- 1Department of Pharmacy, Ganzhou People’s Hospital, The Affiliated Ganzhou Hospital of Nanchang University, Ganzhou, China
- 2Department of Pharmacy, People’s Hospital of Guilin, Guilin, China
Sulfonylaniline motif plays an important role in pharmaceutical sciences. Developed methods towards this structure are typically lack of good modifiability and stability. In this study, visible-light-mediated sulfonylation of aniline using sulfonyl fluoride as a modifiable and stable sulfonylation reagent is described. A variety of substituted sulfonylanilines were synthesized under mild reaction conditions with moderate to good efficiency. The example of late-stage sulfonylation highlighted the advantage of using sulfonyl fluoride as a sulfonylation reagent. In addition, the crucial influence of counterions on the photocatalyst observed in this system would inspire further research on the photochemistry of sulfonyl fluoride.
1 Introduction
Both sulfonyl and amine groups are typically considered privileged skeletons in medicinal chemistry for the discovery of biologically active compounds since more than 60% of bioactive molecules discovered in the past 40 years include amine units, while the percentage of the sulfonyl group is 3.1 (Ertl et al., 2020). The sulfonylaniline motif which combines these two groups also widely exists in diverse drugs, such as the Bcl-2 protein inhibitor navitoclax (Souers et al., 2013), DP receptor antagonist laropiprant (Sturino et al., 2007), histamine H1-receptor blocker oxomemazine (Lee et al., 1994), and hepatitis B virus core protein inhibitor vebicorvir (Sulkowski et al., 2022) (Figure 1A). Therefore, the development of versatile, efficient, and atom economic routes toward diverse sulfonylated anilines is highly important for both organic synthesis and pharmaceutic sciences.
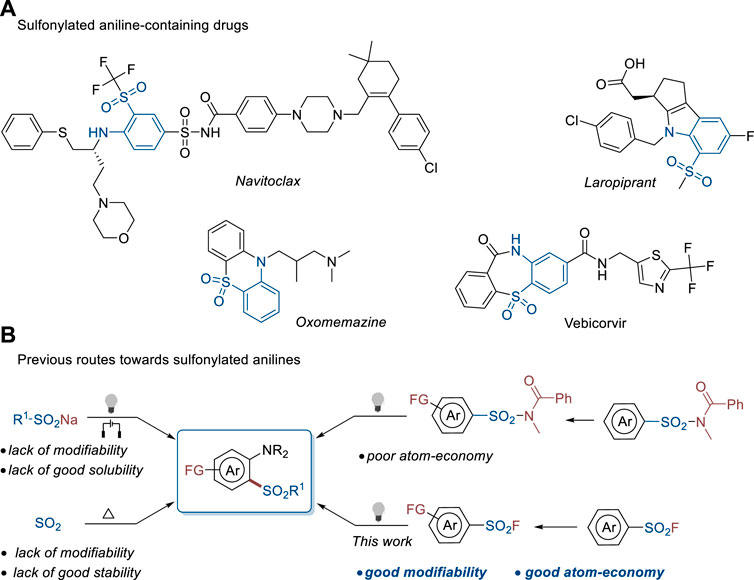
FIGURE 1. Sulfonylaniline-containing drugs and their construction methods. (A) Sulfonylated aniline-containing drugs. (B) Previous routes towards sulfonylated anilines.
Recently, several research groups have achieved outstanding results on the sulfonylation of anilines (Figure 1B). Johnson et al. (2018) reported the reaction of anilines and sulfinate salts mediated by visible light in the presence of an external oxidant. Wu et al. (2019), Lu et al. (2019), Nikl et al. (2019) reported the electrochemical synthesis of 2-sulfonylanilines with sulfinate salts and anilines. Sulfur dioxide has been widely used as a sulfonylation reagent in recent years (Emmett et al., 2015; Qiu et al., 2018; Ye et al., 2019; Zhang et al., 2023). In addition, a three-component reaction of anilines, DABCO(SO2)2, and aryldiazonium tetrafluoroborates was developed by the Wu group to construct sulfonylated anilines in high efficiency under metal-free conditions (Zhou et al., 2018). Several research groups demonstrated that sulfonamides could be used as sulfonylation reagents (Luo et al., 2021; Du et al., 2022; Zhen et al., 2022) to perform sulfonylation of anilines (Xu et al., 2022) and generated diverse complex desired products. Despite these achievements, there are obvious shortages in these methodologies. Using sulfinate salts as substrates usually requires an external oxidant. In addition, sulfinate salts have poor solubility in organic solvents, which hinders the modification of the substrates to construct complex products. The aryldiazonium salts used in the capture of SO2 have poor stability and also lack modifiability. Although sulfonamides are stable and can be modified to synthesize complex products, the released amide group underlines the poor atom economy of this method. Therefore, the exploration of novel strategies and sulfonylation reagents to construct sulfonylated anilines is of high demand in both synthetic chemistry and drug discovery.
Sulfonyl fluoride, which is readily available in the form of aryl halide (Davies et al., 2017), sulfonyl chloride (Dong et al., 2014), sulfonic acid (Jang et al., 2010), sulfinate salt (Banks et al., 1996), and thiophenol (Wright et al., 2005), is usually used in chemical probes (Jones et al., 2018; Mortenson et al., 2018; Martin-Gago et al., 2019) and polymer materials (Gao et al., 2017; Durie et al., 2018). However, it has rarely been used as a sulfonylation reagent for functionalization of the C–H bond. The successful examples required harsh reaction conditions to proceed with Friedel–Crafts substitution of arenes in the presence of AlCl3 (Hyatt et al., 1984). Because of the inertness of the SO2–F bond (Chinthakindi et al., 2018), sulfonyl fluoride can survive under diverse functionalization reaction conditions, including Suzuki–Miyaura cross-coupling (Chinthakindi et al., 2016), Heck (Qin et al., 2016), Stille (Hett et al., 2015), and Sonogashira reactions (Fadeyi et al., 2016). Therefore, we explored whether sulfonyl fluoride could be used as a stable, modifiable, and atom economic sulfonylation reagent to react with aniline under mild reaction conditions. Herein, we report a simple and mild protocol to construct sulfonylated aniline by a visible-light-mediated reaction of sulfonyl fluoride and aniline via a radical process.
2 Results and discussion
4-Methylbenzenesulfonyl fluoride 1a and N,N,4-trimethylaniline 2a were used to optimize the reaction conditions. No or only trace amounts of the product were observed using methylene blue or 4-CzIPN as the photocatalyst in MeCN under the irradiation of blue light (Table 1, entries 1 and 2). Ru(ppy)3 provided the desired product 3a in 10% yield (entry 3). Ir[(dFCF3ppy)2(dtbbpy)]PF6, Ir[(dFCF3ppy)2(bpy)]PF6, and Ir [(ppy)2(dtbbpy)]PF6 could not afford better results (entries 4–6). Ir[(ppy)2(dtbbpy)]PF6 showed slightly higher efficiency, furnished 3a in a 21% yield. Interestingly, we found that the counterion of the photocatalyst had a crucial influence on the efficiency. Ir[(ppy)2(dtbbpy)]Cl, which was rarely used in the organic synthesis, dramatically increased the yield of 3a to 46%. Other organic solvents, such as THF, DMSO, DCE, and CH3OH, exhibited a much inferior efficiency than MeCN. In an attempt to develop an environment-benign strategy, water with a combination of diverse surfactants was used as the solvent. However, only a 32% yield was obtained as the best result in the presence of Tween 40 (entries 13–17). Finally, various organic and inorganic bases were also screened. We found that the weak base KF provided a 68% yield of 3a (entry 20). In addition, NaHCO3 gave the best result with an 82% yield (entry 23). Only low yields (0%–25%) were obtained using stronger bases, including K3PO4, CsF, DABCO, and Na2CO3, probably because of the hydrolysis of sulfonyl fluorides (Chinthakindi et al., 2016).
With the optimal reaction conditions in hand, the scope of anilines was explored in the next step (Figure 2). Anilines with diverse alkyl substituents on the para position could afford corresponding products in good yields (3a–3e). A strong electron-donating methoxyl group-attached product 3f could be synthesized in a 55% yield. Unprotected hydroxyl group-containing aniline was also used as a suitable substrate, giving the product 3g in a 41% yield. The cyclopropane unit remained intact under the standard reaction conditions as 3h was furnished in a 43% yield. Triphenylamine could be mono-sulfonylated on the para position in this reaction system to provide 3i in a 70% yield, probably because the electron-deficient sulfonyl group hindered the di-sulfonylation process. The protecting group of the nitrogen atom was also varied. In addition, diethyl group-attached aniline was sulfonylated to result in 3j in a 57% yield. Both para and ortho sulfonylation (3k and 3l) were observed when unsubstituted aniline was used. Sulfonylation of diamine only resulted in a 35% yield of 3m, probably because the diamine was too reactive. In addition, electron-withdrawing group-attached aniline could not be employed in this system, probably because of the lower reactivity.
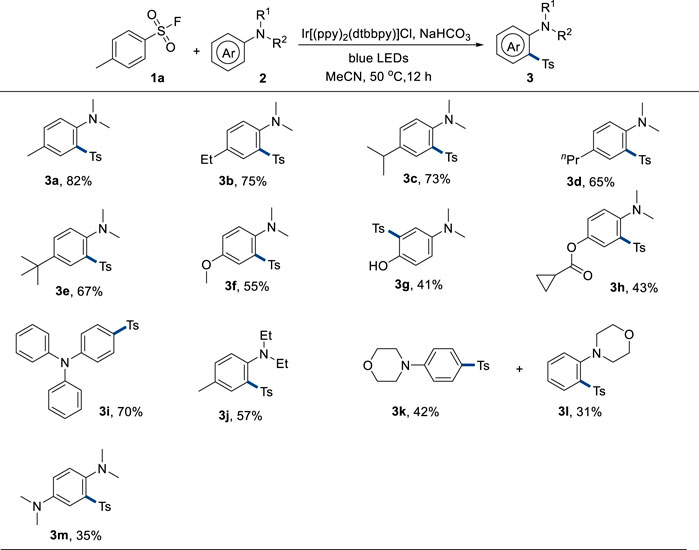
FIGURE 2. Scope of anilines. Reaction conditions: 1a (0.36 mmol, 1.8 equivalent), 2 (0.2 mmol, 1.0 equivalent), Ir[(ppy)2(dtbbpy)]Cl (5 mol%), NaHCO3 (0.36 mmol, 1.8 equivalent), solvent (2.0 mL), 50°C, 30-W blue LEDs, and 12 h.
Subsequently, the scope of sulfonyl fluorides was tested (Figure 3). Para alkyl-substituted substrates provided moderate to good yields of the corresponding products (3m–3p). Bromomethyl and aryl bromide groups survived under the reaction conditions (3q and 3u), which could proceed to late-stage functionalization to construct diverse and complex sulfones. Electron-donating and bicyclic sulfonyl fluorides could also generate the corresponding products in moderate to good yields (3r–3t and 3v). However, alkyl sulfonyl fluoride could not afford the desired product.
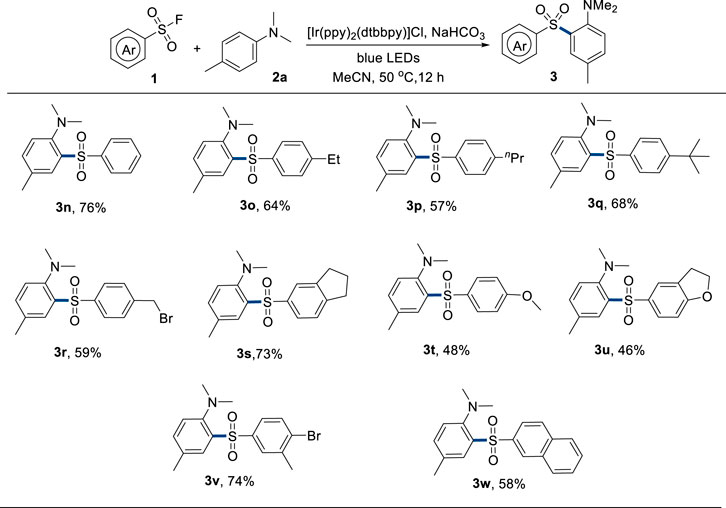
FIGURE 3. Scope of sulfonyl fluorides. Reaction conditions: 1 (0.36 mmol, 1.8 equivalent), 2a (0.2 mmol, 1.0 equivalent), Ir[(ppy)2(dtbbpy)]Cl (5 mol%), NaHCO3 (0.36 mmol, 1.8 equiv), solvent (2.0 mL), 50°C, 30-W blue LEDs, and 12 h.
In order to outline the advantage of our protocol, a route for late-stage sulfonylation of aniline was developed (Figure 4). First, a Suzuki–Miyaura cross-coupling reaction (Chinthakindi et al., 2016) was performed with 4-bromobenzenesulfonyl fluoride and phenylboronic acid to generate functionalized sulfonyl fluoride 4. Then, the crude product obtained by a simple work-up was used under the standard reaction conditions. A 57% yield of functionalized product 5 was synthesized. This success highlighted that sulfonyl fluorides could be used as modifiable, stable, and atom economic sulfonyl reagents to synthesize diverse complex sulfones.
In an attempt to gain a deep insight into the reaction mechanism, a control experiment was performed to explore the effect of the counterion (Figure 5). To investigate whether sulfonyl chloride generated from the reaction of sulfonyl fluoride and chloride anions in Ir[(ppy)2(dtbbpy)]Cl was the authentic substrate, NaCl or Bu4NCl with a combination of Ir[(ppy)2(dtbbpy)]PF6 was employed in the model reaction. However, only a trace amount of the product was detected, implying that sulfonyl fluoride was the authentic substrate. In addition, the reference also implies that harsh reaction conditions are required in the generation of sulfonyl chloride from sulfonyl fluoride (Norris et al., 1978). Based on this result and previous explorations in references (Johnson et al., 2018; Zhou et al., 2018; Lu et al., 2019; Nikl et al., 2019; Wu et al., 2019; Xu et al., 2022), a proposed mechanism is depicted in Figure 5. Aniline 2a was oxidized by the photoexcited catalyst Ir[(ppy)2(dtbbpy)]Cl (Ir(III)*), generating radical cation A (Brown et al., 2015) and Ir(II) complex. This reduced iridium species possessed high reduction potential and could reduce sulfonyl fluoride to result in fluoride anion and sulfonyl radical C via single electron transfer (SET). After that, the radical coupling of C and the intermediate B generated from the tautomerization of A followed to generate the desired product 3a.
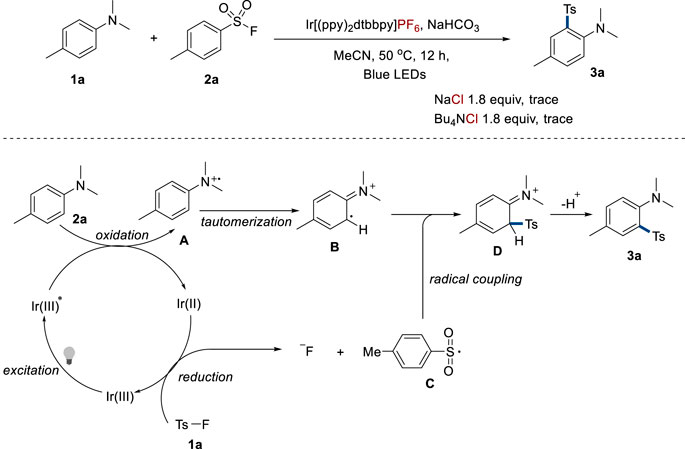
FIGURE 5. Proposed mechanism. (A) Radical cation intermediate. (B) Tautomerized intermediate. (C) Sulfonyl radical. (D) Radical coupling product.
3 Conclusion
In summary, we have developed a useful and simple strategy to synthesize sulfonylated anilines via visible-light-mediated reactions of sulfonyl fluorides and anilines. It has been demonstrated that sulfonyl fluorides could be used as modifiable and stable sulfonylation reagents to carry out late-stage functionalization and synthesize complex and diverse sulfones. The reaction conditions are simple and mild. In addition, the mechanism research reveals that the counterion of the photocatalyst has a crucial effect on the efficiency, which would inspire further exploration in this field.
Data availability statement
The original contributions presented in the study are included in the article/Supplementary Material; further inquiries can be directed to the corresponding author.
Author contributions
X-QL: conceptualization, funding acquisition, project administration, supervision, and writing—original draft. Q-QL: data curation, methodology, and writing—review and editing. JL: data curation, methodology, and writing—review and editing. Y-YL: data curation, methodology, and writing—review and editing.
Funding
The author(s) declare financial support was received for the research, authorship, and/or publication of this article. The authors are grateful for the financial support by the Science and Technology Program of Ganzhou (2022-YB1422).
Conflict of interest
The authors declare that the research was conducted in the absence of any commercial or financial relationships that could be construed as a potential conflict of interest.
Publisher’s note
All claims expressed in this article are solely those of the authors and do not necessarily represent those of their affiliated organizations, or those of the publisher, the editors, and the reviewers. Any product that may be evaluated in this article, or claim that may be made by its manufacturer, is not guaranteed or endorsed by the publisher.
Supplementary material
The Supplementary Material for this article can be found online at: https://www.frontiersin.org/articles/10.3389/fchem.2023.1267223/full#supplementary-material
References
Banks, R. E., Besheesh, M. K., Mohialdin-Khaffaf, S. N., and Sharif, I. (1996). N-halogeno compounds. Part 18. l-Alky1-4-fluoro-l,4-diazoniabicyclo[2.2.2]octane salts: User-friendly site-selective electrophilic fluorinating agents of the N-fluoroammonium class. J. Chem. Soc. Perkin Trans. 1, 2069–2076. doi:10.1039/P19960002069
Brown, T. A., Chen, H., and Zare, R. N. (2015). Detection of the short-lived radical cation intermediate in the electrooxidation of N,N-dimethylaniline by mass spectrometry. Angew. Chem. Int. Ed. 54, 11183–11185. doi:10.1002/anie.201506316
Chinthakindi, P. K., and Arvidsson, P. I. (2018). Sulfonyl fluorides (SFs): More than click reagents? Eur. J. Org. Chem. 2018, 3648–3666. doi:10.1002/ejoc.201800464
Chinthakindi, P. K., Kruger, H. G., Govender, T., Naicker, T., and Arvidsson, P. I. (2016). On-water synthesis of biaryl sulfonyl fluorides. J. Org. Chem. 81, 2618–2623. doi:10.1021/acs.joc.5b02770
Davies, A. T., Curto, J. M., Bagley, S. W., and Willis, M. C. (2017). One-pot palladium-catalyzed synthesis of sulfonyl fluorides from aryl bromides. Chem. Sci. 8, 1233–1237. doi:10.1039/c6sc03924c
Dong, J., Krasnova, L., Finn, M. G., and Sharpless, K. B. (2014). Sulfur(VI) fluoride exchange (SuFEx): Another good reaction for click chemistry. Angew. Chem. Int. Ed. 53, 9430–9448. doi:10.1002/anie.201309399
Du, X., Zhen, J. S., Xu, X. H., Yuan, H., Li, Y. H., Zheng, Y., et al. (2022). Hydrosulfonylation of alkenes with sulfonyl imines via Ir/Cu dual photoredox catalysis. Org. Lett. 24, 3944–3949. doi:10.1021/acs.orglett.2c01260
Durie, K., Yatvin, J., Kovaliov, M., Crane, G. H., Horn, J., Averick, S., et al. (2018). SuFEx postpolymerization modification kinetics and reactivity in polymer brushes. Macromolecules 51, 297–305. doi:10.1021/acs.macromol.7b02372
Emmett, Edward J., and Willis, Michael C. (2015). The development and application of sulfur dioxide surrogates in synthetic organic chemistry. Asian J. Org. Chem. 4, 602–611. doi:10.1002/ajoc.201500103
Ertl, P., Altmann, E., and McKenna, J. M. (2020). The most common functional groups in bioactive molecules and how their popularity has evolved over time. J. Med. Chem. 63, 8408–8418. doi:10.1021/acs.jmedchem.0c00754
Fadeyi, O., Parikh, M. D., Chen, M. Z., Kyne, R. E., Taylor, A. P., O'Doherty, I., et al. (2016). Chemoselective preparation of clickable aryl sulfonyl fluoride monomers: A toolbox of highly functionalized intermediates for chemical biology probe synthesis. Chembiochem 17, 1925–1930. doi:10.1002/cbic.201600427
Gao, B., Zhang, L., Zheng, Q., Zhou, F., Klivansky, L. M., Lu, J., et al. (2017). Bifluoride-catalysed sulfur(VI) fluoride exchange reaction for the synthesis of polysulfates and polysulfonates. Nat. Chem. 9, 1083–1088. doi:10.1038/nchem.2796
Hett, E. C., Xu, H., Geoghegan, K. F., Gopalsamy, A., Kyne, R. E., Menard, C. A., et al. (2015). Rational targeting of active-site tyrosine residues using sulfonyl fluoride probes. ACS Chem. Biol. 10, 1094–1098. doi:10.1021/cb5009475
Hyatt, J. A., and White, A. W. (1984). Synthesis of aryl alkyl and aryl vinyl sulfones via friedel-crafts reactions of sulfonyl fluorides. Synthesis 1984, 214–217. doi:10.1055/s-1984-30774
Jang, D., and Kim, J.-G. (2010). A convenient, one-pot procedure for the preparation of acyl and sulfonyl fluorides using Cl3CCN, Ph3P, and TBAF(t-BuOH)4. Synlett 2010, 3049–3052. doi:10.1055/s-0030-1259051
Johnson, T. C., Elbert, B. L., Farley, A. J. M., Gorman, T. W., Genicot, C., Lallemand, B., et al. (2018). Direct sulfonylation of anilines mediated by visible light. Chem. Sci. 9, 629–633. doi:10.1039/c7sc03891g
Jones, L. H. (2018). Emerging utility of fluorosulfate chemical probes. ACS Med. Chem. Lett. 9, 584–586. doi:10.1021/acsmedchemlett.8b00276
Lee, S. W., Woo, C. W., and Kim, J. G. (1994). Selectivity of oxomemazine for the M1 muscarinic receptors. Arch. Pharm. Res. 17, 443–451. doi:10.1007/bf02979123
Lu, F., Li, J., Wang, T., Li, Z., Jiang, M., Hu, X., et al. (2019). Electrochemical oxidative C−H sulfonylation of anilines. Asian J. Org. Chem. 8, 1838–1841. doi:10.1002/ajoc.201900447
Luo, Y., Ding, H., Zhen, J. S., Du, X., Xu, X. H., Yuan, H., et al. (2021). Catalyst-free arylation of sulfonamides via visible light-mediated deamination. Chem. Sci. 12, 9556–9560. doi:10.1039/d1sc02266k
Martin-Gago, P., and Olsen, C. A. (2019). Arylfluorosulfate-based electrophiles for covalent protein labeling: A new addition to the arsenal. Angew. Chem. Int. Ed. 58, 957–966. doi:10.1002/anie.201806037
Mortenson, D. E., Brighty, G. J., Plate, L., Bare, G., Chen, W., Li, S., et al. (2018). Inverse drug discovery" strategy to identify proteins that are targeted by latent electrophiles as exemplified by aryl fluorosulfates. J. Am. Chem. Soc. 140, 200–210. doi:10.1021/jacs.7b08366
Nikl, J., Ravelli, D., Schollmeyer, D., and Waldvogel, S. R. (2019). Straightforward electrochemical sulfonylation of arenes and aniline derivatives using sodium sulfinates. ChemElectroChem 6, 4450–4455. doi:10.1002/celc.201901212
Norris, T. (1978). The reaction of arenesulphonyl fluorides with anhydrous aluminium chloride. J. Chem. Soc. Perkin Trans. 1, 1378–1380. doi:10.1039/P19780001378
Qin, H. L., Zheng, Q., Bare, G. A., Wu, P., and Sharpless, K. B. (2016). A heck-matsuda process for the synthesis of beta-arylethenesulfonyl fluorides: Selectively addressable bis-electrophiles for SuFEx click chemistry. Angew. Chem. Int. Ed. 55, 14155–14158. doi:10.1002/anie.201608807
Qiu, G., Zhou, K., and Wu, J. (2018). Recent advances in the sulfonylation of C-H bonds with the insertion of sulfur dioxide. Chem. Commun. 54, 12561–12569. doi:10.1039/c8cc07434h
Souers, A. J., Leverson, J. D., Boghaert, E. R., Ackler, S. L., Catron, N. D., Chen, J., et al. (2013). ABT-199, a potent and selective BCL-2 inhibitor, achieves antitumor activity while sparing platelets. Nat. Med. 19, 202–208. doi:10.1038/nm.3048
Sturino, C. F., O’Neill, G., Lachance, N., Boyd, M., Berthelette, C., Labelle, M., et al. (2007). Discovery of a potent and selective prostaglandin D2 receptor antagonist, [(3R)-4-(4-Chlorobenzyl)-7-fluoro-5-(methylsulfonyl)-1,2,3,4-tetrahydrocyclopenta[b]indol-3-yl]-acetic acid (MK-0524). J. Med. Chem. 50, 794–806. doi:10.1021/jm0603668
Sulkowski, M. S., Agarwal, K., Ma, X., Nguyen, T. T., Schiff, E. R., Hann, H. L., et al. (2022). Safety and efficacy of vebicorvir administered with entecavir in treatment-naive patients with chronic hepatitis B virus infection. J. Hepatol. 77, 1265–1275. doi:10.1016/j.jhep.2022.05.027
Wright, S. W., and Hallstrom, K. N. A. (2005). A convenient preparation of heteroaryl sulfonamides and sulfonyl fluorides from heteroaryl thiols. J. Org. Chem. 71, 1080–1084. doi:10.1021/jo052164+
Wu, Y. C., Jiang, S. S., Luo, S. Z., Song, R. J., and Li, J. H. (2019). Transition-metal- and oxidant-free directed anodic C-H sulfonylation of N,N-disubstituted anilines with sulfinates. Chem. Commun. 55, 8995–8998. doi:10.1039/c9cc03789f
Xu, X. H., Zhen, J. S., Du, X., Yuan, H., Li, Y. H., Chu, M. H., et al. (2022). Visible-light-mediated late-stage sulfonylation of anilines with sulfonamides. Org. Lett. 24, 853–858. doi:10.1021/acs.orglett.1c04144
Ye, S., Li, X., Xie, W., and Wu, J. (2019). Photoinduced sulfonylation reactions through the insertion of sulfur dioxide. Eur. J. Org. Chem. 2020, 1274–1287. doi:10.1002/ejoc.201900396
Zhang, J., Wang, P., Li, Y., and Wu, J. (2023). Asymmetric sulfonylation with sulfur dioxide surrogates: A new access to enantiomerically enriched sulfones. Chem. Commun. 59, 3821–3826. doi:10.1039/d2cc06339e
Zhen, J., Du, X., Xu, X., Li, Y., Yuan, H., Xu, D., et al. (2022). Visible-light-mediated late-stage sulfonylation of boronic acids via N–S bond activation of sulfonamides. ACS Catal. 12, 1986–1991. doi:10.1021/acscatal.1c05669
Keywords: sulfonylation, photoredox, radical, sulfonyl fluoride, aniline
Citation: Li X-Q, Liao Q-Q, Lai J and Liao Y-Y (2023) Visible-light-mediated sulfonylation of anilines with sulfonyl fluorides. Front. Chem. 11:1267223. doi: 10.3389/fchem.2023.1267223
Received: 26 July 2023; Accepted: 11 August 2023;
Published: 25 August 2023.
Edited by:
Gu Zhan, Chengdu University of Traditional Chinese Medicine, ChinaReviewed by:
Kenkera Rayappa Naveen, Julius Maximilian University of Würzburg, GermanyTao Cao, Nanjing University of Chinese Medicine, China
Qing-Zhu Li, Chengdu University, China
Copyright © 2023 Li, Liao, Lai and Liao. This is an open-access article distributed under the terms of the Creative Commons Attribution License (CC BY). The use, distribution or reproduction in other forums is permitted, provided the original author(s) and the copyright owner(s) are credited and that the original publication in this journal is cited, in accordance with accepted academic practice. No use, distribution or reproduction is permitted which does not comply with these terms.
*Correspondence: Xin-Qing Li, lixinqing16@163.com