- 1School of Pharmaceutical Sciences, Shoolini University of Biotechnology and Management Sciences, Solan, Himachal Pradesh, India
- 2Formulation Research and Development, Perrigo Company Plc, Allegan, MI, United States
- 3Department of Pharmaceutical Sciences, College of Pharmacy, Larkin University, Miami, FL, United States
Carbon dots (CDs), which have particle size of less than 10 nm, are carbon-based nanomaterials that are used in a wide range of applications in the area of novel drug delivery in cancer, ocular diseases, infectious diseases, and brain disorders. CDs are biocompatible, eco-friendly, easy to synthesize, and less toxic with excellent chemical inertness, which makes them very good nanocarrier system to deliver multi-functional drugs effectively. A huge number of researchers worldwide are working on CDs-based drug delivery systems to evaluate their versatility and efficacy in the field of pharmaceuticals. As a result, there is a tremendous increase in our understanding of the physicochemical properties, diagnostic and drug delivery aspects of CDs, which consequently has led us to design and develop CDs-based theranostic system for the treatment of multiple disorders. In this review, we aim to summarize the advances in application of CDs as nanocarrier including gene delivery, vaccine delivery and antiviral delivery, that has been carried out in the last 5 years.
1 Introduction
First discovered by Walter Scrivens and his colleagues in 2004 (Xu et al., 2004), carbon dots (CDs) possess properties of good photostability, biocompatibility, adaptability, less cytotoxicity, high chemical inertness, easy method of synthesis, eco-friendliness, easy functionalization, non-blinking photoluminescence, and better water solubility. These properties make CDs suitable for use in various fields like drug delivery, bioimaging, and optoelectronics (Ren et al., 2019). In pharmaceuticals, CDs are mainly used as a nanomaterial for drug delivery in various disorders such as cancer, neurological disorders, eye diseases, infectious diseases and for gene delivery (Figure 1) (Zhang et al., 2019a). CDs have functional groups on their surfaces to enhance their water solubility and allow conjugation with organic, inorganic polymers and biomolecules for different applications (Singh et al., 2017). Functional groups, specifically amino, carboxyl, and hydroxyl groups on CDs surface also help further modifications that improve their biocompatibility, optical properties, target ability while increasing their sensitivity and selectivity (Zuo et al., 2016).
The application of CDs depends upon their interaction with the analytes. The interaction of analyte with CDs either decreases fluorescence by quenching or increases fluorescence by inhibiting the quenching effect. The CDs quenching mechanism consists of static quenching, energy transfer, dynamic quenching, photoinduced electron transferred, and inner filter effect. Energy transfer further includes dexter energy transfer, fluorescence resonance energy transfer, and static energy transfer (Zu et al., 2017). CDs gives the advantage to choose surface functionality through electrostatic interaction for selective drug molecules (Singh et al., 2017). To date, different types of CDs have been synthesized for multiple purposes such as for therapeutic drug delivery, drug targeting, gene delivery, biosensing, chemical sensing, bioimaging electrolysis, etc. Researchers also discovered that under near infrared (NIR) light stimulation, CDs emit in the NIR spectral range, expanding its applicability in drug administration, bioimaging, photoacoustic imaging, and anticancer treatment. Due to the excellent photostability and fluorescent property, CDs can also be used as probes in analytical field (Zuo et al., 2016). Recently, green and red-emitting CDs are synthesized to characterize the normal cell and cancer cell through bioimaging (Zeng et al., 2016; An et al., 2021).
Reportedly, research shows that CDs can suppress growth of HepG2 human hepatocellular carcinoma cells (Li et al., 2014). It can also suppress breast cancer cells by generating a huge amount of reactive oxygen species (Hsu et al., 2013). CDs can pass the blood-brain barrier (BBB), which helps in treating brain-related diseases like Alzheimer’s and brain tumor (Dong, 2018; Zhang W. et al., 2021). Worldwide, a significant number of researchers are working on CDs-based drug delivery systems to evaluate their versatility and efficacy in the field of pharmaceuticals. As per the reported scientific data, there is a tremendous increase in our understanding of the physicochemical properties, diagnostic and drug delivery aspects of CDs, which has helped researchers to design and develop CDs-based theranostic system for the treatment of multiple disorders. CDs has potential application in antimicrobial delivery, gene delivery, ocular delivery, cancer targeted delivery, vaccine delivery, antiviral delivery, drug targeting to neurological conditions and enhancing BBB penetration. In this review, we have discussed recent advances in CDs research with special emphasis on their drug delivery applications for different diseases.
2 Structure, method of synthesis and properties of CDs
2.1 Structure
Structurally, the average size of CDs is reported to be less than 10 nm and they may be either graphitic or amorphous in nature (El-Shafey, 2021). CDs exhibit dot like structure with varying size and surface morphology depending up on the selection of precursor and method of synthesis (Wang B. et al., 2022). Amorphous core shell structure with mixed sp2/sp3 hybridization is suggested by some researchers while others have suggested graphitic crystalline structure with sp2 carbon (Mintz et al., 2021; Yadav et al., 2023). CDs are endowed with various functional groups on their surface, which may include hydroxyl (–OH), carboxyl (–COOH), aldehyde (–CHO), amino (–NH2) and sulfhydryl (–SH) group. The type of functional group present on the surface is governed by the precursor used for the preparation of CDs which can further affect their properties. For example, oxygen containing functional groups imparts negative charge to CDs, whereas nitrogen containing functional groups give positive charge, and the difference in charge affects the passivation capabilities of CDs. Therefore, it is important to know the functional groups present on the surface of CDs that can be determined using various conventional techniques such as ninhydrin colorimetry for the determination of amino groups and Boehm titration for carboxyl group determination. The analytical attributes of CDs can be characterized by using transmission electron microscopy (TEM) and X-ray diffraction (XRD).
2.2 Synthesis of CDs
A wide range of methods are available for the preparation of CDs. An ideal method is the one that can synthesize CDs of uniform size, with high quantum yield (QY), scalable, and cost effective. CDs are generally synthesized by two methods, i.e., top-down and bottom-up.
2.2.1 Top-down method
The top-down approach for synthesis of CDs involves breakdown of large carbon precursors (graphene, ash, or soot) to generate nanosized particles (Carbonaro et al., 2019). Different top-down methods include arc discharge, oxidative cracking, laser ablation and electrochemical oxidation (Lim et al., 2015). The arc discharge method of making CDs has the limitation of forming non-uniform size CDs and purification. Other methods such as laser ablation and oxidative cracking uses toxic reagents passivation and are therefore not the preferred choice. The electrochemical oxidation method uses electrolytic graphite rods that can produce crystalline CDs with high photocatalytic activity (Ming et al., 2012). Moreover, the CDs produced by electrochemical oxidation method confer protection from immune-mediated hepatitis due to the interference with activation of T cells and macrophages and their inherent accumulation in liver. The CDs produced by electrolysis have fewer surface functional groups resulting in its poor water dispersibility. This deficiency can be overcome by adding surface functional groups via refluxing.
Top-down approaches provide the advantage of scalability and availability of abundant raw materials for the synthesis of CDs. However, it suffers from the limitation of producing CDs of non-uniform morphology with a wider size distribution. It may also introduce impurities in CDs that can affect its fluorescence property (Wang B. et al., 2022).
2.2.2 Bottom-up method
The bottom-up approach involves production of CDs using carbon precursors that are small organic molecules and polymers containing -OH, -COOH, and -NH2 functional groups. The common procedure used involves dehydration followed by carbonization resulting in formation of CDs with stable properties and uniform morphology with narrow particle size distribution. The bottom-up approach for making CDs includes synthesis via microwave, hydrothermal method, template assisted, cage opening, etc. (Wang B. et al., 2022). Among these, microwave and hydrothermal methods have gained more attraction and are discussed in more detail herein.
2.2.2.1 Microwave
The microwave method used to synthesize CDs provides an efficient route due to its fast heating rate and reaction kinetics. This method produces CDs that are uniform in size and the size obtained can be controlled using microwave power and treatment time. Microwave causes the dehydration and pyrolysis of reaction precursor followed by carbonization to form CDs (Xu et al., 2016). Despite the benefits that microwave method offers, it suffers from the limitation of making unwanted byproducts and needs extensive purification process. Tables 1 and 2 shows several studies in which microwave method was used to make CDs that were later used for biomedical application.
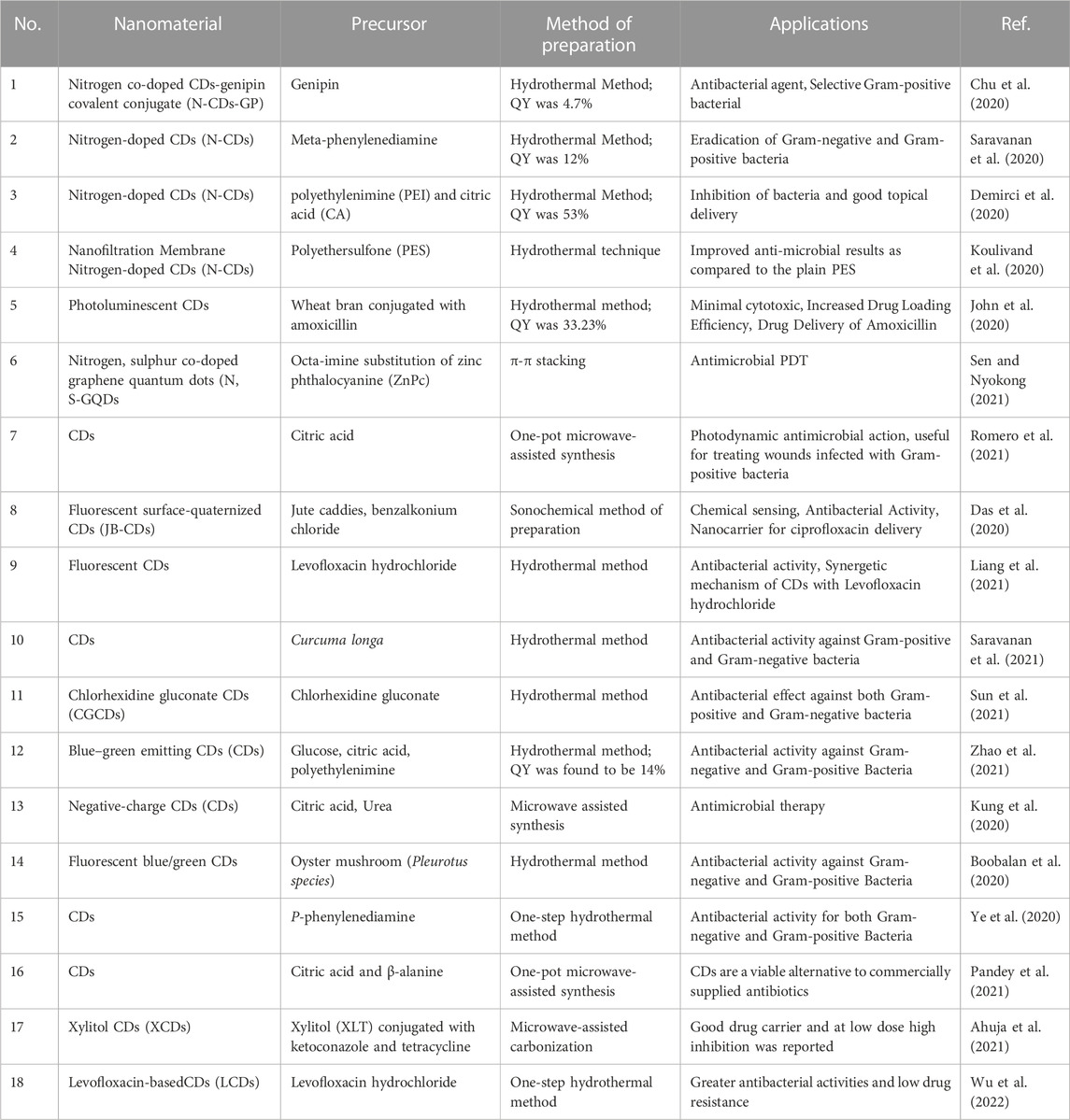
TABLE 1. Recently reported CDs as antimicrobials and their applications for delivery of antimicrobials.
2.2.2.2 Hydrothermal
This is the most commonly used method for CDs synthesis as it is reported to be ecofriendly and inexpensive. This method has gained a lot of popularity and is found to surpass the benefits of microwave methods. The CDs produced by hydrothermal method are endowed with hydrophilic surface functional groups such as -OH, -COOH, or -NH2 groups and thus imparts good water dispersibility (Wang et al., 2019).
In the past few years, the synthetic procedures for the fabrication of fluorescent CDs have become significantly advanced. However, developing simple methods for the synthesis of multifunctional CDs with high QY is still challenging due to limited availability of raw materials and multiple variables involved in the process (Zuo et al., 2016; An et al., 2021). Recently, fabrication of CDs using plant material as a carbon source, such as orange juice (Sahu et al., 2012), beetroot extract (Singh et al., 2018), bread, jaggery and sugar (Sk et al., 2012), has been reported.
2.3 Properties of CDs
CDs have gained a lot of attraction in recent years due to their unique optical properties and biocompatibility. CDs possess optical properties which include absorption and photoluminescence. Due to these properties, CDs are reported to have potential application in the field of bioimaging and theranostics (Naik et al., 2022). CDs are also reported to have biocompatibility and low toxicity which allows the application of CDs as nanocarrier in the field of drug delivery and diagnostics (Biswal and Bhatia, 2021).
2.3.1 Absorption
The absorption behavior of CDs depends on its method of preparation and precursor carbon source. CDs show strong absorbance in the UV region of 200–400 nm and that further extends to visible range. The absorption bands in the visible region are assigned π- π* of C=C bond or n- π* transition of C=O/C=N bond (Adrita et al., 2020; Liu et al., 2020). Some CDs that emit red or near infrared (NIR) emission possess conjugated π domains that allow absorption in the range of 50–800 nm. Moreover, CDs absorption properties are further affected by the size of π conjugated domains, type and content of surface functional groups, and the oxygen/nitrogen content of the carbon cores (Biswal and Bhatia, 2021).
2.3.2 Photoluminescence
Photoluminescence is one of the most important properties of CDs finding wide range of applications especially in the field of biomedical sciences. Various parameters such as size, morphology, internal structure, and composition affect the photoluminescence of CDs. Moreover, the method of synthesis, surface passivation, and the precursor used regulates the photoluminescence of CDs. Among the methods, synthesis via hydrothermal carbonization and microwave are found to augment the photoluminescence of CDs. For passivation, polyethylene glycol (PEG) and polyethylenimine (PEI) are some of the commonly used passivating agents (Biswal and Bhatia, 2021). Studies have shown that imparting chirality to CDs results in enhanced light stability and provides long term imaging. The addition of L-cysteine to the surface of CDs forming L-CDs allows precise imaging of dynamic changes in the Golgi apparatus in the early stages of an infection (Li et al., 2017).
2.3.3 Toxicity of CDs
The increased possibility of the use of CDs in humans has mandated the evaluation of its toxicity profile. The toxicity of CDs can be evaluated by in vitro and in vivo methods. For in vitro methods, assays such as MTT or WST-1 are frequently used in which cultured cells are exposed to CDs and cell viability is determined and compared with the positive control and naïve group (Zhang M. et al., 2021). For in vivo study, CDs are administered to mice or zebra fish and toxicity is assessed through blood analysis, hematological analysis, and inflammatory analysis on various organs such as kidney, spleen, liver, etc (Singh et al., 2018). The prevalence of reports on the toxicity of CDs suggests that CDs are either non-toxic or minimally toxic. The toxicity of CDs depends on their individual physicochemical properties such as surface charge, concentration, photolysis, etc. It has been found that a positive charge on CDs is mainly responsible for its toxicity. Further studies have revealed that increased surface charge density causes significant oxidative stress, IL-8 release, and mitochondrial dysfunction resulting in airway inflammation or allergen-induced immune response (Weiss et al., 2021).
One of the in-vitro studies used HeLa cells for toxicity evaluation of reactive red 2 (RR2), a raw material used for the synthesis of CDs. The results obtained showed that CDs prepared from RR2 showed 70% more cell viability than its precursor, RR2. The in vivo studies performed on zebra fish showed reduced cytotoxicity of CDs and thus further confirmed its safety at preclinical level (Chen W. et al., 2021; Wang B. et al., 2022). Furthermore, to evaluate the biological distribution of CDs in mammals, radiolabeling of CDs was done and the results revealed that CDs were eliminated via kidney and fecal excretion (Tao et al., 2012). This indicates that CDs does not get accumulated and have negligible toxicity in vivo.
3 CDs applications in drug delivery
3.1 CDs in antimicrobial drug delivery
Microbial infection is caused by a number of microorganisms such as Escherichia. coli, Salmonella typhimurium, Streptococcal pneumoniae Bacillus cereus, Mycobacterium tuberculosis, clostridium perfringens, and Staphylococcus aureus (Cui et al., 2020). There is a wide range of antibiotics which are used against these infectious diseases. Inconsistent and regular use of antibiotics are now the main cause of developing antimicrobial resistance (AMR) among humans. Due to AMR, small injuries and common infections will become life-threatening diseases (Zhao et al., 2019). Thus, it is necessary to develop an antimicrobial substrate against resistant bacteria. CDs have significant potential for microbial imaging and itself acts as antimicrobial agents owing to their physicochemical and optical properties which includes simple method of preparation, low toxicity, excellent photostability, good water dispersibility, and flexible surface functionalization (Dong et al., 2020). Antibacterial mechanism of CDs occurs via electrostatic interaction between negatively charged bacterial cell walls and positively charged CDs. It will cause bacterial cell wall lysis and cell apoptosis. CDs acts as a photosensitizer nanomaterial for detection and photodynamic inhibition of bacterial strains, which is a one of the most prominent approaches to fight against multidrug resistance (MDR) pathogens (Figure 2) (Ghirardello et al., 2021).
3.1.1 Antimicrobial applications of nitrogen-doped CDs
Antibacterial activity of CDs mainly depends upon the nitrogen (N) content and their surface charges. In this context, many studies utilized amines or quaternary ammonium salts as precursors for cationic group-based CDs probes synthesis. Also, phosphorus and sulphur-doped CDs were reported as antimicrobials (Ghirardello et al., 2021). Chu et al. (2020), produced nitrogen co-doped CDs-genipin covalent conjugate (N-CDs-GP) for Gram-positive bacterial bioimaging and as antibacterial agents. Saravanan et al. (2020), synthesized nitrogen-doped CDs (N-CDs) in which meta-phenylenediamine was used as a source of carbon and nitrogen. The N-CDs showed bright blue color fluorescence emission with a quantum yield of 12% and inhibited the Gram-negative E. coli and Gram-positive S. aureus at a minimum inhibitory concentration (MIC) of 1 and 0.75 mg/mL, respectively. N-CDs were also used as nanocarrier for ciprofloxacin that was used as a model drug and its release was proven time-dependent. The release kinetics followed Korsmeyer-Peppas model at the physiological pH. Also, trinitrophenol (TNP), an explosive, was analyzed by using N-CDs via fluorescence quenching (Saravanan et al., 2020). Zhao et al. (2019), developed nitrogen and phosphorus co-doped CDs (N, P-CDs), which were used as effective antibacterial material against S. aureus and E. coli. The developed CDs were also utilized for fluorescence imaging of S. aureus, and as a fluorescence probe for the detection of Sudan Red I (Zhao et al., 2019). Demirci et al. (2020), prepared N-CDs using PEI and citric acid (CA) as starting material. The N-CDs were prepared at a yield of 53% ± 4.8% as per quinine sulfate standard using PEI:CA in 1:1 wt. ratio. These N-CDs inhibited the development of both Gram-negative and Gram-positive bacteria without any toxicity to in vitro mammalian cells. Additionally, skin permeation studies indicated good permeation of CDs into and through the dermis, showing their topical administration potential (Demirci et al., 2020). Koulivand et al. (2020), prepared N-CDs added to polyethersulfone (PES) to create new antifouling and antibacterial nanofiltration membranes using hydrothermal technique. All the N-CD-blended membranes displayed improved performance compared to the plain PES when evaluated. It also showed a flux recovery ratio (FRR) of 73.1% revealing its antifouling properties. Membrane showed inhibition of both Gram-positive and Gram-negative bacteria in antibacterial assessment showing antibacterial potential of N-CD-blended membranes (Koulivand et al., 2020).
John et al. (2020), developed photoluminescent CDs from wheat bran using hydrothermal method in which they conjugated CDs with amoxicillin (AMX) in order to assess the application of CDs for delivery of antimicrobial drug. CDs showed blue-green fluorescence emission and higher drug loading efficacy of 69.8% particularly in CD-AMX: 2-800. CD-AMX release profile was tested in different pH conditions (5, 6.8, and 7.2) and showed initial rapid release followed by sustained release up to 70 h. The CD-AMX conjugate showed faster release in acidic conditions (pH-5.0) due to protonation of AMX β-lactam ring in acidic conditions, and thus showed that the conjugate CD-AMX confer sustained pH-sensitive drug delivery. MTT assay was performed at various dilution of CD-AMX prepared using ratio of CD-AMX:2-400 and results showed higher survival rate of 92.23% using 25% diluted CD-AMX. Prepared CDs showed effectiveness against Gram- negative and Gram- positive bacteria with causing significant toxicity, showing its potential as a promising carrier for antibacterial drug delivery (John et al., 2020). Antimicrobial photodynamic therapy (PDT) is an alternate therapeutic technique which is cost-effective and practicable. For this objective, octa-imine substituted zinc phthalocyanine (ZnPc) was synthesised and attached to nitrogen, sulphur co-doped graphene quantum dots (N, S-GQDs) through π-π stacking. Prior to in vitro cell studies, the photochemical and photophysical properties of Pc alone and Pc-conjugated to the GQD nanomaterial were investigated in solutions, including fluorescence, absorption, fluorescence lifetime, singlet oxygen quantum yields, triplet state quantum yields, and excited state lifetimes. Results showed that photodynamic inactivation increased with ZnPc conjugation to N, S-GQDs (Sen and Nyokong, 2021).
3.1.2 CDs-mediated delivery of antimicrobials
Romero et al. (2021), studied antimicrobial photodynamic effect of CDs developed from citric acid against S. aureus suspension and biofilm. In vivo studies were conducted in mice with wounds contaminated with S. aureus. The viability test showed 104 log reduction in bacterial count on the skin lesions via CDs-mediated photodynamic inactivation. These findings showed promising application of CDs based antibacterial PDT in the treatment of wounds infected with Gram-positive bacteria (Romero et al., 2021). Das et al. (2020), prepared fluorescent surface-quaternized CDs (JB-CDs) sonochemically from jute caddies and modified their surface characteristics by adding benzalkonium chloride (BZC). Results showed that JB-CDs exhibited good water solubility, photostability and excitation-dependent emission. These JB-CDs were used as a fluorescent nano switch for the detection of inorganic pollutants in aqueous solutions, such as chromium (VI) [Cr (VI)] ions. To confirm antibacterial activity, JB-CDs were tested against S. aureus and E. coli indicating substantial inhibition of bacterial growth. Apart from this, JB-CDs were studied as nanocarrier for drug delivery of ciprofloxacin. In vitro release study results showed pH-responsive controlled release behavior of JB-CDs at physiological pH (Das et al., 2020). In another study, Liang et al. (2021), reported antibacterial activity of CDs incorporated with levofloxacin hydrochloride. CDs showed capable antibacterial properties against both Gram-positive and Gram-negative bacteria without causing any cytotoxicity. Mechanism of antibacterial action of CDs included their physical/chemical attachment to cell membrane, surface wrapping and then cell membrane breakdown, which caused increased production of reactive oxygen species (ROS) within the cell without the use of additional light or oxidant (Liang et al., 2021). Saravanan et al. (2021), prepared CDs from Curcuma longa, which were examined for their antibacterial efficacy against Gram-positive bacteria, S. aureus and S. epidermidis, and Gram-negative bacteria, E. coli and K. pneumoniae. Sun et al. (2021), performed a study in which chlorhexidine gluconate-derived CDs were divided into three groups on the basis of their particle size by applying different molecular weight cut-off membranes. Their findings revealed that antibacterial activity against Gram-negative bacteria and Gram-positive bacteria improves as CDs size decreases. This phenomenon might be caused by changes in the cellular absorption and CDs distribution in the cell membrane, or by a limitation between the polar functional group and the DNA molecule (Sun et al., 2021). Zhao et al. (2021), synthesized CDs with blue-green fluorescence under low reaction temperature (30 min, 60°C). The inhibitory action of developed CDs against diverse microbes, including bacteria and fungus, were compared using inhibition zone tests and minimum inhibitory concentration (MIC) studies (Zhao et al., 2021). In another study, negative charge CDs were prepared from citric acid and urea as precursors and evaluated for their antibacterial action against MDR bacteria. Results revealed that the developed CDs have the potential to be effective against MDR S. aureus and can be used as an alternative to antibacterial treatment (Kung et al., 2020). Boobalan et al. (2020), developed fluorescent blue/green CDs using oyster mushroom and demonstrated their antibacterial activity. Ye et al. (2020), developed CDs for antibacterial action against S. aureus and E. coli using p-phenylenediamine as carbon source through a simple hydrothermal method. The minimum bactericidal concentrations of the CDs were found to be 2 and 30 μg/mL, against S. aureus and E. coli, respectively (Ye et al., 2020). Similarly, Pandey et al. (2021), prepared CDs from citric acid and β-alanine. Its antimicrobial activity was studied against several Gram-negative bacteria, including E. coli, Salmonella, Agrobacterium, Pseudomonas, and Pectobacterium species. Results from this showed that CDs can act as an effective alternate to commercially available antibiotics (Pandey et al., 2021). Ahuja et al. (2021), synthesized CDs using xylitol (XCDs) which were conjugated with antimicrobial drugs ketoconazole and tetracycline for fungi and bacteria, respectively. Results showed higher inhibitory potential with reduced dose of antimicrobials using XCDs as compared to xylitol against C. neoformans, C. albicans, S. pyogenes, E. coli, L. monocytogenes and S. typhi. Drug delivery was also improved in the presence of XCDs showing drug carrier potential of CDs (Ahuja et al., 2021). Levofloxacin-based CDs (LCDs) with low drug resistance and greater antibacterial activities were developed by Wu et al. (2022). In vitro and in vivo studies showed excellent antibacterial activity of LCDs (Wu et al., 2022). The antibacterial activity of recently reported CDs and their applications in delivery of antimicrobials are summarized in Table 1.
More research in the use of CDs as antimicrobials can be explored by preventing ROS from damaging the normal cells. To further improvise the antimicrobial efficacy, it is needed to investigate the use of high performing precursor as a source of carbon for the synthesis of CDs and that should be water soluble and dispersible for ease of penetration and delivery.
3.2 CDs in ocular drug delivery
Ocular infection such as bacterial keratitis or endophthalmitis are not uncommon and various nanomaterials such as silver, copper oxide, iron oxide, titanium oxide, and zinc oxide particles received attention for their anti-bacterial properties. However, the toxicity of these inorganic materials and metal oxide poses a major concern and can be circumvented with the use of CDs. Moreover, the small nanoparticle size of CDs (<10 nm) further makes them suitable drug delivery vehicle as it causes no or minimal irritation in the eyes following administration (Garner et al., 2020).
Though CDs have good potential in novel biomedical applications due to their physicochemical properties like biocompatibility, fluorescence, and conjugation ability with various therapeutic agents, there are limited studies available regarding their applications for ocular drug delivery (Garner et al., 2020). Jian et al. (2017), developed an antibacterial agent for topical treatment of bacterial keratitis (BK) using carbon quantum dots (CQDs). The possible antibacterial mechanism suggested that the super-cationic CQDs prepared by direct pyrolysis of spermidine (Spd) (CQDSpds) had a small size and a large positive charge which ruptured the bacterial membrane. In addition, topical ocular application of CQDSpds can cause the tight junction of corneal epithelial cells to open, resulting in a significant antibacterial effect in rabbits with S. aureus induced BK. These findings demonstrated CQDSpds to be a viable antibacterial option for the treatment of eye-related bacterial infections and even chronic bacteria-induced illnesses (Jian et al., 2017). Shoval et al. (2019), developed ocular nanomedicine using hybrid aptamer modified-CDs for controlled release of inhibitors of vascular endothelial growth factor (VEGF), which plays a main role in the pathogenesis of angiogenic ocular diseases. The hybrid nanoparticles were made using CDs that had been functionalized with the VEGF aptamer. The hybrid CDs efficiently suppressed VEGF-stimulated angiogenesis in choroidal blood vessels in both in vitro and in vivo models (Shoval et al., 2019). Wang et al. (2022b), developed ocular drug delivery system based upon CDs embedded in thermosensitive in situ gel for the topical administration of diclofenac sodium (DS). In vitro results showed sustained release of DS for 12 h and ex vivo fluorescence delivery indicated that this system could be used for cell imaging and ocular tissues tracing. A positive charge on the composite of DS-CD along with the gel prolonged the precorneal retention and thus improved the bioavailability (Wang et al., 2022b). In another study, Wang et al. (2021a), prepared a composite system for ocular delivery that combined self-targeted CDs and thermosensitive in situ hydrogels. Electrostatic interactions were used to load DS onto the surface of CDs, resulting in DS-CDs nanoparticles that have the characteristics of biphasic drug release. The in vivo fluorescence and corneal penetrability studies revealed that drug retention duration was extended, and corneal transmittance was increased. The DS-CD Gel demonstrated high cytocompatibility and CD44 targeting in cellular studies (Wang et al., 2021a). There are only limited number of scientific reports available on CDs application in the ocular field, therefore the potential application of CDs in this area needs to be explored further.
3.3 CDs for drug delivery in the brain
The human brain is the most complex interconnected network and the most important part of the body. Major causes for brain disorders are illness, genetic changes and traumatic injury. Main hurdle for drug delivery in brain is the BBB (Dong, 2018). Drug delivery across the BBB is very challenging, particularly in brain tumor and Alzheimer’s disease (AD). Most remedies and drugs have limited application when it comes to brain delivery due to their inability to cross the BBB. To that end, some exciting research is being performed to enhance the delivery of therapeutic agents to the brain using nanomaterials, especially CDs. CDs were reported to cross the BBB through passive diffusion owing to their very small size, amphiphilicity, positive surface charge and receptor-mediated endocytosis (Zhang W. et al., 2021).
3.3.1 CDs-mediated BBB permeability
CDs also have limitations regarding BBB penetrability as other small molecules. Mintz et al. (2019), proposed that if CDs can be synthesized from a precursor molecule that can cross the BBB, there are chances that the attached original precursor molecule with the CDs can enter the brain. As a result, they produced tryptophan CDs utilizing the amino acid method for crossing the BBB via LAT1 transporter-mediated endocytosis. Urea and 1,2-ethylenediamine were used as nitrogen source to develop tryptophan CDs. These CDs were evaluated in zebrafish central nervous system to assess their BBB permeability. Results revealed that CDs prepared with tryptophan amino acid acted as a promising carrier system for drug delivery and imaging in the brain (Mintz et al., 2019). Zhou et al. (2019), synthesized yellow-emissive CDs (Y-CDs) as promising drug nanocarriers across BBB using o-phenylenediamine and citric acid as raw material. The ability of synthesized Y-CDs to cross BBB was evaluated in wild-type zebrafish using confocal image analysis. Results showed that Y-CDs crossed the BBB most possibly by means of passive diffusion owing to their amphiphilic nature. Furthermore, Y-CDs suppressed the overexpression of human amyloid precursor protein (APP) and amyloid (A), both of which are involved in AD pathogenesis. Hence, results suggested the drug delivery potential of Y-CDs in brain as well as their ability to inhibit Aβ-related pathology in AD (Zhou et al., 2019). Zhou et al. (2020), directly conjugated two different CD models, black CDs (B-CDs) and gel-like CDs (G-CDs) for potential application of CDs as nanocarrier for brain delivery. As a result of conjugation, black-gel CDs (B-G CDs) possessed properties from both CDs, such as greater thermostability, better aqueous stability and red-shifted photoluminescence emission. Also, nanostructure formed with the decreased mass ratio of B-CDs to G-CDs showed good potential of CDs as Lego-like building blocks. Moreover, zebrafish bioimaging exhibited the bone targeting and BBB crossing potential of B-G CDs (Zhou et al., 2020). Despite showing promising drug carrier potential, a better understanding of drug loading capacity and release kinetics needs to be investigated. Few in vitro studies have shown CDs to produce an immune response at high concentrations (Lategan et al., 2018). Therefore, it is also important to optimize the dose of CDs for future clinical studies.
3.3.2 CDs as nanocarriers for delivery of neuroprotective drugs
In order to treat a brain-related medical condition, delivery of therapeutic agent across BBB was a major bottleneck. Since CDs have the ability to cross BBB, various therapeutic agents can be delivered in desired concentration to achieve a desired pharmacological effect in the brain. Herein, we have discussed studies showing efficacy of CDs in delivering therapeutic agents either in vitro or in vivo. Hettiarachchi et al. (2019), developed CDs based triple conjugated system for delivering drug in glioblastoma brain tumors. Triple conjugated system of CDs was formed with targeted ligand transferrin and anticancer drugs, temozolomide and epirubicin. Glioblastoma brain tumor cell lines were used for in vitro evaluation. The efficacy of the synthesized triple conjugated CDs system was compared to free drug combinations, non-transferrin CDs–drugs, and dual conjugated systems (single drug conjugation along with transferrin). Triple conjugated CDs system at a very low concentration showed the lowest cell viability. Moreover, the triple conjugated CDs system caused more cytotoxicity to brain tumor cell lines as compared to other groups (Hettiarachchi et al., 2019). Chung et al. (2020), developed β-amyloid (Aβ) protein targeted photomodulating CDs for treatment of AD, as Aβ peptide aggregates worsen neuropathy and cognitive impairment in AD. Similarly, nanocarrier ability of carbon nitride dots (CNDs) were evaluated for targeted pediatric glioblastoma cells using gemcitabine as model drug by Liyanage et al. (2020). In another work, Li et al. (2021), created new boron-containing CDs (BCDs) for boron neutron capture therapy (BNCT) for glioma treatment with higher water solubility and excellent optical properties for tracing 10B in vitro and in vivo. Fluorescent imaging showed internalization of BCD–Exosomes around the nuclei of U-87-MG glioma cells in vitro. Prominent BNCT effect of the BCD–Exosomes-treated brain glioma in the mice model was demonstrated with 100% survival ratio (Li et al., 2021). CDs derived from metformin (Met-CDs) were developed for mitochondrial and nucleus localizations along with BBB penetration. Bioimaging studies showed Met-CDs penetration in the cell membrane and their localization specifically inside the cancer cells mitochondria. In vivo study performed in zebrafish study confirmed BBB penetrability of Met-CDs without the prerequisite of any other ligands (Kirbas Cilingir et al., 2021). Zhang et al. (2021c), derived novel Crinis Carbonisatus CDs (CrCi-CDs) obtained from carbonization of human hair, and investigated their neuroprotective effect against cerebral infarction in stroke. Neuroprotective study results in in vivo middle cerebral artery occlusion (MCAO) model showed significant reduction in neurological deficits (Zhang et al., 2021c). Presently, most CDs based nanocarriers showed BBB penetration ability which depends upon specific ligands for ligand-mediated endocytosis. So, self-targeting CDs with potential of penetrating the BBB need to be explored further by considering various precursors, drug and nanocarrier compared to non-self-targeting CDs. Apart from this, CDs mechanism for BBB penetration need to be understood well for their CNS drug delivery applicability (Zhang W. et al., 2021).
3.4 CDs as nanocarrier for gene delivery
Progress of novel multifunctional gene delivery systems with greater efficacy is important. In recent years, CDs has emerged as an imaging-trackable nanocarrier for gene delivery applications which may yield nanoparticles with positive charge for interaction with the nucleic acids bearing negative charge (Mickaël et al., 2019; Mohammadinejad et al., 2019). Due to their high transfection efficiency (TE), CDs can be used efficiently for plasmid DNA and siRNA delivery to the targeted cells without any toxic effects (Mohammadinejad et al., 2019). He et al. (2019), synthesized CDs from Gd(III) salts/complexes (L-CD/C-CD), cationic polymers, and citric acid for application in gene delivery and multi-modal (MR/FL) imaging. In vitro gene transfection studies using L-CD exhibited 74 times higher efficiency of transfection and anti-serum abilities compared to PEI 25 kDa. Gene delivery process was evaluated by confocal laser scanning microscopy, which showed blue or green fluorescence in HeLa cell lines. Besides, the L-CD/C-CDs possess appropriate particle size that resulted in increased buildup at the tumor location via the enhanced permeability and retention effect (EPR) thereby proving it to be more effective than a widely used contrast agent, i.e., Gd-DTPA (diethylenetriamine penta-acetic acid) in in vivo tumor-specific MRI imaging (He et al., 2019a). Another study from the same lab also reported two cationic polymer-derived CDs with blue fluorescence for creating biocompatible, multipurpose gene vectors with high TE (He et al., 2019b). Mickaël et al. (2019), developed cationic CDs by citric acid/bPEI600 (1/4, w/w) pyrolysis to remove the unreacted low molecular weight reagents which causes nanoparticles separation problem from residual polymer that is harmful to cells. Eleven CDs exhibiting greater water solubility were developed after evaluating reaction conditions and activation modes. CDs showed noticeable variation in their gene delivery efficiency in epithelial cell line A549 using a pDNA encoding the Gaussia princeps luciferase gene after having similar physical properties. CDs synthesized in domestic oven under microwave irradiation revealed to be superior to all the other CDs. It was also compared with the bPEI25k, gold standard transfection reagent which is associated with cytotoxicity. Results showed that optimal CD/pDNA w/w ratio shifted down to 2 while it was ca. 4 with bPEI25k and thus limiting the toxicity associated with bPEI and related compounds (Mickaël et al., 2019). Chen et al. (2020), synthesized hydrophobically modified CDs from PEI via an epoxide ring-opening reaction. Study results presented the dual-channel imaging capability of CDs, due to which intracellularly delivered DNA can be tracked. Oleyl-modified-CDs showed 200 times more TE as compare to PEI 25 kDa in the presence of serum in A549 cells (Chen et al., 2020). In another study, CDs PAMAM nanohybrids were developed by self-assembling of CDs and G4-G6 (polyamidoamine) PAMAM-NH2 dendrimers for transfection and bioimaging purposes. The nanohybrids were found to be more photostable, compared to pristine CDs. These nanoscale hybrids were internalized in the cells with excellent TE thereby proving promising applications in the biomedical field (Martins et al., 2021). In summary, more studies are needed to fully understand the interaction of CDs with living cells and to further explore their potential in living cells or species that are resistant to transformation. More work needs to be pursued at the clinical level for efficient bench-to-bedside translation of CDs as a carrier for gene delivery.
3.5 CDs as nanocarrier in anticancer drug delivery
Cancer refers to a group of diseases which can affect any part of the body causing uncontrollable and abnormal cell growth which potentially invades or spreads to the adjoining parts of the body. Over 100 types of cancer forms are there which are affecting humans worldwide. As per World Health Organization, it is estimated that cancer-related deaths are expected to increase to around 13.1 million by the year 2030 (Stewart and Kleihues, 2003). Advancements of various nanotechnology tools is being used for the treatment of various deadly diseases including cancer (Montané et al., 2020). CDs belonging to the family of carbonaceous nanomaterials are identified as potential candidates in nanotheranostics for the treatment, bioimaging and early diagnosis of cancer due to their exclusive optical properties and intrinsic theranostic properties, which are summarized in Table 2 (Jia et al., 2020; Singh et al., 2021).
Recent studies showed greater potential of CDs as nanocarriers in the field of cancer drug delivery and biological imaging. Das et al. (2019), developed sulfur and nitrogen doped photoluminescent CDs from κ-carrageenan and folic acid for cancer cell targeting. The folate receptor present on cancer cells led to remarkable cancer cells targetability by CDs which makes them a potential tool for biomedical studies as shown in Figure 3 (Das et al., 2019). Arsalani et al. (2019), developed polyethylene glycol passivated fluorescent CDs (CDs-PEG) from gelatin and PEG as nanocarrier for tumor treatment using methotrexate (MTX) as anticancer drug. The prepared CDs-PEG emitted blue photoluminescence with a maximum quantum yield of 34%. The effect of PEG on PL intensity of CDs was investigated and results showed stronger PL behavior of CDs-PEG as compared to pure CDs from gelatin. In vitro anticancer activity of CDs-PEG showed good antitumor efficacy than free MTX because of its greater in vitro nuclear delivery and thus proving the potential of CDs for targeted cancer therapy (Arsalani et al., 2019). Li et al. (2020a), developed smart mesoporous silica nanoparticles (MSNs) grafted with CDs and poly (N-vinylcaprolactam) (PNVCL) as a mixed shell (CDs/PNVCL polymer grafted MSNs) that allowed real-time monitoring and pH-triggered anticancer drug release. Doxorubicin (DOX) was loaded with the prepared CDs as a model drug. The study results demonstrated the qualities of excellent nanocarriers, such as low toxicity, limiting early release in the bloodstream by “caps” of CDs, and collapse of PNVCL. The composite allowed burst release of anticancer drug at the tumor site due to the rupture of Schiff base bonds. Through MTT experiments it was also suggested that CDs/PNVCL polymer grafted MSNs killed cancer cells without affecting normal cells. The ability of CDs fluorescence to monitor medication release in real time was studied and the results revealed linear connection in cumulative release of DOX and the fluorescence change of CDs (Li et al., 2020a). On the other hand, Su et al. (2020), reported that red-emissive carbon quantum dots (CQD), can be targeted to nuclei of cancer cells and cancer stem cells. DOX loaded on the surface of CQD reduced the cell viability of HeLa cells to 21% in contrast to 50% when cells were exposed to free DOX. The study results showed remarkable killing impact of CDs on tumor cells and improved treatment effectiveness with no recurrence (Su W. et al., 2020).
Li et al. (2020b), developed fluorescent-based CQD with photosensitizer functionalized and chemo drug-loaded nanocarrier system in which CQD and 5-aminolevulinic acid (5-ALA) were coupled with a mono-(5-BOC-protected-glutamine-6-deoxy) b-cyclodextrin (CQD-Glub-CD) moiety, and then DOX was loaded into the 5-ALA-CQD-Glub-CD system. In vitro drug release pattern of DOX from the system was found to be pH-dependent with higher release at acidic pH 2.8. The photodynamic action of ROS production causes cell damage and morphological alterations in cells tested for breast cancer cell line (Li et al., 2020b). Hailing et al. (2020), developed fluorescent CDs loaded with DOX and passivated with PEI (CD-PEI-DOX) as a drug delivery system for treating cancer. Results showed prolonged drug release from the system with improved toxic effect on liver cancer cells (MHCC-97L and Hep3B cells) compared to free DOX (Hailing et al., 2020). Sun et al. (2020), developed DOX conjugated CDs (DOX-CDs) for improved intracellular drug delivery and cellular imaging. Uptake of DOX-CDs by cancer cells was seen in fluorescent cell labelling studies. Moreover, it was also observed that the DOX release from DOX-CDse was endo-lysosomal pH-dependent. MTT assay performed in ovarian cancer cell lines showed the enhanced cytotoxic potential of DOX-CDs. Additionally, in an animal imaging study, the CDs displayed bright fluorescent signal and low toxicity after administration for 7 and 21 days (Sun et al., 2020). Liu et al. (2021), developed endogenous NO-releasing carbon nanodots for gas therapy as cancer treatment. In vitro studies performed on human breast cancer cell line MCF-7, female gastric cancer cell line BGC-823, male lung cancer cell line A549 and female leukemic cell line K562 showed that carbon nanodots killed tumor cells without affecting normal cells. In vivo results also showed antitumor efficacy of carbon nanodots (Liu et al., 2021). Wang et al. (2020), developed a CDs-DOX delivery carrier and imaging probe for liver cancer targeted therapy with high fluorescence quantum yield (97%). Folic acid, as targeting component was used to modify CDs (FA-CDs) to which DOX was further loaded to form FA-CDs-DOX. Confocal microscope showed excellent capability of fluorescence imaging of developed system in liver cancer cells. In vivo imaging study showed stronger fluorescence intensity of FA-CDs-DOX which makes it easy to penetrate tumor tissue and skin. In vivo tumor inhibition studies indicated the higher targeting ability of FA-CDs-DOX as compare to free DOX, showing improved therapeutic effect. Cutrim et al. (2021), showed the nanofabrication of drug delivery system based on the self-assembly of the 5-Flurouracil (5-FU) onto the surface of CQD. The 5-FU-CQD nanoconjugate was less hazardous to normal cells than free 5-FU at equal concentrations, yet it had the same anticancer effect against a breast cancer cell line (MCF-7) as free 5-FU. The findings clearly suggested that the 5-FU-CQD nanoconjugate may improve cancer therapy effectiveness by lowering the toxicity of 5-FU to normal cells, reduce side effects as well that are intolerable for patients while retaining the therapeutic efficacy of 5-FU (Cutrim et al., 2021). In another study, Qin et al. (2021), reported fluorescent CQDs for in vivo bioimaging and targeted cancer therapy. Similarly, Ren et al. (2021), developed a red emissive polymer CDs-based nanocarrier system of coptisine, a poorly bioavailable drug, with integrated functionality for in vivo and in vitro simultaneous imaging and drug administration. Red emissive CDs serve many purposes in this nanocarrier system, including drug carrier, EPR effect, and simultaneous imaging. In vitro test demonstrated that polymer-based red emissive CDs might provide a prolonged drug release and improve coptisine effectiveness against cancer cells. The fluorescent CDs usefulness as a tumor-targeted drug delivery carrier of coptisine for cancer treatment was proven in an in vivo investigation (Ren et al., 2021). Lv et al. (2021), synthesized multi-functional CDs using folic acid as nitrogen source and for tumor targeting. Gallic acid was used as a carbon source as well as active antitumor moiety. In vitro cell imaging studies and in vivo antitumor studies performed on HeLa cell lines showed targeted imaging and antitumor abilities (Lv et al., 2021).
There are various phytochemicals which are reported for its anti-cancer activity, curcumin is one of them which has been reported effective against various cancers but due to its low aqueous solubility, stability, and poor bioavailability, its clinical results were not satisfactory. Sharma et al. (2021), developed curcumin CDs (CurCDs) using curcumin as carbon source and as an active molecule owing to its anti-cancer properties. CurCDs were compared with the plain curcumin and evaluated further for its anti-proliferative, apoptotic, and anti-migratory activities in glioblastoma (GBM) cells. Study revealed the superiority of CurCDs over free curcumin in terms of improved stability and enhanced bioavailability (Sharma et al., 2021). Mohammadi et al. (2021), developed CDs-chitosan nanocomposite hydrogels for multicolor imaging of MCF-7 cancer cells. These nanocomposite detects microRNA-21 in MCF-7 cancer cells and thus demonstrate enhanced sensing properties for biomarker detection (Mohammadi et al., 2021). 6-mercaptopurine (6-MP) used for treatment of acute lymphoblastic leukemia, suffers from short half-life, poor bioavailability with severe side effects. To overcome all these shortcomings, 6-MP was conjugated with CDs through GSH-sensitive carbonyl vinyl sulfide linkage, and biotin was attached to the surface. Resulting compound revealed excellent stability in the phosphate buffer saline (PBS), lower cellular toxicity on normal cell lines (CHO) and good activity, comparable to free 6-MP against MCF-7 and HepG2 cancer cells (Talib and Mohammed, 2021). In another study, folic acid-based CDs (FACDs) were investigated for DOX delivery and diagnosis by Fahmi et al. (2021). Wen et al. (2020), synthesized novel nitrogen and sulfur co-doped CDs with bright orange fluorescence for mitoxantrone (MTO) delivery. In wide pH range, the prepared CDs displayed good fluorescence stability in a wide range of pH and its fluorescence quenched by folate, showing its sensitivity to FA with a detection limit as low as 0.85 nM. CDs also exhibited biocompatibility and greater photobleaching resistance due to which they could be applicable in cell imaging and drug tracking. In vitro studies were performed using MTO-CDs and their results showed cell inhibition confirmed by MTT assay and change in cell morphology. The combination of MTO-CDs provide a nanosystem allowing simultaneous imaging and anticancer effect and the presence of fluorescence did not affect the cell killing property of MTO (Wen et al., 2020).
CDs are also reported to have potential application for photodynamic and photothermal therapy in diagnosing and treating cancer (Nocito et al., 2021). Metal and heteroatom-doped CDs have potential applications in PDT for cancer diagnosis and treatment (Sekar et al., 2022). Nasrin et al. (2020), synthesized nucleus targeting CDs for PDT in oral cancer treatment. Li et al., (2020c), prepared sulphur doped CDs (S-CDs), as an PI3/Akt pathway inhibitor thereby significantly reducing cancer cell survival with high PDT performance. In another study, nuclei acid targeted CDs were developed by Xu et al. (2021), from quinolone derivatives (Cl-CDs, I-CDs). In vitro, these CDs produces RNA fluorescence and due to iodine doping possesses PDT led to the killing of cancer cells (Xu et al., 2021). Chen et al. (2021b), fabricated an injectable hydrogel by developing amido modified CDs (NCDs) for simultaneous phototherapy and PDT for cancer treatment. Phototherapy agents were incorporated into hydrogels with high loading efficiency. NCDs, acting as phototherapy agent, showed effective tumor inhibition and thus provided a new strategy for advanced tumor treatment (Chen et al., 2021b). In another study, Yue et al. (2021), synthesized green fluorescent riboflavin-based CDs having singlet oxygen generation ability for PDT in cancer treatment. In vivo results showed significant inhibition of tumor growth after PDT with CDs (Yue et al., 2021). Novel iodine doped CDs (I-CDs) exhibiting PDT performance and nucleic acid targeting properties were synthesized by Xu et al. (2021). In vitro results revealed that photodynamic I-CDs significantly killed cancer cells thereby confirming the targeting capabilities of CDs (Xu et al., 2021). Hu et al. (2021), synthesized and evaluated Sn nanocluster CDs for potential application in PDT in cancer treatment. In vitro and in vivo study results showed promising potential of CDs in PDT (Hu et al., 2021). Also, novel green fluorescent fluorine and nitrogen co-doped carbon dots (F, NCDs) for image guided PDT of cancers were synthesized and evaluated by Wu et al. (2022). In vitro study on HepG2 cell lines showed higher photodynamic efficiency and better cell imaging capability of the F, NCDs (Wu et al., 2022). Conclusively, recently reported studies showed significant potential of CDs as photosensitizer in photodynamic and photothermal therapy in cancer treatment. Although the applications of CDs in cancer theranostics showed promising potential, various challenges limit its use in humans due to lack of information on its genotoxic potential. This needs to be investigated thoroughly so that the CDs can be delivered to the cell nuclei targeting bad genetic material in order to augment antitumor efficacy. Furthermore, currently phototherapy entails an imaging agent that has an overlapping absorption coefficient and lies in the biological transparency window of 650–950 nm. Therefore, it is an urgent requirement to develop CDs with emission in the NIR region.
3.6 CDs in controlled release and smart stimuli-responsive drug delivery system
A sustained drug delivery system tries to release the drug slowly for an extended period of time to maintain the constant concentration of the drug in blood or target tissue and thus leads to desired therapeutic effect in diseased conditions that requires drug concentration to be maintained for a long period of time. Smart stimuli-responsive drug delivery system uses natural or synthetic polymers that are designed to show therapeutic responses against the physicochemical and physiological processes, as well as external stimuli. This is particularly advantageous in reducing the side effects due to premature release of drug and assist in achieving the delivery of drug at the targeted site (Indermun et al., 2018). Zhang et al. (2019a), developed hollow CDs (HCDs) as a drug carrier for sustained delivery of DOX. After 80 h of in vitro drug release study, 74.7% DOX was released at pH 5.0, 42.6% at pH 6.5% and 29.5% at pH 7.4 (Figure 4). This was due to the dissociation of carboxylic acid hydrazine bond of HCDs-DOX by hydrolysis in acidic condition. In the case of free DOX release profile, no significant difference was observed at 5.0, 6.5, and 7.4 pH. 60% of the drug was released within 2 h. This indicated that free DOX could not reach the tumor cell effectively and HCD-DOX could be used as a potential sustained release drug delivery system for cancer treatment. The results of the study further demonstrated that HCDs-DOX effectively inhibited cancer cell proliferation and showed lower cytotoxicity to healthy cells as compared to DOX (Zhang et al., 2019a). Duan et al. (2020) introduced heparin (Hep), an anticoagulant, in conjunction with a drug delivery method based on CDs, i.e., DOX-CDs-Hyaluronic acid (HA) that can delivers DOX to cancer patients with the complication of thrombosis with reduced adverse effect. Drug release studies showed double trigger release of DOX from CDs-HA-Hep/DOX system due to acidic environment of the tumor and presence of HA, which was found to be dependent on the concentration of HA and pH value. In vitro MTT and scratch tests results further confirmed the inhibition of growth and migration of cancer cells, respectively. Visual tracking of the drug from the developed delivery system based upon CDs was also feasible (Duan et al., 2020). Chung et al. (2022), synthesized CDs/hydroxyapatite (CD-HAP) nanocomposite as a carrier system for acetaminophen using sugarcane bagasse char as a biowaste precursor via hydrothermal method. The pairing of CDs with hydroxyapatite improved the fluorescence property. The highest drug loading capacity of 48.5% was found in CD-HAP-40, which was synthesized using 40 mL of CDs. However, the best sustained acetaminophen release behavior was seen in CD-HAP-20 synthesized using 20 mL of CD, and the release kinetics followed Higuchi model showing diffusion mechanism of drug release. This study concluded the use of CD-HAP as a potential candidate for drug delivery application (Chung et al., 2020). Rahmani et al. (2021), developed pH-sensitive chitosan nanogel of rivastigmine by incorporating N-CDs. These nanogels exhibited pH-sensitive drug release. Swelling capacity and drug entrapment efficiency also improved by incorporating N-CDs in the nanogel. Many stimuli sensitive polymers exist that show immense potential, but their use is restricted due to their biocompatibility and toxicity related issues and therefore more research is warranted for safe and effective drug delivery using stimuli polymers.
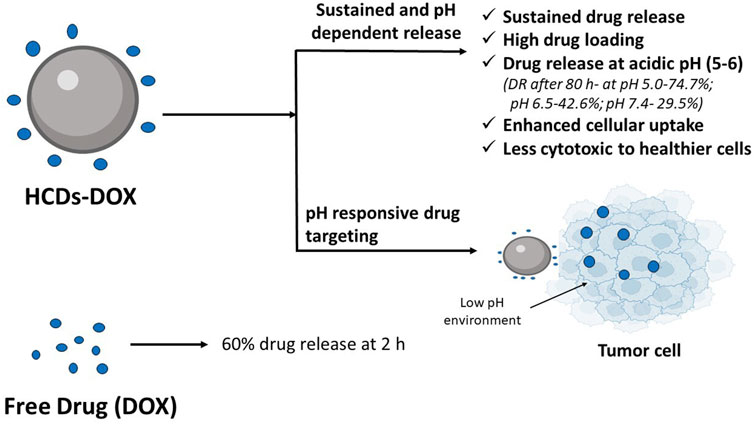
FIGURE 4. Hollow CDs (HCDs) for sustained and pH responsive drug delivery of doxorubicin (DOX). In vitro drug release study showed 74.7% of DOX release from HCDs at pH 5.0, 42.6% at pH 6.5% and 29.5% at pH 7.4 after 80 h. In case of free DOX release profile, 60% of the drug was released after 2 h (Zhang et al., 2019a).
3.7 CDs in vaccine delivery
CDs finds application in field of vaccine delivery and especially in cancer immunotherapy. The performance of therapeutic vaccine in cancer immunotherapy is not promising as it is not able to generate a robust immune response in body’s incapacitated immune system. The tumor associated antigen (TAAs) used in therapeutic vaccine is not able to stimulate the immune response resulting in limited success in developing vaccine for cancer. Ideally, a vaccine drug delivery system should be able to deliver antigen effectively and it should also act as adjuvant. Photoluminescent CDs are reported to have potential application as a vaccine adjuvant. Luo et al. (2018), successfully designed a nanocomposite consisting of uniform-sized CDs and tumor model antigen protein, ovalbumin (OVA). OVA can stimulate the maturation of dendritic cells resulting in augmenting the expression of costimulatory molecules, CD80 and CD86. It further led to the activation of T cells and subsequently their proliferation, vital for killing the cancer cells (Luo et al., 2018). Li et al. (2018), evaluated the efficacy of PEI modified fluorescent CDs as vaccine delivery system via intranasal route. In this study, a model protein antigen, i.e., OVA was delivered through intranasal route using PEI modified CDs as a carrier. Their results showed elevated levels of IgG and IgA titres and increased cytokine IFN-γ secretion by splenocytes, and memory T cells following administration. This study further validated the role of CDs as a carrier of vaccine antigen as well as also helps on trafficking the movement from the administration site to immune organs (Li et al., 2018). In another study, Huang et al. (2020), studied the role of quarternized cationic CDs prepared using biquarternary ammonium salt (BQAS-CDs) as a potent vaccine adjuvant in vivo and a viable alternative to alum in producing both Th1 and Th2 response. It is well known that alum is incapable of producing cellular immune response required for cancer management and microbial infections. In this study, OVA was used as a model antigen that is negatively charged and was adsorbed on cationic BQAS-CDs to form nanocomplex. In vivo studies in mice showed that the nanocomplex triggered Th2 humoral response which was measured by the IgG1 antibodies and was found to be similar to alum. However, the Th1 cellular immune response determined by measuring the IgG2a and IgG2b antibodies was more dominant in group of mice administered with BQAS-CDs + OVA nanocomplex. Furthermore, it was also found that a BQAS-CDs + OVA nanocomplex induce proliferation of OVA-specific CD4+ and CD8+ T cells compared to alum-OVA and naked OVA groups without affecting the hematological and histological parameters. Notably, the results of this study confirmed that BQAS-CDs can serve as a potential adjuvant and a delivery vehicle of antigen (Huang et al., 2020). Cheng et al. (2019), investigated the potential of CQD’s as a nanovehicle for protein antigen gp85. The protein antigen had been found to be effective in producing immune response against avian leukosis virus subgroup J (ALV-J) in chickens. Their study results revealed that the nanocomplex of gp85 with CQD produced high IgG levels and confer long-term protection. The study also confirmed the role of CQD’s as an adjuvant in potentiating the immune response (Cheng et al., 2019). Conclusively, several reports highlight the potential application of CDs as a nanovehicle for vaccine delivery. Furthermore, CDs-mediated vaccine drug delivery system needs to be explored preclinically and clinically to investigate the ability of CDs to induce cellular or humoral immunity.
3.8 Antiviral properties of CDs and as nanocarrier in antiviral drug delivery
The current approach of dealing with the viral infection is to prevent its adherence to host cells or to interfere with its replication. Virus eradication is an enduring challenge now a days and CDs play a vital role due to their antiviral properties. One of the studies conducted by Fahmi et al. (2016), demonstrated the potential of boronic acid modified CQD’s against HIV virus using MOLT-4 cells as a host cell system. The results of this study found that the boronic acid sites on CQD’s could bind to the glycoprotein expressed on HIV virus which is responsible for its adherence to host cells (Fahmi et al., 2016). A similar study was performed on CDs derived from benzoxazine monomer and found to be effective against a variety of viruses such as adenovirus-associated virus, porcine parvovirus, zika virus, dengue virus, and Japanese encephalitis virus. CDs hinders virus attachment to the host cells through the mechanism of binding to proteins present on the virus surface (Kotta et al., 2020).
There are studies that further explore the potential of modified CDs and found that it does not only interfere with the entry of viruses into host cells, but also affects its replication. Garg et al. (2020), found that the antiviral properties of CDs inhibiting the replication of virus can be achieved by doping it with heteroatom. In their study, CDs were doped with triazole derivatives and formed a series of bioisosters. The study results found that the heteroatom co-doped with CQD’s act by targeting the viral enzymes such as helicase and 3CLpro, required for viral replication. Triazaole derivatized CQD’s can be used to combat the SARS-CoV-2 infection, owing to the ability of virus to encode for non-structural proteins including the 3CLpro. Their mode of action is contrary to surface functionalized CQD’s that prevents the entry of virus to host cells (Garg et al., 2020). In another study, Łoczechin et al. (2019), studied the CQD’s potential to inhibit human coronavirus HCoV-229E. CQDs were linked separately with different chemical moieties to form seven different CQD’s and it was found that all of them inhibited the interaction of S protein receptor of HCoV-229E with the host cells. Chemical modification of CQD’s was done by introducing the boronic acid sites on it which resulted in augmentation of its antiviral property by interfering with S protein receptor and thus suppressed the syncytium formation. Preventing the syncytium formation led to prevention of HCoV-229E entry into the host cells. The timing of addition of CQD’s had been found to affect its mechanism of action as the addition of CQD’s after 5.5 h after the virus entered in host cells resulted in inhibition of genomic replication of virus. This could be due to interaction of CQD’s with the cell surface protein affecting the signal transduction and consequently replication or the interaction of CQD’s with the cytosolic proteins of cells after internalization. The antiviral effect of cationic curcumin CDs (CCM-CQD’s) was investigated, and it was found that they not only inhibit the viral entry but also its replication in a porcine epidemic diarrhea virus (PEDV) coronavirus model. The CCM-CQD’s interferes with the surface protein of virus and thereby preventing its entry into host cells. The study results also showed that CCM-CQD’s target viral RNA replication by producing interferon-stimulating genes (ISGs) and pro-inflammatory cytokines. Based on the study results, it was deduced that CCM-CQD’s can be used as alternative treatment strategy for patients infected with coronavirus (Ting et al., 2020).
Aung et al. (2020), developed amino phenylboronic acid-modified CQD’s (APBA-CQD’s) and conjugated it with antiviral drug, Duviral to further enhance its antiviral efficacy against HIV-1 infection. The modification on CDs improved its toxicity profile, biocompatibility, and imparts higher stability. The APBA-CQD’s acts by preventing the entry of virus to host cells by engaging with gp120 expressed on virus and thus prevents the formation of syncytia. Combination of APBA-CQD’s with Duviral, which is a multicomponent drug consisting of Lamivudine and Zidovudine, act by inhibiting the enzyme nucleoside reverse transcriptase and inactivate HIV-1 by intracellular and extracellular blocking. This combination showed better results in targeting virus and thus warrants further investigation as it is expected to improve the survival rate and life expectancy of patients (Aung et al., 2020). Iannazzo et al. (2018), also explored the potential of polycarboxylated graphene quantum dots (GQD’s) as a carrier of two antiviral drugs. The drug molecules chosen for the study were CHI499 and CDF119, which belong to the class of non-nucleoside reverse transcriptase inhibitors (NNRTI) and anchored to GQD’s. Results showed that the conjugate of GQD’s and antiviral drugs showed marked enhancement in antiviral efficacy compared to drug alone. The increased anti-HIV activity of the conjugate was due to dual nature of mechanism achieved by the conjugate. The NNRTI acts by inhibiting the reverse transcriptase and GQD’s acts by inhibiting the binding of virus to host cells (Iannazzo et al., 2018). In another study, Ju et al. (2020), successfully demonstrated the CQD’s-mediated delivery of locked nucleic acid (LNA) against Kaposi’s sarcoma-associated herpesvirus (KSHV) and AIDS related non-Hodgkin B-cell lymphoma. In vitro results showed that the conjugate of CQD-LNA acts by inhibition of proliferation of KSHV-associated primary effusion lymphoma (PEL) cells by inducing apoptosis and knockdown of KSHV micro-RNA’s, such as miR-K12-1, miR-K12-4 and miR-K12-11. The conjugate had proven its efficacy in PEL nurtured xenograft mouse model by causing the regression of tumor growth and thus greatly increasing the survival rate (Ju et al., 2020). On similar lines, a study used antiviral compound glycyrrhizic acid (Gly), a traditional Chinese herbal medicine, as a precursor to synthesize antiviral CQD’s. It was found that Gly-CQD’s inhibited virus proliferation by approximately 5 orders of magnitude. This conjugate also provides the advantage of reduced side effects of glycyrrhizic acid that are more pronounced when given alone. Gly-CQD conjugate had been found to be act in many ways, namely: a) inhibiting virus proliferation; b) inhibiting virus invasion and replication; c) controlling the expression of antiviral genes; d) stimulating the production of interferons; e) regulating the production of virus-induced reactive oxygen species (ROS) (Tong et al., 2020; Xue et al., 2022). Undoubtedly, CDs confer alternative option in dealing with viral infection, however, most of the studies were performed in-vitro and there is a need to do more studies in vivo to validate the efficacy of CDs in the delivery of antiviral drugs. Additionally, CDs must be hydrophilic and exhibit colloidal stability in physiological conditions. A profound understanding of the structure activity relationship in terms of size, shape, surface charge, etc., to increase the efficacy of CDs as carrier of antiviral agents is required as well. Moreover, more research in developing CDs is required to combat virus mutation and the emergence of new strains.
4 Diagnostic applications of CDs
As a captivating part of fluorescent carbon nanomaterials, CDs demonstrates significant potential in bioimaging and biosensing field owing to their small size, better aqueous stability, excellent photostability and biocompatibility (Kong et al., 2020).
4.1 Bioimaging
Bioimaging enables visualization of cell and its orgnanelles that allows better understanding of their structure and physiological functions. In the past decade, the use of green fluorescent protein (GFP) in biomedical research allowed monitoring of interaction among proteins and gene expression. However, poor photostability and weak fluorescence of GFP restricted its further widespread utility. The discovery of fluorescent CDs possessed unique properties such as multicolor emission with high QY, tunable optical properties, excellent photostability and dispersion properties. CD-based optical imaging utilizes optical contrast between the image and surrounding region and enables early detection, screening, and diagnosis of life threatening disease conditions. The imaging capabilities of CDs is either due to its unique optical property, i.e., intrinsic fluorescence or due to the presence of functionality in its core or surface (Wang B. et al., 2022).
Herein, we have discussed the research outcomes of the various studies conducted using fluorescent CD probes. Hua et al. (2019) prepared red emissive, nucleolus targeted Ni–pPCDs using p-phenylenediame (pPDA) and nickel ions (Ni2+) to achieve the maximum quantum yield (QY) with excellent photostability. In vitro and in vivo studies revealed the biocompatibility of Ni–pPCDs and their utilization for high-resolution imaging of cell nuclei and high-contrast imaging of tumor-bearing mice and zebrafish (Hua et al., 2019). Zhang et al. (2019b), prepared N-CDs, a kind of green and red emissive CDs, that were loaded with multifunctional Mn2+ complex-modified polydopamine (PDA) nanoparticles (NPs) for fluorescent imaging. PDA-N-CDs (Mn) NPs can provide contrast for magnetic resonance imaging (MR) due to the automated chelation of Mn2+ ions. It was possible to effectively show the use of PDA-N-CDs (Mn) NPs for fluorescence, photothermal, and MR in vivo imaging. Furthermore, the PDA-N-CDs (Mn) NPs exhibited outstanding biocompatibility and minimal biological toxicity, as evidenced by cytotoxicity testing, hemolytic testing, histological analysis of viscera sections, and blood biochemical analysis (Zhang et al., 2019b). Mintz et al. (2021) showed that CDs are capable of quantitatively detecting water in organic solvents. Also, transferrin conjugation enhanced the CDs biocompatibility. These were used for cellular imaging of neuroblastoma cell lines (Mintz et al., 2022). Ren et al. (2020) developed blue fluorescent N-CDs using microwave-assisted method for cell imaging and metronidazole detection. Kong et al. (2020) developed an effective nanosystem based on the DNA aptamer AS1411 modified CDs with PEI as connecting bridge for bioimaging. Confocal microscopy and flow cytometry displayed higher cellular uptake of the CDs-PEI-AS1411 in MCF-7 breast cancer cells as compared to non-cancerous L929 fibroblast cells, thereby showing very specific capacity to identify nucleolin-positive cells. Results showed the application of synthesized CDs-PEI-AS1411 nanosystem in cancer cell targeted imaging (Kong et al., 2020). Gudimella et al. (2021) reported a sand bath assisted method for the preparation of fluorescent CDs using peels of citrus fruit. Prepared CDs revealed biocompatibility, low toxicity and significant free radical scavenging activity. Folic acid was conjugated with the CDs to enhance its potential as biological labels for cellular imaging at multiple excitations (Gudimella et al., 2021). CDs with protein like structure were created with the combination of fluorescent blinking domains and RNA-binding motifs, allowing for better nucleolar ultrastructure imaging. The picture enables precise differentiation of distinct cells from the same cell type by extracting multidimensional information from the nucleolus. Furthermore, it was shown that this CD-depicted nucleolar ultrastructure can be used as a sensitive hallmark to recognize and distinguish subtle responses to several stressors, as well as to provide RNA-related information that has previously been unavailable using traditional immunofluorescence methods. This protein-mimicking CDs might be used to investigate nucleolar stress in cell diagnostics and therapies (He et al., 2021). Ermis et al. (2022) also reported red emissive biocompatible CDs (R-CDs) based fluorescent probe for live 3D bioimaging of U-87 microglia tumor spheroids.
CDs exhibiting fluorescence in NIR region (700–1700 nm) are also gaining interest because of there application as nanomaterials in bioimaging. They are reported to be highly efficient in photothermal therapy. CDs exhibiting fluorescence in NIR window has a distinct advantage over other photoluminescent agents in high-contrast in vivo fluorescence imaging owing to its deeper tissue penetration, high resolution, lower tissue self-absorption/scattering, and less autofluorescence (Wang T. et al., 2021; Zhao et al., 2023). Bao et al. (2018) developed sulfur and nitrogen codoped NIR CDs for in vivo fluorescence and photoacoustic imaging with high photothermal conversion efficiency of 59%. Ding et al. (2019) synthesised highly fluorescent CDs emitting near infrared from lemon juice for potential bioimaging application. QY was reported to be 31%. Liu et al. (2022) fabricated large conjugated perylene derivates for synthesising CDs which exhibited high contrast in vivo NIR fluorescence bioimaging in mice model. Zhao et al. (2023) demonstrated application of CDs with NIR-I (700–950 nm) emission and NIR-II (1000–1700 nm) absorption in multiple photon bioimaging and photothermal therapy. Multiphoton-induced NIR-I emission with quantum yield of 29% and NIR-II induced heat with photothermal conversion efficiency of 41.19% was reported (Zhao et al., 2023). Overall, CDs exhibiting photoluminescence in the NIR region are reported to be of potential application in the field of bioimaging. However, synthesis of CDs exhibiting fluorescence in NIR region with higher QY is a major challenge.
Imaging using CDs emitting blue/green light of shorter wavelength have limitation of background interference and poor penetration in the biological tissues. Therefore, it is desired to use CDs that can emit in the NIR region while enabling good penetration of light in the biological tissue with minimal photodamage. Various studies have been done on different cell lines, mice and zebra fish have reported excellent biocompatibility of CDs, but more studies needs to be done at clinical level to ensure the safety of CDs. To gain more confidence in the use of CDs at clinical level, studies related to mechanism of interaction of CDs with biological system warrants further exploration.
4.2 Biological sensing
Biological sensing refers to identification and measuring the analytes concentration based on properties such as photoluminescence and electrical conductivity. The CDs are endowed with both of them and upon interaction with analytes lead to change in the optical emission characteristics such as change in fluorescence intensity or colorimetric wavelength. Based on these properties, CDs are further classified into three categories, i.e., on-off, off-on, and fluorescence shift. The on-off category is also called fluorescence quenching and used for the detection of cations, anions, and small molecules. In this, upon interaction of CDs with metal ion, the electron are transferred from CDs to empty d-orbital of metal ions resulting in fluorescence quenching. In the case of off-on category, the CDs regain its fluorescence and reach back to emission state upon interaction with the analyte. And lastly fluorescence shift category detects analytes based on partial overlap of donor emission and acceptor absorption spectra (Wang B. et al., 2022).
Herein, we have discussed the snapshot of various studies conducted by researchers on biosensing analytes using CDs. Zhu et al. (2019), prepared titanium carbides (Ti3C2) nanosheets mixed with red-emitting CDs (RCDs), where Ti3C2 showed selective and effective fluorescent turn-on nano sensor for glucose detection. The nanosensor can also be used to monitor glucose by using H2O2 produced by the oxidation of glucose catalysed by glucose oxidase (Zhu et al., 2019). For calcium ions detection, novel fluorescent CDs were developed in which the surface of the CDs was modified by ethylene-bis (oxyethylenenitrilo) tetra acetic acid (EGTA). The CDs emit bright blue fluorescence, and the intensity of the fluorescence declines significantly as the calcium concentration increases. The findings proved that calcium ion detection by CDs is a static fluorescence quenching mechanism. Also, cytotoxicity and cellular imaging investigations have demonstrated nontoxic and biocompatibility profile of CDs (Yue et al., 2019). Yu et al. (2019), prepared N-CDs which were extremely dispersible in water and can be utilised as an optical probe for label-free detection of Fe3+ via a switched off change. Also, in the presence of pyrophosphate, such sensing nanoplatforms can be restored. This on-off-on technique is likely to open up new opportunities for producing efficient sensors in industrial settings (Yu et al., 2019). A selective and environmentally friendly fluorescence immunoassay approach based on N-CDs for the exposure of nuclear matrix protein 22 (NMP22, antigen), was developed by Othman et al. (2020). The immunocomplex on the carboxylated N-CDs caused fluorescence intensity quenching. NMP22 was effectively detected in human urine samples using this approach, with recoveries ranging from 96.50% to 103.61%. These findings give substantial evidence that N-CDs can be used as fluorescent labels in immunoassays. Geng et al. (2020), reported a lysosome-specific fluorescent CDs for ATP detection in acidic lysosomes with “off-on” changes of yellow fluorescence. These CDs were effectively used for real-time monitoring of lysosomal ATP shifting concentration caused due to drug stimulation (e.g., chloroquine, etoposide, and oligomycin) (Geng et al., 2020). Orange–red emissive CDs (OR-CDs) were developed by calcining carbon sources like 5-amino-1, 10-phenanthroline (Aphen), and salicylic acid (SA), which worked as a “turn on” type fluorescence probe for Cd2+ detection. OR-CDs showed potential to reduce cadmium poisoning in living organisms in addition to being employed for intracellular Cd2+ imaging (Xu et al., 2022). Chattopadhyay et al. (2021), prepared CDs from peptic digest of animal tissue, which is easily available, without using any other chemical reagent for surface functionalization. The utilisation of these CDs as a fluorescent nanoprobe for the detection of metal ions (Cu2+ and Hg2+) via chelation, radical scavenging, and cellular imaging has been examined. They also displayed fast cell exocytosis, indicating that they have a lot of potential for chelation treatment. For fluorescence-based cell imaging, they can also be utilised as an alternative to synthetic organic dyes (Chattopadhyay et al., 2021). Tang et al. (2021), developed CDs using black pepper as raw material and designed them as NIR ratiometric nanoprobe for ascorbic acid measurement based on off-on mode with good selectivity and sensitivity. The suggested technique has enormous potential to increase the applications of dual-emission CDs in biological disciplines (Tang et al., 2021). Rajendran et al. (2021), reported green fluorescent CDs with quantum yield of up to 61% for tetracycline detection and mitochondrial labelling in cancer cells. CDs derived from coconut husk with good quantum yield and biocompatibility were synthesized by Chauhan et al. (2022), for sensing amino acid, L-tyrosine. Mathad et al. (2022), developed β-Cyclodextrin (CD) anchored neem (Azadirachta indica) CDs for greater electrochemical sensing performance of lapatinib, an anticancer drug, via host-guest inclusion. The proposed sensor could be used for determining lapatinib in pharmaceutical formulations and urine samples (Mathad et al., 2022). Blue fluorescent CDs conjugated with thiomalic acid (TMA)-capped AgInS2 quantum dots were developed and evaluated for determination of ibandronic acid, a bisphosphonate pharmaceutical, by Castro et al. (2022). Sohail et al. (2022), investigated Zn-doped CDs-based bioanalytical probe for the determination of antioxidant activity index (AAI) as traditional techniques lack truncated strength, high interference effect in complex samples, photo-bleaching and stability. Tang et al. (2022), synthesised nitrogen-doped fluorescent carbon dots from grape peel biowaste for detection of baicalin, a flavonoid compound used for liver cancer treatment. Further advancement in technology led to rapid detection of analytes via smartphones due to their ease of portability and accessibility. Su et al. (2020) developed light sensing CDs and integrate with smartphones that allows rapid detection of 2,4-dichlorophenoxyacetic acid in real time. Currently, there is limited application of this technology and more work is needed in the area to expand this technology for the detection of analytes in other disease conditions. Recently reported diagnostic applications of CDs are summarized in Table 3.
5 Miscellaneous applications of CDs
Some other miscellaneous applications of CDs are reported in the literature, such as Kandra and Bajpai (2020), reported that impregnation of CDs into chitosan film caused seven-fold reduction in its water absorption capacity. Moisture permeation abilities of the plain chitosan and CDs loaded chitosan film matrix was found to be 1758 and 956 g/m2/day, respectively. Additionally, for chitosan film, bovine serum albumin (BSA) adsorption was found to be 24.2 mg/m2 which got reduced to 14.1 mg/m2 for CDs loaded chitosan film matrix. (Kandra and Bajpai, 2020). Lee et al. (2020), developed a CDs nanoparticle to investigate their potential as a new antiplatelet agent for arterial thrombotic diseases treatment without causing any cytotoxicity. Antiplatelet action was exerted due to the inhibition of collagen-stimulated human platelet aggregation. In vivo study showed reduced survival in mice with ADP-induced acute pulmonary thromboembolism without altering the bleeding time, which is the foremost adverse effect of antiplatelet agents (Lee et al., 2020). Zhao et al. (2022), evaluated Armeniacae Semen Amarum (ASA)-Carbonisata-derived CDs (ASAC-CDs) for its anti-inflammatory effects in rat model of acute lung injury (ALI). ASAC-CDs reduced inflammation by reducing the level of inflammatory mediators. CDs also showed antioxidant activity by reducing malondialdehyde (MDA), myeloperoxidase (MPO) content and increasing superoxide dismutase (SOD) activity and glutathione (GSH) content. Results demonstrated the potential application of developed system in clinical anti-pneumonia (Zhao et al., 2022). Gudimella et al. (2022), prepared fluorescent CDs from Carica papaya leaves which were examined for free radical scavenging activity, antioxidant activity and in vitro anti-inflammatory activity.
6 Conclusion and future perspectives
CDs are emerging nanoparticles due to various advantages, such as high photostability, less cytotoxicity, biocompatibility, high chemical inertness, eco-friendliness, nontoxicity, easy functionalization, non-blinking photoluminescence, and good water solubility. CDs demonstrates potential drug delivery applications as nanomaterial for treating cancer, brain, ocular and infectious diseases. They also have application in gene, vaccine and antiviral drug delivery. Due to their versatile physicochemical properties, CDs are capable of achieving sustained release action, pH responsive drug delivery, smart stimuli-responsive and targeted drug delivery. Although CDs based nanomaterials are found to be promising nanocarriers in diagnostics and therapeutics drug delivery, further advances are still desirable to enhance their performance, sustainability, durability and cost-effectiveness for efficient industrial scale-up. Although, numerous cell lines and in vivo cytotoxicity studies have reported that the CDs based nanomaterials did not induce any toxic effects, more detailed investigations are still required to explain their toxicity profiles and biodegradation mechanism for efficient drug delivery application. CDs needs to be explored for preclinical long-term toxicity before proceeding to their clinical applicability.
Author contributions
Conceptualization, HK and DV; methodology, HK and DV; resources, HK, DV, and DK; data curation, HK and DV; writing—original draft preparation, HK and DV; writing—review and editing, HK, AB, and SS; supervision, HK, AB, and SS; project administration, HK. All authors contributed to the article and approved the submitted version.
Acknowledgments
The authors would like to thank Shoolini University of Biotechnology and Management Sciences for constant support and guidance. The authors would also like to thank Larkin University for the funds granted for the publication of this manuscript.
Conflict of interest
Author AB was employed by Perrigo Company Plc.
The remaining authors declare that the research was conducted in the absence of any commercial or financial relationships that could be construed as a potential conflict of interest.
Publisher’s note
All claims expressed in this article are solely those of the authors and do not necessarily represent those of their affiliated organizations, or those of the publisher, the editors and the reviewers. Any product that may be evaluated in this article, or claim that may be made by its manufacturer, is not guaranteed or endorsed by the publisher.
References
Adrita, S. H., Tasnim, K. N., Ryu, J. H., and Sharker, S. M. (2020). Nanotheranostic carbon dots as an emerging platform for cancer therapy. J. Nanotheranostics 1 (1), 58–77. doi:10.3390/jnt1010006
Ahuja, V., Banerjee, S., Roy, P., and Bhatt, A. K. J. B. (2021). Fluorescent xylitol carbon dots: A potent antimicrobial agent and drug carrier. Biotechnol. Appl. Biochem. 69, 1679–1689. doi:10.1002/bab.2237
An, Y., Lin, X., Zhou, Y., Li, Y., Zheng, Y., Wu, C., et al. (2021). Red, green, and blue light-emitting carbon dots prepared from o-phenylenediamine. RSC Adv. 11 (43), 26915–26919. doi:10.1039/d1ra02298a
Arsalani, N., Nezhad-Mokhtari, P., and Jabbari, E. J. A. (2019). Microwave-assisted and one-step synthesis of PEG passivated fluorescent carbon dots from gelatin as an efficient nanocarrier for methotrexate delivery. Artif. cells, nanomedicine, Biotechnol. 47 (1), 540–547. doi:10.1080/21691401.2018.1562460
Aung, Y. Y., Kristanti, A. N., Khairunisa, S. Q., Nasronudin, N., and Fahmi, M. Z. (2020). Inactivation of HIV-1 infection through integrative blocking with amino phenylboronic acid attributed carbon dots. ACS Biomater. Sci. Eng. 6 (8), 4490–4501. doi:10.1021/acsbiomaterials.0c00508
Bao, X., Yuan, Y., Chen, J., Zhang, B., Li, D., Zhou, D., et al. (2018). In vivo theranostics with near-infrared-emitting carbon dots-highly efficient photothermal therapy based on passive targeting after intravenous administration. Light Sci. Appl. 7, 91. doi:10.1038/s41377-018-0090-1
Biswal, M. R., and Bhatia, S. (2021). Carbon dot nanoparticles: Exploring the potential use for gene delivery in ophthalmic diseases. Nanomater. (Basel). 11 (4), 935. doi:10.3390/nano11040935
Boobalan, T., Sethupathi, M., Sengottuvelan, N., Kumar, P., Balaji, P., Gulyás, B., et al. (2020). Mushroom-derived carbon dots for toxic metal ion detection and as antibacterial and anticancer agents. ACS Appl. Nano Mater. 3 (6), 5910–5919. doi:10.1021/acsanm.0c01058
Carbonaro, C. M., Corpino, R., Salis, M., Mocci, F., Thakkar, S. V., Olla, C., et al. (2019). On the emission properties of carbon dots: Reviewing data and discussing models. C 5 (4), 60. doi:10.3390/c5040060
Castro, R. C., Páscoa, R. N., Saraiva, M. L. M., Santos, J. L., and Ribeiro, D. S. J. S. A. P. A. M. (2022). Photoluminescent and visual determination of ibandronic acid using a carbon dots/AgInS2 quantum dots ratiometric sensing platform. Spectrochim. Acta A Mol. Biomol. Spectrosc. 267, 120592. doi:10.1016/j.saa.2021.120592
Chattopadhyay, S., Mehrotra, N., Jain, S., and Singh, H. J. M. J. (2021). Development of novel blue emissive carbon dots for sensitive detection of dual metal ions and their potential applications in bioimaging and chelation therapy. Microchem. J. 170, 106706. doi:10.1016/j.microc.2021.106706
Chauhan, P., Mundekkad, D., Mukherjee, A., Chaudhary, S., Umar, A., and Baskoutas, S. J. N. (2022). Coconut carbon dots: Progressive large-scale synthesis, detailed biological activities and smart sensing aptitudes towards tyrosine. Nanomaterials 12 (1), 162. doi:10.3390/nano12010162
Chen, P., Zhang, J., He, X., Liu, Y. H., and Yu, X. Q. (2020). Hydrophobically modified carbon dots as a multifunctional platform for serum-resistant gene delivery and cell imaging. Biomater. Sci. 8 (13), 3730–3740. doi:10.1039/d0bm00651c
Chen, T., Yao, T., Peng, H., Whittaker, A. K., Li, Y., Zhu, S., et al. (2021b). An injectable hydrogel for simultaneous photothermal therapy and photodynamic therapy with ultrahigh efficiency based on carbon dots and modified cellulose nanocrystals. Adv. Funct. Mater 31 (45), 2106079. doi:10.1002/adfm.202106079
Chen, W., Shen, J., Wang, Z., Liu, X., Xu, Y., Zhao, H., et al. (2021a). Turning waste into wealth: Facile and green synthesis of carbon nanodots from pollutants and applications to bioimaging. Chem. Sci. 12 (35), 11722–11729. doi:10.1039/d1sc02837e
Cheng, J., Xu, Y., Zhou, D., Liu, K., Geng, N., Lu, J., et al. (2019). Novel carbon quantum dots can serve as an excellent adjuvant for the gp85 protein vaccine against avian leukosis virus subgroup J in chickens. Poult. Sci. 98 (11), 5315–5320. doi:10.3382/ps/pez313
Chu, X., Wu, F., Sun, B., Zhang, M., Song, S., Zhang, P., et al. (2020). Genipin cross-linked carbon dots for antimicrobial, bioimaging and bacterial discrimination. Colloids Surfaces B Biointerfaces. 190, 110930. doi:10.1016/j.colsurfb.2020.110930
Chung, H. K., Wongso, V., Sambudi, G. S., and Isnaeni, N. S. (2020). Biowaste-derived carbon dots/hydroxyapatite nanocomposite as drug delivery vehicle for acetaminophen. J. Sol-Gel Sci. Technol. 93 (1), 214–223. doi:10.1007/s10971-019-05141-w
Cui, F., Ye, Y., Ping, J., and Sun, X. J. B. (2020). Carbon dots: Current advances in pathogenic bacteria monitoring and prospect applications. Biosens. Bioelectron. 156, 112085. doi:10.1016/j.bios.2020.112085
Cutrim, E. S., Vale, A. A., Manzani, D., Barud, H. S., Rodriguez-Castellon, E., Santos, A. P., et al. (2021). Preparation, characterization and in vitro anticancer performance of nanoconjugate based on carbon quantum dots and 5-Fluorouracil. Mater Sci. Eng. C Mater Biol. Appl. 120, 111781. doi:10.1016/j.msec.2020.111781
Das, P., Ganguly, S., Agarwal, T., Maity, P., Ghosh, S., Choudhary, S., et al. (2019). Heteroatom doped blue luminescent carbon dots as a nano-probe for targeted cell labeling and anticancer drug delivery vehicle. Mater. Chem. Phys. 237, 121860. doi:10.1016/j.matchemphys.2019.121860
Das, P., Maruthapandi, M., Saravanan, A., Natan, M., Jacobi, G., Banin, E., et al. (2020). Carbon dots for heavy-metal sensing, pH-sensitive cargo delivery, and antibacterial applications. ACS Appl. Nano Mater. 3 (12), 11777–11790. doi:10.1021/acsanm.0c02305
Demirci, S., McNally, A. B., Ayyala, R. S., Lawson, L. B., and Sahiner, N. (2020). Synthesis and characterization of nitrogen-doped carbon dots as fluorescent nanoprobes with antimicrobial properties and skin permeability. J. Drug Deliv. Sci. Technol. 59, 101889. doi:10.1016/j.jddst.2020.101889
Ding, H., Zhou, X., Qin, B., Zhou, Z., and Zhao, Y. (2019). Highly fluorescent near-infrared emitting carbon dots derived from lemon juice and its bioimaging application. J. Luminescence 211, 298–304. doi:10.1016/j.jlumin.2019.03.064
Dong, X. (2018). Current strategies for brain drug delivery. Theranostics 8 (6), 1481–1493. doi:10.7150/thno.21254
Dong, X., Liang, W., Meziani, M. J., Sun, Y-P., and Yang, L. J. T. (2020). Carbon dots as potent antimicrobial agents. Theranostics 10 (2), 671–686. doi:10.7150/thno.39863
Duan, Q., Ma, L., Zhang, B., Zhang, Y., Li, X., Wang, T., et al. (2020). Construction and application of targeted drug delivery system based on hyaluronic acid and heparin functionalised carbon dots. Colloids Surf. B Biointerfaces 188, 110768. doi:10.1016/j.colsurfb.2019.110768
El-Shafey, A. M. (2021). Carbon dots: Discovery, structure, fluorescent properties, and applications. Green Process. Synthesis 10 (1), 134–156. doi:10.1515/gps-2021-0006
Ermis, E., Bagheri, Z., Behroodi, E., Latifi, H., Rahimifard, M., and Ajorlou, E. (2022). Red emissive N–S co-doped carbon dots for live imaging of tumor spheroid in the microfluidic device. J. Sci. Adv. Mater. Devices 7 (2), 100404. doi:10.1016/j.jsamd.2021.11.006
Fahmi, M. Z., Sholihah, N. F., Wibrianto, A., Sakti, S. C. W., Firdaus, F., and Chang, J. Y. (2021). Simple and fast design of folic acid-based carbon dots as theranostic agent and its drug release aspect. Mater. Chem. Phys. 267, 124596. doi:10.1016/j.matchemphys.2021.124596
Fahmi, M. Z., Sukmayani, W., Khairunisa, S. Q., Witaningrum, A. M., Indriati, D. W., Matondang, M. Q. Y., et al. (2016). Design of boronic acid-attributed carbon dots on inhibits HIV-1 entry. RSC Adv. 6 (95), 92996–93002. doi:10.1039/c6ra21062g
Garg, P., Sangam, S., Kochhar, D., Pahari, S., Kar, C., and Mukherjee, M. (2020). Exploring the role of triazole functionalized heteroatom co-doped carbon quantum dots against human coronaviruses. Nano Today 35, 101001. doi:10.1016/j.nantod.2020.101001
Garner, I., Vichare, R., Paulson, R., Appavu, R., Panguluri, S. K., Tzekov, R., et al. (2020). Carbon dots fabrication: Ocular imaging and therapeutic potential. Front. Bioeng. Biotechnol. 8, 573407. doi:10.3389/fbioe.2020.573407
Geng, X., Sun, Y., Guo, Y., Zhao, Y., Zhang, K., Xiao, L., et al. (2020). Fluorescent carbon dots for in situ monitoring of lysosomal ATP levels. Anal. Chem. 92 (11), 7940–7946. doi:10.1021/acs.analchem.0c01335
Ghirardello, M., Ramos-Soriano, J., and Galan, M. C. J. N. (2021). Carbon dots as an emergent class of antimicrobial agents. Nanomaterials 11 (8), 1877. doi:10.3390/nano11081877
Gudimella, K. K., Appidi, T., Wu, H-F., Battula, V., Jogdand, A., Rengan, A. K., et al. (2021). Sand bath assisted green synthesis of carbon dots from citrus fruit peels for free radical scavenging and cell imaging. Colloids Surf. B Biointerfaces 197, 111362. doi:10.1016/j.colsurfb.2020.111362
Gudimella, K. K., Gedda, G., Kumar, P. S., Babu, B. K., Yamajala, B., Rao, B. V., et al. (2022). Novel synthesis of fluorescent carbon dots from bio-based Carica Papaya Leaves: Optical and structural properties with antioxidant and anti-inflammatory activities. Environ. Res. 204, 111854. doi:10.1016/j.envres.2021.111854
Hailing, Y., Xiufang, L., Lili, W., Baoqiang, L., Kaichen, H., Yongquan, H., et al. (2020). Doxorubicin-loaded fluorescent carbon dots with PEI passivation as a drug delivery system for cancer therapy. Nanoscale 12 (33), 17222–17237. doi:10.1039/d0nr01236j
He, H., Chen, X., Feng, Z., Liu, L., Wang, Q., and Bi, S. (2021). Nanoscopic imaging of nucleolar stress enabled by protein-mimicking carbon dots. Nano Lett. 21 (13), 5689–5696. doi:10.1021/acs.nanolett.1c01420
He, X., Chen, P., Zhang, J., Luo, T. Y., Wang, H. J., Liu, Y. H., et al. (2019b). Cationic polymer-derived carbon dots for enhanced gene delivery and cell imaging. Biomater. Sci. 7 (5), 1940–1948. doi:10.1039/c8bm01578c
He, X., Luo, Q., Zhang, J., Chen, P., Wang, H-J., Luo, K., et al. (2019a). Gadolinium-doped carbon dots as nano-theranostic agents for MR/FL diagnosis and gene delivery. Nanoscale 11 (27), 12973–12982. doi:10.1039/c9nr03988k
Hettiarachchi, S. D., Graham, R. M., Mintz, K. J., Zhou, Y., Vanni, S., Peng, Z., et al. (2019). Triple conjugated carbon dots as a nano-drug delivery model for glioblastoma brain tumors. Nanoscale 11 (13), 6192–6205. doi:10.1039/c8nr08970a
Hsu, P-C., Chen, P-C., Ou, C-M., Chang, H-Y., and Chang, H-T. (2013). Extremely high inhibition activity of photoluminescent carbon nanodots toward cancer cells. J. Mater. Chem. B 1 (13), 1774–1781. doi:10.1039/c3tb00545c
Hu, X., Wang, S., Luo, Q., Ge, B., Cheng, Q., Dong, C., et al. (2021). Synthesis of Sn nanocluster@ carbon dots for photodynamic therapy application. J. Chin. Chem. Lett. 32 (7), 2287–2291. doi:10.1016/j.cclet.2021.01.039
Hua, X-W., Bao, Y-W., Zeng, J., and Wu, F-G. (2019). Nucleolus-targeted red emissive carbon dots with polarity-sensitive and excitation-independent fluorescence emission: High-resolution cell imaging and in vivo tracking. ACS Appl. Mater Interfaces 11 (36), 32647–32658. doi:10.1021/acsami.9b09590
Huang, S., Li, B., Ashraf, U., Li, Q., Lu, X., Gao, X., et al. (2020). Quaternized cationic carbon dots as antigen delivery systems for improving humoral and cellular immune responses. ACS Appl. Nano Mater 3 (9), 9449–9461. doi:10.1021/acsanm.0c02062
Iannazzo, D., Pistone, A., Ferro, S., De Luca, L., Monforte, A. M., Romeo, R., et al. (2018). Graphene quantum dots based systems as HIV inhibitors. J. Bioconjugate Chem. 29 (9), 3084–3093. doi:10.1021/acs.bioconjchem.8b00448
Indermun, S., Govender, M., Kumar, P., Choonara, Y. E., and Pillay, V. (2018). Stimuli-responsive polymers as smart drug delivery systems: Classifications based on carrier type and triggered-release mechanism. Stimuli Responsive Polym. Nanocarriers Drug Deliv. Appl. 1, 43–58. doi:10.1016/B978-0-08-101997-9.00002-3
Jia, Q., Zhao, Z., Liang, K., Nan, F., Li, Y., Wang, J., et al. (2020). Recent advances and prospects of carbon dots in cancer nanotheranostics. Mater. Chem. Front. 4 (2), 449–471. doi:10.1039/c9qm00667b
Jian, H-J., Wu, R-S., Lin, T-Y., Li, Y-J., Lin, H-J., Harroun, S. G., et al. (2017). Super-cationic carbon quantum dots synthesized from spermidine as an eye drop formulation for topical treatment of bacterial keratitis. ACS Nano 11 (7), 6703–6716. doi:10.1021/acsnano.7b01023
John, T. S., Yadav, P. K., Kumar, D., Singh, S. K., and Hasan, S. H. (2020). Highly fluorescent carbon dots from wheat bran as a novel drug delivery system for bacterial inhibition. Luminescence 35 (6), 913–923. doi:10.1002/bio.3801
Ju, E., Li, T., Liu, Z., da Silva, S. R., Wei, S., Zhang, X., et al. (2020). Specific inhibition of viral MicroRNAs by carbon dots-mediated delivery of locked nucleic acids for therapy of virus-induced cancer. J. ACS Nano 14 (1), 476–487. doi:10.1021/acsnano.9b06333
Kandra, R., and Bajpai, S. (2020). Synthesis, mechanical properties of fluorescent carbon dots loaded nanocomposites chitosan film for wound healing and drug delivery. Arabian J. Chem. 13 (4), 4882–4894. doi:10.1016/j.arabjc.2019.12.010
Kirbas Cilingir, E., Seven, E. S., Zhou, Y., Walters, B. M., Mintz, K. J., Pandey, R. R., et al. (2021). Metformin derived carbon dots: Highly biocompatible fluorescent nanomaterials as mitochondrial targeting and blood-brain barrier penetrating biomarkers. J. Colloid Interface Sci. 592, 485–497. doi:10.1016/j.jcis.2021.02.058
Kong, T., Zhou, R., Zhang, Y., Hao, L., Cai, X., and Zhu, B. (2020). AS1411 aptamer modified carbon dots via polyethylenimine-assisted strategy for efficient targeted cancer cell imaging. Cell Prolif. 53 (1), e12713. doi:10.1111/cpr.12713
Kotta, S., Aldawsari, H. M., Badr-Eldin, S. M., Alhakamy, N. A., Shadab, M., Nair, A. B., et al. (2020). Exploring the potential of carbon dots to combat COVID-19. Front. Mol. Biosci. 7, 616575. doi:10.3389/fmolb.2020.616575
Koulivand, H., Shahbazi, A., Vatanpour, V., and Rahmandoost, M. (2020). Novel antifouling and antibacterial polyethersulfone membrane prepared by embedding nitrogen-doped carbon dots for efficient salt and dye rejection. Mater Sci. Eng. C Mater Biol. Appl. 111, 110787. doi:10.1016/j.msec.2020.110787
Kung, J-C., Tseng, I-T., Chien, C-S., Lin, S-H., Wang, C-C., and Shih, C-J. (2020). Microwave assisted synthesis of negative-charge carbon dots with potential antibacterial activity against multi-drug resistant bacteria. RSC Adv. 10 (67), 41202–41208. doi:10.1039/d0ra07106d
Lategan, K., Fowler, J., Bayati, M., Fidalgo de Cortalezzi, M., and Pool, E. (2018). The effects of carbon dots on immune system biomarkers, using the murine macrophage cell line RAW 264.7 and human whole blood cell cultures. Nanomater. (Basel) 8 (6), 388. doi:10.3390/nano8060388
Lee, T-Y., Jayakumar, T., Thanasekaran, P., Lin, K-C., Chen, H-M., Veerakumar, P., et al. (2020). Carbon dot nanoparticles exert inhibitory effects on human platelets and reduce mortality in mice with acute pulmonary thromboembolism. Nanomaterials 10 (7), 1254. doi:10.3390/nano10071254
Li, C-L., Ou, C-M., Huang, C-C., Wu, W-C., Chen, Y-P., Lin, T-E., et al. (2014). Carbon dots prepared from ginger exhibiting efficient inhibition of human hepatocellular carcinoma cells. J. Mater. Chem. 2 (28), 4564–4571. doi:10.1039/c4tb00216d
Li, J., Kong, J., Ma, S., Li, J., Mao, M., Chen, K., et al. (2021). Exosome-Coated 10B carbon dots for precise boron neutron capture therapy in a mouse model of glioma in situ. Adv. Funct. Mater. 31 (24), 2100969. doi:10.1002/adfm.202100969
Li, R. S., Gao, P. F., Zhang, H. Z., Zheng, L. L., Li, C. M., Wang, J., et al. (2017). Chiral nanoprobes for targeting and long-term imaging of the Golgi apparatus. Chem. Sci. 8 (10), 6829–6835. doi:10.1039/c7sc01316g
Li, S., Guo, Z., Zeng, G., Zhang, Y., Xue, W., and Liu, Z. (2018). Polyethylenimine-modified fluorescent carbon dots as vaccine delivery system for intranasal immunization. ACS Biomater. Sci. Eng. 4 (1), 142–150. doi:10.1021/acsbiomaterials.7b00370
Li, X., Hu, S., Lin, Z., Yi, J., Liu, X., Tang, X., et al. (2020a). Dual-responsive mesoporous silica nanoparticles coated with carbon dots and polymers for drug encapsulation and delivery. Nanomedicine 15 (25), 2447–2458. doi:10.2217/nnm-2019-0440
Li, X., Vinothini, K., Ramesh, T., Rajan, M., and Ramu, A. (2020b). Combined photodynamic-chemotherapy investigation of cancer cells using carbon quantum dot-based drug carrier system. Drug Deliv. 27 (1), 791–804. doi:10.1080/10717544.2020.1765431
Li, Y., Wu, S., Zhang, J., Zhou, R., and Cai, X. (2020c). Sulphur doped carbon dots enhance photodynamic therapy via PI3K/Akt signalling pathway. Cell Prolif. 53 (5), e12821. doi:10.1111/cpr.12821
Liang, J., Li, W., Chen, J., Huang, X., Liu, Y., Zhang, X., et al. (2021). Antibacterial activity and synergetic mechanism of carbon dots against gram-positive and-negative bacteria. ACS Appl. Bio Mater. 4 (9), 6937–6945. doi:10.1021/acsabm.1c00618
Lim, S. Y., Shen, W., and Gao, Z. (2015). Carbon quantum dots and their applications. Chem. Soc. Rev. 44 (1), 362–381. doi:10.1039/c4cs00269e
Liu, J., Li, R., and Yang, B. (2020). Carbon dots: A new type of carbon-based nanomaterial with wide applications. ACS Cent. Sci. 6 (12), 2179–2195. doi:10.1021/acscentsci.0c01306
Liu, X., Liu, Y., Thakor, A. S., Kevadiya, B. D., Cheng, J., Chen, M., et al. (2021). Endogenous NO-releasing carbon nanodots for tumor-specific gas therapy. Acta Biomater. 136, 485–494. doi:10.1016/j.actbio.2021.09.051
Liu, Y., Lei, J. H., Wang, G., Zhang, Z., Wu, J., Zhang, B., et al. (2022). Toward strong near-infrared absorption/emission from carbon dots in aqueous media through solvothermal fusion of large conjugated perylene derivatives with post-surface engineering. Adv. Sci. (Weinh). 9 (23), e2202283. doi:10.1002/advs.202202283
Liyanage, P. Y., Zhou, Y., Al-Youbi, A. O., Bashammakh, A. S., El-Shahawi, M. S., Vanni, S., et al. (2020). Pediatric glioblastoma target-specific efficient delivery of gemcitabine across the blood–brain barrier via carbon nitride dots. Nanoscale 12 (14), 7927–7938. doi:10.1039/d0nr01647k
Łoczechin, A., Séron, K., Barras, A., Giovanelli, E., Belouzard, S., Chen, Y-T., et al. (2019). Functional carbon quantum dots as medical countermeasures to human coronavirus. ACS Appl. Mater Interfaces 11 (46), 42964–42974. doi:10.1021/acsami.9b15032
Luo, L., Liu, C., He, T., Zeng, L., Xing, J., Xia, Y., et al. (2018). Engineered fluorescent carbon dots as promising immune adjuvants to efficiently enhance cancer immunotherapy. Nanoscale 10 (46), 22035–22043. doi:10.1039/c8nr07252c
Lv, R., Li, G., Lu, S., and Wang, T. (2021). Synthesis of multi-functional carbon quantum dots for targeted antitumor therapy. J. Fluoresc. 31 (2), 339–348. doi:10.1007/s10895-020-02661-5
Martins, I., Tomás, H., Lahoz, F., and Rodrigues, J. (2021). Engineered fluorescent carbon dots and G4-G6 PAMAM dendrimer nanohybrids for bioimaging and gene delivery. Biomacromolecules 22 (6), 2436–2450. doi:10.1021/acs.biomac.1c00232
Mathad, A. S., Seetharamappa, J., and Kalanur, S. S. (2022). β-Cyclodextrin anchored neem carbon dots for enhanced electrochemical sensing performance of an anticancer drug, lapatinib via host-guest inclusion. J. Mol. Liq. 350, 118582. doi:10.1016/j.molliq.2022.118582
Mickaël, C., Jiahui, F., Mickaël, R., Françoise, P., and Luc, L. (2019). Influence of carbonization conditions on luminescence and gene delivery properties of nitrogen-doped carbon dots. RSC Adv. 9 (6), 3493–3502. doi:10.1039/c8ra09651a
Ming, H., Ma, Z., Liu, Y., Pan, K., Yu, H., Wang, F., et al. (2012). Large scale electrochemical synthesis of high quality carbon nanodots and their photocatalytic property. Dalton Trans. 41 (31), 9526–9531. doi:10.1039/c2dt30985h
Mintz, K. J., Bartoli, M., Rovere, M., Zhou, Y., Hettiarachchi, S. D., Paudyal, S., et al. (2021). A deep investigation into the structure of carbon dots. Carbon 173, 433–447. doi:10.1016/j.carbon.2020.11.017
Mintz, K. J., Cilingir, E. K., Nagaro, G., Paudyal, S., Zhou, Y., Khadka, D., et al. (2022). Development of red-emissive carbon dots for bioimaging through a building block approach: Fundamental and applied studies. Bioconjug Chem. 33 (1), 226–237. doi:10.1021/acs.bioconjchem.1c00544
Mintz, K. J., Mercado, G., Zhou, Y., Ji, Y., Hettiarachchi, S. D., Liyanage, P. Y., et al. (2019). Tryptophan carbon dots and their ability to cross the blood-brain barrier. Colloids Surfaces B Biointerfaces. 176, 488–493. doi:10.1016/j.colsurfb.2019.01.031
Mohammadi, S., Mohammadi, S., and Salimi, A. (2021). A 3D hydrogel based on chitosan and carbon dots for sensitive fluorescence detection of microRNA-21 in breast cancer cells. Talanta 224, 121895. doi:10.1016/j.talanta.2020.121895
Mohammadinejad, R., Dadashzadeh, A., Moghassemi, S., Ashrafizadeh, M., Dehshahri, A., Pardakhty, A., et al. (2019). Shedding light on gene therapy: Carbon dots for the minimally invasive image-guided delivery of plasmids and noncoding RNAs-A review. J. Adv. Res. 18, 81–93. doi:10.1016/j.jare.2019.01.004
Montané, X., Bajek, A., Roszkowski, K., Montornés, J. M., Giamberini, M., Roszkowski, S., et al. (2020). Encapsulation for cancer therapy. Molecules 25 (7), 1605. doi:10.3390/molecules25071605
Naik, K., Chaudhary, S., Ye, L., and Parmar, A. S. (2022). A strategic review on carbon quantum dots for cancer-diagnostics and treatment. Front. Bioeng. Biotechnol. 10, 882100. doi:10.3389/fbioe.2022.882100
Nasrin, A., Hassan, M., and Gomes, V. G. (2020). Two-photon active nucleus-targeting carbon dots: Enhanced ROS generation and photodynamic therapy for oral cancer. Nanoscale 12 (40), 20598–20603. doi:10.1039/d0nr05210h
Nocito, G., Calabrese, G., Forte, S., Petralia, S., Puglisi, C., Campolo, M., et al. (2021). Carbon dots as promising tools for cancer diagnosis and therapy. Cancers 13 (9), 1991. doi:10.3390/cancers13091991
Othman, H. O., Salehnia, F., Hosseini, M., Hassan, R., Faizullah, A., and Ganjali, M. R. (2020). Fluorescence immunoassay based on nitrogen doped carbon dots for the detection of human nuclear matrix protein NMP22 as biomarker for early stage diagnosis of bladder cancer. Microchem. J. 157, 104966. doi:10.1016/j.microc.2020.104966
Pandey, A., Devkota, A., Yadegari, Z., Dumenyo, K., and Taheri, A. (2021). Antibacterial properties of citric acid/β-alanine carbon dots against gram-negative bacteria. Nanomaterials 11 (8), 2012. doi:10.3390/nano11082012
Qin, X., Liu, J., Zhang, Q., Chen, W., Zhong, X., and He, J. (2021). Synthesis of yellow-fluorescent carbon nano-dots by microplasma for imaging and photocatalytic inactivation of cancer cells. Nanoscale Res. Lett. 16 (1), 14–19. doi:10.1186/s11671-021-03478-2
Rahmani, Z., Ghaemy, M., and Olad, A. (2021). Preparation of nanogels based on kappa-carrageenan/chitosan and N-doped carbon dots: Study of drug delivery behavior. Polym. Bull. 78 (5), 2709–2726. doi:10.1007/s00289-020-03236-x
Rajendran, S., Zichri, S. B., Usha Vipinachandran, V., Jelinek, R., and Bhunia, S. K. (2021). Triphenylphosphonium-derived bright green fluorescent carbon dots for mitochondrial targeting and rapid selective detection of tetracycline. ChemNanoMat 7 (5), 545–552. doi:10.1002/cnma.202100125
Ren, G., Hou, X., Kang, Y., Zhang, R., Zhang, M., Liu, W., et al. (2020). Efficient preparation of nitrogen-doped fluorescent carbon dots for highly sensitive detection of metronidazole and live cell imaging. Spectrochim. Acta A Mol. Biomol. Spectrosc. 234, 118251. doi:10.1016/j.saa.2020.118251
Ren, W., Chen, S., Liao, Y., Li, S., Ge, J., Tao, F., et al. (2019). Near-infrared fluorescent carbon dots encapsulated liposomes as multifunctional nano-carrier and tracer of the anticancer agent cinobufagin in vivo and in vitro. Colloids Surfaces B Biointerfaces. 174, 384–392. doi:10.1016/j.colsurfb.2018.11.041
Ren, W., Nan, F., Li, S., Yang, S., Ge, J., and Zhao, Z. (2021). Red emissive carbon dots prepared from polymers as an efficient nanocarrier for coptisine delivery in vivo and in vitro. ChemMedChem 16 (4), 646–653. doi:10.1002/cmdc.202000420
Romero, M. P., Alves, F., Stringasci, M. D., Buzzá, H. H., Ciol, H., Inada, N. M., et al. (2021). One-pot microwave-assisted synthesis of carbon dots and in vivo and in vitro antimicrobial photodynamic applications. Front. Microbiol. 12, 662149. doi:10.3389/fmicb.2021.662149
Sahu, S., Behera, B., Maiti, T. K., and Mohapatra, S. (2012). Simple one-step synthesis of highly luminescent carbon dots from orange juice: Application as excellent bio-imaging agents. Chem. Commun. (Camb). 48 (70), 8835–8837. doi:10.1039/c2cc33796g
Saravanan, A., Maruthapandi, M., Das, P., Ganguly, S., Margel, S., Luong, J. H., et al. (2020). Applications of N-doped carbon dots as antimicrobial agents, antibiotic carriers, and selective fluorescent probes for nitro explosives. ACS Appl. Bio Mater. 3 (11), 8023–8031. doi:10.1021/acsabm.0c01104
Saravanan, A., Maruthapandi, M., Das, P., Luong, J. H., and Gedanken, A. (2021). Green synthesis of multifunctional carbon dots with antibacterial activities. Nanomaterials 11 (2), 369. doi:10.3390/nano11020369
Sekar, R., Basavegowda, N., Jena, S., Jayakodi, S., Elumalai, P., Chaitanyakumar, A., et al. (2022). Recent developments in heteroatom/metal-doped carbon dot-based image-guided photodynamic therapy for cancer. Pharmaceutics 14 (9), 1869. doi:10.3390/pharmaceutics14091869
Sen, P., and Nyokong, T. (2021). Promising photodynamic antimicrobial activity of polyimine substituted zinc phthalocyanine and its polycationic derivative when conjugated to nitrogen, sulfur, co-doped graphene quantum dots against Staphylococcus aureus. Photodiagnosis Photodyn. Ther. 34, 102300. doi:10.1016/j.pdpdt.2021.102300
Sharma, A., Panwar, V., Thomas, J., Chopra, V., Roy, H. S., and Ghosh, D. (2021). Actin-binding carbon dots selectively target glioblastoma cells while sparing normal cells. Colloids Surf. B Biointerfaces 200, 111572. doi:10.1016/j.colsurfb.2021.111572
Shoval, A., Markus, A., Zhou, Z., Liu, X., Cazelles, R., Willner, I., et al. (2019). Anti-VEGF-aptamer modified C-dots—a hybrid nanocomposite for topical treatment of ocular vascular disorders. Small 15 (40), 1902776. doi:10.1002/smll.201902776
Singh, G., Kaur, H., Sharma, A., Singh, J., Alajangi, H. K., Kumar, S., et al. (2021). Carbon based nanodots in early diagnosis of cancer. Front. Chem. 9, 669169. doi:10.3389/fchem.2021.669169
Singh, S., Mishra, A., Kumari, R., Sinha, K. K., Singh, M. K., and Das, P. (2017). Carbon dots assisted formation of DNA hydrogel for sustained release of drug. Carbon 114, 169–176. doi:10.1016/j.carbon.2016.12.020
Singh, V., Rawat, K. S., Mishra, S., Baghel, T., Fatima, S., John, A. A., et al. (2018). Biocompatible fluorescent carbon quantum dots prepared from beetroot extract for in vivo live imaging in C. elegans and BALB/c mice. J. Mater Chem. B 6 (20), 3366–3371. doi:10.1039/c8tb00503f
Sk, M. P., Jaiswal, A., Paul, A., Ghosh, S. S., and Chattopadhyay, A. (2012). Presence of amorphous carbon nanoparticles in food caramels. Sci. Rep. 2, 383. doi:10.1038/srep00383
Sohail, M., Xie, B., Li, B., and Huang, H. (2022). Zn-Doped carbon dots-based versatile bioanalytical probe for precise estimation of antioxidant activity index of multiple samples via fenton Chemistry. J. Sensors Actuators B Chem. 363, 131558. doi:10.1016/j.snb.2022.131558
Su, D., Han, X., Yan, X., Jin, R., Li, H., Kong, D., et al. (2020b). Smartphone-assisted robust sensing platform for on-site quantitation of 2,4-dichlorophenoxyacetic acid using red emissive carbon dots. Anal. Chem. 92 (18), 12716–12724. doi:10.1021/acs.analchem.0c03275
Su, W., Guo, R., Yuan, F., Li, Y., Li, X., Zhang, Y., et al. (2020a). Red-emissive carbon quantum dots for nuclear drug delivery in cancer stem cells. J. Phys. Chem. Lett. 11 (4), 1357–1363. doi:10.1021/acs.jpclett.9b03891
Sun, B., Wu, F., Zhang, Q., Chu, X., Wang, Z., Huang, X., et al. (2021). Insight into the effect of particle size distribution differences on the antibacterial activity of carbon dots. J. Colloid Interface Sci. 584, 505–519. doi:10.1016/j.jcis.2020.10.015
Sun, Y., Zheng, S., Liu, L., Kong, Y., Zhang, A., Xu, K., et al. (2020). The cost-effective preparation of green fluorescent carbon dots for bioimaging and enhanced intracellular drug delivery. Nanoscale Res. Lett. 15 (1), 55–59. doi:10.1186/s11671-020-3288-0
Talib, A. B., and Mohammed, M. H. (2021). Preparation, characterization and preliminary cytotoxic evaluation of 6-mercaptopurine-coated biotinylated carbon dots nanoparticles as a drug delivery system. Mater. Today Proc. 80, 2327–2333. doi:10.1016/j.matpr.2021.06.341
Tang, C., Long, R., Tong, X., Guo, Y., Tong, C., and Shi, S. (2021). Dual-emission biomass carbon dots for near-infrared ratiometric fluorescence determination and imaging of ascorbic acid. Microchem. J. 164, 106000. doi:10.1016/j.microc.2021.106000
Tang, X., Wang, H., Yu, H., Bui, B., Zhang, W., Wang, S., et al. (2022). Exploration of nitrogen-doped grape peels carbon dots for baicalin detection. Mater. Today Phys. 22, 100576. doi:10.1016/j.mtphys.2021.100576
Tao, H., Yang, K., Ma, Z., Wan, J., Zhang, Y., Kang, Z., et al. (2012). In vivo NIR fluorescence imaging, biodistribution, and toxicology of photoluminescent carbon dots produced from carbon nanotubes and graphite. Small 8 (2), 281–290. doi:10.1002/smll.201101706
Ting, D., Dong, N., Fang, L., Lu, J., Bi, J., Xiao, S., et al. (2020). Correction to multisite inhibitors for enteric coronavirus: Antiviral cationic carbon dots based on curcumin. ACS Appl. Nano Mater 3 (5), 4913. doi:10.1021/acsanm.0c00970
Tong, T., Hu, H., Zhou, J., Deng, S., Zhang, X., Tang, W., et al. (2020). Glycyrrhizic-acid-based carbon dots with high antiviral activity by multisite inhibition mechanisms. Small 16 (13), 1906206. doi:10.1002/smll.201906206
Wang, B., Cai, H., Waterhouse, G. I. N., Qu, X., Yang, B., and Lu, S. (2022a). Carbon dots in bioimaging, biosensing and therapeutics: A comprehensive review. Small Sci. 2 (6), 2200012. doi:10.1002/smsc.202200012
Wang, L., Pan, H., Gu, D., Li, P., Su, Y., and Pan, W. (2022b). A composite system combining self-targeted carbon dots and thermosensitive hydrogels for challenging ocular drug delivery. J. Pharm. Sci. 111 (5), 1391–1400. doi:10.1016/j.xphs.2021.09.026
Wang, L., Pan, H., Gu, D., Sun, H., Chen, K., Tan, G., et al. (2021a). A novel carbon dots/thermo-sensitive in situ gel for a composite ocular drug delivery system: Characterization, eEx-vVivo imaging, and in vivo evaluation. Int. J. Mol. Sci. 22 (18), 9934. doi:10.3390/ijms22189934
Wang, Q., Zhang, S., Wang, B., Yang, X., Zou, B., Yang, B., et al. (2019). Pressure-triggered aggregation-induced emission enhancement in red emissive amorphous carbon dots. Nanoscale Horizons 4 (5), 1227–1231. doi:10.1039/c9nh00287a
Wang, S., Chen, L., Wang, J., Du, J., Li, Q., Gao, Y., et al. (2020). Enhanced-fluorescent imaging and targeted therapy of liver cancer using highly luminescent carbon dots-conjugated foliate. Mater Sci. Eng. C Mater Biol. Appl. 116, 111233. doi:10.1016/j.msec.2020.111233
Wang, T., Wang, S., Liu, Z., He, Z., Yu, P., Zhao, M., et al. (2021b). A hybrid erbium(III)-bacteriochlorin near-infrared probe for multiplexed biomedical imaging. Nat. Mater 20 (11), 1571–1578. doi:10.1038/s41563-021-01063-7
Weiss, M., Fan, J., Claudel, M., Sonntag, T., Didier, P., Ronzani, C., et al. (2021). Density of surface charge is a more predictive factor of the toxicity of cationic carbon nanoparticles than zeta potential. J. Nanobiotechnology 19 (1), 5. doi:10.1186/s12951-020-00747-7
Wen, X., Zhao, Z., Zhai, S., Wang, X., and Li, Y. (2020). Stable nitrogen and sulfur co-doped carbon dots for selective folate sensing, in vivo imaging and drug delivery. Diam. Relat. Mater. 105, 107791. doi:10.1016/j.diamond.2020.107791
Wu, L-N., Yang, Y-J., Huang, L-X., Zhong, Y., Chen, Y., Gao, Y-R., et al. (2022). Levofloxacin-based carbon dots to enhance antibacterial activities and combat antibiotic resistance. Carbon 186, 452–464. doi:10.1016/j.carbon.2021.10.020
Xu, N., Du, J., Yao, Q., Ge, H., Shi, C., Xu, F., et al. (2021). Carbon dots inspired by structure-inherent targeting for nucleic acid imaging and localized photodynamic therapy. Sensors Actuators B Chem. 344, 130322. doi:10.1016/j.snb.2021.130322
Xu, X., Ray, R., Gu, Y., Ploehn, H. J., Gearheart, L., Raker, K., et al. (2004). Electrophoretic analysis and purification of fluorescent single-walled carbon nanotube fragments. J. Am. Chem. Soc. 126 (40), 12736–12737. doi:10.1021/ja040082h
Xu, X., Zhang, K., Zhao, L., Li, C., Bu, W., Shen, Y., et al. (2016). Aspirin-based carbon dots, a good biocompatibility of material applied for bioimaging and anti-inflammation. ACS Appl. Mater Interfaces 8 (48), 32706–32716. doi:10.1021/acsami.6b12252
Xu, Y., Wang, C., Jiang, T., Ran, G., and Song, Q. (2022). Cadmium induced aggregation of orange–red emissive carbon dots with enhanced fluorescence for intracellular imaging. J. Hazard. Mater. 427, 128092. doi:10.1016/j.jhazmat.2021.128092
Xue, Y., Liu, C., Andrews, G., Wang, J., and Ge, Y. (2022). Recent advances in carbon quantum dots for virus detection, as well as inhibition and treatment of viral infection. J. Nano Converg. 9 (1), 15–31. doi:10.1186/s40580-022-00307-9
Yadav, P. K., Chandra, S., Kumar, V., Kumar, D., and Hasan, S. H. (2023). Carbon quantum dots: Synthesis, structure, properties, and catalytic applications for organic synthesis. Catalysts 13 (2), 422. doi:10.3390/catal13020422
Ye, Z., Li, G., Lei, J., Liu, M., Jin, Y., and Li, B. (2020). One-Step and one-precursor hydrothermal synthesis of carbon dots with superior antibacterial activity. ACS Appl. Bio Mater. 3 (10), 7095–7102. doi:10.1021/acsabm.0c00923
Yu, A., Tang, Y., Li, K., Gao, J., Zheng, Y., and Zeng, Z. (2019). Tunable photoluminescence studies based on blue-emissive carbon dots and sequential determination of Fe (III) and pyrophosphate ions. Spectrochim. Acta A Mol. Biomol. Spectrosc. 222, 117231. doi:10.1016/j.saa.2019.117231
Yue, J., Li, L., Cao, L., Zan, M., Yang, D., Wang, Z., et al. (2019). Two-step hydrothermal preparation of carbon dots for calcium ion detection. ACS Appl. Mater. Interfaces 11 (47), 44566–44572. doi:10.1021/acsami.9b13737
Yue, J., Li, L., Jiang, C., Mei, Q., Dong, W-F., and Yan, R. (2021). Riboflavin-based carbon dots with high singlet oxygen generation for photodynamic therapy. J. Mater Chem. B 9 (38), 7972–7978. doi:10.1039/d1tb01291f
Zeng, Q., Shao, D., He, X., Ren, Z., Ji, W., Shan, C., et al. (2016). Carbon dots as a trackable drug delivery carrier for localized cancer therapy in vivo. J. Mater. Chem. B 4 (30), 5119–5126. doi:10.1039/c6tb01259k
Zhang, M., Zhai, X., Ma, T., Huang, Y., Yan, C. H., and Du, Y. (2021b). Multifunctional cerium doped carbon dots nanoplatform and its applications for wound healing. Chem. Eng. J. 423, 130301. doi:10.1016/j.cej.2021.130301
Zhang, M., Zheng, T., Sheng, B., Wu, F., Zhang, Q., Wang, W., et al. (2019b). Mn2+ complex-modified polydopamine-and dual emissive carbon dots based nanoparticles for in vitro and in vivo trimodality fluorescent, photothermal, and magnetic resonance imaging. Chem. Eng. J. 373, 1054–1063. doi:10.1016/j.cej.2019.05.107
Zhang, W., Sigdel, G., Mintz, K. J., Seven, E. S., Zhou, Y., Wang, C., et al. (2021a). Carbon dots: A future blood–brain barrier penetrating nanomedicine and drug nanocarrier. Int. J. nanomedicine 16, 5003–5016. doi:10.2147/ijn.s318732
Zhang, Y., Wang, S., Lu, F., Zhang, M., Kong, H., Cheng, J., et al. (2021c). The neuroprotective effect of pretreatment with carbon dots from Crinis Carbonisatus (carbonized human hair) against cerebral ischemia reperfusion injury. J. Nanobiotechnology 19 (1), 257. doi:10.1186/s12951-021-00908-2
Zhang, Z., Lei, Y., Yang, X., Shi, N., Geng, L., Wang, S., et al. (2019a). High drug-loading system of hollow carbon dots–doxorubicin: Preparation, in vitro release and pH-targeted research. J. Mater Chem. B 7 (13), 2130–2137. doi:10.1039/c9tb00032a
Zhao, C., Wang, X., Wu, L., Wu, W., Zheng, Y., Lin, L., et al. (2019). Nitrogen-doped carbon quantum dots as an antimicrobial agent against Staphylococcus for the treatment of infected wounds. Colloids Surfaces B Biointerfaces 179, 17–27. doi:10.1016/j.colsurfb.2019.03.042
Zhao, D., Zhang, Z., Liu, X., Zhang, R., and Xiao, X. (2021). Rapid and low-temperature synthesis of N, P co-doped yellow emitting carbon dots and their applications as antibacterial agent and detection probe to Sudan Red I. Mater. Sci. Eng. C 119, 111468. doi:10.1016/j.msec.2020.111468
Zhao, W-B., Chen, D-D., Liu, K-K., Wang, Y., Zhou, R., Song, S-Y., et al. (2023). Near-infrared I/II emission and absorption carbon dots via constructing localized excited/charge transfer state for multiphoton imaging and photothermal therapy. Chem. Eng. J. 452, 139231. doi:10.1016/j.cej.2022.139231
Zhao, Y., Zhang, Y., Kong, H., Cheng, G., Qu, H., and Zhao, Y. (2022). Protective effects of carbon dots derived from Armeniacae semen Amarum carbonisata against acute lung injury induced by lipopolysaccharides in rats. Int. J. Nanomedicine 17, 1–14. doi:10.2147/ijn.s338886
Zhou, Y., Liyanage, P. Y., Devadoss, D., Guevara, L. R. R., Cheng, L., Graham, R. M., et al. (2019). Nontoxic amphiphilic carbon dots as promising drug nanocarriers across the blood–brain barrier and inhibitors of β-amyloid. Nanoscale 11 (46), 22387–22397. doi:10.1039/c9nr08194a
Zhou, Y., Mintz, K. J., Cheng, L., Chen, J., Ferreira, B., Hettiarachchi, S. D., et al. (2020). Direct conjugation of distinct carbon dots as Lego-like building blocks for the assembly of versatile drug nanocarriers. J. Colloid Interface Sci. 576, 412–425. doi:10.1016/j.jcis.2020.05.005
Zhu, X., Pang, X., Zhang, Y., and Yao, S. (2019). Titanium carbide MXenes combined with red-emitting carbon dots as a unique turn-on fluorescent nanosensor for label-free determination of glucose. J. Mater Chem. B 7 (48), 7729–7735. doi:10.1039/c9tb02060h
Zu, F., Yan, F., Bai, Z., Xu, J., Wang, Y., Huang, Y., et al. (2017). The quenching of the fluorescence of carbon dots: A review on mechanisms and applications. Microchim. Acta 184 (7), 1899–1914. doi:10.1007/s00604-017-2318-9
Keywords: carbon dots, drug delivery, gene delivery, nanocarriers, bioimaging
Citation: Kaurav H, Verma D, Bansal A, Kapoor DN and Sheth S (2023) Progress in drug delivery and diagnostic applications of carbon dots: a systematic review. Front. Chem. 11:1227843. doi: 10.3389/fchem.2023.1227843
Received: 23 May 2023; Accepted: 05 July 2023;
Published: 14 July 2023.
Edited by:
Rita Petrucci, Sapienza University of Rome, ItalyReviewed by:
Zhimei He, Nanjing University of Posts and Telecommunications, ChinaLuís Pinto Da Silva, University of Porto, Portugal
Qi Zhao, Technical Institute of Physics and Chemistry (CAS), China
Copyright © 2023 Kaurav, Verma, Bansal, Kapoor and Sheth. This is an open-access article distributed under the terms of the Creative Commons Attribution License (CC BY). The use, distribution or reproduction in other forums is permitted, provided the original author(s) and the copyright owner(s) are credited and that the original publication in this journal is cited, in accordance with accepted academic practice. No use, distribution or reproduction is permitted which does not comply with these terms.
*Correspondence: Sandeep Sheth, c3NoZXRoQGxhcmtpbi5lZHU=
†These authors have contributed equally to this work and share first authorship