- 1Department of Medical Services and Techniques, Vocational School of Health Services, Mardin Artuklu University, Mardin, Türkiye
- 2Department of Food Technology, Vocational School of Technical Sciences, Batman University, Batman, Türkiye
- 3Department of Biochemistry, Faculty of Science, Ege University, Izmir, Türkiye
- 4Department of Biophysics and Biochemistry, Baku State University, Baku, Azerbaijan
- 5Department of Biology and Ecology, Faculty of Natural sciences, Lankaran State University, Lankaran, Azerbaijan
- 6Educational and Scientific Institute of Neurosurgery, Peoples’ Friendship University of Russia (RUDN University), Moscow, Russia
- 7Department of Neurosurgery, Sechenov First Moscow State Medical University (Sechenov University), Moscow, Russia
- 8Department of Obstetrics and Gynecology, Tyumen State Medical University, Tyumen, Russia
- 9Central Research Laboratory, Bashkir State Medical University, Ufa, Bashkortostan, Russia
The eco-friendly synthesis of metallic nanoparticles (MNPs) using biological materials is an encouraging and innovativeness approach to nanotechnology. Among other synthesizing methods, biological methods are chosen because of their high efficiency and purity in many aspects. In this work, using the aqueous extract obtained from the green leaves of the D. kaki L. (DK); silver nanoparticles were synthesized in a short time and simply with an eco-friendly approach. The properties of the synthesized silver nanoparticles (AgNPs) were characterized using various techniques and measurements. In the characterization data of AgNPs, Maximum absorbance at 453.34 nm wavelengths, the average size distribution of 27.12 nm, the surface charge of −22.4 mV, and spherical appearance were observed. LC-ESI-MS/MS analysis was used to assess the compound composition of D. kaki leaf extract. The chemical profiling of the crude extract of D. kaki leaves revealed the presence of a variety of phytochemicals, predominantly phenolics, resulting in the identification of five major high-feature compounds: two major phenolic acids (Chlorogenic acid and Cynarin), and tree flavonol glucosides (hyperoside, quercetin-3-glucoside, and quercetin-3- D-xyloside). The components with the highest concentrations were cynarin, chlorogenic acid, quercetin-3- D-xyloside, hyperoside, and quercetin-3-glucoside, respectively. Antimicrobial results were determined by a MIC assay. The biosynthesized AgNPs exhibited strong antibacterial activity against the human and food pathogen Gram (+ and −) bacteria and good antifungal activity against pathogenic yeast. It was determined that 0.03–0.050 μg/mL concentrations ranges of DK-AgNPs were growth suppressive concentrations on all pathogen microorganisms. The MTT technique was used to study the cytotoxic effects of produced AgNPs on cancer cell lines (Glioblastoma (U118), Human Colorectal Adenocarcinoma (Caco-2), Human Ovarian Sarcoma (Skov-3) cancer cell lines, and Human Dermal Fibroblast (HDF) healthy cell line). It has been observed that they have a suppressive effect on the proliferation of cancerous cell lines. After 48 h of treatment with Ag-NPs, the DK-AgNPs were found to be extremely cytotoxic to the CaCo-2 cell line, inhibiting cell viability by up to 59.49% at a concentration of 50 g mL−1. It was found that the viability was inversely related to the DK-AgNP concentration. The biosynthesized AgNPs had dose-dependent anticancer efficacy. Because of the high concentration of bioactive chemicals in Diospyros kaki, it may be employed as a biological resource in medicinal applications. DK-AgNPs were shown to be an effective antibacterial agent as well as a prospective anticancer agent. The results provide a potential approach for the biogenic production of DK-AgNPs utilizing D. kaki aqueous leaf extract.
Introduction
Green nanotechnology, which is included in nanotechnology, has recently attracted great attention with the elements it reveals at the molecular level. These products continue to be a developing field of study with their use in the treatment of various diseases in medicine and many areas (Ramazanli and Ahmadov, 2022). Nanoparticles (NPs) have superior properties such as physical and chemical. In addition to the large surface areas of NPs, their resistance to high-temperature changes are among their superior features (Syafiuddin et al., 2017). In particular, nanometals such as gold (Au), zinc (Zn), silver (Ag), palladium (Pd), and titanium (Ti) is used in areas such as drug delivery systems, biological labeling, optical devices, and some processes such as the synthesis and stability of metal nanoparticles (NPs) attract great attention in this field (Emmanuel et al., 2015). In addition, NPs are valuable materials with uses in biomedical applications, cosmetics, and food industries, bioremediation studies, etc. (Khalilov, 2023; Arroyo et al., 2020). Different methods are used to obtain metallic NPs. Among these methods, synthesis by biological methods has some advantages. Among these are advantages such as being eco-friendly, nominal energy, and cost, as it does not contain toxic chemicals in the synthesis stages (Singh et al., 2018). Long before antibiotics were brought into contemporary medicine, silver was used to cure infected wounds. However, because of its high toxicity and the availability of antibiotics, the use of silver is restricted. Recently, silver nanoparticles (AgNPs) have piqued the interest of scientists due to their potential application as antibacterial agents (Gunashova, 2022). Because of their broad bactericidal and fungicidal spectrum, they have a wide range of uses in medicine, including medicines, cosmetics, and medical devices. The most pressing public health issues for physicians have been antibiotic resistance and anti-cancer agents that may be administered at low dosages to inhibit malignant cell proliferation. As a result, research into employing nanotechnology to improve medication delivery has begun (Hawar et al., 2022). There are many studies using plant sources (leaf, flower, root, fruit, or whole plant) to obtain silver nanoparticles (AgNPs) with biological approaches (Namburi et al., 2021). AgNPs obtained in synthesis studies with plant sources do not require special conditions and are synthesized with an eco-friendly approach, as well as the fact that the synthesis process is easy, and cheap and the amount of product is higher, among the factors that increase the interest in biological approaches (Rather et al., 2022). Plants are the finest alternatives for finding novel anti-cancer chemicals that have fewer side effects and are more affordable. Infectious diseases, cancer, pyrexia, algesia, and inflammation can all be treated using a variety of therapeutic substances that can be found in medicinal plants (Majid et al., 2022a; Majid et al., 2022b). Phenolic compounds, alcohols, flavonoids, and phytochemicals containing carboxyl groups in the extract obtained from plant sources are compounds that form silver nanoparticles by reducing the plus-valent silver in the aqueous structure and also have an effect on stability (Srikar et al., 2016). AgNPs are used in medical applications as antimicrobial (Rather et al., 2022) and anticancer (Salman et al., 2022) agents, in cosmetics, bioremediation studies (Rani et al., 2020), in the food industry (Velmurugan et al., 2014), and in many other areas. Diospyros kaki L. (Persimmon of Paradise) plant, which has about 400 members of the Ebenaceae family, is distributed throughout Asia, Africa, and Central and South America. It is an economically valuable plant. The leaves of the plant have medicinal uses with their positive effect in the treatment of paralysis, skin burns, stopping bleeding, and frostbite. It is chemical composition is rich in variable bioactive components such as phenolic compounds, flavonoid oligomers, tannins, ascorbic acid, and caffeine (Çifçi et al., 2021). In this work, AgNPs were produced utilizing the aqueous extract of D. kaki leaf. AgNPs that were produced in the plant-based green method were assessed for two biological activities. Two Gram-positive, two Gram-negative, and one fungus were used in the current study to evaluate the antibacterial properties of biogenic AgNPs. Four cell lines were employed in the study to determine cytotoxicity. By using LCMS-MS to analyze the chemical composition of the leaf extract, it was possible to identify probable biologically active groups. The novelty of the present study is that it successfully highlighted the antimicrobial and cytotoxic potential of AgNPs synthesized for the first time using D. kaki leaf aqueous extract using different cell lines, with abundant evidence (Figure 1).
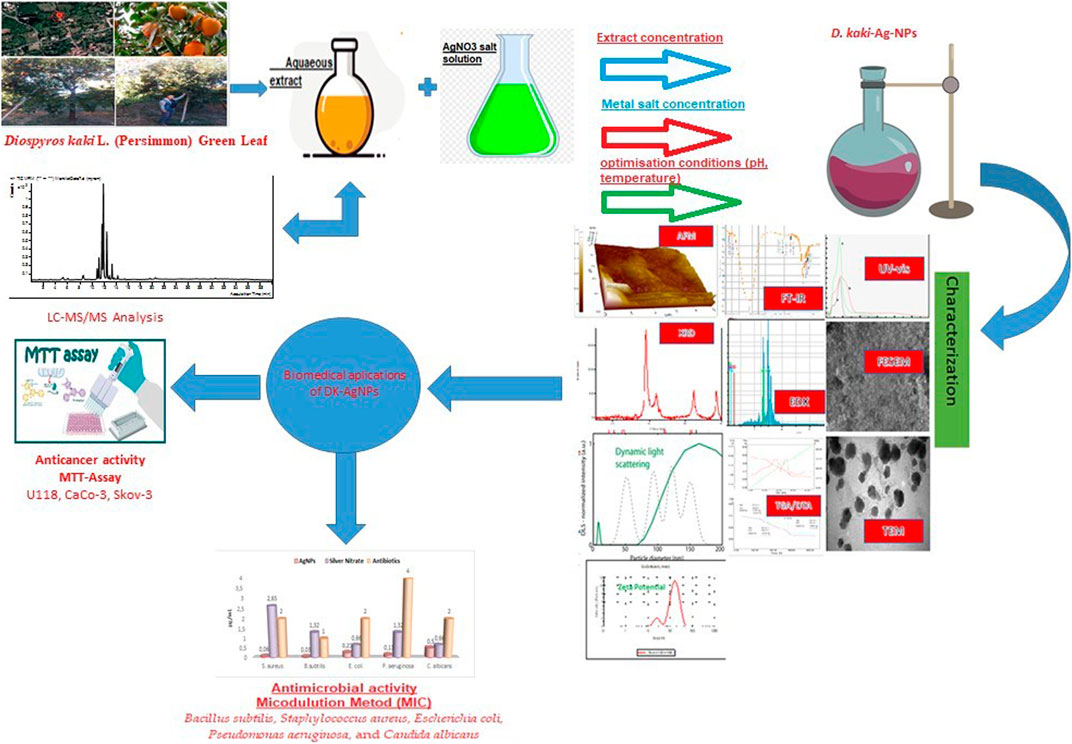
FIGURE 1. Schematic representation of synthesis, characterization, and biomedical applications of silver nanoparticles synthesized using D. kaki leaf aqueous extract.
Materials and methods
Extraction
The leaves of D. kaki (DK) were collected close to shedding in late November. The leaves were washed three times with deionized water to eliminate any pollutants and dirt. The plants were then diced and dried in the open air until they reached a consistent weight. Following that, dried leaves were pulverized into a fine powder in a grinder. 150 grams of the resulting powder were combined with 500 mL of deionized water before being heated at 85°C and swirled at 240 rpm with a magnetic stirrer for around 60 min. The heated solutions were eventually filtered, and the filtrate extracts were kept in a glass beaker at 4.0°C for further use.
Synthesis process of DK-AgNPs
5 mM (millimolar) metal solution was prepared by using silver nitrate (99.8% purity), ACS reagent, ≥99.0% (Sigma Aldrich) for the synthesis of DK-AgNPs. DK the leaf extract and AgNO3 solution (1:4) were mixed at 30°C for synthesis. The colour change was observed in 15 min. Depending on the colour change, samples were taken from the reaction medium.
Characterization process of DK-AgNPs
UV-Vis spectroscopy is an essential method for assessing the synthesis and stability of metal nanoparticles in aqueous solutions. The maximum absorbance value does not change when samples taken at various periods are analyzed, which is the stability criteria (Jabir et al., 2021). Under optimum circumstances, a 50 mL AgNP colloid solution was prepared with 5 mM silver nitrate and centrifuged at 15,000 revolutions per minute for 10 min for Fourier transform infrared (FTIR) spectroscopy analysis. After that, the pellets were resuspended and lyophilized for 24 h. FTIR analysis utilizing (Perkin Elmer Spectrum one) may be used to determine the various functional groups in the generated Ag nanoparticles. It provides information on the structure of a molecule, which is frequently obtained through absorption spectra (Keskin et al., 2022). The crystal patterns and crystal sizes of the particles formed as a result of the synthesis were analyzed using an XRD. Using the results obtained with XRD at 2θ in the range of 20–80, the crystal nano dimensions of AgNPs formed after synthesis was calculated by the Debye-Scherrer formula (Keskin et al., 2022). Morphological appearances of synthesized AgNPs were determined using TEM micrograph images with EVO 40 LEQ SEM, and Jeol Jem 1010 FESEM instruments. Topographic features and phase contrast structure, morphological appearance, and size of DK-AgNPs were determined using the AFM (Park System XE-100 AFM) device. In addition, the data obtained from TEM and SEM images were also used for morphological structure evaluation. Surface charges and size distributions of synthesized DK-AgNPs were measured by Zeta potential distribution (Malvern) device.
Determination of chemical composition of plant extract
To determine the phytochemicals that may be responsible for bioreduction, the phytochemical profile of the extracted content was defined using liquid chromatography-mass spectrometry (LC-MS/MS) and FTIR devices. The results were also used to determine the chemical components of the extract.
Antimicrobial activities of biogenic AgNPs
The suppressive effects of AgNPs synthesized from the extract of DK leaves on the growth of pathogenic microorganism strains were determined according to the minimum inhibition concentration (MIC) value using the microdilution method (Keskin et al., 2022). Gram-positive B. subtilis ATCC 11774 (Bacillus subtilis) and Staphylococcus aureus ATCC 29213 (S. aureus) and Gram-negative P. aeruginosa ATCC27833 (Pseudomonas aeruginosa) and E. coli (Escherichia coli) ATCC25922 bacterial strains and Candida albicans yeast (C. albicans) were used to determine antipathogenic activities. Standard antibiotics (vancomycin, colistin, and fluconazole) used to compare the effects of biogenic nanoparticles were commercially purchased from Sigma Aldrich. Solutions containing AgNPs at 1 μg/mL concentration were prepared and transferred to microplates in appropriate amounts, and AgNPs were dispersed in the medium with a series of micro dilutions. Microorganisms grown on the medium plates were taken and solutions containing microorganisms prepared by the McFarland 0.5 (Emmanuel et al., 2015; Maillard et al., 2018; Nishanthi et al., 2019) turbidity criteria were transferred. Some microplate wells were defined for control steps such as sterilization. After all these procedures, the prepared microplates were incubated at 37°C. It was incubated overnight (24 h) for antimicrobial interaction. The next day, the microplate wells were checked for the proliferation of microorganisms, and the MIC was determined.
Cytotoxic effects of biogenix AgNPs on healthy and cancerous cell lines
Cytotoxic effects of synthesized AgNPs and their suppressive effects on cancer cells and healthy cells were investigated using the MTT method (Khatamifar et al., 2022) at Dicle University Scientific Research Center, Diyarbakır, Turkey. In the experimental study, the effects of AgNPs on Glioblastoma (U118), Human Colorectal Adenocarcinoma (Caco-2), Human Ovarian sarcoma (Skov-3) cancer cell lines, and Human Dermal Fibroblast (HDF) healthy cell lines were studied. In 75 t-flasks of Dulbecco’s Modified Eagle (DMEM) medium, cell lines HDF, Caco-2, and U118 were cultured. DMEM medium contains pentrep, 2 mM L-Glutamine, and 10% FBS. The Skov-3 cell line was cultured in 75 t-flasks in RPMI media with 100 U/mL penstrep and 10% FBS. The cultured flasks were stored in a 37°C oven with 95% air, 5% CO2, and humidity. The cell lines were then measured using a hemocytometer and resuspended at varying concentrations depending on whether or not they were at 80% confluence. Cell lines were then incubated overnight in 96-well microplates (24 h). After processing, varied doses of AgNPs were introduced to the wells where the cell lines were cultivated, and the oven was set to 37°C for 48 h. After the interval, MTT solution was added to the wells and the microplates were incubated for 3 h. After applying DMSO and waiting 15 min, absorbance measurements of the cells at 540 nm wavelength were collected using a Multi Scan Go, Thermo equipment. The equation below was used to determine AgNPs generated with cell line absorbance values, which inhibit their viability in cell lines (Remya et al., 2015).
Statistical analysis
The obtained data were statically analyzed using an unpaired t-test with GraphPad Prism. The values were presented as the mean ± SD (n = 3) (Ali et al., 2018).
Results and discussion
UV-vis spectrum data of DK-AgNPs
UV-vis spectrum (Figure 2) illustrates the influence of silver nitrate concentration during the creation of silver nanoparticles using D. kaki leaf extract. Brown-colored silver nanoparticles made from 1 mM silver nitrate were found to have a distinctive surface plasmon absorption band at 453 nm. While other concentrations displayed a spacious peak at 453 nm, the 1 mM concentration exhibited a narrow band with enhanced absorbance (Figure 2). When the number of silver ions was raised from 1 to 5 mM, the absorption rate increased. Due to the greater availability of functional groups in the leaf extract at 1 mM concentration, the formation of nanoparticles and size reduction got underway right away. Due to competition between the functional groups in the 10 mL leaf extract and the silver ions, as the substrate concentration was increased, the enormous size and aggregation of nanoparticles occurred. Therefore, a considerable impact of concentration on the production of silver nanoparticles was demonstrated by the optimization investigation. According to the results of this experiment, a concentration of 1 mM silver nitrate was ideal for the creation of nanoparticles. Similar to intensity, an increased concentration of nanoparticles is indicated by increasing intensity. A higher silver nitrate concentration may indicate the creation of bigger nanoparticles.
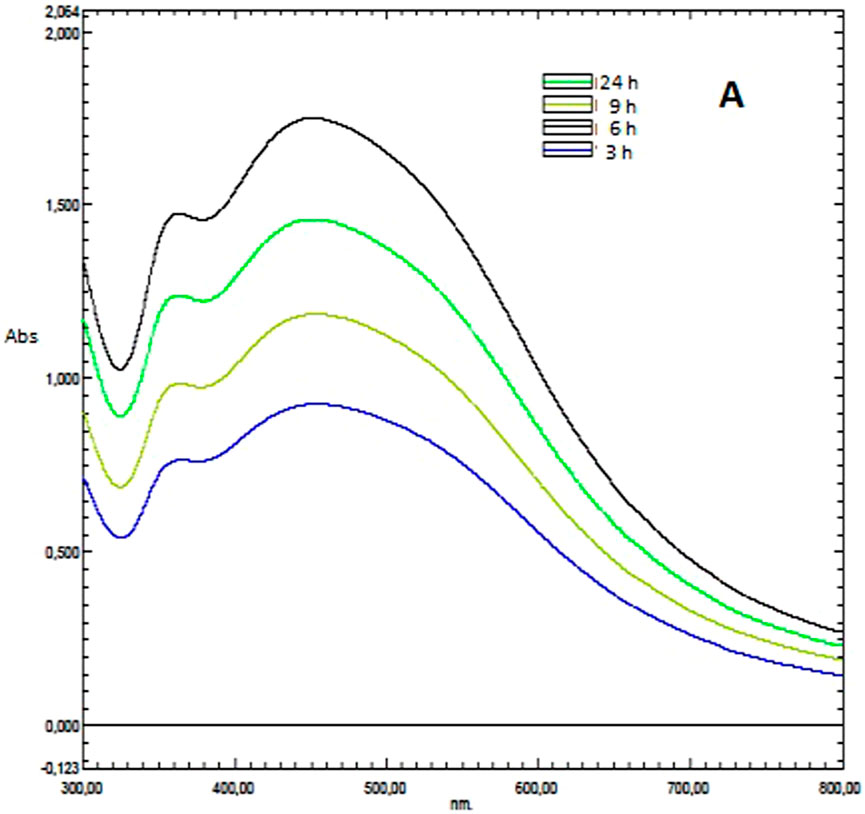
FIGURE 2. (A) Maximum wavelength absorbance bands showing the presence of AgNPs synthesized by leaf extract.
XRD data of DK-AgNPs
The characteristic peaks in the XRD spectra validated and demonstrated the XRD pattern of AgNPs formed from the leaf extract (Figure 3). The spectra taken at 111°, 200°, 220°, and 311° in the analysis data were performed at 2θ to elucidate the crystal structures of the particles synthesized with DK leaf extract and to determine the crystal nano sizes showed that the particles had a cubic (face-centered cubic; JCPDS File No. 04–0783) pattern. Four Bragg’s reflexion patterns at 2θ, that is, 111.00°, 200.00°, 220.00°, 311.00°, and in the whole spectrum of values ranging from 37 to 78, were interpreted from XRD. Using the values of these spectra, the crystal nano dimensions of AgNPs were calculated as 48.90 nm using the Debye-Scherrer equation (Khan et al., 2018). Using this equation, it was stated that the crystal nano dimensions of AgNPs were calculated as 27.30 nm (Some et al., 2019) and 39.37 nm (Baran, 2019) in Eco-Friendly synthesis studies.
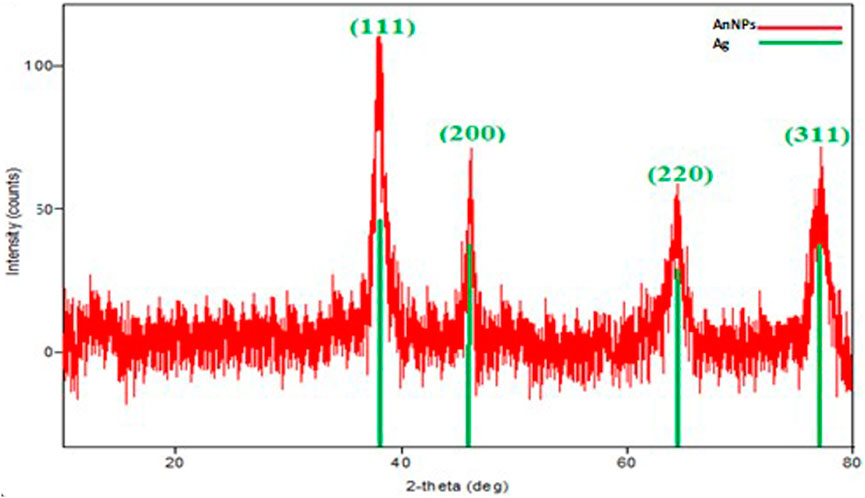
FIGURE 3. Maximum wavelength absorbance bands showing the presence of AgNPs synthesized by leaf extract.
Morphologies of biogenic AgNPs
SEM, FESEM, and TEM micrograph analysis results used to define the morphological structures of the synthesized AgNPs were spherical in appearance and 8.13–38.5 nm in size (Figure 4). In a study, it was reported that AgNPs obtained by mixing Pine, Ginkgo, Persimmon, Magnolia, and Platanus plant extracts were spherical in SEM images (Song and Kim, 2009). In another biosynthesis study, micrographs of the spherical morphological appearance of AgNPs were presented (Hasanzadeh et al., 2021). The same findings were expressed in the FESEM results of AgNPs synthesized in the environmentally friendly synthesis study of Sterculia foetida leaf extract (Premkumar et al., 2018). Previous research, FESEM for Phyto-synthesised silver nanoparticles, strongly validated this evaluation of AgNPs analyzed using the FESEM approach (Khashan et al., 2020). The TEM is used to evaluate the morphological properties of the produced NPs (TEM) (Al-Musawi et al., 2020). From TEM images obtained in a study in which AgNPs were synthesized and characterized (Kumar et al., 2017), it has been reported that nanoparticles have a spherical appearance and dimensions of 12–50 nm. In the EDX analysis to determine the elemental content of the synthesized particles, strong peaks of silver in Figure 4 showed the presence of AgNPs. Analysis using an Energy Dispersive X-ray (EDX) spectrometer verified the occurrence of silver’s elemental signal and its uniform pattern of dispersion of nanoparticles. The horizontal axis showed energy in KeV, whereas the vertical axis showed the quantity of X-ray counts. This confirmed that silver particles were appropriately identified and is present in the solution. Identification lines for the main silver emission energies were displayed, and these coincided with peaks in the spectra. In addition to these strong peaks, the presence of weak peaks such as carbon and oxygen was also due to the phytochemicals in the particles (Khatamifar et al., 2022).

FIGURE 4. AgNPs synthesized by DK extract; (A) SEM, (B) FESEM, (C) TEM micrographs, and (D) EDX Profile.
AFM micrograph of biogenic AgNPs
The topographic distributions and size distributions of AgNPs obtained as a result of the Eco-Friendly method were determined by the results of AFM analysis. As seen in Figure 5, it was seen that The Atomic Force Microscopy (AFM) image depicts monodispersed silver nanoparticles that were created, and it agrees well with the SEM and TEM images. In the AFM results of similar studies, the data showing that AgNPs have a spherical appearance and are below 100 nm were examined (Khashan et al., 2020).
Zeta potential distribution of DK-AgNPs
Zeta potential analysis data were evaluated in determining the surface charges of DK-AgNPs synthesized with leaf extract. As seen in Figure 6, the surface charge distribution of the synthesized AgNPs was found to be −22.4 mV on average. The presence of phytochemicals affects the negative surface charge (Remya et al., 2015). The negative charge distribution of NPs is important for their stability. The formation of different charges causes negative situations such as aggregation and fluctuation with electrostatic interaction (Pugazhendhi et al., 2018). The fact that AgNPs synthesized with DK extract were only negatively charged was the data showing that these NPs were stable. When previous Eco-Friendly synthesis studies were examined, it was seen that the surface charge distributions in the zeta potential results of AgNPs were −18.52 mV (Tian et al., 2022), −13.7 mV (Shoaib et al., 2021), and −43.3 mV (Soltani and Darbemamieh, 2021).
Size distribution of DK-AgNPs
The sizes of AgNPs synthesized with DK extract were defined by zeta size distribution analysis. It was observed that AgNPs exhibited an average size distribution of 27.12 nm below 100 nm (Figure 7). It was stated that AgNPs synthesized with Zataria multiflora extract at pH 9 had a size distribution of 25 nm (Barabadi et al., 2021). It was shown that AgNPs with an average size distribution of 49.04 nm were synthesized using Commiphora molmol extract (Awad et al., 2021).
TGA-DTA data of DK-AgNPs
TGA-DTA analysis was performed to determine the stability and resistance of the synthesized AgNPs against heat treatments. As seen in Table 1 and Figure 8, mass losses occurred at temperatures at three points. The initial mass loss from these points was due to the loss of retained water with 6.94% at 153.44°C. It was observed that the mass losses of 12.48% and 7.67% at the second and third points, respectively, were caused by the phytochemicals (organic compounds) surrounding the AgNPs (Mobin et al., 2022). TGA-DT results of AgNPs are given in some green synthesis studies performed in Table 2.
FTIR analysis
FTIR analysis was used to characterize the potential functional groups involved in the reduction of silver metal ions to silver nanoparticles. Frequency shifts in 3334.66–3314.00 cm−1, 2106.57–2121.85 cm−1, and 1635.47–1635.12 cm−1 in the results of the FTIR analysis performed to evaluate the functional groups participating in the reaction showed that hydroxyl, carboxyl and amine groups may be responsible for the reduction and stabilization, respectively (Figure 9) (Hatipoğlu, 2022). Proteins and flavonoids may have contributed to the quick reduction and capping of silver ions into silver nanoparticles in the current analysis. Strong reducing agents, flavonoids, which can be indicative of the reduction of silver nitrate to generate AgNPs, were detected in the leaf extract. The decrease of Ag+ to Ag0 may be directly attributed to the flavonoid components found in the D. kaki extract.
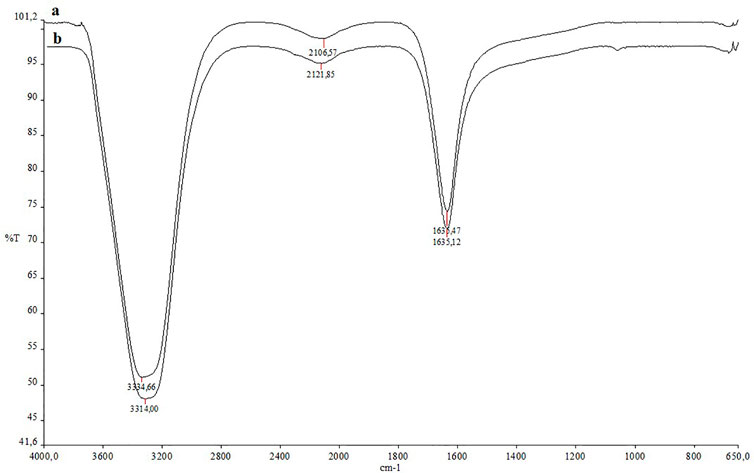
FIGURE 9. (A) FTIR spectroscopy data of the plant extract, (B) FTIR spectroscopy data of reaction liquid with synthesized AgNPs.
Antimicrobial activities
The antimicrobial effects of AgNPs obtained after synthesis was determined by the microdilution method. The efficacy of synthesised DK-AgNPs (1 μg/mL concentration) was comparing with Ag NO3 solution (11.60 μg/mL initial concentration) and antibiotics used as standard (128 μg/mL concentration). As seen in Table 3, concentrations of 0.03 and 0.050 μg mL−1 showed suppression of growth of tested strains. These concentrations of AgNPs showed that they were effective at very low concentrations against antibiotics and AgNO3 solution. In Table 4, the findings of the antimicrobial effect studies obtained in other Eco-Friendly synthesis studies are given to compare with our results.
Cytotoxic effects of DK-AgNPs
The cytotoxic effects of synthesized AgNPs on cell lines were examined using the MTT method. As seen in Table 5, it was determined that 25 μg mL−1 concentration did not have a toxic effect on healthy cell lines, and had an anticancer effect on Caco-2 and skov-3 cancer cell lines, with suppression rates of 44.40% and 18.62%, respectively. It was observed that AgNPs at a concentration of 25 μg mL−1 showed a proliferative effect on U118. Some concentrations may exert a proliferative effect on cancer cell lines (Zhang and Jiang, 2020). It was determined that 50 μg mL−1 concentration of AgNPs did not show any toxic effect in healthy cells and the best anticancer effect of this concentration occurred on the Caco-2 cell line with a suppression rate of 59.49% at 50 μg mL−1 concentration (Table 5). In addition, 50 μg mL−1 concentration of AgNPs synthesized on U118 and Skov-3 cancer cell lines caused a 16.01% and 51.07% suppression in cell viability and proliferation, respectively.

TABLE 5. Viability rates of cell lines after 48 h of the interaction with synthesized AgNPs (n = 3).
The cytotoxic effects of AgNPs synthesized in the previous green synthesis studies on cancer cells using the MTT method are given in Table 5. Some properties of nanoparticles such as concentration, shape, and size have a significant effect on their toxic effect (Swamy et al., 2015). NPs contact cells by electrostatic interaction and cause an increase in the amount of ROS DNA, RNA, and some vital enzymes that have a high affinity for these species (Gopu et al., 2022). In addition, AgNPs cause cell death by activating structures responsible for cell apoptosis (Selvan et al., 2018). Some of the studies that evaluated the cytotoxic activities of silver nanoparticles with similar cell lines are given in Table 6 for comparison.
LC-MS profile of DK leaf extract
Phytochemicals have very important activities such as anticancer, antioxidant, and anti-inflammatory. Phytochemicals such as phenolic compounds and flavonoids are biologically highly active compounds. We take them into our bodies, especially with plant-based foods. LC-MS/MS analysis results for the analysis of phenolic compounds of DK extract were evaluated with the results given in Figure 10 and Table 7. When the compounds with high concentrations in the extract were evaluated, 11210.6471 μg mL−1, 13359.8649 μg mL−1, 3091.3887 μg mL−1, 2100.6615 μg mL−1, and 3546.9109 μg mL−1 concentrations of compounds such as chlorogenic acid, cynarin, hyperoside, quercetin-3-glucoside, quercetin-3-D-xyloside were determined, respectively. These compounds have very important benefits. It is a member of the ester family of chlorogenic acids between quinic acid and trans-hydroxycinnamic acid. It has pharmacological effects such as antioxidant, antispasmodic, inhibition of DNA methyltransferase, and inhibition of carcinogenic components (Jaiswal et al., 2014). Cynarine (1,3-dicaffeoylquinic acid) is formed by the esterification of two units of caffeic acid and one unit of quinic acid. It is a derivative of hydroxycinnamic acid and is a biologically active functional group. It is a compound with pharmacological effects such as antioxidant, anticholinergic, antihistamine, and antibacterial (Topal et al., 2016). Caffeic acid exhibits antifungal activity via inhibiting fungal 1,3-d-glucan synthase (Mohsin et al., 2022). Quercetin-based compounds in the flavonoid group have antiviral effects. In particular, quercetin-3-glucoside has a strong antiviral effect against the influenza virus (Nile et al., 2020).
Conclusion
The use of environmentally friendly technology to create nanoparticles has various benefits, including the process’s simplicity and practicality from an economic standpoint. We have created a quick, environmentally friendly, and practical process for creating silver nanoparticles with a diameter range of 8–38 nm from D. kaki aqueous leaf extract. Changes in AgNPs were seen in UV-vis spectra at 453 nm, as well as time-dependent color changes. The XRD pattern indicated that the sample was made up of elemental silver crystalline face-centred cubic (fcc) lattice structures. The essential biological components responsible for the silver reduction in the FTIR technique were discovered. EDX was used to examine the nanoparticles’ high absorption. The SEM image shows the size, shape, and high density of the nanoparticles. All of these findings indicated that AgNPs produced with Diospyros kaki leaf extract were stable. The presence of significant phenolic compounds in the LC-MS analysis findings for the detection and identification of phytochemicals of extract components indicated that these components were actively involved in the reaction. In MIC tests, the DK-AgNPs demonstrated significant antibacterial activity. The DK-AgNPs also suppressed the activity of treated cell lines, confirming the viability reduction. The creation of application phases is expected to be beneficial to future research, particularly in the quest for anticancer and antibacterial medicines. Finally, because the synthesis procedure is simple and inexpensive, and the raw material is inexpensive, more in vivo tests are required to confirm the results of this work.
Data availability statement
The original contributions presented in the study are included in the article/supplementary material, further inquiries can be directed to the corresponding authors.
Author contributions
CK, conceptualization, study design, supervision, manuscript revision, and final approval of the version to be declaration of competing interest. AÖ, data curation, formal analysis, investigation, and writing—original draft. AB and MB, methodology, visualization, formal analysis, investigation, and writing—original draft. AE, conceptualization, study design, supervision, manuscript revision, SO, formal analysis, methodology, and visualization. RK, resources, writing—original draft, and writing—review and editing. EA, methodology, visualization, formal analysis, data curation, writing—review and editing. AS, methodology, visualization, formal analysis, data curation, and writing—review and editing. AB and IG, methodology, formal analysis, data curation, visualization, writing—original draft, and writing—review and editing. All authors contributed to the article and approved the submitted version.
Funding
This work was supported by the Bashkir State Medical University Strategic Academic Leadership Program (PRIORITY-2030).
Acknowledgments
The authors thank Dr. Sevgi İrtegün Kandemir from Dicle University for her valuable contribution and comments on the manuscript. The authors are thankful to Mardin Artuklu University for providing all necessary research facilities to carry out this research.
Conflict of interest
The authors declare that the research was conducted in the absence of any commercial or financial relationships that could be construed as a potential conflict of interest.
Publisher’s note
All claims expressed in this article are solely those of the authors and do not necessarily represent those of their affiliated organizations, or those of the publisher, the editors and the reviewers. Any product that may be evaluated in this article, or claim that may be made by its manufacturer, is not guaranteed or endorsed by the publisher.
References
Al-Musawi, S., Albukhaty, S., Al-Karagoly, H., Sulaiman, G. M., Jabir, M. S., and Naderi-Manesh, H. (2020). Dextran-coated superparamagnetic nanoparticles modified with folate for targeted drug delivery of camptothecin. Adv. Nat. Sci. Nanosci. Nanotechnol. 11 (4), 045009. doi:10.1088/2043-6254/abc75b
Ali, I. H., Jabir, M. S., Al-Shmgani, H. S., Sulaiman, G. M., and Sadoon, A. H. (2018). Pathological and immunological study on infection with Escherichia coli in ale balb/c mice. Journal of Physics: Conference Series. England: IOP Publishing.
Arroyo, G. V., Madrid, A. T., Gavilanes, A. F., Naranjo, B., Debut, A., Arias, M. T., et al. (2020). Green synthesis of silver nanoparticles for application in cosmetics. J. Environ. Sci. Health, Part A 55 (11), 1304–1320. doi:10.1080/10934529.2020.1790953
Awad, M. A., Al Olayan, E. M., Siddiqui, M. I., Merghani, N. M., Alsaif, S. S. A.-l., and Aloufi, A. S. (2021). Antileishmanial effect of silver nanoparticles: Green synthesis, characterization, in vivo and in vitro assessment. Biomed. Pharmacother. 137, 111294. doi:10.1016/j.biopha.2021.111294
Barabadi, H., Mojab, F., Vahidi, H., Marashi, B., Talank, N., Hosseini, O., et al. (2021). Green synthesis, characterization, antibacterial and biofilm inhibitory activity of silver nanoparticles compared to commercial silver nanoparticles. Inorg. Chem. Commun. 129, 108647. doi:10.1016/j.inoche.2021.108647
Baran, A., Baran, M. F., Keskin, C., Kandemir, S. I., Valiyeva, M., Mehraliyeva, S., et al. (2021). Ecofriendly/rapid synthesis of silver nanoparticles using extract of waste parts of artichoke (Cynara scolymus L) and evaluation of their cytotoxic and antibacterial activities. J. Nanomater. 2021, 1–10. doi:10.1155/2021/2270472
Baran, M. F. (2019). Synthesis, characterization and investigation of antimicrobial activity of silver nanoparticles from Cydonia oblonga leaf, Appl. Ecol. Environ. Res. 17 (2), 2583–2592. doi:10.15666/aeer/1702_25832592
Çiftçi, H., Çalışkan, Ç. E., Öztürk, K., and Yazıcı, B. (2021). Bioactive nanoparticles synthesized by green method. Black Sea J. Eng. Sci. 4 (1), 29–42. doi:10.34248/bsengineering.816084
Ejidike, I. P., and Clayton, H. S. (2022). Green synthesis of silver nanoparticles mediated by daucus carota L.: Antiradical, antimicrobial potentials, in vitro cytotoxicity against brain glioblastoma cells. Green Chem. Lett. Rev. 15 (2), 298–311. doi:10.1080/17518253.2022.2054290
Emmanuel, R., Palanisamy, S., Chen, S.-M., Chelladurai, K., Padmavathy, S., Saravanan, M., et al. (2015). Antimicrobial efficacy of green synthesized drug blended silver nanoparticles against dental caries and periodontal disease causing microorganisms. Mater. Sci. Eng. C 56, 374–379. doi:10.1016/j.msec.2015.06.033
Gopu, C., Chirumamilla, P., Kagithoju, S., and Taduri, S. (2022). Green synthesis of silver nanoparticles using momordica cymbalaria aqueous leaf extracts and screening of their antimicrobial activity: AgNPs studies in momordica cymbalaria. Proc. Natl. Acad. Sci. India Sect. B Biol. Sci. 92 (4), 771–782. doi:10.1007/s40011-022-01367-x
Gunashova, G. Y. (2022). Synthesis of silver nanoparticles using a thermophilic bacterium strain isolated from the spring Yukhari istisu of the Kalbajar region (Azerbaijan). Advances in Biology and Earth Sciences 7 (3), 198–204.
Hasanzadeh, A., Gholipour, B., Rostamnia, S., Eftekhari, A., Tanomand, A., Khaksar, S., et al. (2021). Biosynthesis of AgNPs onto the urea-based periodic mesoporous organosilica (AgxNPs/Ur-PMO) for antibacterial and cell viability assay. J. Colloid Interface Sci. 585, 676–683. doi:10.1016/j.jcis.2020.10.047
Hatipoğlu, A. (2022). Green synthesis of silver nanoparticles and their antimicrobial effects on some food pathogens. Süleyman Demirel Üniversitesi Fen Bilim. Enstitüsü Derg. 26 (1), 106–114. doi:10.19113/sdufenbed.970654
Hawar, S. N., Al-Shmgani, H. S., Al-Kubaisi, Z. A., Sulaiman, G. M., Dewir, Y. H., and Rikisahedew, J. J. (2022). Green synthesis of silver nanoparticles from Alhagi graecorum leaf extract and evaluation of their cytotoxicity and antifungal activity. J. Nanomater. 2022, 1–8. doi:10.1155/2022/1058119
Jabir, M. S., Nayef, U. M., Abdulkadhim, W. K., Taqi, Z. J., Sulaiman, G. M., Sahib, U. I., et al. (2021). Fe 3 O 4 nanoparticles capped with PEG induce apoptosis in breast cancer AMJ13 cells via mitochondrial damage and reduction of NF-κB translocation, J. Inorg. Organomet. Polym. Mater. 31, 1241–1259. doi:10.1007/s10904-020-01791-4
Jaiswal, R., Müller, H., Müller, A., Karar, M. G. E., and Kuhnert, N. (2014). Identification and characterization of chlorogenic acids, chlorogenic acid glycosides and flavonoids from Lonicera henryi L(Caprifoliaceae) leaves by LC–MSn, Phytochemistry 108, 252–263. doi:10.1016/j.phytochem.2014.08.023
Jun, Y.-w., Seo, J.-w., and Cheon, J. (2008). Nanoscaling laws of magnetic nanoparticles and their applicabilities in biomedical sciences. Accounts Chem. Res. 41 (2), 179–189. doi:10.1021/ar700121f
Keskin, C., Baran, A., Baran, M. F., Hatipoğlu, A., Adican, M. T., Atalar, M. N., et al. (2022). Green synthesis, characterization of gold nanomaterials using Gundelia tournefortii leaf extract, and determination of their nanomedicinal (antibacterial, antifungal, and cytotoxic) potential." J. Nanomater. 2022, 1, 10. doi:10.1155/2022/7211066
Khalilov, R. (2023). A comprehensive review of advanced nano-biomaterials in regenerative medicine and drug delivery. Advances in Biology and Earth Sciences 8 (1), 5–18.
Khan, A. U., Yuan, Q., Khan, Z. U. H., Ahmad, A., Khan, F. U., Tahir, K., et al. (2018). An eco-benign synthesis of AgNPs using aqueous extract of Longan fruit peel: Antiproliferative response against human breast cancer cell line MCF-7, antioxidant and photocatalytic deprivation of methylene blue. J. Photochem. Photobiol. B Biol. 183, 367–373. doi:10.1016/j.jphotobiol.2018.05.007
Khashan, K. S., Abdulameer, F. A., Jabir, M. S., Hadi, A. A., and Sulaiman, G. M. (2020). Anticancer activity and toxicity of carbon nanoparticles produced by pulsed laser ablation of graphite in water. Adv. Nat. Sci. Nanosci. Nanotechnol. 11 (3), 035010. doi:10.1088/2043-6254/aba1de
Khatamifar, M., Fatemi, S. J., Torkzadeh-Mahani, M., Mohammadi, M., and Hassanshahian, M. (2022). Green and eco-friendly synthesis of silver nanoparticles by quercus infectoria galls extract: Thermal behavior, antibacterial, antioxidant and anticancer properties. Part. Sci. Technol. 40 (3), 281–289. doi:10.1080/02726351.2021.1941455
Kumar, B., Smita, K., Cumbal, L., and Debut, A. (2017). Green synthesis of silver nanoparticles using Andean blackberry fruit extract. Saudi J. Biol. Sci. 24 (1), 45–50. doi:10.1016/j.sjbs.2015.09.006
Maillard, A. P. F., Dalmasso, P. R., de Mishima, B. A. L., and Hollmann, A. (2018). Interaction of green silver nanoparticles with model membranes: Possible role in the antibacterial activity. Colloids Surfaces B Biointerfaces 171, 320–326. doi:10.1016/j.colsurfb.2018.07.044
Majid, M., Farhan, A., Asad, M. I., Khan, M. R., Hassan, S. S. U., Haq, I. U., et al. (2022a). An extensive pharmacological evaluation of new anti-cancer triterpenoid (nummularic acid) from Ipomoea batatas through in vitro, in silico, and in vivo studies. Molecules 27 (8), 2474. doi:10.3390/molecules27082474
Majid, M., Farhan, A., Baig, M. W., Khan, M. T., Kamal, Y., Hassan, S. S. ul, et al. (2022b). Ameliorative effect of structurally divergent oleanane triterpenoid, 3-epifriedelinol from Ipomoea batatas against BPA-induced gonadotoxicity by targeting PARP and NF-κB signaling in rats. Molecules 28 (1), 290. doi:10.3390/molecules28010290
Mobin, M., Ahmad, I., and Shoeb, M. (2022). Investigation into the highly efficient Artemisia absinthium-silver nanoparticles composite as a novel environmentally benign corrosion inhibitor for mild steel in 1M HCl. J. Adhesion Sci. Technol. 36 (23-24), 2562–2587. doi:10.1080/01694243.2022.2075523
Mohsin, S. A., Shaukat, S., Nawaz, M., Ur-Rehman, T., Irshad, N., Majid, M., et al. (2022). Appraisal of selected ethnomedicinal plants as alternative therapies against onychomycosis: Evaluation of synergy and time-kill kinetics. Front. Pharmacol. 13, 1067697. doi:10.3389/fphar.2022.1067697
Namburi, K. R., Kora, A. J., Chetukuri, A., and Kota, V. S. M. K. (2021). Biogenic silver nanoparticles as an antibacterial agent against bacterial leaf blight causing rice phytopathogen Xanthomonas oryzae pv. oryzae. Bioprocess Biosyst. Eng. 44 (9), 1975–1988. doi:10.1007/s00449-021-02579-7
Nile, S. H., Kim, D. H., Nile, A., Park, G. S., Gansukh, E., and Kai, G. (2020). Probing the effect of quercetin 3-glucoside from Dianthus superbus L against influenza virus infection-in vitro and in silico biochemical and toxicological screening. Food Chem. Toxicol. 135, 110985. doi:10.1016/j.fct.2019.110985
Nishanthi, R., Malathi, S., Palani, P., and P., P. (2019). Green synthesis and characterization of bioinspired silver, gold and platinum nanoparticles and evaluation of their synergistic antibacterial activity after combining with different classes of antibiotics. Mater. Sci. Eng. C 96, 693–707. doi:10.1016/j.msec.2018.11.050
Premkumar, J., Sudhakar, T., Dhakal, A., Shrestha, J. B., Krishnakumar, S., and Balashanmugam, P. (2018). Synthesis of silver nanoparticles (AgNPs) from cinnamon against bacterial pathogens. Biocatal. Agric. Biotechnol. 15, 311–316. doi:10.1016/j.bcab.2018.06.005
Pugazhendhi, S., Palanisamy, P., and Jayavel, R. (2018). Synthesis of highly stable silver nanoparticles through a novel green method using Mirabillis jalapa for antibacterial, nonlinear optical applications. Opt. Mater. 79, 457–463. doi:10.1016/j.optmat.2018.04.017
Ramazanli, V. N., and Ahmadov, I. S. (2022). Synthesis of silver nanoparticles by using extract of olive leaves. Advances in Biology and Earth Sciences 7 (3), 238–244.
Rani, P., Kumar, V., Singh, P. P., Matharu, A. S., Zhang, W., Kim, K.-H., et al. (2020). Highly stable AgNPs prepared via a novel green approach for catalytic and photocatalytic removal of biological and non-biological pollutants. Environ. Int. 143, 105924. doi:10.1016/j.envint.2020.105924
Rather, M. A., Deori, P. J., Gupta, K., Daimary, N., Deka, D., Qureshi, A., et al. (2022). Ecofriendly phytofabrication of silver nanoparticles using aqueous extract of Cuphea carthagenensis and their antioxidant potential and antibacterial activity against clinically important human pathogens. Chemosphere 300, 134497. doi:10.1016/j.chemosphere.2022.134497
Remya, R., Rajasree, S. R., Aranganathan, L., and Suman, T. (2015). An investigation on cytotoxic effect of bioactive AgNPs synthesized using Cassia fistula flower extract on breast cancer cell MCF-7. Biotechnol. Rep. 8, 110–115. doi:10.1016/j.btre.2015.10.004
Salman, G., Pehlivanoglu, S., Aydin Acar, C., and Yesilot, S. (2022). Anticancer effects of Vitis vinifera L. mediated biosynthesized silver nanoparticles and cotreatment with 5 fluorouracil on HT-29 cell line. Biol. Trace Elem. Res. 200 (7), 3159–3170. doi:10.1007/s12011-021-02923-8
Satpathy, S., Patra, A., Ahirwar, B., and Delwar Hussain, M. (2018). Antioxidant and anticancer activities of green synthesized silver nanoparticles using aqueous extract of tubers of Pueraria tuberosa. Artif. cells, Nanomedicine, Biotechnol. 46 (3), 71–85. doi:10.1080/21691401.2018.1489265
Selvan, D. A., Mahendiran, D., Kumar, R. S., and Rahiman, A. K. (2018). Garlic, green tea and turmeric extracts-mediated green synthesis of silver nanoparticles: Phytochemical, antioxidant and in vitro cytotoxicity studies. J. Photochem. Photobiol. B Biol. 180, 243–252. doi:10.1016/j.jphotobiol.2018.02.014
Shoaib, M., Naz, A., Osra, F. A., Abro, S. H., Qazi, S. U., Siddiqui, F. A., et al. (2021). Green synthesis and characterization of silver-entecavir nanoparticles with stability determination. Arabian J. Chem. 14 (3), 102974. doi:10.1016/j.arabjc.2020.102974
Singh, J., Mehta, A., Rawat, M., and Basu, S. (2018). Green synthesis of silver nanoparticles using sun dried tulsi leaves and its catalytic application for 4-Nitrophenol reduction. J. Environ. Chem. Eng. 6 (1), 1468–1474. doi:10.1016/j.jece.2018.01.054
Soltani, L., and Darbemamieh, M. (2021). Biosynthesis of silver nanoparticles using hydroethanolic extract of Cucurbita pepo L. fruit and their anti-proliferative and apoptotic activity against breast cancer cell line (MCF-7), Multidiscip. Cancer Investig. 5 (3), 1–10. doi:10.30699/mci.5.3.525-1
Some, S., Bulut, O., Biswas, K., Kumar, A., Roy, A., Sen, I. K., et al. (2019). Effect of feed supplementation with biosynthesized silver nanoparticles using leaf extract of Morus indica L V1 on Bombyx mori L(Lepidoptera: Bombycidae), Sci. Rep. 9 (1), 14839. doi:10.1038/s41598-019-50906-6
Song, J. Y., and Kim, B. S. (2009). Rapid biological synthesis of silver nanoparticles using plant leaf extracts. Bioprocess Biosyst. Eng. 32, 79–84. doi:10.1007/s00449-008-0224-6
Srikar, S. K., Giri, D. D., Pal, D. B., Mishra, P. K., and Upadhyay, S. N. (2016). Green synthesis of silver nanoparticles: A review. Green Sustain. Chem. 6 (1), 34–56. doi:10.4236/gsc.2016.61004
Swamy, M. K., Akhtar, M. S., Mohanty, S. K., and Sinniah, U. R. (2015). Synthesis and characterization of silver nanoparticles using fruit extract of Momordica cymbalaria and assessment of their in vitro antimicrobial, antioxidant and cytotoxicity activities. Spectrochimica Acta Part A Mol. Biomol. Spectrosc. 151, 939–944. doi:10.1016/j.saa.2015.07.009
Syafiuddin, A., Salim, M. R., Beng Hong Kueh, A., Hadibarata, T., and Nur, H. (2017). A review of silver nanoparticles: Research trends, global consumption, synthesis, properties, and future challenges. J. Chin. Chem. Soc. 64 (7), 732–756. doi:10.1002/jccs.201700067
Tian, S., Hu, Y., Chen, X., Liu, C., Xue, Y., and Han, B. (2022). Green synthesis of silver nanoparticles using sodium alginate and tannic acid: Characterization and anti-S. aureus activity, Int. J. Biol. Macromol. 195, 515–522. doi:10.1016/j.ijbiomac.2021.12.031
Topal, M., Gocer, H., Topal, F., Kalin, P., Köse, L. P., Gülçin, İ., et al. (2016). Antioxidant, antiradical, and anticholinergic properties of cynarin purified from the Illyrian thistle (Onopordum illyricum L), J. Enzyme Inhibition Med. Chem. 31 (2), 266–275. doi:10.3109/14756366.2015.1018244
Velmurugan, P., Anbalagan, K., Manosathyadevan, M., Lee, K.-J., Cho, M., Lee, S.-M., et al. (2014). Green synthesis of silver and gold nanoparticles using Zingiber officinale root extract and antibacterial activity of silver nanoparticles against food pathogens. Bioprocess Biosyst. Eng. 37, 1935–1943. doi:10.1007/s00449-014-1169-6
Wongpreecha, J., Polpanich, D., Suteewong, T., Kaewsaneha, C., and Tangboriboonrat, P. (2018). One-pot, large-scale green synthesis of silver nanoparticles-chitosan with enhanced antibacterial activity and low cytotoxicity. Carbohydr. Polym. 199, 641–648. doi:10.1016/j.carbpol.2018.07.039
Keywords: Diospyros kaki, gold nanoparticles, antimicrobial, anticancer, plant based nanoparticles
Citation: Keskin C, Ölçekçi A, Baran A, Baran MF, Eftekhari A, Omarova S, Khalilov R, Aliyev E, Sufianov A, Beilerli A and Gareev I (2023) Green synthesis of silver nanoparticles mediated Diospyros kaki L. (Persimmon): determination of chemical composition and evaluation of their antimicrobials and anticancer activities. Front. Chem. 11:1187808. doi: 10.3389/fchem.2023.1187808
Received: 16 March 2023; Accepted: 15 May 2023;
Published: 30 May 2023.
Edited by:
Syed Shams ul Hassan, Shanghai Jiao Tong University, ChinaReviewed by:
Muhammad Majid, Hamdard University, PakistanMuhammad Ishaq, Shanghai Jiao Tong University, China
Copyright © 2023 Keskin, Ölçekçi, Baran, Baran, Eftekhari, Omarova, Khalilov, Aliyev, Sufianov, Beilerli and Gareev. This is an open-access article distributed under the terms of the Creative Commons Attribution License (CC BY). The use, distribution or reproduction in other forums is permitted, provided the original author(s) and the copyright owner(s) are credited and that the original publication in this journal is cited, in accordance with accepted academic practice. No use, distribution or reproduction is permitted which does not comply with these terms.
*Correspondence: Cumali Keskin, ckeskinoo@gmail.com; Aziz Eftekhari, Ftekhari@ymail.com