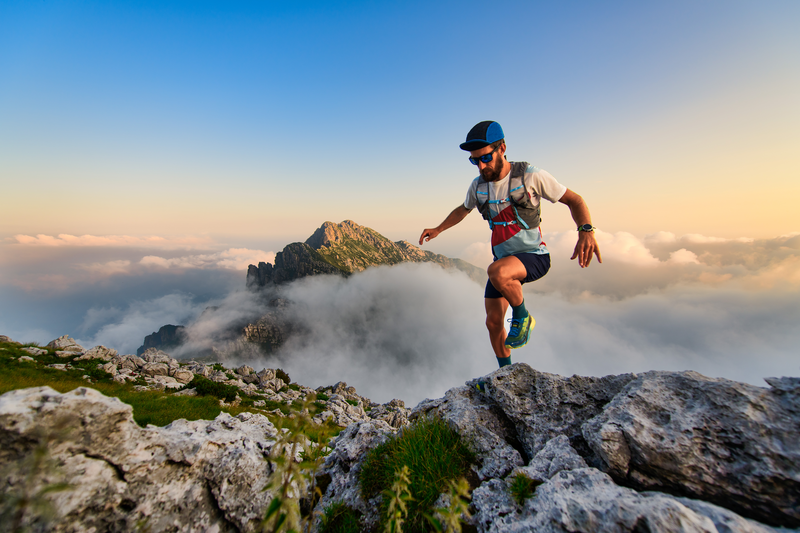
94% of researchers rate our articles as excellent or good
Learn more about the work of our research integrity team to safeguard the quality of each article we publish.
Find out more
REVIEW article
Front. Chem. , 01 November 2023
Sec. Chemical Biology
Volume 11 - 2023 | https://doi.org/10.3389/fchem.2023.1199799
This article is part of the Research Topic Bioactive Natural Products for Health: Isolation, Structural Elucidation, Biological Evaluation, Structure-activity Relationship, and Mechanism View all 10 articles
Rutaecarpine (RUT) is a natural pentacyclic indolopyridoquinazolinone alkaloid first isolated from one of the most famous traditional Chinese herbs, Evodia rutaecarpa, which is used for treating a variety of ailments, including headaches, gastrointestinal disorders, postpartum hemorrhage, amenorrhea, difficult menstruation, and other diseases. Accumulating pharmacological studies showed that RUT possesses a wide range of pharmacological effects through different mechanisms. However, its poor physicochemical properties and moderate biological activities have hampered its clinical application. In this regard, the modification of RUT aimed at seeking its derivatives with better physicochemical properties and more potency has been extensively studied. These derivatives exhibit diverse pharmacological activities, including anti-inflammatory, anti-atherogenic, anti-Alzheimer’s disease, antitumor, and antifungal activities via a variety of mechanisms, such as inhibiting cyclooxygenase-2 (COX-2), acetylcholine (AChE), phosphodiesterase 4B (PDE4B), phosphodiesterase 5 (PDE5), or topoisomerases (Topos). From this perspective, this paper provides a comprehensive description of RUT derivatives by focusing on their diverse biological activities. This review aims to give an insight into the biological activities of RUT derivatives and encourage further exploration of RUT.
Rutaecarpine [8,13-dihydroindolo (2',3': 3,4) pyrido (2,1-b) quinazolin-5 (7H)-one, RUT, 1, Figure 1] is a pentacyclic indolopyridoquinazolinone first isolated by Asahina and Kashiwaki from Evodia rutaecarpa (Qiu, 2012), which is one of the most frequently used traditional Chinese herbs for treating diverse ailments, including headaches, gastrointestinal disorders, postpartum hemorrhage, amenorrhea, difficult menstruation, and other diseases (Lee et al., 2008; Liao et al., 2011). As one of the most abundant compounds in Evodia rutaecarpa, RUT has also been demonstrated to possess a broad spectrum of intriguing pharmacological activities, including anti-inflammation (Moon et al., 1999), anti-platelet (Sheen et al., 1996; Sheu et al., 1996), vasodilatory (Chiou et al., 1994; Li et al., 2021), analgesic (Zhang et al., 2017; Zhang et al., 2020), cytotoxic (Byun et al., 2022; Chan et al., 2022), anti-AD (Zhao et al., 2021), and anti-obesity activities (Kim et al., 2009), as well as anti-diabetic potential (Surbala et al., 2020; Xie et al., 2020). Nevertheless, RUT is still unsuitable for direct clinical application due to its poor water solubility, moderate potency, or cytotoxicity (Lee et al., 2017; Luo et al., 2020). From this perspective, the modification of RUT aimed at seeking its derivatives with better physicochemical properties (water solubility, oral bioavailability, etc.), more potency, and less cytotoxicity to normal tissues has been extensively explored.
In the past few years, there have been several reviews on RUT. In 2008, Lee et al. provided a review on the isolation, synthesis, structure–activity relationship (SAR) studies, pharmacological activities, and metabolism of RUT (Lee et al., 2008). Two years later, Jia et al. summarized its pharmacological effects as a cardiovascular protective agent (Jia and Hu, 2010). In 2015, Son et al. gave an updated review of RUT on its synthesis, biological activities, and SAR of RUT derivatives (Son et al., 2015). In 2019, Tian et al. gave an updated review of RUT as a promising cardiovascular protective agent (Tian et al., 2019). Recently, Li et al. comprehensively summarized the recent progress of RUT as a promising hepatoprotective agent (Li et al., 2020). These aforementioned reviews were either limited to RUT itself (its derivatives were not described) or to one type of pharmacological activity (such as cardiovascular protective activity or hepatoprotective activity), and recent studies were not included.
Previously, a comprehensive description of the derivatives of evodamine (EVO, 2, a congener of RUT, which is also one of the most bioactive alkaloids isolated from Evodia rutaecarpa) was conducted by our group (Li et al. 2022). In this study, we focus on the broad spectrum of intriguing biological activities of RUT derivatives, aiming to provide a reference for further studies of RUT (Figure 2).
Alcoholic liver disease (ALD) represents the main type of chronic liver disease in the world (Seitz et al., 2018). It has been reported that cyclooxygenase-2 (COX-2) plays a vital role in the pathogenesis of ALD (Dunn and Shah, 2016). Enhanced expression of COX-2 can be seen in ALD (Nanji et al., 1997), and downregulating COX-2 can reduce inflammation and oxidative stress (Deol et al., 2018). Hence, an inhibitor of COX-2 may be an appropriate choice for the treatment of ALD.
Previously, Lee and coworkers demonstrated that 10-fluoro-2-methoxyrutaecarpine (3) (Figure 3) retained anti-inflammatory activity both in vitro and in vivo (Lee et al., 2019). Based on the results, they further found that 3 exhibited better COX-2 suppression activity and lower cytotoxicity compared to its parent RUT as a COX-2 selective inhibitor.
Another team, Xu and coworkers, introduced a sulfonyl group to RUT to improve the anti-inflammatory effect and obtained a novel analog termed 3-B-RUT (4) (Figure 3) (Xu et al., 2021). Then, they explored its protective effect on alcoholic liver injury (ALI) in vivo and in vitro. The results showed that 4 (20 μg/kg) attenuated ALI and suppressed oxidative stress and liver inflammation, and the effect was comparable to that of RUT (20 mg/kg). Mechanistically, the results of Western blot showed that administration of 4 inhibited the phosphorylation of P65 and IκBα (silencing COX2 also achieves this effect), leading to suppressing the transfer of P65 into the nucleus. As is known, NF-κB commonly refers to the dimer protein formed by the P65/P50 subunits, and it controls DNA transcription, cytokine production, cell survival, and other important cell events; it specifically plays a key role in regulating immune development, immune responses, oxidative stress, and inflammation (Mitchell et al., 2016). Therefore, 4 might suppress oxidative stress and inflammation by regulating the COX-2/NF-κB pathway. The increased anti-inflammatory activity leading to a lower concentration may overcome the undesirable side effects, such as in gastrointestinal or heart diseases. Therefore, 4 was considered to be a promising clinical candidate for the treatment of ALI.
Acute kidney injury (AKI) is a clinical syndrome caused by multiple factors, including ischemic–reperfusion injury, sepsis, and drug toxicity (such as cisplatin). Similarly, inflammation and oxidative stress have been shown to play critical roles in the pathogenesis of AKI (Liu et al., 2020).
Liu et al. found that phosphodiesterase 4B (PDE4B), a key enzyme modulator in immune and inflammation-related diseases, was significantly upregulated in the serum of AKI patients and participated in the progression of AKI (Liu et al., 2020). Therefore, they synthesized and obtained a series of 3-aromatic sulphonamide-substituted RUT derivatives, and compound 5 (Figure 4) exhibited the best renoprotective and anti-inflammatory activity (Liu et al., 2020). Using target prediction (performed by Discovery Studio 2017 software), molecular docking (pdb code: 5O0J), and cellular thermal shift assay (CETSA), they identified PDE4B as the target of 5. Therefore, 5 might serve as a therapeutic candidate for AKI via the PDE4B pathway.
Atherosclerosis is a chronic disease featuring lipid deposition and an inflammatory response (Libby et al., 2011). It is well known that nitric oxide (NO) and tumor necrosis factor (TNF)-α play vital roles in inflammation (Kaltenmeier et al., 2022). Furthermore, the NLRP3 inflammasome, a protein complex associated with the inflammatory progression of atherosclerosis, has attracted much interest in the pathogenesis of atherosclerosis (Abderrazak et al., 2015; Guo et al., 2015; Grebe et al., 2018). Therefore, compounds with reduced effects on NO, TNF-α, or NLRP3 that alleviate vascular inflammation would be beneficial for anti-atherogenic progression.
Previously, Lee et al. (2017) reported that compound 3 could also inhibit the production of NO and TNF-α in lipopolysaccharide (LPS)-stimulated RAW264.7 macrophages. In addition, no cytotoxicity was observed toward RAW264.7 macrophages at a concentration of 20 µM. Although Luo et al. first demonstrated that 5-deoxy-rutaecarpine (R3, 6) (Figure 5), a novel derivative of RUT, could alleviate atherosclerotic burden in Apoe−/− mice fed a high-fat diet, pharmacokinetics studies showed that 6 had better oral bioavailability (F% = 27.89) than RUT (F% = 0.07) in male rats via gastric gavage at a dosage of 20 mg/kg. Subsequently, they explored its anti-atherosclerotic mechanisms (Luo et al., 2020). The results showed that 6 inhibited NLRP3 inflammasome activation through NF-κB, and the MAPK signaling pathway blocked atherosclerotic progression via debilitating NLRP3 inflammasome-related inflammation.
Based on the aforementioned information, we found that i) modification of the D-ring of RUT, especially the introduction of a sulfonyl group to the D-ring of RUT, seemed beneficial to enhance its anti-inflammatory activity; and ii) removal of the carbonyl group from the C-ring of RUT may improve its oral bioavailability (Figure 6).
Alzheimer’s disease (AD) is an incurable and long-term neurodegenerative brain disorder, and its pathogenic mechanism is still not fully understood. Currently, many hypotheses, including low levels of acetylcholine (AChE) and butyrylcholinesterase (BChE), aggregation of tau protein, and deposition of amyloid β-protein (Aβ) plaques, etc., have been proposed to explain the pathophysiology of AD (Wilson et al., 2012). Currently, the development of anti-AD drugs mainly focuses on reducing the levels of AChE and/or BChE, Aβ, and tau proteins.
Although 6 was demonstrated to exhibit anti-atherogenic activity, it is worth noting that it was first synthesized by Decker in early 2005, and its cholinesterase inhibitory activity was evaluated (Decker, 2005). The results showed that 6 exhibited moderate inhibitory activity toward both AChE and BChE with IC50 values of 3.4 μM and 0.5 μM, respectively.
In 2010, the research group of Huang synthesized a series of novel RUT derivatives and evaluated their AChE inhibitory activity (Wang et al., 2010). Most of the derivatives were found to be selective for AChE over BChE. In particular, compound 7 showed the highest inhibitory activity toward AChE and also demonstrated the highest selectivity index (SI) over BChE. However, it showed a cytotoxic effect on SH-SY5Y cells. Considering that the dehydrorutaecarpine moiety had better potency than derivatives with the RUT moiety, they reported another series of dehydrorutaecarpine derivatives (He et al., 2013). All these synthetic compounds, particularly 8, showed higher inhibitory activity against AChE, Aβ aggregation, and oxidative stress compared to RUT. Notably, 8 showed a lower cytotoxic effect on SH-SY5Y cells (Figure 7).
Another research group, Li et al. (2013), reported a series of novel 2-(2-indolyl-)-4 (3H)-quinazoline (2IQ, 9) derivatives, which were regarded as the ring-opened analogs of RUT. Subsequently, they evaluated their pharmacological activity against AChE. Among them, 10 possessed the strongest inhibitory activity toward AChE with high selectivity over BChE (Figure 8).
Phosphodiesterases (PDEs), being responsible for hydrolyzing two second messengers, cyclic GMP (cGMP) and cyclic AMP (cAMP), can affect neuronal cell survival (Tsunada et al., 2021). Thus, PDEs play important roles in neurodegenerative diseases, including AD (Zuccarello et al., 2020). Among the different PDEs, PDE5 specifically hydrolyzes cGMP. Recently, an upregulated PDE5 expression has been found in the brains of mild AD patients, and studies have confirmed that PDE5 inhibitors exhibit therapeutic effects on AD patients by improving learning and memory, and reversing cognitive impairment (Boccia et al., 2011; Hosseini-Sharifabad et al., 2012; Al-Amin et al., 2014). Therefore, several researchers are developing anti-AD drugs by reducing PDE5 levels.
Considering RUT containing a quinoline structure, which has been demonstrated to be a privileged structure for the PDE5 inhibitor (Bi et al., 2004), Huang et al. decided to use RUT as the lead compound to develop PDE5 inhibitors for the treatment of AD (Huang et al., 2020). Thus, a series of RUT derivatives were synthesized, and their PDE5 inhibitory activities were tested. As a result, 11 showed the most potent PDE5 inhibitory activity with an IC50 value of 0.086 μM. Moreover, it also exhibited good effects against scopolamine-induced cognitive impairment in vivo. These results might provide significant instruction for further developing PDE5 inhibitors derived from RUT (Figure 9).
It is well known that topoisomerases are important targets in tumor therapy, and their inhibitors, including camptothecin, irinotecan, topotecan, etc., are widely used in clinic applications. In 2006, Xu et al. revealed that RUT itself did not show any significant activity against topoisomerases I and II (Topo I and Topo II) despite its significant cytotoxicity on several cancer cell lines (Xu et al., 2006). However, Kim et al. found that two derivatives of RUT (12 and 13) not only exhibited cytotoxicity against selected human cancer cell lines (GI50 values of 5 μM, 3 μM, and 3 μM for 15b against U251, SKOV3, and DU145 cell lines, respectively; GI50 values of 2.5 μM, 2 μM, and 2 μM for 15c against U251, SKOV3, and DU145 cell lines, respectively) (Baruah et al., 2004) but also showed inhibitory activities against Topo I and Topo II (Kim et al., 2012) (Figure 10).
Huang et al. synthesized two sets of RUT derivatives with amine groups to improve their solubility (Huang et al., 2017). The results showed that the solubility of the compounds with the amine moieties connected to the indole-N atom (14) was improved significantly. Moreover, cell viability on HeLa cells and reduction of metabolic activity were in the same range or better for these derivatives compared to the chemically unaltered parent compounds (Figure 11).
To explore the potential applications of RUT and its derivatives against phytopathogenic fungi (Botrytis cinerea, Fusarium graminearum, Fusarium oxysporum, Magnaporthe oryzae, Rhizoctonia solani, and Sclerotinia sclerotiorum), various kinds of RUT derivatives were designed and synthesized by Yang et al. (2022), and their antifungal abilities against the aforementioned six phytopathogenic fungi were evaluated. The results showed that imidazole derivatives of RUT (A1, 15) exhibited broad-spectrum inhibitory activities against B. cinerea, F. graminearum, F. oxysporum, M. oryzae, R. solani, and S. sclerotiorum, with EC50 values of 5.97 mg/mL, 12.72 mg/mL, 16.58 mg/mL, 1.97 mg/mL, and 2.87 mg/mL, respectively. However, RUT showed no activity against these six phytopathogenic fungi (the inhibition rate was 0% at 100 mg/mL). Moreover, the curative effects of 15 against S. sclerotiorum were 94.7%, 81.5%, 80.8%, and 65.0% at different concentrations of 400 mg/mL, 200 mg/mL, 100 mg/mL, and 50 mg/mL, respectively, in in vivo experiments, which was far more effective than the positive drug azoxystrobin. Notably, no phytotoxicity of 15 on oilseed rape leaves was observed even at a high concentration of 400 mg/mL. Therefore, 15 was considered a novel leading compound for the development of antifungal agents (Figure 12).
In summary, the diverse biological activities of RUT derivatives have been presented in this review. Undoubtedly, RUT and its derivatives exhibit diverse pharmacological activities, indicating that this class of alkaloids shows great potential in medicinal chemistry (Figure 13). Although there are currently no RUT-based drugs available in the market, research and development of RUT and its derivatives should be continued.
Nevertheless, the future directions of RUT and its derivatives could be summarized as follows: i) more studies should be carried out to clarify the similarities and differences between RUT and EVO. Both of them contain a pentacyclic scaffold, and their derivatives possess diverse pharmacological activities, including antitumor, anti-AD, and antifungal activities. However, based on the studies, it seems that RUT derivatives show better activity against inflammation and cardiovascular disease, while EVO derivatives show better activity against cancer. Zhang and coworkers explored the activity of the main bioactive indoloquinazoline alkaloids isolated from Euodia rutaecarpa on the aryl hydrocarbon receptor (AHR) (Zhang et al., 2018). The results showed that RUT was more efficient than EVO. These findings revealed that the methyl substitute at the N-14 atom was a key factor affecting AHR activation. However, similar studies addressing the underlying reasons for their different biological activities are rare. ii) The underlying reasons for one compound exhibiting different biological activities should be reinforced, as observed in the case of compounds 3 and 4. In other words, detailed mechanism research related to the activity should be reinforced. iii) The structural modification of RUT to obtain a variety of sufficient derivatives still needs to be carried out: on one hand, to improve its physicochemical properties or selectivity, and on the other hand, to broaden the scope of compounds with diverse pharmacological activities. Moreover, SARs between different biological activities need to be summarized. iv) How to increase the bioavailability for clinical practice should be given more attention. Most of the compounds introduced in this article have not reached clinical trials; so, their physiological functions have not been clinically confirmed, which could mislead or cause bad effects.
DL and ZH prepared the first version of the manuscript. DL, XX, and YL critically reviewed the final version. All authors contributed to the article and approved the submitted version.
This project was supported by the Research Foundation of Jiangxi Provincial Health and Family Planning Commission (grant no. 202310841) and doctoral research start-up funds for doctors in The First Affiliated Hospital of Gannan Medical University (grant no. QD202219).
The authors declare that the research was conducted in the absence of any commercial or financial relationships that could be construed as a potential conflict of interest.
All claims expressed in this article are solely those of the authors and do not necessarily represent those of their affiliated organizations, or those of the publisher, the editors, and the reviewers. Any product that may be evaluated in this article, or claim that may be made by its manufacturer, is not guaranteed or endorsed by the publisher.
AChE, acetylcholine; AD, Alzheimer’s disease; AKI, acute kidney injury; ALI, alcoholic liver disease; Aβ, amyloid β-protein; BChE, butyrylcholinesterase; CETSA, cellular thermal shift assay; COX-2, cyclooxygenase-2; EVO, evodamine; GI50, 50% growth inhibition concentration; IC50, 50% inhibition concentration; LPS, lipopolysaccharide; NLRP3, NOD-, LRR-, and pyrin domain-containing protein 3; NO, nitric oxide; PDE, phosphodiesterase; RUT, rutaecarpine; SAR, structure–activity relationship; SI, selectivity index; TNF-α, tumor necrosis factor-α; Topo, topoisomerase.
Abderrazak, A., Couchie, D., Mahmood, D., Elhage, R., Vindis, C., Laffargue, M., et al. (2015). Anti-inflammatory and antiatherogenic effects of the NLRP3 inflammasome inhibitor arglabin in ApoE2.Ki mice fed a high-fat diet. Circulation 131, 1061–1070. doi:10.1161/CIRCULATIONAHA.114.013730
Al-Amin, M., Hasan, S., Alam, T., Hasan, A., Hossain, I., Didar, R., et al. (2014). Tadalafil enhances working memory, and reduces hippocampal oxidative stress in both young and aged mice. Eur. J. Pharmacol. 745, 84–90. doi:10.1016/j.ejphar.2014.10.026
Baruah, B., Dasu, K., Vaitilingam, B., Mamnoor, P., Venkata, P. P., Rajagopal, S., et al. (2004). Synthesis and cytotoxic activity of novel quinazolino-beta-carboline-5-one derivatives. Bioorg Med. Chem. 12, 1991–1994. doi:10.1016/j.bmc.2004.03.005
Bi, Y., Stoy, P., Adam, L., He, B., Krupinski, J., Normandin, D., et al. (2004). Quinolines as extremely potent and selective PDE5 inhibitors as potential agents for treatment of erectile dysfunction. Bioorg Med. Chem. Lett. 14, 1577–1580. doi:10.1016/j.bmcl.2003.12.090
Boccia, M., Blake, M., Krawczyk, M., and Baratti, C. (2011). Sildenafil, a selective phosphodiesterase type 5 inhibitor, enhances memory reconsolidation of an inhibitory avoidance task in mice. Behav. Brain Res. 220, 319–324. doi:10.1016/j.bbr.2011.02.016
Byun, W., Bae, E., Kim, W., and Lee, S. (2022). Antitumor activity of rutaecarpine in human colorectal cancer cells by suppression of wnt/β-catenin signaling. J. Nat. Prod. 85, 1407–1418. doi:10.1021/acs.jnatprod.2c00224
Chan, S., Sun, R., Tu, X., Guo, M., Yuan, Q., Yu, Z., et al. (2022). Rutaecarpine suppresses the proliferation and metastasis of colon cancer cells by regulating the STAT3 signaling. J. Cancer 13, 847–857. doi:10.7150/jca.66177
Chiou, W., Chou, C., Liao, J., Sham, A., and Chen, C. (1994). The mechanism of the vasodilator effect of rutaecarpine, an alkaloid isolated from Evodia rutaecarpa. Eur. J. Pharmacol. 257, 59–66. doi:10.1016/0014-2999(94)90694-7
Decker, M. (2005). Novel inhibitors of acetyl- and butyrylcholinesterase derived from the alkaloids dehydroevodiamine and rutaecarpine. Eur. J. Med. Chem. 40, 305–313. doi:10.1016/j.ejmech.2004.12.003
Deol, P., Khare, P., Bishnoi, M., Kondepudi, K., and Kaur, I. (2018). Coadministration of ginger extract-Lactobacillus acidophilus (cobiotic) reduces gut inflammation and oxidative stress via downregulation of COX-2, i-NOS, and c-Myc. Phytother. Res. 32, 1950–1956. doi:10.1002/ptr.6121
Dunn, W., and Shah, V. (2016). Pathogenesis of alcoholic liver disease. Clin. Liver Dis. 20, 445–456. doi:10.1016/j.cld.2016.02.004
Grebe, A., Hoss, F., and Latz, E. (2018). NLRP3 inflammasome and the IL-1 pathway in atherosclerosis. Circ. Res. 122, 1722–1740. doi:10.1161/CIRCRESAHA.118.311362
Guo, H., Callaway, J., and Ting, J. (2015). Inflammasomes: mechanism of action, role in disease, and therapeutics. Nat. Med. 21, 677–687. doi:10.1038/nm.3893
He, Y., Yao, P. F., Chen, S. B., Huang, Z. H., Huang, S. L., Tan, J. H., et al. (2013). Synthesis and evaluation of 7,8-dehydrorutaecarpine derivatives as potential multifunctional agents for the treatment of Alzheimer's disease. Eur. J. Med. Chem. 63, 299–312. doi:10.1016/j.ejmech.2013.02.014
Hosseini-Sharifabad, A., Ghahremani, M., Sabzevari, O., Naghdi, N., Abdollahi, M., Beyer, C., et al. (2012). Effects of protein kinase A and G inhibitors on hippocampal cholinergic markers expressions in rolipram- and sildenafil-induced spatial memory improvement. Pharmacol. Biochem. Behav. 101, 311–319. doi:10.1016/j.pbb.2012.01.017
Huang, G., Drakopoulos, A., Saedtler, M., Zou, H., Meinel, L., Heilmann, J., et al. (2017). Cytotoxic properties of the alkaloid rutaecarpine and its oligocyclic derivatives and chemical modifications to enhance water-solubility. Bioorg Med. Chem. Lett. 27, 4937–4941. doi:10.1016/j.bmcl.2017.08.045
Huang, X. F., Dong, Y. H., Wang, J. H., Ke, H. M., Song, G. Q., and Xu, D. F. (2020). Novel PDE5 inhibitors derived from rutaecarpine for the treatment of Alzheimer's disease. Bioorg Med. Chem. Lett. 30, 127097. doi:10.1016/j.bmcl.2020.127097
Jia, S., and Hu, C. (2010). Pharmacological effects of rutaecarpine as a cardiovascular protective agent. Molecules 15, 1873–1881. doi:10.3390/molecules15031873
Kaltenmeier, C., Wang, R., Popp, B., Geller, D., Tohme, S., and Yazdani, H. O. (2022). Role of immuno-inflammatory signals in liver ischemia-reperfusion injury. Cells 11 (14), 2222. doi:10.3390/cells11142222
Kim, S. I., Lee, S. H., Lee, E. S., Lee, C. S., and Jahng, Y. (2012). New topoisomerases inhibitors: synthesis of rutaecarpine derivatives and their inhibitory activity against topoisomerases. Arch. Pharm. Res. 35, 785–789. doi:10.1007/s12272-012-0504-1
Kim, S., Lee, S., Lee, S., Chae, S., Han, M., Mar, W., et al. (2009). Rutecarpine ameliorates bodyweight gain through the inhibition of orexigenic neuropeptides NPY and AgRP in mice. Biochem. Biophys. Res. Commun. 389, 437–442. doi:10.1016/j.bbrc.2009.08.161
Lee, S. H., Son, J. K., Jeong, B. S., Jeong, T. C., Chang, H. W., Lee, E. S., et al. (2008). Progress in the studies on rutaecarpine. Molecules 13, 272–300. doi:10.3390/molecules13020272
Lee, C., Liao, J., Chen, S., Yen, C., Lee, Y., Huang, S., et al. (2019). Fluorine-modified rutaecarpine exerts cyclooxygenase-2 inhibition and anti-inflammatory effects in lungs. Front. Pharmacol. 10, 91. doi:10.3389/fphar.2019.00091
Lee, C. M., Gu, J. A., Rau, T. G., Wang, C., Yen, C. H., Huang, S. H., et al. (2017). Synthetic fluororutaecarpine inhibits inflammatory stimuli and activates endothelial transient receptor potential vanilloid-type 1. Molecules 22, 656. doi:10.3390/molecules22040656
Li, D., Li, Y., Jiang, X., Liu, W., and Zhao, Q. (2022). Evodiamine: a privileged structure with broad-ranging biological activities. Mini Rev. Med. Chem. 22, 2680–2701. doi:10.2174/1389557522666220404090835
Li, S., Huang, B., Zhou, C., Shi, J., Wu, Q., and Jiang, Q. (2021). Rutaecarpine ameliorates pressure overload cardiac hypertrophy by suppression of calcineurin and angiotensin II. Evid. Based Complement. Altern. Med. 2021, 1–8. doi:10.1155/2021/8857329
Li, X., Ge, J., Zheng, Q., Zhang, J., Sun, R., and Liu, R. (2020). Evodiamine and rutaecarpine from Tetradium ruticarpum in the treatment of liver diseases. Phytomedicine 68, 153180. doi:10.1016/j.phymed.2020.153180
Li, Z., Wang, B., Hou, J. Q., Huang, S. L., Ou, T. M., Tan, J. H., et al. (2013). 2-(2-indolyl-)-4(3H)-quinazolines derivates as new inhibitors of AChE: design, synthesis, biological evaluation and molecular modelling. J. Enzyme Inhib. Med. Chem. 28, 583–592. doi:10.3109/14756366.2012.663363
Liao, J., Chiou, W., Shen, Y., Wang, G., and Chen, C. (2011). Anti-inflammatory and anti-infectious effects of Evodia rutaecarpa (Wuzhuyu) and its major bioactive components. Chin. Med. 6, 6. doi:10.1186/1749-8546-6-6
Libby, P., Ridker, P., and Hansson, G. (2011). Progress and challenges in translating the biology of atherosclerosis. Nature 473, 317–325. doi:10.1038/nature10146
Liu, X. Q., Jin, J., Li, Z., Jiang, L., Dong, Y. H., Cai, Y. T., et al. (2020). Rutaecarpine derivative Cpd-6c alleviates acute kidney injury by targeting PDE4B, a key enzyme mediating inflammation in cisplatin nephropathy. Biochem. Pharmacol. 180, 114132. doi:10.1016/j.bcp.2020.114132
Luo, J., Wang, X., Jiang, X., Liu, C., Li, Y., Han, X., et al. (2020). Rutaecarpine derivative R3 attenuates atherosclerosis via inhibiting NLRP3 inflammasome-related inflammation and modulating cholesterol transport. FASEB J. 34, 1398–1411. doi:10.1096/fj.201900903RRR
Mitchell, S., Vargas, J., and Hoffmann, A. (2016). Signaling via the NF-κB system. Wiley Interdiscip. Rev. Syst. Biol. Med. 8 (3), 227–241. doi:10.1002/wsbm.1331
Moon, T., Murakami, M., Kudo, I., Son, K., Kim, H., Kang, S., et al. (1999). A new class of COX-2 inhibitor, rutaecarpine from Evodia rutaecarpa. Inflamm. Res. 48, 621–625. doi:10.1007/s000110050512
Nanji, A., Miao, L., Thomas, P., Rahemtulla, A., Khwaja, S., Zhao, S., et al. (1997). Enhanced cyclooxygenase-2 gene expression in alcoholic liver disease in the rat. Gastroenterology 112, 943–951. doi:10.1053/gast.1997.v112.pm9041257
Qiu, M. (2012). Chemical constituents of the fruits of Evodia rutaecarpa. Sci. Technol. Inf. 1, 651–652.
Seitz, H. K., Bataller, R., Cortes-Pinto, H., Gao, B., Gual, A., Lackner, C., et al. (2018). Alcoholic liver disease. Nat. Rev. Dis. Prim. 4 (1), 16. doi:10.1038/s41572-018-0014-7
Sheen, W., Tsai, I., Teng, C., Ko, F., and Chen, I. (1996). Indolopyridoquinazoline alkaloids with antiplatelet aggregation activity from Zanthoxylum integrifoliolum. Planta medica. 62, 175–176. doi:10.1055/s-2006-957846
Sheu, J., Hung, W., Lee, Y., and Yen, M. (1996). Mechanism of inhibition of platelet aggregation by rutaecarpine, an alkaloid isolated from Evodia rutaecarpa. Eur. J. Pharmacol. 318, 469–475. doi:10.1016/s0014-2999(96)00789-3
Son, J. K., Chang, H. W., and Jahng, Y. (2015). Progress in studies on rutaecarpine. II.--Synthesis and structure-biological activity relationships. Molecules 20, 10800–10821. doi:10.3390/molecules200610800
Surbala, L., Singh, C., Devi, R., and Singh, O. (2020). Rutaecarpine exhibits anti-diabetic potential in high fat diet-multiple low dose streptozotocin induced type 2 diabetic mice and in vitro by modulating hepatic glucose homeostasis. J. Pharmacol. Sci. 143, 307–314. doi:10.1016/j.jphs.2020.04.008
Tian, K. M., Li, J. J., and Xu, S. W. (2019). Rutaecarpine: a promising cardiovascular protective alkaloid from Evodia rutaecarpa (Wu Zhu Yu). Pharmacol. Res. 141, 541–550. doi:10.1016/j.phrs.2018.12.019
Tsunada, S., Sugiura, M., and Kandori, H. (2021). Molecular properties and optogenetic applications of enzymerhodopsins. Adv. Exp. Med. Biol. 1293, 153–165. doi:10.1007/978-981-15-8763-4_9
Wang, B., Mai, Y. C., Li, Y., Hou, J. Q., Huang, S. L., Ou, T. M., et al. (2010). Synthesis and evaluation of novel rutaecarpine derivatives and related alkaloids derivatives as selective acetylcholinesterase inhibitors. Eur. J. Med. Chem. 45, 1415–1423. doi:10.1016/j.ejmech.2009.12.044
Wilson, R., Segawa, E., Boyle, P., Anagnos, S., Hizel, L., and Bennett, D. (2012). The natural history of cognitive decline in Alzheimer's disease. Psychol. Aging 27, 1008–1017. doi:10.1037/a0029857
Xie, J., Wang, S. Y., Ma, P., Ma, F., Li, J., Wang, W., et al. (2020). Selection of small molecules that bind to and activate the insulin receptor from a DNA-encoded library of natural products. iScience 23, 101197. doi:10.1016/j.isci.2020.101197
Xu, J. J., Li, H. D., Wu, M. F., Zhu, L., Du, X. S., Li, J. J., et al. (2021). 3-B-RUT, a derivative of RUT, protected against alcohol-induced liver injury by attenuating inflammation and oxidative stress. Int. Immunopharmacol. 95, 107471. doi:10.1016/j.intimp.2021.107471
Xu, M., Li, G., Moon, D., Lee, C., Woo, M., Lee, E., et al. (2006). Cytotoxicity and DNA topoisomerase inhibitory activity of constituents isolated from the fruits of Evodia officinalis. Arch. Pharm. Res. 29, 541–547. doi:10.1007/BF02969262
Yang, C. J., Li, H. X., Wang, J. R., Zhang, Z. J., Wu, T. L., Liu, Y. Q., et al. (2022). Design, synthesis and biological evaluation of novel evodiamine and rutaecarpine derivatives against phytopathogenic fungi. Eur. J. Med. Chem. 227, 113937. doi:10.1016/j.ejmech.2021.113937
Zhang, W., Chen, X., Wu, C., Lian, Y., Wang, Y., Wang, J., et al. (2020). Evodiamine reduced peripheral hypersensitivity on the mouse with nerve injury or inflammation. Mol. Pain 16, 174480692090256. doi:10.1177/1744806920902563
Zhang, Y., Li, Z., Zhang, K., Zhang, H., He, Z., Xia, Q., et al. (2017). Co-delivery of evodiamine and rutaecarpine in a microemulsion-based hyaluronic acid hydrogel for enhanced analgesic effects on mouse pain models. Int. J. Pharm. 528, 100–106. doi:10.1016/j.ijpharm.2017.05.064
Zhang, Y., Yan, T., Sun, D., Xie, C., Zheng, Y., Zhang, L., et al. (2018). Structure-activity relationships of the main bioactive constituents of Euodia rutaecarpa on aryl hydrocarbon receptor activation and associated bile acid homeostasis. Drug Metab. Dispos. 46, 1030–1040. doi:10.1124/dmd.117.080176
Zhao, B., Wang, Y., Liu, R., Jia, X., Hu, N., An, X., et al. (2021). Rutaecarpine ameliorated high sucrose-induced alzheimer's disease like pathological and cognitive impairments in mice. Rejuvenation Res. 24, 181–190. doi:10.1089/rej.2020.2349
Keywords: rutaecarpine, alkaloid, natural products, bioactivity, evodiamine, β-carboline
Citation: Li D, Huang Z, Xu X and Li Y (2023) Promising derivatives of rutaecarpine with diverse pharmacological activities. Front. Chem. 11:1199799. doi: 10.3389/fchem.2023.1199799
Received: 04 April 2023; Accepted: 16 October 2023;
Published: 01 November 2023.
Edited by:
Rongrui Wei, Jiangxi University of Traditional Chinese Medicine, ChinaReviewed by:
Hongtao Xu, ShanghaiTech University, ChinaCopyright © 2023 Li, Huang, Xu and Li. This is an open-access article distributed under the terms of the Creative Commons Attribution License (CC BY). The use, distribution or reproduction in other forums is permitted, provided the original author(s) and the copyright owner(s) are credited and that the original publication in this journal is cited, in accordance with accepted academic practice. No use, distribution or reproduction is permitted which does not comply with these terms.
*Correspondence: Xiaojun Xu, Z254eGo2NzA5QDE2My5jb20=; Yan Li, bGl5YW42NzA5QDE2My5jb20=
Disclaimer: All claims expressed in this article are solely those of the authors and do not necessarily represent those of their affiliated organizations, or those of the publisher, the editors and the reviewers. Any product that may be evaluated in this article or claim that may be made by its manufacturer is not guaranteed or endorsed by the publisher.
Research integrity at Frontiers
Learn more about the work of our research integrity team to safeguard the quality of each article we publish.