- Department of Organic Chemistry, Faculty of Chemistry, Alzahra University, Tehran, Iran
We describe the first classic example of green synthesis of pyrrolo[3,4-c]quinolones scaffolds by catalyst-free unusual reaction of diketene, isatin, and primary amines in ethanol in the presence of pyrazole as a promoter for 4 h. The whole structure of the new product was confirmed by X-ray analysis. The overall transformation involves the cleavage and generation of multiple carbon-nitrogen and carbon-carbon bonds. This report represents a simple and straightforward approach for the synthesis of pyrrolo[3,4-c]quinoline-1,3-diones, which has significant advantages like readily available precursors, non-use of toxic solvent, operational simplicity, mild conditions, good atom economy, and excellent yields; therefore it provides a green and sustainable strategy for access to a range of interesting N-containing heterocyclic compounds in medicinal and organic chemistry.
1 Introduction
Quinolines have received lots of attention from biologists and chemists as they are significant elements in the synthesis of dyes, fragrances, and natural products with biological activities (Michael, 2001; Michael, 2002; Michael, 2003; Michael, 2004; Michael, 2005; Michael, 2007; Michael, 2008; Isaac-Márquez et al., 2010; Cai et al., 2011; Russ et al., 2012). In pharmaceuticals, they have been outlined as, antibiotic (Mahamoud et al., 2006), anticancer (Insuasty et al., 2013; Sun et al., 2013), anti-inflammatory (Leatham et al., 1983), antimalarial (Nasveld and Kitchener, 2005), antihypertensive (Muruganantham et al., 2004), anti-HIV (Strekowski et al., 1991; Wilson et al., 1992), inhibition of Platelet-derived growth factor (PDGF) (Maguire et al., 1994), and anti-tuberculosis (Lilienkampf et al., 2009) agents. In other words, pyrrolidones are also typical buildings in several important categories of bioactive compounds (Jouyban et al., 2010; Kato and Nagao, 2012). Molecules bearing a pyrrolidone structure, are used in dye-sensitized solar cells and several natural products with biologically activeties (Daly et al., 1999; Dewick, 2009; Ikai et al., 2012). For instance, arcyria rubin A and its derivatives show potent antiviral activities (KIM et al., 1995; Slater et al., 1995), antimicrobial (Mahboobi et al., 2006), and powerful protein kinase C inhibitors (Davis et al., 1992).
The merger of these outstanding heterocycles, pyrrolidone, and quinolone is promising classes of pharmaceutical frameworks with antifungal (Chen et al., 2004), anti-inflammatory (Kategaonkar et al., 2010), anticancer (Eswaran et al., 2010), anti-tuberculosis (Tseng et al., 2010), anti-Alzheimer (Tseng et al., 2009), anti-HIV, anti-hypertension, and anticancer activities (Camps et al., 2009; Sharma et al., 2018). They also have inhibitory activities versus hepatitis C virus (HCV) polymerase (Thomas and Tallman, 1981; Summa et al., 2009), ADAMTS-5 (A disintegrin and metalloproteinase with thrombospondin motifs 5) and ADAMTS-4 (A disintegrin and metalloproteinase with thrombospondin motifs 4) (Sharma et al., 2008; Cappelli et al., 2010).
In this regard, the pyrrolo[3,4-c]quinoline-1,3-dione segment (1) exhibits a perfect range of pharmacologically and biologically enjoyable activities (Figure 1) (Okun et al., 2006a; Okun et al., 2006b; Segura-Cabrera et al., 2011; Mollin et al., 2012). For example, pyrrolo[3,4-c]quinoline (2) is a potent inhibitor of caspase-3 (Kravchenko et al., 2005a), which plays a clef role in apoptosis (Porter and Jänicke, 1999; Hentze et al., 2003). Caspases are interesting goals for therapeutic intervention in neurodegenerative, cardiovascular and metabolic disorders (Lockshin et al., 1998; Lockshin and Zakeri, 2004). In particular, caspase-3 inhibitors have been reported as powerful hepatoprotectants (Lockshin et al., 1998; Hoglen et al., 2001; Segawa et al., 2001; Lockshin and Zakeri, 2004; Meki et al., 2004), cardioprotectants (Chapman et al., 2002; Isabel et al., 2003), and neuroprotectants (Scott et al., 2003). Also, compound (3) has inhibitory activity against HCV polymerase (Di Francesco et al., 2009). Furthermore, alpkinidine (4) has shown potent therapeutic efficacy in vivo in HCT-116-bearing mice (Valeriote et al., 2012).
Because of broad applications in medicinal chemistry, the synthesis of these fused interesting heterocycles has specific importance to the pharmaceutical and organic chemists. Newly, there has been increasing attentiveness in the construction of pyrrole-fused-quinolines, and various procedures have been reported. Main synthetic approaches include Lewis acid-catalyzed electrophilic cyclization (Aggarwal et al., 2012), copper (Kiruthika et al., 2014) and palladium-catalyzed (Chai and Lautens, 2009; Shukla et al., 2012; Kiruthika et al., 2014) reactions, DDQ-mediated intramolecular cyclization (Wald et al., 1980), allene-based reaction cascades (Baumann and Baxendale, 2015), photo substituted reactions and flash vacuum pyrolysis. Although the majority of synthetic plans were applied for the synthesis of pyrrolo[3,2-c]quinoline and pyrrolo[1,2-a]quinoline analogs. Few synthetic relate have been released on the synthesis of pyrrolo[3,4-c]quinolones, and only multi-step synthetic methods are known to date.
In this matter, there are notable examples based on the cyclo condensation of b-keto amides and 2-amino-5-fluorophenyl glyoxylic acid (Ivachtchenko et al., 2003), Pfitzinger reaction (Mortoni et al., 2004; Kravchenko et al., 2005b), the one-pot two-component method by DMAP-catalyzed (Avula et al., 2013), the BF3Et2O-catalyzed isocyanide-based cycloaddition reaction (Li et al., 2013), and microwave-assisted reaction methods (Xia et al., 2014), which all are multi-step reactions (Scheme 1). However, these procedures are limited by low yields, harsh reaction conditions, the long reaction time, and their complexity.
Multicomponent reactions (MCRs) have become increasingly popular as a simple and powerful tool for the rapid formation of new scaffolds from simple starting materials with structural diversity and molecular complexity in a convergent manner (Mashayekh and Shiri, 2019; Sedighian et al., 2021). MCRs are one-pot strategies exploiting three or more simple substrates where most of the reactant atoms are incorporated into the final desired product (Chen et al., 2017; Kurhade et al., 2019; Shiri and Aboonajmi, 2020; Yavari and Safaei, 2020). In comparison to the traditional multistep sequential assembly of target compounds, MCRs manifest several advantages including easy handling, selective bond formation, time-saving, high atom economy, fewer purification steps and structural variability (Younus et al., 2021; Shiri et al., 2022).
Due to our experience and interest in the synthesis of novel heterocycles, we became engrossed in how Knoevenagel product obtained from isatine and pyrazole could be in situ trapped by keto amides resulting from diketene and primary amines to give a heterocycle product. We considered the utilization of diketene as starting material and reagent because it is extensively used for the generation for a diverse range of different heterocycles. For this purpose, in continuation of our successive attempts towards the synthesis of heterocycles by multicomponent strategies, (Rezvanian et al., 2018a; Talaei et al., 2018; Rezvanian et al., 2020a; Rezvanian et al., 2020b), especially using diketene reactions (Alizadeh et al., 2012; Rezvanian, 2015; Rezvanian, 2016; Rezvanian et al., 2017; Rezvanian et al., 2018b; Rezvanian et al., 2019; Rezvanian et al., 2020c; Rezvanian et al., 2020d; Rezvanian et al., 2020e; Rezvanian et al., 2021a; Rezvanian et al., 2021b), we herein explain an efficient approach to synthesize pyrrolo[3,4-c]quinoline-1,3-diones 7 from the reaction of isatin, diketene, and primary amines based on the unique reactivity of pyrazole as a promoter in high yields (Scheme 3).
2 Experimental section
2.1 Instrumentation, analyses, and starting materials
The diketene, various amines, hydrazine, Hydrate, ethyl acetoacetate, and isatines were obtained from commercial sources with high purity. The 1H NMR and 13C NMR spectra were run on a Bruker spectrophotometer at 300/500 and 75/125 MHz respectively. Coupling constants are reported in Hz. All mass spectra were measured on a mass spectrometer (Agilent5973 Network) at the ionization potential of 70 Ev. The IR spectra were recorded by BRUKER TENSOR 27 FT-IR instrument.
2.2 General procedure for the synthesis of 3-methyl-pyrazole-5-one
Hydrazine hydrate 70% (2 mmol) was added to ethyl acetoacetate (1.4 mmol) and was treated at room temperature without solvent. After 30 min, the product was precipitate and filtered, and washed with a few drops of water, and pyrazole was obtained as a white crystal dried and used for further steps.
2.3 General procedure for the synthesis of structurally diverse pyrrolo[3,4-c]quinoline-1,3-diones 7
To a round-bottom flask (25 mL), the following were added; pyrazole (1.0 mmol), isatin 5 (1.0 mmol), diketene (1.0 mmol), primary amine 6 (1.0 mmol); and the reaction mixture was stirred at reflux for approximately 4 h and monitored by TLC until the substrates were wholly consumed. Upon the formation of the desired product 7, the product was precipitated, filtered, and washed with a few drops of EtOH, and the target compound 7 was obtained as a yellow solid with excellent yield (73%–90%). Post separating product 7, the reaction mixture was cooled to 20°C–25°C, and upon cooling the reaction mixture and evaporation of the solvent, the sediment solid was filtered and washed with ethanol, and finally the pyrazole was obtained again with 81% yield.
3 Results and discussion
At the outset of our investigation, the reaction of hydrazine, ethyl acetoacetate, isatine 5, diketene, and primary amine 6 in the lack of any catalyst at room temperature was designed. To study this new process, isatin 5a, ethyl amine 6a, and ethyl acetoacetate were selected as model reactions (Scheme 2). In this route, firstly, hydrazine (1 mmol), ethyl acetoacetate (1 mmol), and isatin 5a (1 mmol) in ethanol (4 ml) were stirred at room temperature for 1 h, which afforded the Michael adduct 8a. Next, ethylamine 6a (1 mmol) and diketene (1 mmol) were added to the reaction mixture. The advance of the reaction was followed by TLC (1-6 ethyl acetate-hexane). Unfortunately, no product was obtained at room temperature after 48 h (Table 1, entries 1). However, when the mixture reaction was heated at 70°C gratifyingly, we observed that the acceptable product was formed in an isolated yield of 84% within 5 h (Table 1, entry 2). Upon the construction of the desired outcome, immediately the precipitated solid was filtered off, washed with ethanol, and crystallized from hot ethanol in excellent yield. Amazingly, instead of the expected spiro pyridine product 11 (Scheme 4), we observed an unanticipated process leading to pyrrolo[3,4-c]quinolone 7a in excellent yield (Scheme 2). On the other hand, post-workup product 7a, the solvent was evaporated. We observed the precipitated white solid of pyrazole (from hydrazine and ethyl acetoacetate) slowly settling in which it was filtered and washed with water. The absolute structure of the newly synthesized product 7a was explicitly confirmed by X-ray analysis.
Then, in a controlled testing, the reaction of this five-component manufacturing process proceeds in the absence of pyrazole resulting from hydrazine and ethyl acetoacetate in which the effect of pyrazole was evaluated for this reaction. We concluded that the response could not advance without pyrazole under these conditions, and when the reaction mixture was carried with pyrazole (1 mol), the objective compound 7a obtained an 84% yield. Also, the change in amounts of pyrazole was explored for the reaction. The best result (90%) of the product was formed when (1 mol) of the pyrazole was exploited. By decreasing the amount of pyrazole to (0.7 and 0.5 mol), the development was accomplished at 35% and 27%, and it was observed that increasing the pyrazole loading had a considerable effect on the formation product. However, without using pyrazole, the reaction failed to develop even after 48 h.
Thus, to increase the yield of pyrrolo[3,4-c]quinoline-1,3-dione 7a and minimize reaction time, four-component reactions between isatin 5a, pyrazole, diketene, and ethylamine 6a were designed (Table 1), because of the success achieved using pyrazole promoted response. We found pyrrolo[3,4-c]quinoline-1,3-dione 7a as the only product when the reaction mixture was composed of a 1:1:1:1 variety of compounds.
In this reaction, solvent and temperature were examined to optimize the reaction conditions. Due; to the impossibility of carrying out the reaction at ambient temperature, the response was performed under reflux conditions. Also, it was observed that increasing the temperature above 70°C has no significant effect on the product yield. Despite obtaining good results, organic solvents did not improve much compared to water, including acetonitrile, methanol, water, water/ethanol, and tetrahydrofuran). Therefore, all reactions were performed under reflux conditions at 70°C in water to give satisfactory and excellent results.
Reaction conditions: pyrazole (1.0 mmol), isatine 5a (1.0 mmol), diketene (1.0 mmol), ethyl amine 6a (1.0 mmol), solvent (4.0 ml). [b] Isolated yield.
Having identified the best available conditions, to explore the efficiency and generality of this approach, the reactions between another isatine 1 and primary amines 2 were conducted, and the outcomes are shown in Scheme 3. The corresponding functionalized pyrrolo[3,4-c]quinoline-1,3-diones 7 were obtained in excellent yields at 70°C in ethanol in the presence of pyrazole (1 mmol) as a promoter. Various primary amines (6a-f) reacted with isatines to generate corresponding pyrrolo[3,4-c]quinoline-1,3-diones 7a-h.
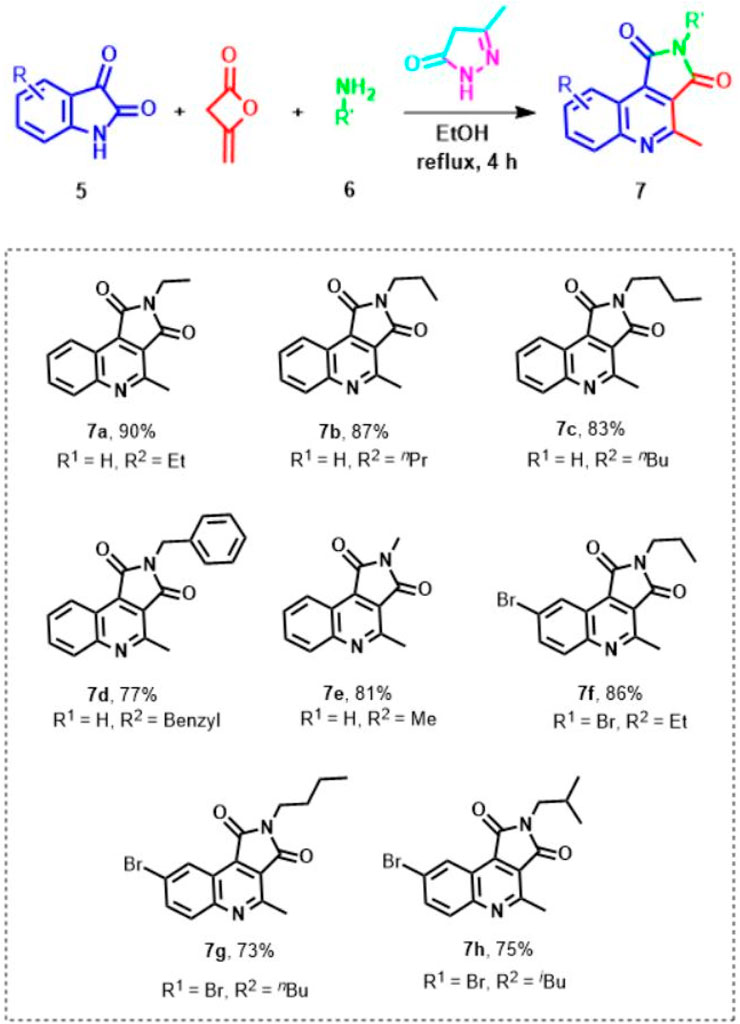
SCHEME 3. Scope; of the reaction.a,b.a[a] The reaction was performed at 70°C with 1 equivalent of substrates. [b] The new pyrrolo[3,4-c]quinoline-1,3-diones were afforded (see the Supporting Information).
The skeleton of all synthetic compounds 7a-h was elucidated by ESI-MS, IR, 1HNMR, 13CNMR spectroscopy, and X-ray analysis. FTIR of 7a exhibited absorption bands in 1764, 1705, and 1622 due to the two CO and C=N stretching frequencies. In the 1H-NMR spectrum of 7a, triplet and quartet in δ = 1.31 (3JH-H = 7.2 Hz) and δ = 3.77 (3JH-H = 7.2 Hz) ppm are due to CH3 and CH2 groups. The Singlet peak in δ = 3.01 is due to the CH3 group. Also the signals in the aromatic section confirmed the presence of the four aromatic hydrogens of the aromatic ring. The presence of 14 apparent signs in the 13C-NMR spectrum is in accordance with the suggested structure of 7a. The highlighted areas in the 13C NMR are due to two CH3, two CH2, and two C=O groups, which are evident at δ = 13.94, 22.03, 33.01, 168.06, 168.33 ppm. Single crystal X-ray crystallography structure of 7b was certified as the product structure (Figure 2).
This reaction is a particular case, and a probable tool is illustrated in Scheme 4 for the generation of compound 7. It is advisable to suggest that the first stage starts via a Knoevenagel-type condensation of isatine and pyrazole to provide the intermediate 8 as the Michael acceptor. Then, nucleophilic attack of the amine to diketene and ring-opening of diketene pursue by proton transfer to give β-ketoamide 9. After the formation of adduct 8, nucleophilic addition enol form 10 on the Michael acceptor 8 afforded intermediate 11 via Michael addition. At this stage, attending to the preceding articles, we expected adduct 11 with O-cyclization, attacking the carbonyl group, and tautomerization to give the desired heterocyclic compound 13. But contemplating X-ray diffraction and 1H and 13C NMR spectra, product 12 was not formed, and another extraordinary incident happened. In truth, in this step, nucleophilic addition of the enol form 10 is followed the elimination of pyrazole to give 14 via proton transfer. Then, in intermediate 14, with an intramolecular cyclization via N-nucleophilic attack of the amide group and proton transfer to form middle 15, ring opening and proton transfer gives the medium 17. Finally, nucleophilic attack of the amine 17 to C=O bond, intramolecular cyclization to form middle 18, elimination of H2O, and deprotonation product 7 are created.
4 Conclusion
As a result, we have described an unusual three-component reaction to construct novel molecules containing a pyrrolo[3,4-c]quinoline-1,3-dione core from readily available reagents of pyrazole, isatine, diketene, and primary amine in ethanol. The most significant aspects of the process are the accessibility of the starting precursors, mild reaction conditions, short reaction times, high yields of the synthesized products, and easy operation at the manufacturing scale. The overall process of reaction includes all the aspects of green chemistry and has new portals for the growth of more sustainable multicomponent reactions. This category of heterocycles with several pharmacophores may be interesting for medicine and pharmacology.
Data availability statement
Detailed experimental procedures and compound characterization data in the Supporting Information (PDF) are available. X-ray Crystallography: Deposition Number 7a is 2202802c and contains the supplementary crystallographic data for this paper. www.ccdc.cam.ac.uk/structures.
Author contributions
AR analyzed spectral characterization of synthesized molecules. AR wrote and edited the article. All authors contributed to the article and approved the submitted version.
Acknowledgments
Financial support from the Research Council of Alzahra University is sincerely acknowledged.
Conflict of interest
The authors declare that the research was conducted in the absence of any commercial or financial relationships that could be construed as a potential conflict of interest.
Publisher’s note
All claims expressed in this article are solely those of the authors and do not necessarily represent those of their affiliated organizations, or those of the publisher, the editors and the reviewers. Any product that may be evaluated in this article, or claim that may be made by its manufacturer, is not guaranteed or endorsed by the publisher.
Supplementary material
The Supplementary Material for this article can be found online at: https://www.frontiersin.org/articles/10.3389/fchem.2023.1219986/full#supplementary-material
References
Aggarwal, T., Kumar, S., Dhaked, D. K., Tiwari, R. K., Bharatam, P. V., and Verma, A. K. (2012). Site-selective electrophilic cyclization and subsequent ring-opening: A synthetic route to pyrrolo [1, 2-a] quinolines and indolizines. J. Org. Chem. 77 (19), 8562–8573. doi:10.1021/jo3015374
Alizadeh, A., Rezvanian, A., and Zhu, L. G. (2012). Synthesis of heterocyclic [3.3. 3] propellanes via a sequential four-component reaction. J. Org. Chem. 77 (9), 4385–4390. doi:10.1021/jo300457m
Avula, S., Koppireddi, S., Komsani, J. R., Nanubolu, J. B., and Yadla, R. (2013). One-pot two-component tandem multiple transformations in the synthesis of N, 4-diaryl-2, 3-dihydropyrrolo [3, 4-c] quinolin-1, 3-diones and their 3-thioxo-analogues under neat conditions. RSC Adv. 3 (43), 20990–20998. doi:10.1039/c3ra43489c
Baumann, M., and Baxendale, I. R. (2015). Batch and flow synthesis of pyrrolo [1, 2-a]-quinolines via an allene-based reaction cascade. J. Org. Chem. 80 (21), 10806–10816. doi:10.1021/acs.joc.5b01982
Cai, X. H., Li, Y., Su, J., Liu, Y. P., Li, X. N., and Luo, X. D. (2011). Novel indole and quinoline alkaloids from Melodinus yunnanensis. Nat. Prod. bioprospecting 1 (1), 25–28. doi:10.1007/s13659-011-0001-0
Camps, P., Formosa, X., Galdeano, C., Munoz-Torrero, D., Ramírez, L., Gómez, E., et al. (2009). Pyrano [3, 2-c] quinoline− 6-chlorotacrine hybrids as a novel family of acetylcholinesterase-and β-amyloid-directed anti-Alzheimer compounds. J. Med. Chem. 52 (17), 5365–5379. doi:10.1021/jm900859q
Cappelli, A., Nannicini, C., Valenti, S., Giuliani, G., Anzini, M., Mennuni, L., et al. (2010). Design, synthesis, and preliminary biological evaluation of pyrrolo [3, 4-c] quinolin-1-one and oxoisoindoline derivatives as aggrecanase inhibitors. ChemMedChem 5 (5), 739–748. doi:10.1002/cmdc.200900523
Chai, D. I., and Lautens, M. (2009). Tandem Pd-catalyzed double C− C bond formation: Effect of water. J. Org. Chem. 74 (8), 3054–3061. doi:10.1021/jo900053b
Chamulitrat, W., Zhang, W., Xu, W., Pathil, A., Setchell, K., and Stremmel, W. (2012). Hepatoprotectant ursodeoxycholyl lysophosphatidylethanolamide increasing phosphatidylcholine levels as a potential therapy of acute liver injury. Front. Physiology 3, 24. doi:10.3389/fphys.2012.00024
Chapman, J. G., Magee, W. P., Stukenbrok, H. A., Beckius, G. E., Milici, A. J., and Tracey, W. R. (2002). A novel nonpeptidic caspase-3/7 inhibitor,(S)-(+)-5-[1-(2-methoxymethylpyrrolidinyl) sulfonyl] isatin reduces myocardial ischemic injury. Eur. J. Pharmacol. 456 (1-3), 59–68. doi:10.1016/s0014-2999(02)02484-6
Chen, Y. L., Chen, I. L., Lu, C. M., Tzeng, C. C., Tsao, L. T., and Wang, J. P. (2004). Synthesis and anti-inflammatory evaluation of 4-anilinofuro [2, 3-b] quinoline and 4-phenoxyfuro [2, 3-b] quinoline derivatives. Part 3. Bioorg. Med. Chem. 12 (2), 387–392. doi:10.1016/j.bmc.2003.10.051
Chen, Z., Liu, Z., Cao, G., Li, H., and Ren, H. (2017). Recent advances in multicomponent synthesis of 1, 4, 5-trisubstituted 1, 2, 3-triazoles. Adv. Synthesis Catal. 359 (2), 202–224. doi:10.1002/adsc.201600918
Daly, J. W., Garraffo, H. M., and Spande, T. F. (1999). Alkaloids from amphibian skins. Alkaloids Chem. Biol. Perspect. 13, 1–161.
Davis, P. D., Hill, C. H., Lawton, G., Nixon, J. S., Wilkinson, S. E., Hurst, S. A., et al. (1992). Inhibitors of protein kinase C. 1. 2, 3-Bisarylmaleimides. J. Med. Chem. 35 (1), 177–184. doi:10.1021/jm00079a024
Dewick, P. M. (2009). Medicinal natural products: A biosynthetic approach. 3. Chichester, U.K.: John Wiley & Sons. Chapter 6 and references therein.
Di Francesco, M. E., Dessole, G., Nizi, E., Pace, P., Koch, U., Fiore, F., et al. (2009). Novel macrocyclic inhibitors of hepatitis C NS3/4A protease featuring a 2-amino-1, 3-thiazole as a P4 carbamate replacement. J. Med. Chem. 52 (22), 7014–7028. doi:10.1021/jm900524b
Eswaran, S., Adhikari, A. V., and Kumar, R. A. (2010). New 1, 3-oxazolo [4, 5-c] quinoline derivatives: Synthesis and evaluation of antibacterial and antituberculosis properties. Eur. J. Med. Chem. 45 (3), 957–966. doi:10.1016/j.ejmech.2009.11.036
Hentze, H., Lin, X. Y., Choi, M. S. K., and Porter, A. G. (2003). Critical role for cathepsin B in mediating caspase-1-dependent interleukin-18 maturation and caspase-1-independent necrosis triggered by the microbial toxin nigericin. Cell. Death Differ. 10 (9), 956–968. doi:10.1038/sj.cdd.4401264
Hoglen, N. C., Hirakawa, B. P., Fisher, C. D., Weeks, S., Srinivasan, A., Wong, A. M., et al. (2001). Characterization of the caspase inhibitor IDN-1965 in a model of apoptosis-associated liver injury. J. Pharmacol. Exp. Ther. 297 (2), 811–818.
Hulcoop, D. G., and Lautens, M. (2007). Palladium-catalyzed annulation of aryl heterocycles with strained alkenes. Org. Lett. 9 (9), 1761–1764. doi:10.1021/ol070475w
Ikai, T., Azam, A. F., Kuzuba, M., Kuwabara, T., Maeda, K., Takahashi, K., et al. (2012). Synthesis of seleno [3, 4-c] pyrrole-4, 6-dione-based polymers for polymer solar cells. Synth. Met. 162 (17-18), 1707–1712. doi:10.1016/j.synthmet.2012.07.003
Insuasty, B., Montoya, A., Becerra, D., Quiroga, J., Abonia, R., Robledo, S., et al. (2013). Synthesis of novel analogs of 2-pyrazoline obtained from [(7-chloroquinolin-4-yl) amino] chalcones and hydrazine as potential antitumor and antimalarial agents. Eur. J. Med. Chem. 67, 252–262. doi:10.1016/j.ejmech.2013.06.049
Isaac-Márquez, A. P., McChesney, J. D., Nanayakara, N. D., Satoskar, A. R., and Lezama-Dávila, C. M. (2010). Leishmanicidal activity of racemic±8-[(4-Amino-1-methylbutyl) amino]-6-methoxy-4-methyl-5-[3, 4-dichlorophenoxy] quinoline. Nat. Product. Commun. 5 (3), 1934578X1000500. doi:10.1177/1934578x1000500309
Isabel, E., Black, W. C., Bayly, C. I., Grimm, E. L., Janes, M. K., McKay, D. J., et al. (2003). Nicotinyl aspartyl ketones as inhibitors of caspase-3. Bioorg. Med. Chem. Lett. 13 (13), 2137–2140. doi:10.1016/s0960-894x(03)00390-1
Ivachtchenko, A. V., Kobak, V. V., Il'yin, A. P., Trifilenkov, A. S., and Busel, A. A. (2003). New scaffolds for combinatorial synthesis. II. 6-sulfamoylquinolinecarboxylic acids. J. Comb. Chem. 5 (5), 645–652. doi:10.1021/cc020090z
Jouyban, A., Fakhree, M. A. A., and Shayanfar, A. (2010). Review of pharmaceutical applications of N-methyl-2-pyrrolidone. J. Pharm. Pharm. Sci. 13 (4), 524–535. doi:10.18433/j3p306
Kategaonkar, A. H., Pokalwar, R. U., Sonar, S. S., Gawali, V. U., Shingate, B. B., and Shingare, M. S. (2010). Synthesis, in vitro antibacterial and antifungal evaluations of new α-hydroxyphosphonate and new α-acetoxyphosphonate derivatives of tetrazolo [1, 5-a] quinoline. Eur. J. Med. Chem. 45 (3), 1128–1132. doi:10.1016/j.ejmech.2009.12.013
Kato, Y., and Nagao, Y. (2012). Effect of polyvinylpyrrolidone on sperm function and early embryonic development following intracytoplasmic sperm injection in human assisted reproduction. Reproductive Med. Biol. 11 (4), 165–176. doi:10.1007/s12522-012-0126-9
Kim, Y., Sagara, J., and KawAI, A. (1995). Studies on the antiviral activity of protein kinase inhibitors against the replication of vesicular stomatitis virus. Biol. Pharm. Bull. 18 (6), 895–899. doi:10.1248/bpb.18.895
Kiruthika, S. E., Nandakumar, A., and Perumal, P. T. (2014). Synthesis of pyrrolo-/indolo [1, 2-a] quinolines and naphtho [2, 1-b] thiophenes from gem-dibromovinyls and sulphonamides. Org. Lett. 16 (17), 4424–4427. doi:10.1021/ol5019085
Kravchenko, D. V., Kysil, V. M., Tkachenko, S. E., Maliarchouk, S., Okun, I. M., and Ivachtchenko, A. V. (2005a). Pyrrolo [3, 4-c] quinoline-1, 3-diones as potent caspase-3 inhibitors. Synthesis and SAR of 2-substituted 4-methyl-8-(morpholine-4-sulfonyl)-pyrrolo [3, 4-c] quinoline-1, 3-diones. Eur. J. Med. Chem. 40 (12), 1377–1383. doi:10.1016/j.ejmech.2005.07.011
Kravchenko, D. V., Kysil, V. M., Tkachenko, S. E., Maliarchouk, S., Okun, I. M., and Ivachtchenko, A. V. (2005b). Synthesis and caspase-3 inhibitory activity of 8-sulfonyl-1, 3-dioxo-2, 3-dihydro-1H-pyrrolo [3, 4-c] quinolines. Il Fármaco 60 (10), 804–809. doi:10.1016/j.farmac.2005.08.001
Kurhade, S., Konstantinidou, M., Sutanto, F., Kurpiewska, K., Kalinowska-Tłuścik, J., and Dömling, A. (2019). Sequential multicomponent synthesis of 2-(Imidazo[1,5-α]pyridin-1-yl)-1,3,4-Oxadiazoles: Sequential multicomponent synthesis of 2-(Imidazo[1,5-α]pyridin-1-yl)-1,3,4-Oxadiazoles. Eur. J. Org. Chem. 10, 2029–2034. doi:10.1002/ejoc.201801880
Leatham, P. A., Bird, H. A., Wright, V., Seymour, D., and Gordon, A. (1983). A double blind study of antrafenine, naproxen and placebo in osteoarthrosis. Eur. J. rheumatology Inflamm. 6 (2), 209–211.
Li, J., Su, S., Huang, M., Song, B., Li, C., and Jia, X. (2013). Unexpected isocyanide-based cascade cycloaddition reaction with methyleneindolinone. Chem. Commun. 49 (91), 10694–10696. doi:10.1039/c3cc46237d
Lilienkampf, A., Mao, J., Wan, B., Wang, Y., Franzblau, S. G., and Kozikowski, A. P. (2009). Structure− activity relationships for a series of quinoline-based compounds active against replicating and nonreplicating Mycobacterium tuberculosis. J. Med. Chem. 52 (7), 2109–2118. doi:10.1021/jm900003c
Lockshin, R. A., and Zakeri, Z. (2004). Apoptosis, autophagy, and more. Int. J. Biochem. Cell Biol. 36 (12), 2405–2419. doi:10.1016/j.biocel.2004.04.011
Lockshin, R. A., Zakeri, Z., and Tilly, J. L. (1998). When cells die: A comprehensive evaluation of apoptosis and programmed cell death. New Jersey, United States: Wiley-Liss.
Maguire, M. P., Sheets, K. R., McVety, K., Spada, A. P., and Zilberstein, A. (1994). A new series of PDGF receptor tyrosine kinase inhibitors: 3-substituted quinoline derivatives. J. Med. Chem. 37 (14), 2129–2137. doi:10.1021/jm00040a003
Mahamoud, A., Chevalier, J., Davin-Regli, A., Barbe, J., and Pages, J. M. (2006). Quinoline derivatives as promising inhibitors of antibiotic efflux pump in multidrug resistant Enterobacter aerogenes isolates. Curr. drug targets 7 (7), 843–847. doi:10.2174/138945006777709557
Mahboobi, S., Eichhorn, E., Popp, A., Sellmer, A., Elz, S., and Möllmann, U. (2006). 3-Bromo-4-(1H-3-indolyl)-2, 5-dihydro-1H-2, 5-pyrroledione derivatives as new lead compounds for antibacterially active substances. Eur. J. Med. Chem. 41 (2), 176–191. doi:10.1016/j.ejmech.2005.10.006
Mashayekh, K., and Shiri, P. (2019). An overview of recent advances in the applications of click chemistry in the synthesis of bioconjugates with anticancer activities. ChemistrySelect 4 (46), 13459–13478. doi:10.1002/slct.201902362
Meki, A. R. M., Esmail, E. E. D. F., Hussein, A. A., and Hassanein, H. M. (2004). Caspase-3 and heat shock protein-70 in rat liver treated with aflatoxin B1: Effect of melatonin. Toxicon 43 (1), 93–100. doi:10.1016/j.toxicon.2003.10.026
Michael, J. P. (2001). Quinoline, quinazoline and acridone alkaloids (July 1999 to June 2000). Nat. Product. Rep. 18 (5), 543–559. doi:10.1039/b005387m
Michael, J. P. (2002). Quinoline, quinazoline and acridone alkaloids. Nat. Product. Rep. 19 (6), 742–760. doi:10.1039/b104971m
Michael, J. P. (2003). Quinoline, quinazoline and acridone alkaloids. Nat. Product. Rep. 20 (5), 476–493. doi:10.1039/b208140g
Michael, J. P. (2004). Quinoline, quinazoline and acridone alkaloids. Nat. Product. Rep. 21 (5), 650–668. doi:10.1039/b310691h
Michael, J. P. (2005). Quinoline, quinazoline and acridone alkaloids. Nat. Product. Rep. 22 (5), 627–646. doi:10.1039/b413750g
Michael, J. P. (2007). Quinoline, quinazoline and acridone alkaloids. Nat. Product. Rep. 24 (1), 223–246. doi:10.1039/b509528j
Michael, J. P. (2008). Quinoline, quinazoline and acridone alkaloids. Nat. Product. Rep. 25 (1), 166–187. doi:10.1039/b612168n
Mollin, S., Blanck, S., Harms, K., and Meggers, E. (2012). Cyclometalated phenylquinoline rhodium complexes as protein kinase inhibitors. Inorganica Chim. Acta 393, 261–268. doi:10.1016/j.ica.2012.04.035
Mortoni, A., Martinelli, M., Piarulli, U., Regalia, N., and Gagliardi, S. (2004). Microwave-assisted solvent-free synthesis of a quinoline-3, 4-dicarboximide library on inorganic solid supports. Tetrahedron Lett. 45 (35), 6623–6627. doi:10.1002/chin.200450111
Muruganantham, N., Sivakumar, R., Anbalagan, N., Gunasekaran, V., and Leonard, J. T. (2004). Synthesis, anticonvulsant and antihypertensive activities of 8-substituted quinoline derivatives. Biol. Pharm. Bull. 27 (10), 1683–1687. doi:10.1248/bpb.27.1683
Nasveld, P., and Kitchener, S. (2005). Treatment of acute vivax malaria with tafenoquine. Trans. R. Soc. Trop. Med. Hyg. 99 (1), 2–5. doi:10.1016/j.trstmh.2004.01.013
Okun, I., Malarchuk, S., Dubrovskaya, E., Khvat, A., Tkachenko, S., Kysil, V., et al. (2006a). Screening for caspase-3 inhibitors: Effect of a reducing agent on identified hit chemotypes. J. Biomol. Screen. 11 (6), 694–703. doi:10.1177/1087057106289231
Okun, I., Malarchuk, S., Dubrovskaya, E., Khvat, A., Tkachenko, S., Kysil, V., et al. (2006b). Screening for caspase-3 inhibitors: A new class of potent small-molecule inhibitors of caspase-3. SLAS Discov. 11 (3), 277–285. doi:10.1177/1087057105285048
Patel, D. B., Parmar, J. A., Patel, S. S., Naik, U. J., and Patel, H. D. (2021). Recent advances in ester synthesis by multi-component reactions (MCRs): A review. Curr. Org. Chem. 25 (5), 539–553. doi:10.2174/1385272825666210111111805
Porter, A. G., and Jänicke, R. U. (1999). Emerging roles of caspase-3 in apoptosis. Cell. death Differ. 6 (2), 99–104. doi:10.1038/sj.cdd.4400476
Rezvanian, A., Heravi, M. M., Shaabani, Z., and Tajbakhsh, M. (2017). Five-component synthesis of dihydropyridines based on diketene. Tetrahedron 73 (15), 2009–2013. doi:10.1016/j.tet.2017.02.027
Rezvanian, A., Alinaghian, F., and Heravi, M. M. (2018a). Metal-free assemblage of four C− N and two C− C bonds via a cascade five component diastereoselective synthesis of pyrido [1, 2-a] pyrimidines. ChemistrySelect 3 (41), 11565–11568. doi:10.1002/slct.201802481
Rezvanian, A., Alinaghian, F., and Heravi, M. M. (2018b). Metal-free assemblage of four C− N and two C− C bonds via a cascade five component diastereoselective synthesis of pyrido [1, 2-a] pyrimidines. ChemistrySelect 3 (41), 11565–11568. doi:10.1002/slct.201802481
Rezvanian, A., Babashah, M., and Anafcheh, M. (2019). A novel pseudo six-component synthesis of functionalized pyrazoles in ethanol by cascade reaction. Mol. Divers. 23 (4), 875–883. doi:10.1007/s11030-018-9908-2
Rezvanian, A., Moradi, F., Zadsirjan, V., Mohammadnejad, M., and Heravi, M. M. (2020a). Cascade process for direct synthesis of indeno [1, 2-b] furans and indeno [1, 2-b] pyrroles from diketene and ninhydrin. Mol. Divers. 24 (4), 1313–1325. doi:10.1007/s11030-019-09996-7
Rezvanian, A., Moradi, F., Zadsirjan, V., Mohammadnejad, M., and Heravi, M. M. (2020b). Cascade process for direct synthesis of indeno [1, 2-b] furans and indeno [1, 2-b] pyrroles from diketene and ninhydrin. Mol. Divers. 24 (4), 1313–1325. doi:10.1007/s11030-019-09996-7
Rezvanian, A., Moradi, F., Zadsirjan, V., Mohammadnejad, M., and Heravi, M. M. (2020c). Cascade process for direct synthesis of indeno [1, 2-b] furans and indeno [1, 2-b] pyrroles from diketene and ninhydrin. Mol. Divers. 24 (4), 1313–1325. doi:10.1007/s11030-019-09996-7
Rezvanian, A., Mahmoodi, F., Zadsirjan, V., Salimi, M., and Heravi, M. M. (2020d). Efficient and uncatalyzed synthesis of highly functionalized new symmetrical indeno [1, 2-b] pyrroles via a one-pot four-component reaction. ChemistrySelect 5 (12), 3503–3507. doi:10.1002/slct.201904860
Rezvanian, A., Noorakhtar, F., Ziarani, G. M., and Mahajer, F. (2020e). Quinoline conjugated imidazopyridine and pyridopyrimidine synthesis in water as highly selective fluoride sensors via a catalyst-free four-component reaction. Monatsh. für Chemie-Chemical Mon. 151 (10), 1581–1589. doi:10.1007/s00706-020-02681-8
Rezvanian, A., Kuhzadeh, P., and Roosta, A. (2021a). Synthesis of novel 1, 3-cyclohexadiene derivatives bearing 2-oxo-quinoline moiety via a 4-CR strategy. ChemistrySelect 6 (45), 12965–12969. doi:10.1002/slct.202103240
Rezvanian, A., Amoozadkhalili, F., and Roosta, A. (2021b). Sequential four-component protocol for the synthesis of pyrido [1, 2-a] pyrimidin-6-one derivatives in water. Chem. Pap. 75 (6), 2417–2424. doi:10.1007/s11696-020-01450-5
Rezvanian, A. (2015). Iodine catalyzed mild 4CR protocol for synthesis of tetrahydroimidazo [1, 2-a] pyridines: Cascade construction of multiple C–C and C–hetero bonds. Tetrahedron 71 (29), 4752–4756. doi:10.1016/j.tet.2015.05.062
Rezvanian, A. (2016). An expedient synthesis strategy to the 1, 4-dihydropyridines and pyrido [1, 2-a] quinoxalines: Iodine catalyzed one-pot four-component domino reactions. Tetrahedron 72 (41), 6428–6435. doi:10.1016/j.tet.2016.08.049
Russ, T. H., Pramanik, A., Khansari, M. E., Wong, B. M., and Hossain, A. (2012). A quinoline based bis-urea receptor for anions: A selective receptor for hydrogen sulfate. Nat. Product. Commun. 7 (3), 1934578X1200700–304. doi:10.1177/1934578x1200700307
Scott, C. W., Sobotka-Briner, C., Wilkins, D. E., Jacobs, R. T., Folmer, J. J., Frazee, W. J., et al. (2003). Novel small molecule inhibitors of caspase-3 block cellular and biochemical features of apoptosis. J. Pharmacol. Exp. Ther. 304 (1), 433–440. doi:10.1124/jpet.102.039651
Sedighian, H., Mamaghani, M. B., Notash, B., and Bazgir, A. (2021). Iodide-catalyzed selenium-assisted sequential multicomponent synthesis of a luminescence benzo-oxazino-isoindole framework. J. Org. Chem. 86 (3), 2244–2253. doi:10.1021/acs.joc.0c02391
Segawa, Y., Tsuzuike, N., Itokazu, Y., Omata, T., Inoue, N., Nagasawa, M., et al. (2001). Effects of a novel hepatoprotective drug, ZNC-2381, on Fas-induced hepatocellular caspase-3 activity and apoptosis in mice. Pharmacology 62 (2), 80–86. doi:10.1159/000056075
Segura-Cabrera, A., Guo, X., Rojo-Domínguez, A., and Rodríguez-Pérez, M. A. (2011). Integrative computational protocol for the discovery of inhibitors of the Helicobacter pylori nickel response regulator (NikR). J. Mol. Model. 17 (12), 3075–3084. doi:10.1007/s00894-011-0962-2
Sharma, S., Ravichandran, V., Jain, P. K., Mourya, V. K., and Agrawal, R. K. (2008). Prediction of caspase-3 inhibitory activity of 1, 3-dioxo-4-methyl-2, 3-dihydro-1h-pyrrolo [3, 4-c] quinolines: QSAR study. J. Enzyme Inhibition Med. Chem. 23 (3), 424–431. doi:10.1080/14756360701652476
Sharma, K., Das, B., and Gogoi, P. (2018). Synthesis of pyrrolo [3, 4-c] quinoline-1, 3-diones: A sequential oxidative annulation followed by dehydrogenation and N-demethylation strategy. New J. Chem. 42 (23), 18894–18905. doi:10.1039/c8nj04443k
Shiri, P., and Aboonajmi, J. (2020). A systematic review on silica-carbon-and magnetic materials-supported copper species as efficient heterogeneous nanocatalysts in “click” reactions. Beilstein J. Org. Chem. 16 (1), 551–586. doi:10.3762/bjoc.16.52
Shiri, P., Niknam, E., Aboonajmi, J., Khalafi-Nezhad, A., and Amani, A. M. (2022). A quick access to structurally diverse triazoloquinazoline heterocycles via the MIL-101 (Cr)-Catalyzed one-pot multi-component reaction of a series of benzaldehydes, dimedone, and 1H-1, 2, 4-triazol-3-amine under green conditions. Front. Chem. 10, 898658. doi:10.3389/fchem.2022.898658
Shukla, S. P., Tiwari, R. K., and Verma, A. K. (2012). Palladium-catalyzed sonogashira-coupling conjoined C–H activation: A regioselective tandem strategy to access indolo-and pyrrolo [1, 2-a] quinolines. J. Org. Chem. 77 (22), 10382–10392. doi:10.1021/jo302112a
Slater, M. J., Cockerill, G. S., and Robinson, J. E. (1995). Preparation of antiviral heterocyclylindole derivatives PCT WO 95/07,910. Chem. Abstr. 123, 256682.
Strekowski, L., Mokrosz, J. L., Honkan, V. A., Czarny, A., Cegla, M. T., Wydra, R. L., et al. (1991). Synthesis and quantitative structure-activity relationship analysis of 2-(aryl or heteroaryl) quinolin-4-amines, a new class of anti-HIV-1 agents. J. Med. Chem. 34 (5), 1739–1746. doi:10.1021/jm00109a031
Sun, J., Zhu, H., Yang, Z. M., and Zhu, H. L. (2013). Synthesis, molecular modeling and biological evaluation of 2-aminomethyl-5-(quinolin-2-yl)-1, 3, 4-oxadiazole-2 (3H)-thione quinolone derivatives as novel anticancer agent. Eur. J. Med. Chem. 60, 23–28. doi:10.1016/j.ejmech.2012.11.039
Talaei, B., Heravi, M. M., Oskooie, H. A., and Rezvanian, A. (2018). An approach to the diastereoselective synthesis of cyclohexane-1, 3-dicarboxamide derivatives via a pseudo five-component reaction based on diketene. Synlett 29 (02), 225–229. doi:10.1055/s-0036-1590980
Thomas, J. W., and Tallman, J. F. (1981). Characterization of photoaffinity labeling of benzodiazepine binding sites. J. Biol. Chem. 256 (19), 9838–9842. doi:10.1016/s0021-9258(19)68705-3
Tseng, C. H., Chen, Y. L., Chung, K. Y., Cheng, C. M., Wang, C. H., and Tzeng, C. C. (2009). Synthesis and antiproliferative evaluation of 6-arylindeno [1, 2-c] quinoline derivatives. Bioorg. Med. Chem. 17 (21), 7465–7476. doi:10.1016/j.bmc.2009.09.021
Tseng, C. H., Tzeng, C. C., Yang, C. L., Lu, P. J., Chen, H. L., Li, H. Y., et al. (2010). Synthesis and antiproliferative evaluation of certain indeno [1, 2-c] quinoline derivatives. Part 2. J. Med. Chem. 53 (16), 6164–6179. doi:10.1021/jm1005447
Valeriote, F. A., Tenney, K., Pietraszkiewicz, H., Edelstein, M., Johnson, T. A., Amagata, T., et al. (2012). Discovery and development of anticancer agents from marine sponges: Perspectives based on a chemistry-experimental therapeutics collaborative program. J. Exp. Ther. Oncol. 10 (2), 119–134.
Wald, K. M., Nada, A. A., Szilágyi, G., and Wamhoff, H. (1980). Photochemie von Heterocyclen, 9. Zur anellierenden Photosubstitution von Dibrom-N-methylmaleinimid (DBMI). Chem. Berichte 113 (9), 2884–2890. doi:10.1002/cber.19801130907
Wilson, W. D., Zhao, M., Patterson, S. E., Wydra, R. L., Janda, L., Strekowski, L., et al. (1992). Design of RNA interactive anti-HIV-1 agents: Unfused aromatic intercalators. ChemInform 23 (44), 1739–1746.
Xia, L., Idhayadhulla, A., Lee, Y. R., Kim, S. H., and Wee, Y. J. (2014). Microwave-assisted synthesis of diverse pyrrolo [3, 4-c] quinoline-1, 3-diones and their antibacterial activities. ACS Comb. Sci. 16 (7), 333–341. doi:10.1021/co500002s
Yavari, I., and Safaei, M. (2020). Synthesis of functionalized γ-spiroiminolactones from isocyanides, acetylenic esters, and cyclopenta [a] acenaphthylen-8-ones. Synth. Commun. 50 (14), 2156–2162. doi:10.1080/00397911.2020.1765389
Keywords: pyrrolo[3,4-c]quinolones, pyrazole, diketene, heterocycles, green solvent, multicomponent reaction
Citation: Rezvanian A and Esfandsar Z (2023) Pyrazole-promoted synthesis of pyrrolo[3,4-c] quinoline-1,3-diones in a novel diketene-based reaction. Front. Chem. 11:1219986. doi: 10.3389/fchem.2023.1219986
Received: 09 May 2023; Accepted: 08 September 2023;
Published: 26 September 2023.
Edited by:
Guigen Li, Texas Tech University, United StatesReviewed by:
Bubun Banerjee, Akal University, IndiaS. Hassan Hosseini, University of Science and Technology of Mazandaran, Iran
Yanrong Li, Boston College, United States
Copyright © 2023 Rezvanian and Esfandsar. This is an open-access article distributed under the terms of the Creative Commons Attribution License (CC BY). The use, distribution or reproduction in other forums is permitted, provided the original author(s) and the copyright owner(s) are credited and that the original publication in this journal is cited, in accordance with accepted academic practice. No use, distribution or reproduction is permitted which does not comply with these terms.
*Correspondence: Atieh Rezvanian, cmV6dmFuaWFuYUBhbHphaHJhLmFjLmly