1. This study suggests that FDPP is a promising electron deficient unit for high performance semiconductors.
In the past few years, organic-field effect transistors (OFETs) have received significant attention by the academic society due to their multiple advantages and potential applications, such as solution processing, lightweight, large area, and compatibility with flexible substrates. (Surya et al., 2019; Sonalin et al., 2020; Zhu et al., 2020; Chen et al., 2022; Shibuya et al., 2022; Zhang et al., 2022; Mooney et al., 2023). Rapid progress in the field of OFETs has led to a renaissance in the chemistry of dye molecules, compelling scientists to revitalize well-known and relatively new, so-called high-performance pigments such as diketopyrrolopyrroles (DPPs), (Zhang et al., 2020a), isoindigo, (Zhang et al., 2020b), quinacridones, (Yuvaraja et al., 2020), and naphthodifuranone (Tao et al., 2022). Thus, an increasing number of researchers have focused their efforts on the development of high-performance pigments with high charge transport mobility (Ali and Siddiqui, 2022). Among these, diketopyrrolopyrrole, indigo, and their derivatives, as electron deficient building bulks, are very popular and promising for the design high-performance semiconductors (Shaker et al., 2020; Li et al., 2022; Lu et al., 2022).
For organic semiconductors, the charge transport mobility, open-circuit voltage (Voc), and on/off current ratio are three crucial factors. DPP represents a key structural unit in an important class of red pigments with deep color, which were commercialized in the 1980s (Cui et al., 2022). It was shown that DPP-containing conjugated materials exhibit high charge transport mobility and excellent photovoltaic properties (Rabindranath et al., 2006; Tung et al., 2016; Balambiga et al., 2022). Charge transport in the semiconductor should not only occur in single molecules, but also across neighboring molecules. Thus, charge transport is divided into inter- and intracharge transport. For small molecules, due to short effective π-electron delocalization and good molecular packing, intercharge transport plays a key role in small molecule–based semiconductors (Hofkens et al., 1998; Guo et al., 2009; Yang et al., 2013).
π-conjugation extension and good planar structures are not only effective for intra-charge transport, but also benefit molecular packing and π-π overlap (Devibala et al., 2021; Feriancová et al., 2022). A new small molecule, based on π-expanded fused DPP, namely, FDPP, received our attention (Grzybowski et al., 2012). To the best of our knowledge, only a few articles have reported FDPP-based polymers and applied them in OFETs that present ambipolar properties with excellent ambipolar semiconducting properties under ambient conditions, reaching 2.23 and 1.08 cm2 V−1 s−1 for the n- and p-channels, respectively. However, semiconductor properties based on FDPP are completely unknown. In this work, FDPP is synthesized according to the literature and is applied in an OFET as the p-type semiconductor for the first time, presenting a hole mobility of approximately 1.3 × 10−3 cm2 V−1 s−1. In addition, the optical and electrochemical properties, as well as computation results, are investigated.
For the purpose of evaluating the optical properties, UV/vis spectroscopies of FDPP as well as of the DPP precursor are performed in dichloromethane solution and in the thin film state. The corresponding optical data are presented in Figure 1A. The alkylated DPP presents pink and purple colors in the solution and thin film state, respectively, while FDPP exhibits a pink (solution state) and blue color (thin film state). The absorption spectrum of the DPP precursor in dichloromethane exhibited a strong absorption maximum (λabs.max) at 532 nm, with a band peak at 505 nm. The extinction coefficient at 532 nm was 4.1×104 L mol−1 cm−1. Compared to the DPP precursor, the λabs.max of fused FDPP is shifted to 584 and 538 nm, which is red-shifted by 52 nm. The large red-shift is ascribed to fusing of the thiophene ring and the DPP core, resulting in improved planarity of the molecule backbone, as well as π-conjugation extension, which is advantageous for delocalized intramolecular π-orbitals and thus increased, efficient conjugation lengths (Dai et al., 2022). In the thin film state, both molecules presented a red-shift (70 nm for DPP and 66 nm for FDPP) in the optical absorption spectra compared to the solution state. The large red-shift indicates strong aggregation. In addition, in the long-wavelength absorption range, the bathochromic shift seems stronger for FDPP (around 150 nm). This observation indicates that FDPP presented a stronger π-π interaction as well as long-range ordered packing. This could be ascribed to the fact that FDPP presents a more planar structure core compared to DPP, which is advantageous for molecular packing as well as for π-π overlap. According to the onset of absorption, the optical band gap is calculated to be 1.55 eV for FDPP.
FIGURE 1. (A) UV/vis absorption spectra of DPP and FDPP in solution and thin film state; (B) cyclic voltammetry spectrum of FDPP; and (C) computational calculations of FDPP obtained at the B3LYP/6-31G* level.
The electrochemical properties of FDPP are investigated through cyclic voltammetry. As shown in Figure 1B, FDPP exhibited quasi-reversible reduction and oxidation curves. The reductive cycle showed two quasi-reversible cathodic waves at −0.63/−0.90 V and −1.37/−1.45 V. These quasi-reversible cathodic waves might have originated from the reduction of the DPP core from quinoid to a benzoid anion and dianion structures (Zhang et al., 2013). During the positive scans, a quasi-reversible redox wave was observed at 1.13/0.88 V and 1.55 + 1.63/1.36 V. The oxidative cycle exhibited three anodic waves at +1.13, +1.55, and +1.63 V, which are reverted at +1.36 and +0.88 V. Based on the onset of oxidation and reduction potentials, the HOMO/LUMO energy levels as well as the electrochemical band gap of the FDPP are estimated. As shown in Figure 1B, the onset of oxidation and reduction occurred for FDPP at 0.94 and −0.70 eV, respectively, based on which the HOMO and LUMO energy levels were calculated to be −5.74 eV and −4.10 eV, respectively. The HOMO energy level of FDPP is lower than the oxidation threshold of air, i.e., −5.27 eV, indicating the good stability of the material in air. According to the HOMO/LUMO energy levels, the electrochemical band gap is calculated to be 1.64 eV. The electrochemical band gap is slightly larger compared to the optical, which might be due to an interfacial barrier for charge injection (Zhu et al., 2009).
For the purpose of investigating the frontier molecular orbital features, the backbone configuration, and the HOMO/LUMO energy levels of FDPP, computational calculations were conducted using density-functional theory (DFT) at the B3LYP/6-31 (d,p) level of FDPP. As shown in Figure 1C, the core of FDPP is completely planar, which is in agreement with the optical results, while there is an approximately 10° angle between the thiophene and the DPP core (Naik et al., 2012). The electronic cloud distributions of the HOMO are mainly located at the DPP core, while the LUMO electronic cloud is dispersed over the whole of the FDPP. This observation indicates that if the FDPP is excited, electron transfer from the DPP core to the whole FDPP could take place, which means that FDPP has a weak intramolecular charge transfer (ICT) effect. The calculated HOMO/LUMO levels of the FDPP are −4.72 and −2.38 eV, resulting in a band gap of 2.34 eV. This value is larger than the electrochemical results, which is ascribed to the fact that the CV measurements were carried out on thin films of FDPP with strong intermolecular interactions, whereas the calculated results were based on a single FDPP molecule without intermolecular interactions.
The charge transport performance of FDPP was evaluated in bottom-gate, bottom-contact (BGBC) OFET devices on an n-type silicon wafer with a 300 nm layer of SiO2 as a dielectric material. After cleaning the substrate with piranha solution (H2SO4/H2O2, 3/1 v/v), deionized water, and acetone, it was immersed in OTS solution (5% in toluene) at room temperature overnight in an Ar-filled glove box. Subsequently, gold (30 nm) source (S) and drain (D) electrodes were deposited on the wafer through a shadow mask. The channel width was 1,000 µm and the distance between S and D electrode was 50 µm. A 5 mg/mL FDPP solution (in chloroform) was dropped on the surface of the substrate using a syringe (with PTFE filter, 0.45 μm) in an Ar-filled glove box. After 0.5 h, the temperature was increased to 45oC for 1 h on a hotplate. This mild heating is helpful for removing the solvents. The small molecule OFET device was prepared in an Ar-filled glovebox and characterized under vacuum condition. As shown in Figure 2A, FDPP exhibited p-type behavior, with an average hole mobility of 8.9 × 10−3 cm2 V−1 s−1 (highest μh of 9.7 × 10−3 cm2 V−1 s−1), while the non-fused precursor alkylated DPP device exhibited a hole mobility of only approximately 4 × 10−3 cm2 V−1 s−1 (Courtemanche et al., 2015). Compared to the alkylated DPP precursor, the hole transport mobility of the fused FDPP is enhanced more than double. This could be ascribed to the facts that 1), the planar core structure and the π-conjugation extension result in large efficient conjugation lengths in FDPP, which are advantageous for hole transport and 2), strong aggregation and good molecular packing are advantageous for hole transport between neighboring molecules. The current on/off ratio (Ion/Ioff) of the device is ∼105, while the threshold voltage is ∼32 V.
FIGURE 2. (A) Characteristics of FDPP OFET devices; (B) hole transport mobility obtained from 12 different devices; (C) hole mobilities (µh), threshold voltages (VTh), and on/off ratios (Ion/Ioff) of the FDPP-based FET device. The mobility is provided in average (highest)a form, with the performance based on 12 different FETs. Mobility was extracted by fitting the linear part of the plot of IDS1/2 versus VG using the equation IDS = Ciµ(VG-VTh)2 W/2L. a hole mobility.
In this work, a new π-conjugation extended fused DPP, namely, FDPP, was synthesized and characterized. In the thin film state, FDPP presented a large red-shift and strong absorption in the long wavelength range compared to the solution state, indicating that FDPP exhibited strong aggregation and good molecular packing in the solid state, which are advantageous for intercharge transport. The computation results showed that the FDPP core exhibited a completely planar structure. In addition, compared to the DPP core, the core of FDPP exhibited π-conjugation extension behavior, with a weak ICT effect. This observation is beneficial for charge transport within the FDPP molecules. As a consequence, the FDPP based semiconductor presents p-type behavior, with a hole mobility of up to 9.7 × 10−3 cm2 V−1 s−1 and an Ion/Ioff ratio of approximately 105. This study suggested the great potential of FDPP-type chromophores in constructing novel organic semiconductors.
The original contributions presented in the study are included in the article/supplementary material, further inquiries can be directed to the corresponding author.
JL: Data curation, Formal Analysis, Investigation, Writing–original draft. ZJ: Data curation, Formal Analysis, Methodology, Software, Writing–original draft. HZ: Funding acquisition, Project administration, Supervision, Writing–review and editing.
The author(s) declare financial support was received for the research, authorship, and/or publication of this article.
The authors acknowledge the support from Young Taishan Scholars under Grant No. 201909120.
The authors declare that the research was conducted in the absence of any commercial or financial relationships that could be construed as a potential conflict of interest.
All claims expressed in this article are solely those of the authors and do not necessarily represent those of their affiliated organizations, or those of the publisher, the editors and the reviewers. Any product that may be evaluated in this article, or claim that may be made by its manufacturer, is not guaranteed or endorsed by the publisher.
Ali, R., and Siddiqui, R. (2022). Dithieno[3,2-b:2′,3′-d]thiophene (DTT): An emerging heterocyclic building block for future organic electronic materials & functional supramolecular chemistry. RSC Adv. 12, 36073–36102. doi:10.1039/D2RA05768A
Balambiga, B., Devibala, P., Imran, P. M., Bhuvanesh, N. S. P., and Nagarajan, S. (2022). High mobility and ON/OFF ratio of solution-processable p -channel OFETs from arylacetylene end-capped alkoxyphenanthrenes. ChemPhysChem 23, e202200350. doi:10.1002/cphc.202200350
Chen, C.-K., Lin, Y.-C., Ho, J.-C., Yang, W.-C., and Chen, W.-C. (2022). Biomass-derived degradable poly(azomethine)s for flexible bistable photonic transistor memories. ACS Sustain. Chem. Eng. 10, 5268–5277. doi:10.1021/acssuschemeng.2c00396
Courtemanche, M.-A., Pulis, A. P., Rochette, É., Légaré, M.-A., Stephan, D. W., and Fontaine, F.-G. (2015). Intramolecular B/N frustrated Lewis pairs and the hydrogenation of carbon dioxide. Chem. Commun. 51, 9797–9800. doi:10.1039/C5CC03072B
Cui, S., Liang, D., Liu, M., Vivo, P., Zheng, M., Zhuang, T., et al. (2022). From transistors to phototransistors by tailoring the polymer stacking. Adv. Elect. Mater. 8, 2200019. doi:10.1002/aelm.202200019
Dai, Z., Zhang, D., and Zhang, H. (2022). Design, synthesis, and application in OFET of a quinoxaline-based D-A conjugated polymer. Front. Chem. 10, 934203. doi:10.3389/fchem.2022.934203
Devibala, P., Balambiga, B., Mohamed Imran, P., Bhuvanesh, N. S. P., and Nagarajan, S. (2021). Butterfly-like triarylamines with high hole mobility and on/off ratio in bottom-gated OFETs. Chem. Eur. J. 27, 15375–15381. doi:10.1002/chem.202102568
Feriancová, L., Cigáň, M., Kožíšek, J., Gmucová, K., Nádaždy, V., Dubaj, T., et al. (2022). Dithienylnaphthalenes and quaterthiophenes substituted with electron-withdrawing groups as n-type organic semiconductors for organic field-effect transistors. J. Mater. Chem. C 10, 10058–10074. doi:10.1039/D2TC01238C
Grzybowski, M., Glodkowska-Mrowka, E., Stoklosa, T., and Gryko, D. T. (2012). Bright, color-tunable fluorescent dyes based on π-expanded diketopyrrolopyrroles. Org. Lett. 14, 2670–2673. doi:10.1021/ol300674v
Guo, E. Q., Ren, P. H., Zhang, Y. L., Zhang, H. C., and Yang, W. J. (2009). Diphenylamine end-capped 1,4-diketo-3,6-diphenylpyrrolo[3,4-c]pyrrole (DPP) derivatives with large two-photon absorption cross-sections and strong two-photon excitation red fluorescence. Chem. Commun. 5859, 5859–5861. doi:10.1039/b911808j
Hofkens, J., Verheijen, W., Shukla, R., Dehaen, W., and De Schryver, F. C. (1998). Detection of a single dendrimer macromolecule with a fluorescent dihydropyrrolopyrroledione (DPP) core embedded in a thin polystyrene polymer film. Macromolecules 31, 4493–4497. doi:10.1021/ma980346i
Li, Z., Jeong, Y. J., Hong, J., Kwon, H., Ye, H., Wang, R., et al. (2022). Electrohydrodynamic-jet-printed phthalimide-derived conjugated polymers for organic field-effect transistors and logic gates. ACS Appl. Mater. Interfaces 14, 7073–7081. doi:10.1021/acsami.1c20278
Lu, W., Cao, J., Zhai, C., Bu, L., Lu, G., and Zhu, Y. (2022). Enhanced performance of organic field-effect transistors by a molecular dopant with high electron affinity. ACS Appl. Mater. Interfaces 14, 23709–23716. doi:10.1021/acsami.2c02977
Mooney, M., Nyayachavadi, A., Awada, A., Iakovidis, E., Wang, Y., Chen, M.-N., et al. (2023). Asymmetric side-chain engineering in semiconducting polymers: A platform for greener processing and post-functionalization of organic electronics. Polym. Chem. 14, 562–572. doi:10.1039/D2PY01244H
Naik, M. A., Venkatramaiah, N., Kanimozhi, C., and Patil, S. (2012). Influence of side-chain on structural order and photophysical properties in thiophene based diketopyrrolopyrroles: A systematic study. J. Phys. Chem. C 116, 26128–26137. doi:10.1021/jp306365q
Rabindranath, A. R., Zhu, Y., Heim, I., and Tieke, B. (2006). Red emitting N-functionalized poly(1,4-diketo-3,6-diphenylpyrrolo[3,4-c]pyrrole) (Poly-DPP): A deeply colored polymer with unusually large Stokes shift. Macromolecules 39, 8250–8256. doi:10.1021/ma061024e
Shaker, M., Park, B., Lee, S., and Lee, K. (2020). Face-on oriented thermolabile Boc-isoindigo/thiophenes small molecules: From synthesis to OFET performance. Dyes Pigments 172, 107784. doi:10.1016/j.dyepig.2019.107784
Shibuya, Y., Matsunaga, A., Kumaki, D., Tokito, S., and Katagiri, H. (2022). Azulene end-capped 1,3,4-thiadiazole as an n-type organic semiconductor with a herringbone–brickwork cooperative 2D layered structure. Cryst. Growth & Des. 22, 6554–6563. doi:10.1021/acs.cgd.2c00783
Sonalin, S., Mishra, A., Sahu, A. K., Mishra, A. K., Imran, P. M., Bhuvanesh, N. S. P., et al. (2020). Aggregation behavior and high charge-carrier OFET-mobility of functionalized phenanthro[9,10-d]imidazoles. J. Phys. Chem. C 124, 13053–13062. doi:10.1021/acs.jpcc.0c03924
Surya, S. G., Raval, H. N., Ahmad, R., Sonar, P., Salama, K. N., and Rao, V. R. (2019). Organic field effect transistors (OFETs) in environmental sensing and health monitoring: A review. TrAC Trends Anal. Chem. 111, 27–36. doi:10.1016/j.trac.2018.11.027
Tao, J., Sun, W., and Lu, L. (2022). Organic small molecule semiconductor materials for OFET-based biosensors. Biosens. Bioelectron. 216, 114667. doi:10.1016/j.bios.2022.114667
Tung, W.-Y., Li, M.-H., Wu, H.-C., Liu, H.-Y., Hsieh, Y.-T., and Chen, W.-C. (2016). High performance nonvolatile transistor memories utilizing functional polyimide-based supramolecular electrets. Chem. Asian J. 11, 1631–1640. doi:10.1002/asia.201600365
Yang, C., Zheng, M., Li, Y., Zhang, B., Li, J., Bu, L., et al. (2013). N-Monoalkylated 1,4-diketo-3,6-diphenylpyrrolo[3,4-c]pyrroles as effective one- and two-photon fluorescence chemosensors for fluoride anions. J. Mater. Chem. A 1, 5172. doi:10.1039/c3ta00160a
Yuvaraja, S., Nawaz, A., Liu, Q., Dubal, D., Surya, S. G., Salama, K. N., et al. (2020). Organic field-effect transistor-based flexible sensors. Chem. Soc. Rev. 49, 3423–3460. doi:10.1039/C9CS00811J
Zhang, H., Li, R., Deng, Z., Cui, S., Wang, Y., Zheng, M., et al. (2020a). π-Conjugated oligomers based on aminobenzodifuranone and diketopyrrolopyrrole. Dyes Pigments 181, 108552. doi:10.1016/j.dyepig.2020.108552
Zhang, H., Neudörfl, J.-M., and Tieke, B. (2013). Naphthodifuranone-based monomers and polymers. Macromolecules 46, 5842–5849. doi:10.1021/ma401278s
Zhang, H., Tung, W.-Y., Li, X., Jin, H., Deng, R., Chen, Y.-M., et al. (2020b). Conjugated polymer with dynamic and thermoreversible hydrogen bonding on the backbone. Polymer 203, 122787. doi:10.1016/j.polymer.2020.122787
Zhang, Y., Zhang, W., Wang, L., and Yu, G. (2022). Synthetic strategies, molecular engineering and applications of semiconducting polymers based on diarylethylene units in electronic devices. J. Mater. Chem. C 10, 18091–18119. doi:10.1039/D2TC04383A
Zhu, Y., Zhang, K., and Tieke, B. (2009). Electrochemical polymerization of bis(3,4-ethylenedioxythiophene)-substituted 1,4-Diketo-3,6-diphenyl-pyrrolo[3,4-c]pyrrole (DPP) derivative. Macromol. Chem. Phys. 210, 431–439. doi:10.1002/macp.200800507
Keywords: organic field effect transistor (OFET), p-type, charge transport mobility, semiconductor, conjugated materials, DPP
Citation: Li J, Ji Z, He A and Zhang H (2023) Design, synthesis, and application in OFET of a small molecule based on π-expanded fused diketopyrrolopyrrole. Front. Chem. 11:1280816. doi: 10.3389/fchem.2023.1280816
Received: 21 August 2023; Accepted: 14 September 2023;
Published: 09 October 2023.
Edited by:
Yue Liu, Liaoning Technical University, ChinaReviewed by:
Yalong Wang, Hainan University, ChinaCopyright © 2023 Li, Ji, He and Zhang. This is an open-access article distributed under the terms of the Creative Commons Attribution License (CC BY). The use, distribution or reproduction in other forums is permitted, provided the original author(s) and the copyright owner(s) are credited and that the original publication in this journal is cited, in accordance with accepted academic practice. No use, distribution or reproduction is permitted which does not comply with these terms.
*Correspondence: Haichang Zhang, aGFpY2hhbmd6aGFuZ0Bob3RtYWlsLmNvbQ==
Disclaimer: All claims expressed in this article are solely those of the authors and do not necessarily represent those of their affiliated organizations, or those of the publisher, the editors and the reviewers. Any product that may be evaluated in this article or claim that may be made by its manufacturer is not guaranteed or endorsed by the publisher.
Research integrity at Frontiers
Learn more about the work of our research integrity team to safeguard the quality of each article we publish.