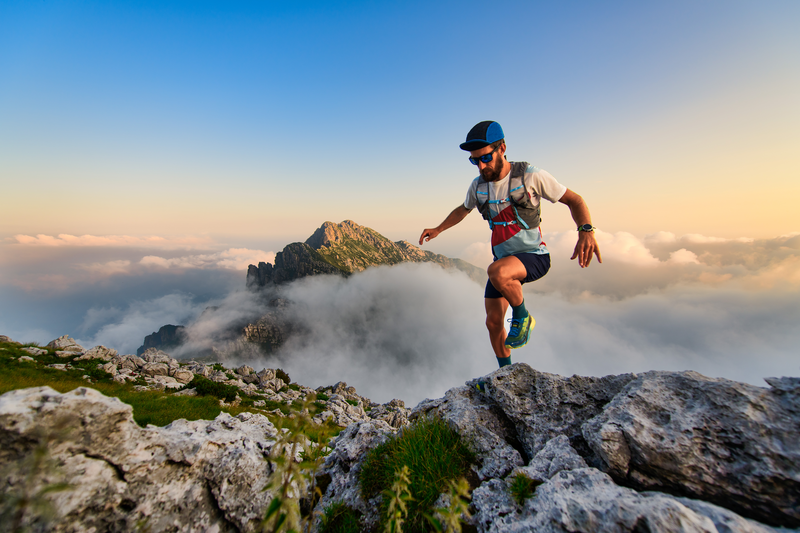
95% of researchers rate our articles as excellent or good
Learn more about the work of our research integrity team to safeguard the quality of each article we publish.
Find out more
ORIGINAL RESEARCH article
Front. Chem. , 06 September 2023
Sec. Catalytic Reactions and Chemistry
Volume 11 - 2023 | https://doi.org/10.3389/fchem.2023.1235415
The preparation of yolk-shell structured magnetic mesoporous composites is a significant subject between researchers. Especially, modification of theses composites with ionic liquid/metal complex is very important for catalytic processes. In the present study, a novel magnetic methylene-based periodic mesoporous organosilica (PMO)-supported ionic liquid/Cu complex with yolk-shell structure (YS-Fe3O4@PMO/IL-Cu) was prepared via the soft template-assisted method. The TGA, FT-IR, SEM, EDX, XRD, VSM, nitrogen-sorption, and ICP techniques were employed to identify YS-Fe3O4@PMO/IL-Cu. The YS-Fe3O4@PMO/IL-Cu material was applied as a powerful nanocatalyst for the synthesis of pyranopyrazoles under ultrasonic media. The study demonstrated that the YS-Fe3O4@PMO/IL-Cu nanocatalyst is highly recyclable, selective, and effective. The leaching test was performed to investigate the nature of the designed catalyst under the applied conditions.
Yolk-shell structured nanoparticles (NPs) are hybrid materials in which a core is encapsulated in a hollow shell and can move freely within this shell, commonly demonstrated as core/void/shell. In this structure, the core is not blocked and thus provides an effective active site for the chemical processes (Kim et al., 2002; Kamata et al., 2003; Yin et al., 2004; Gao et al., 2007; Liu et al., 2013; Purbia and Paria, 2016; Xie et al., 2017). The unique properties of yolk-shell structured materials, such as low density, high surface area, permeable shells, high thermal stability, and interstitial hollow spaces, make them powerful platforms for biotechnology/biomedicine, controlled release, magnetic resonance imaging, data storage, catalysis, environmental remediation, etc. (Kresge et al., 1992; Vartuli et al., 1994; Holmes et al., 1998; Vartuli et al., 1998; Tsuji et al., 1999; Morishita et al., 2006; Puanngam and Unob, 2008; Du and He, 2011; Zhao et al., 2011; Ghaedi et al., 2013; Zhang, 2013; Nasab and Kiasat, 2016; Purbia and Paria, 2016). Among the various categories of yolk-shells (YSs), magnetic composites with Fe3O4 cores and nano-silica shells are very attractive due to their advantages such as good magnetic properties, high chemical and thermal stability, non-toxicity, high adsorption capacity, high surface area, high biocompatibility, and high accessibility of–OH groups on their surface for any modification (Arruebo et al., 2006; Liu et al., 2011; Yang et al., 2015). Recently, the catalytic application of YS-structured magnetic mesoporous silica nanocomposites has received much attention. Some of the newly developed systems in this regard are Au@Void@PMO (Yang et al., 2015), Fe3O4@SiO2@Pd/HSPMO (Dai et al., 2017), PMO-MHS (Zhang et al., 2008), and Fe3O4@void@mSiO2 (Qiu et al., 2015).
Periodic mesoporous organosilica (PMOs), a desirable class of organic-inorganic composite materials with great properties such as high surface area, high lipophilicity, and high thermal and mechanical stability, have emerged as an ideal support (Wang et al., 2015; Yu et al., 2019; Norouzi et al., 2020; Neysi and Elhamifar, 2023). In particular, bifunctional PMOs (BPMOs), which contain organic functionalities on both the mesoporous walls and channels, are highly attractive for catalytic processes
On the other hand, ionic liquids (ILs) have attracted tremendous attention in chemistry and materials science in the last decade owing to their unique characteristics, such as low vapor pressure, high chemical and thermal stability, and their capability to dissolve a variety of compounds. In particular, recently, imidazolium-based ILs have been widely used as linkers for the effective immobilization of catalytic active sites on solid supports (Neysi et al., 2020; Jangra et al., 2021; Veysipour et al., 2021; Neysi and Elhamifar, 2022).
The preparation of pyranopyrazole derivatives has emerged as a powerful tool in organic synthesis because they are an important class of biologically active compounds. Some biological properties of pyranopyrazoles are anticancer, antifungal, anti-anxiety, antiviral, and anti-AIDS (Babaie and Sheibani, 2011; Moosavi-Zare et al., 2013; Zolfigol et al., 2013; Ali et al., 2014; Gujar et al., 2014; Pandit et al., 2015). To date, many homogeneous and heterogeneous catalysts have been reported for the synthesis of pyranopyrazoles under different conditions. However, some of these systems suffer from the problems of high catalyst loading, harsh conditions, and the use of toxic organic solvents. Therefore, design a green and efficient catalytic system to overcome the above limitations is a significant subject between chemists. Given the above and continuing our recent studies on the design and preparation of novel magnetic and mesoporous catalytic systems, herein novel magnetic methylene and ionic liquid-based bifunctional periodic mesoporous organosilica (BPMO) supported copper with yolk-shell structure (YS-Fe3O4@PMO/IL-Cu) was prepared and applied as an effective and recoverable catalyst for the synthesis of pyranopyrazoles under green conditions. In this BPMO, methylene functional groups are incorporated into the mesoporous walls, while ionic liquid functions are located in the mesoporous channels.
For the synthesis of YS-Fe3O4@PMO, Fe3O4 NPs were first prepared according to our previous procedure (Neysi et al., 2019). Then, Fe3O4 NPs (0.25 g) were added to a reaction flask containing EtOH)16 mL (, H2O (36 mL), CTAB (0.72 g), pluronic P123 (17.1 g) and ammonia solution (0.9 mL, 25% wt). This was stirred at 35°C–40°C for 0.5 h. Next, tetraethyl orthosilicate (TEOS, 0.7 g) and bis(triethoxysilyl)methane (BTEM, 2.1 g) were added to the reaction flask, and stirring continued for 1 h. The resulting mixture was statically heated at 100°C for 17 h. The product was separated, washed with ethanol and water, and dried at 80°C for 7 h. Finally, to obtain a yolk-shell structure, the CTAB and pluronic P123 templates were removed by Soxhlet extraction (Zhang et al., 2008).
For this part of the study, YS-Fe3O4@PMO NPs (0.25 g) were added and ultrasonically dispersed in toluene (20 mL) at RT for 20 min. Then, 1-methyl-3-(3-trimethoxysilylpropyl) imidazolium chloride (0.15 g) was added, and the resulting mixture was refluxed under Ar atmosphere for 1 day. After cooling to room temperature, the product was collected using a magnet, washed with ethanol, dried at 75°C for 11 h, and named YS-Fe3O4@PMO/IL.
First, the YS-Fe3O4@PMO@IL NPs (0.25 g) were sonicated in DMSO (40 mL) for 20 min. Then, Cu(OAc)2.4H2O (0.75 g) was added while stirring at RT for 1 day. The resulting mixture was then stirred at 80°C for 2 h. The product was collected using a magnet, washed with ethanol and H2O, dried at 75°C for 11 h, and named YS-Fe3O4@PMO/IL-Cu (Elhamifar et al., 2017). According to the ICP analysis, the loading of copper on the designed material was found to be 0.45 mmol Cu/g of YS-Fe3O4@PMO/IL-Cu.
For this part of the study, YS-Fe3O4@PMO/IL-Cu catalyst (0.36 mol%) was added to a flask containing aldehyde (1 mmol), malononitrile (1 mmol), ethyl acetoacetate (1 mmol), and hydrazine hydrate (1 mmol). The reaction progress was monitored under ultrasonic conditions at RT. After the reaction was completed, the hot EtOH was added to the reaction flask, and YS-Fe3O4@PMO/IL-Cu was separated using an external magnetic field. The pure pyranopyrazoles were obtained after recrystallizing the crude mixture in EtOH.
IR (KBr, cm-1): 3,480 (NH), 3,253, 3,118 (NH2), 3,075 (=C-H stretching vibration, sp2), 2,927 (C-H stretching vibration, sp3), 2,184 (CN), 1,641 (C=N), 1,467 (C=C), 1,411 (C-O, ether), 869 (C-Cl). 1H-NMR (400 MHz, CDCl3): δ (ppm), 1.90 (s, 3H), 4.45 (s, 1H), 7.01 (d, 1H, j = 8 Hz), 7.11 (d, 1H, j = 8 Hz), 7.76 (s, 1H), 8.60 (s, 2H, NH2), 11.95 (s, 1H, NH). 13C-NMR (100 MHz, CDCl3): δ (ppm) 13.2, 16, 59.3, 110.0, 117.4, 126.7, 130.0, 131.0, 132.4, 135.0, 139.6, 142.6, 163.7, 177.4.
IR (KBr, cm-1): 3,495 (OH), 3,380 (NH), 3,255, 3,120 (NH2), 3,079 (=C-H stretching vibration, sp2), 2,917 (C-H stretching vibration, sp3), 2,187 (CN), 1,619 (C=N), 1,475 (C=C), 1,268 (C-O, ether), 823 (C-Br). 1H-NMR (400 MHz, CDCl3): δ (ppm) 1.94 (s, 3H), 4.53 (s,1H), 5.30 (s, 1H, OH), 6.92 (t, 1H, j = 5.8 Hz), 7.10 (d, 1H, j = 8 Hz), 7.11 (d, 1H, j = 8 Hz), 8.67 (s, 2H, NH2), 11.88 (s, 1H, NH). 13C-NMR (100 MHz, CDCl3): δ (ppm),13.3, 16.5, 59.5, 110.2, 113.4, 117.3, 123.7, 123.9, 128.7, 128.9, 139.6, 156.6, 163.9, 177.6.
The synthesis of YS-Fe3O4@PMO/IL-Cu NPs is presented in Scheme 1. Initially, the surface of Fe3O4 NPs was coated with a periodic mesoporous organosilica shell via hydrolysis and co-condensation of TEOS and BTEM in the presence of CTAB and pluronic P123 surfactants. To obtain a yolk-shell structure, the CTAB and pluronic P123 templates were removed by Soxhlet extraction. Subsequently, the surface of YS-Fe3O4@PMO/IL NPs was modified with a complex of ionic liquid and copper salt to obtain YS-Fe3O4@PMO/IL-Cu catalyst. It is important to note that the YS-Fe3O4@PMO/IL-Cu catalyst is a multifunctional material that contains the advantages of magnetic NPs, supported ionic liquids and YS-structured mesoporous materials. For example, as shown in Scheme 1, IL moieties play a key role in the immobilization and stabilization of catalytic copper species.
Figure 1 demonstrates the FT-IR of Fe3O4@surfactants@PMO, YS-Fe3O4@PMO, and YS-Fe3O4@PMO/IL-Cu NPs. For all samples, the peaks observed at 588 and 3300–3450 cm-1 are related to Fe-O and O-H bonds, respectively. Also, the signals observed at 823 and 1078 cm-1 are assigned to the asymmetric and symmetric vibrations of the Si-O-Si bond, respectively (Figures 1A,B). It should be noted that before surfactant extraction, the sharp peaks at 2923 and 2855 cm-1 are due to C-H stretching vibrations of CTAB and P123 (Figure 1A). After the Soxhlet extraction, the intensity of these peaks is significantly decreased, confirming the successful elimination of surfactants (Figure 1B). In Figure 1C, the peaks at 1418 and 1625 cm-1 are related to C=C and C=N of imidazolium rings, respectively.
The XRD analysis of the Fe3O4, YS-Fe3O4@PMO, and YS-Fe3O4@PMO/IL-Cu catalysts is displayed in Figure 2. This clearly illustrates six signals at 2Ɵ = 30.3, 35.7, 43.4, 53.8, 57.7, and 63.0°, which is in agreement with the standard XRD pattern of Fe3O4 NPs. This confirms that the Fe3O4 NPs are very stable during the preparation of the YS-Fe3O4@PMO/IL-Cu catalyst. It is also important to note that for YS-Fe3O4@PMO and YS-Fe3O4@ PMO/IL-Cu materials, the intensity of PXRD peaks is decreased, indicating the successful modification of magnetite NPs with Me-PMO, IL, and copper moieties. (Figure 2).
The N2 adsorption–desorption isotherm of the YS-Fe3O4@PMO/IL-Cu showed a type IV isotherm with an H1 hysteresis loop, which is characteristic of ordered mesostructures with high regularity (Figure 3). Also, the BET surface area, average pore size, and total pore volume of the designed YS-Fe3O4@PMO/IL-Cu nanocomposite were found to be 659 m2/g, 7.6 nm, and 1.30 cm3/g, respectively. These results demonstrate the good formation of an ordered PMO shell for YS-Fe3O4@PMO/IL-Cu.
The SEM image of the YS-Fe3O4@PMO/IL-Cu catalyst showed the presence of uniform particles with spherical structure and an average size of 70 nm (Figure 4). These are very good NPs for catalytic and adsorption processes.
The VSM analysis showed a saturation magnetization of about 30 emu·g−1 for the designed YS-Fe3O4@PMO/IL-Cu nanocatalyst, lower than that of pure magnetic iron oxide NPs (Figure 5) (Norouzi et al., 2020). This proves the successful coating of PMO shell on magnetite NPs and also confirms the high magnetic properties of the catalyst, which is an excellent characteristic in the catalytic field.
The EDX pattern confirmed the presence of the desired elements in the YS-Fe3O4@PMO/IL-Cu catalyst (Figure 6). This analysis illustrated the signals of C, Si, N, Cu, Fe, and O elements in the catalyst, proving the successful incorporation and immobilization of the expected inorganic and organic moieties into/onto Fe3O4 NPs.
In the next step, TGA analysis was conducted to evaluate the thermal stability of the YS-Fe3O4@PMO/IL-Cu catalyst (Figure 7). The first weight loss below 120°C is due to the loss of water and alcoholic solvents left over from the synthesis process. Another weight loss at 200°C–320°C is related to the decomposition of the remaining CTAB and P123 surfactants. The highest weight loss, observed at 325°C–650°C, is attributed to the removal of methylene and ionic liquid functional groups, which are incorporated/immobilized in/on the structure of YS-Fe3O4@PMO/IL-Cu nanocomposite.
After characterizing the YS-Fe3O4@PMO/IL-Cu catalyst, its application in the synthesis of dihydropyrano [2, 3-c]pyrazoles was investigated. For this part of the study, the condensation between malononitrile, PhCHO, ethyl acetoacetate, and hydrazine hydrate was selected as a model reaction. The effects of the solvent and catalyst loading were studied at RT under ultrasonic conditions. As displayed in Table 1, the effects of different solvents such as EtOH, CH3CN, n-Hexane, DMF, H2O, and solvent-free media were studied, and the best results were obtained in H2O at 25°C (Table 1, entries 1–6). The effect of catalyst loading was also investigated, with the best yield obtained in the presence of 0.36 mol% of YS-Fe3O4@PMO/IL-Cu. According to these results, the use of 0.36 mol% of YS-Fe3O4@PMO/IL-Cu in H2O at 25°C under ultrasonic irradiation was chosen as the optimum condition. In order to prove whether the cupper centers act as catalytic sites or not, in the next study the reaction was carried out using Cu-free Fe3O4, YS-Fe3O4@PMO, and YS-Fe3O4@PMO/IL materials under the same conditions as YS-Fe3O4@PMO/IL-Cu (Table 1, entries 10–12). The result showed that for all Cu-free samples, only a low yield of the desired product was obtained, indicating that the reaction is mainly catalyzed by immobilized copper sites.
TABLE 1. Effect of solvent and catalyst loading in the synthesis of dihydropyrano[2, 3-c]pyrazolea.
After optimizing the different parameters, the efficiency of the YS-Fe3O4@PMO/IL-Cu nanocatalyst was evaluated by using different aldehyde substrates for the preparation of pyrazole derivatives. As seen in Table 2, all investigated aldehydes were converted to their corresponding products in high yields. These results confirm the high efficiency of YS-Fe3O4@PMO/IL-Cu for the preparation of a wide range of biologically active pyranopyrazoles.
TABLE 2. Synthesis of pyranopyrazoles by using YS-Fe3O4@PMO/IL-Cua.
The recoverability and reusability of the YS-Fe3O4@PMO/IL-Cu catalyst were investigated in the condensation of malononitrile, ethyl acetoacetate, benzaldehyde, and hydrazine hydrate under optimized conditions. For this purpose, at the end of the reaction, the catalyst was magnetically removed, washed, and reused in the next run under the same conditions as in the first step. The results indicated that the synthesized catalyst can be recovered and reused at least 9 times without significant loss of efficiency (Figure 8).
A leaching test was then performed to investigate the nature of the catalyst under the reaction conditions. For this purpose, the model reaction was selected as the test. After about 50% of the process was completed, the catalyst was removed using an external magnet, and the reaction progress of the residue was monitored for 60 min. The result demonstrated no further progress of the reaction, confirming no leaching of the active catalytic species and also the heterogeneous nature of the designed catalyst. This result confirms the successful immobilization of the copper moieties on the material framework.
Next, a comparative study was performed between the activity of the YS-Fe3O4@PMO/IL-Cu catalyst and several identified catalysts in the synthesis of pyranopyrazoles (Table 3). The results showed that our designed catalyst is better than other catalysts in terms of catalyst loading, reaction time, and recovery numbers. These findings are attributed to the magnetic nature, mesoporous structure, supported ionic liquids, and high stability of the designed YS-Fe3O4@PMO/IL-Cu nanocatalyst.
TABLE 3. Comparative study between the activity of the YS-Fe3O4@PMO/IL-Cu catalyst and several identified catalysts in the synthesis of pyranopyrazoles.
In this study, the magnetic YS-Fe3O4@PMO/IL-Cu catalyst was prepared and identified by using PXRD, FT-IR, TGA, EDX, ICP, SEM, nitrogen sorption, and VSM analyses. The TGA, EDX, and FT-IR analyses demonstrated the high chemical and thermal stability of YS-Fe3O4@PMO/IL-Cu. The VSM and PXRD analyses showed very good magnetic properties of the material. The nano dimensions and particle size of this catalyst were confirmed using SEM analysis. The nitrogen-sorption diagram also showed a mesoporous structure for the designed catalyst. The YS-Fe3O4@PMO/IL-Cu nanocomposite was used as a powerful catalyst in the synthesis of biologically active pyranopyrazoles, giving the desired products in high yields and selectivity. Moreover, the YS-Fe3O4@PMO/IL-Cu catalyst was easily recovered and reused at least 9 times without any significant decrease in its efficiency.
The original contributions presented in the study are included in the article/supplementary material, further inquiries can be directed to the corresponding author.
MN investigation, writing–original draft. DE conceptualization, writing–review and editing, supervision, visualization. All authors contributed to the article and approved the submitted version.
The authors declare that the research was conducted in the absence of any commercial or financial relationships that could be construed as a potential conflict of interest.
All claims expressed in this article are solely those of the authors and do not necessarily represent those of their affiliated organizations, or those of the publisher, the editors and the reviewers. Any product that may be evaluated in this article, or claim that may be made by its manufacturer, is not guaranteed or endorsed by the publisher.
Ali, M. A. E. A. A., Remaily, E., and Mohamed, S. K. (2014). Eco-friendly synthesis of guanidinyltetrazole compounds and 5-substituted 1H-tetrazoles in water under microwave irradiation. Tetrahedron 70, 270–275. doi:10.1016/j.tet.2013.11.069
Arruebo, M., Galán, M., Navascués, N., Téllez, C., Marquina, C., Ibarra, M. R., et al. (2006). Development of magnetic nanostructured silica-based materials as potential vectors for drug-delivery applications. Chem. Mater. 18, 1911–1919. doi:10.1021/cm051646z
Atar, A. B., Kim, J. T., Lim, K. T., and Jeong, Y. T. (2014). Synthesis of 6-amino-2, 4-dihydropyrano [2, 3-c] pyrazol-5-carbonitriles catalyzed by silica-supported tetramethylguanidine under solvent-free conditions. Synth. Commun. 44, 2679–2691. doi:10.1080/00397911.2014.913634
Babaie, M., and Sheibani, H. (2011). Nanosized magnesium oxide as a highly effective heterogeneous base catalyst for the rapid synthesis of pyranopyrazoles via a tandem four-component reaction. Arabian J. Chem. 4, 159–162. doi:10.1016/j.arabjc.2010.06.032
Dai, J., Zou, H., Wang, R., Wang, Y., Shi, Z., and Qiu, S. (2017). Yolk–shell Fe 3 O 4@ SiO 2@ PMO: Amphiphilic magnetic nanocomposites as an adsorbent and a catalyst with high efficiency and recyclability. Green Chem. 19, 1336–1344. doi:10.1039/c6gc02926d
Du, X., and He, J. (2011). Spherical silica micro/nanomaterials with hierarchical structures: Synthesis and applications. Nanoscale 3, 3984–4002. doi:10.1039/c1nr10660k
Elhamifar, D., Mofatehnia, P., and Faal, M. (2017). Magnetic nanoparticles supported schiff-base/copper complex: An efficient nanocatalyst for preparation of biologically active 3, 4-dihydropyrimidinones. J. colloid interface Sci. 504, 268–275. doi:10.1016/j.jcis.2017.05.044
Gao, J., Liang, G., Zhang, B., Kuang, Y., Zhang, X., and Xu, B. (2007). FePt@ CoS2 yolk− shell nanocrystals as a potent agent to kill HeLa cells. J. Am. Chem. Soc. 129, 1428–1433. doi:10.1021/ja067785e
Ghaedi, M., Elhamifar, D., Negintaji, G., and Banakar, M. H. (2013). Thiol functionalised ionic liquid based xerogel: A novel and efficient support for the solid phase extraction of transition metal ions. Int. J. Environ. Anal. Chem. 93, 1525–1536. doi:10.1080/03067319.2013.814118
Ghorbani-Vaghei, R., and Izadkhah, V. (2018). Preparation and characterization of hexamethylenetetramine-functionalized magnetic nanoparticles and their application as novel catalyst for the synthesis of pyranopyrazole derivatives. Appl. Organomet. Chem. 32, e4025. doi:10.1002/aoc.4025
Gujar, J. B., Chaudhari, M. A., Kawade, D. S., and Shingare, M. S. (2014). Molecular sieves: An efficient and reusable catalyst for multi-component synthesis of dihydropyrano [2, 3-c] pyrazole derivatives. Tetrahedron Lett. 55, 6030–6033. doi:10.1016/j.tetlet.2014.08.127
Holmes, S. M., Zholobenko, V. L., Thursfield, A., Plaisted, R. J., Cundy, C. S., and Dwyer, J. (1998). In situ FTIR study of the formation of MCM-41. J. Chem. Soc. Faraday Trans. 94, 2025–2032. doi:10.1039/a801898g
Hosseini Mohtasham, N., and Gholizadeh, M. (2020). Nano silica extracted from horsetail plant as a natural silica support for the synthesis of H3PW12O40 immobilized on aminated magnetic nanoparticles (Fe3O4@ SiO2-EP-NH-HPA): A novel and efficient heterogeneous nanocatalyst for the green one-pot synthesis of pyrano [2, 3-c] pyrazole derivatives. Res. Chem. Intermed. 46, 3037–3066. doi:10.1007/s11164-020-04133-8
Jangra, A., Kumar, P., Khanna, R., Chaudhary, A., Kumar, S., Singh, J., et al. (2021). A brief and specific review on the utility of surface functionalized magnetite nanoparticles for the removal of dye from contaminated water. Rasayan J. Chem. 14, 829–835. doi:10.31788/rjc.2021.1426207
Kamata, K., Lu, Y., and Xia, Y. (2003). Synthesis and characterization of monodispersed core− shell spherical colloids with movable cores. J. Am. Chem. Soc. 125, 2384–2385. doi:10.1021/ja0292849
Kim, M., Sohn, K., Na, H. B., and Hyeon, T. (2002). Synthesis of nanorattles composed of gold nanoparticles encapsulated in mesoporous carbon and polymer shells. Nano Lett. 2, 1383–1387. doi:10.1021/nl025820j
Kresge, A. C., Leonowicz, M., Roth, W. J., Vartuli, J., and Beck, J. (1992). Ordered mesoporous molecular sieves synthesized by a liquid-crystal template mechanism. nature 359, 710–712. doi:10.1038/359710a0
Liu, J., Qiao, S. Z., Chen, J. S., Lou, X. W. D., Xing, X., and Lu, G. Q. M. (2011). Yolk/shell nanoparticles: New platforms for nanoreactors, drug delivery and lithium-ion batteries. Chem. Commun. 47, 12578–12591. doi:10.1039/c1cc13658e
Liu, J., Xu, J., Che, R., Chen, H., Liu, M., and Liu, Z. (2013). Hierarchical Fe3O4@ TiO2 yolk–shell microspheres with enhanced microwave-absorption properties. Chemistry–A Eur. J. 19, 6746–6752. doi:10.1002/chem.201203557
Mecadon, H., Rohman, M. R., Rajbangshi, M., and Myrboh, B. (2011). γ-Alumina as a recyclable catalyst for the four-component synthesis of 6-amino-4-alkyl/aryl-3-methyl-2, 4-dihydropyrano [2, 3-c] pyrazole-5-carbonitriles in aqueous medium. Tetrahedron Lett. 52, 2523–2525. doi:10.1016/j.tetlet.2011.03.036
Moosavi-Zare, A. R., Zolfigol, M. A., Noroozizadeh, E., Tavasoli, M., Khakyzadeh, V., and Zare, A. (2013). Synthesis of 6-amino-4-(4-methoxyphenyl)-5-cyano-3-methyl-1-phenyl-1, 4-dihydropyrano [2, 3-c] pyrazoles using disulfonic acid imidazolium chloroaluminate as a dual and heterogeneous catalyst. New J. Chem. 37, 4089–4094. doi:10.1039/c3nj00629h
Morishita, M., Shiraishi, Y., and Hirai, T. (2006). Ti-containing mesoporous organosilica as a photocatalyst for selective olefin epoxidation. J. Phys. Chem. B 110, 17898–17905. doi:10.1021/jp062355w
Nasab, M. J., and Kiasat, A. R. (2016). Multifunctional Fe 3 O 4@ n SiO 2@ m SiO 2/Pr-Imi-NH 2· Ag core–shell microspheres as highly efficient catalysts in the aqueous reduction of nitroarenes: Improved catalytic activity and facile catalyst recovery. Rsc Adv. 6, 41871–41877. doi:10.1039/c6ra00120c
Neysi, M., and Elhamifar, D. (2023). Magnetic ethylene-based periodic mesoporous organosilica supported palladium: An efficient and recoverable nanocatalyst for Suzuki reaction. Front. Chem. 11, 1112911. doi:10.3389/fchem.2023.1112911
Neysi, M., Elhamifar, D., and Norouzi, M. (2020). Ionic liquid functionalized magnetic organosilica nanocomposite: A powerful and efficient support for manganese catalyst. Mater. Chem. Phys. 243, 122589. doi:10.1016/j.matchemphys.2019.122589
Neysi, M., and Elhamifar, D. (2022). Pd-containing magnetic periodic mesoporous organosilica nanocomposite as an efficient and highly recoverable catalyst. Sci. Rep. 12, 7970. doi:10.1038/s41598-022-11918-x
Neysi, M., Zarnegaryan, A., and Elhamifar, D. (2019). Core–shell structured magnetic silica supported propylamine/molybdate complexes: An efficient and magnetically recoverable nanocatalyst. New J. Chem. 43, 12283–12291. doi:10.1039/c9nj01160a
Norouzi, M., Elhamifar, D., and Abaeezadeh, S. (2020). Magnetic ethyl-based mesoporous organosilica composite supported IL/Mn: A novel and highly recoverable nanocatalyst for preparation of 4H-pyrans. Appl. Surf. Sci. Adv. 2, 100039. doi:10.1016/j.apsadv.2020.100039
Pandit, K. S., Chavan, P. V., Desai, U. V., Kulkarni, M. A., and Wadgaonkar, P. P. (2015). Tris-hydroxymethylaminomethane (THAM): A novel organocatalyst for a environmentally benign synthesis of medicinally important tetrahydrobenzo [b] pyrans and pyran-annulated heterocycles. New J. Chem. 39, 4452–4463. doi:10.1039/c4nj02346c
Puanngam, M., and Unob, F. (2008). Preparation and use of chemically modified MCM-41 and silica gel as selective adsorbents for Hg (II) ions. J. Hazard. Mater. 154, 578–587. doi:10.1016/j.jhazmat.2007.10.090
Purbia, R., and Paria, S. (2016). A simple turn on fluorescent sensor for the selective detection of thiamine using coconut water derived luminescent carbon dots. Biosens. Bioelectron. 79, 467–475. doi:10.1016/j.bios.2015.12.087
Qiu, P., Kang, K., Kim, K., Li, W., Cui, M., and Khim, J. (2015). Facile synthesis of uniform yolk–shell structured magnetic mesoporous silica as an advanced photo-Fenton-like catalyst for degrading rhodamine B. RSC Adv. 5, 96201–96204. doi:10.1039/c5ra15693a
Tsuji, K., Jones, C. W., and Davis, M. E. (1999). Organic-functionalized molecular sieves (OFMSs): I. Synthesis and characterization of OFMSs with polar functional groups. Microporous Mesoporous Mater. 29, 339–349. doi:10.1016/s1387-1811(99)00003-7
Vartuli, J., Roth, W., Beck, J., Mccullen, S., and Kresge, C. (1998). “The synthesis and properties of M41S and related mesoporous materials,” in Synthesis (Berlin, Germany: Springer).
Vartuli, J., Schmitt, K., Kresge, C., Roth, W., Leonowicz, M., Mccullen, S., et al. (1994). Development of a formation mechanism for M41S materials. Stud. Surf. Sci. Catal. 84, 53–60. doi:10.1016/S0167-2991(08)64096-3
Veysipour, S., Nasr-Esfahani, M., Rafiee, Z., and Eftekhari Far, B. (2021). Fe3O4@ SiO2@ IL-PVP magnetic nanoparticles: Effective synthesis of spirooxindoles. Iran. J. Catal. 11, 125–136.
Wang, Y.-X., Yang, J., Chou, S.-L., Liu, H. K., Zhang, W.-X., Zhao, D., et al. (2015). Uniform yolk-shell iron sulfide–carbon nanospheres for superior sodium–iron sulfide batteries. Nat. Commun. 6, 8689. doi:10.1038/ncomms9689
Xie, J., Tong, L., Su, L., Xu, Y., Wang, L., and Wang, Y. (2017). Core-shell yolk-shell Si@ C@ Void@ C nanohybrids as advanced lithium ion battery anodes with good electronic conductivity and corrosion resistance. J. Power Sources 342, 529–536. doi:10.1016/j.jpowsour.2016.12.094
Yang, Y., Zhang, W., Zhang, Y., Zheng, A., Sun, H., Li, X., et al. (2015). A single Au nanoparticle anchored inside the porous shell of periodic mesoporous organosilica hollow spheres. Nano Res. 8, 3404–3411. doi:10.1007/s12274-015-0840-9
Yin, Y., Rioux, R. M., Erdonmez, C. K., Hughes, S., Somorjai, G. A., and Alivisatos, A. P. (2004). Formation of hollow nanocrystals through the nanoscale Kirkendall effect. Science 304, 711–714. doi:10.1126/science.1096566
Yu, L., Pan, P., Zhang, Y., Zhang, Y., Wan, L., Cheng, X., et al. (2019). Nonsacrificial self-template synthesis of colloidal magnetic yolk–shell mesoporous organosilicas for efficient oil/water interface catalysis. Small 15, 1805465. doi:10.1002/smll.201805465
Zhang, L., Qiao, S., Jin, Y., Chen, Z., Gu, H., and Lu, G. Q. (2008). Magnetic hollow spheres of periodic mesoporous organosilica and Fe3O4 nanocrystals: Fabrication and structure control. Adv. Mater. 20, 805–809. doi:10.1002/adma.200700900
Zhang, Q., and Lee, I. (2013). Core–shell nanostructured catalysts. Acc. Chem. Res. 46, 1816–1824. doi:10.1021/ar300230s
Zhao, X., Sánchez, B. M., Dobson, P. J., and Grant, P. S. (2011). The role of nanomaterials in redox-based supercapacitors for next generation energy storage devices. Nanoscale 3, 839–855. doi:10.1039/c0nr00594k
Keywords: periodic mesoporous organosilica, yolk-shell structure, ionic liquid, nanocomposite, pyranopyrazoles
Citation: Neysi M and Elhamifar D (2023) Yolk-shell structured magnetic mesoporous organosilica supported ionic liquid/Cu complex: an efficient nanocatalyst for the green synthesis of pyranopyrazoles. Front. Chem. 11:1235415. doi: 10.3389/fchem.2023.1235415
Received: 08 June 2023; Accepted: 17 August 2023;
Published: 06 September 2023.
Edited by:
Loïc Leclercq, Université de Lille, FranceReviewed by:
Sushobhan Mukhopadhyay, University of Florida, United StatesCopyright © 2023 Neysi and Elhamifar. This is an open-access article distributed under the terms of the Creative Commons Attribution License (CC BY). The use, distribution or reproduction in other forums is permitted, provided the original author(s) and the copyright owner(s) are credited and that the original publication in this journal is cited, in accordance with accepted academic practice. No use, distribution or reproduction is permitted which does not comply with these terms.
*Correspondence: Dawood Elhamifar, ZC5lbGhhbWlmYXJAeXUuYWMuaXI=
Disclaimer: All claims expressed in this article are solely those of the authors and do not necessarily represent those of their affiliated organizations, or those of the publisher, the editors and the reviewers. Any product that may be evaluated in this article or claim that may be made by its manufacturer is not guaranteed or endorsed by the publisher.
Research integrity at Frontiers
Learn more about the work of our research integrity team to safeguard the quality of each article we publish.