- 1Jiangxi Key Laboratory of Birth Defect Prevention and Control, Jiangxi Maternal and Child Health Hospital, Nanchang, China
- 2Maternal and Child Health Hospital of Nanchang Medical College, Nanchang, China
- 3The First Clinical Medical School, Guangdong Medical University, Zhanjiang, China
Prenatal exposure to nicotine that are mainly produced from tobacco smoke has been reported to affect infants. Therefore, nicotine exposure is one of important health concerns for newborn screening. Detecting nicotine and its metabolites such as cotinine in meconium were widely used to evaluate the tobacco exposure of pregnancy. In this study, disposable wooden tips were applied for touch sampling of meconium from newborn infants, and then were directly mounted on mass spectrometer (MS) to perform rapid screening of nicotine and cotinine. Choice of extraction/spray solvents was optimized. The limits of detection, reproducibility, linear response for direct analysis of meconium were also investigated. It is found the limits of detection (S/N = 3) to be as low as 0.36 ng/mg and 1.18 ng/mg for nicotine and cotinine, respectively, while the limits of quantitation (S/N = 10) to be 1.19 ng/mg and 3.94 ng/mg for nicotine and cotinine, respectively. The relative standard deviations (RSD) were found to be at 8.4%–19.8% (n = 6) for nicotine and cotinine, a good linear range from 5–500 ng/mL (R2 > 0.99). These analytical performances are well-accepted levels for ambient mass spectrometer analysis. In this study, evaluation of nicotine and cotinine in 22 puerpera volunteers were conducted by the established wooden-tip spray mass spectrometry (WTS-MS). These results showed that wooden-tip spray mass spectrometry would be useful for newborn screening of nicotine and cotinine in meconium with high reproducibility, speed, sensitivity, and specificity. Owing to the use of disposable wooden tips that involves no sample preparation and no chromatographic separation, our results show that wooden-tip spray mass spectrometry is a powerful tool for determination of nicotine in newborn meconium.
Introduction
Nicotine is one of the most popular air pollutants (Bruin et al., 2010; Gibbs et al., 2016). There remains a potential reproductive health risk during passive nicotine consumption, which is mainly generated from tobacco smoke or electronic nicotine (Wong et al., 2015; Cardenas et al., 2019; Price and Martinez, 2019). For example, pregnant tobacco exposure is associated with many health risks, including premature rupture of membranes, placenta previa, placental abruption, preterm delivery, shortened gestation, fetal growth restriction and low birth weight, sudden infant death syndrome, perinatal mortality, as well as cognitive and neuro developmental disorders (Slotkin et al., 1995; Wickstrom, 2007). Nicotine, the special maker of tobacco, can be used an indicator to directly reflect the impact of tobacco smoke; while the cotinine, a metabolite of nicotine, can be used for evaluating the effect of nicotine exposure and metabolism. There are many clinical samples used for evaluation of tobacco smoke by detecting nicotine and cotinine. Tissue, blood, and urine are usually used for evaluating the nicotine level (Urakawa et al., 1994; Hoofnagle et al., 2006). Among these clinical samples, meconium is useful sources to evaluate the newborn exposure. Meconium is accumulated from 12th–13th weeks of gestation in intestinal compartments to birth, and has been well accepted to be a useful clinical sample (Ostrea Jr et al., 1994; Baranowski et al., 1998; Chan et al., 2004; Gray et al., 2010; Himes et al., 2013; Meyer-Monath et al., 2014; Naritaka et al., 2015). The meconium is usually expelled by the newborn within 24 h after birth. Unlike other fetal biofluids and biomaterials such as urine, blood and hair, the collection of viscous meconium is easy-operation and non-invasiveness for the newborns. Therefore, rapid screening the nicotine and cotinine would be useful for evaluating the nicotine exposure of fetus during pregnancy in tobacco environments.
Mass spectrometry is a powerful analytical platform for disease diagnosis and research, and has been developed for biological and clinical analysis (Fung et al., 2020; Hu and Ouyang, 2021; Yuan and Hu, 2021; Hu, 2022). To determine nicotine in meconium, liquid chromatography-mass spectrometry (LC-MS) and gas chromatography-mass spectrometry (GC-MS) are usually used (Baranowski et al., 1998; Chetiyanukornkul et al., 2004; Yuan et al., 2013; Yuan et al., 2018). Because the meconium is a viscous complex sample, complicated sample preparations and chromatographic separations are required before MS analysis. Current point-of-care (POC) testing requires simple, rapid, sensitive analytical method to perform the fast identification and quantification of target analytes for evaluating follow-up diagnosis and medical management (Ferreira et al., 2016). Therefore, the new application of simple, rapid and reliable analytical tools are highly needed for newborn screening (Yang et al., 2016).
Recently, ambient MS with ambient ionization techniques have been applied for detecting trace analytes in complex clinical samples with no or little sample pretreatment (Ferreira et al., 2016). For example, desorption electrospray ionization, direct analysis in real time, and other ambient desorption/ionization techniques have been successfully applied clinical analysis. Moreover, Similarly, direct electrospray ionization (ESI) techniques have also developed for direct extraction/ionization under ambient conditions (Ferreira et al., 2016). Moreover, various solid substrates such as metal foil (Hu et al., 2014), porous paper (Cai et al., 2021), and biological tissue (Hu et al., 2012), were used for direct loading raw samples, and then were performed ESI emitters (Klampfl and Himmelsbach, 2015). Among these ambient ESI techniques, ESI on wooden tip is one of powerful ambient ESI techniques (Hu et al., 2011; Hu and Yao, 2018; Ng et al., 2019; Millán-Santiago et al., 2022), provides a rapid method for rapid sampling and direct analysis of clinical samples (Hu and Yao, 2022). By using wooden tip, wooden tip was directly used for loading raw samples, and organic solvent was then loaded on the sample to extract analytes and to generate spray ionization by applying a high voltage on wooden tip. Under such conditions, a wooden-tip spray (WTS) was directly generated. WTS-MS have been proven a useful analytical tool in clinical analysis, provides a new analytical strategy for clinical analysis (Hu et al., 2011). Therefore, sample transfer and sample pre-treatment are avoided during WTS-MS analysis, providing a rapid method for direct sample analysis for clinical applications at point-of-care.
In this study, we demonstrated the rapid screening of nicotine and cotinine in meconium by using WTS-MS. The wooden tips were directly used for sampling meconium samples from infants. And then, the samples were directly extracted and ionized by optimized organic solvents. The blank meconium samples were spiked with nicotine and cotinine to investigate the analytical performances of WTS-MS. The sensitivity, reproducibility, and quantitation were investigated in this work. Overall, our results show that WTS-MS is promising method for rapid screening of nicotine and cotinine in meconium.
Materials and methods
Chemicals and materials
Wooden tips (common toothpicks) were purchased from the local supermarket in Nanchang. The toothpicks are labeled to be made of natural wood without chemical modification. Before use, wooden toothpicks were washed by methanol and water to clean the surface contaminants. Nicotine and cotinine standards were purchased from Dr Ehrenstorfer Gmbh (Augsburg, Germany). Isotopic internal standards (IS), nicotine-d4 and cotinine-d3, were purchased from Gerilliant (Round Rock, TX, United States). Pure HPLC-grade organic solvents such as hexane, acetonitrile, methanol, and ethanol were bought from the Chinese Chemical Reagent Co., Ltd. (Shanghai, China). All water used in this study is Milli-Q water.
Collection of clinical meconium samples
All clinical meconium samples were from 22 puerpera volunteers from Jiangxi Provincial Neonatal Screening Center (Nanchang, China). Meconium samples were collected over the first 3 days of life and were immediately stored at −80 C before use. All puerperae have signed the informed consent forms for this study. This study was approved by the Ethics Committee of Jiangxi Provincial Maternal and Child Health Hospital (Nanchang, China).
Wooden-tip spray mass spectrometry analysis
As shown in Figure 1, a pre-cut wooden tip (tip-end: ∼0.2 mm, diameter: 2.0 mm, length: 1.5 cm) was fixed at a nano-ESI device (Thermo Fisher Scientific, Bremen, Germany). Viscous meconium sample (1 µL) was directly loaded on wooden tip (Figure 1A) by pipette, and then 5.0 µL of solvent was loaded on sample surface that loaded on wooden tip and connected a high voltage (Figure 1B). Particularly, under the optimized solvent, methanol solution containing internal standard, nicotine-d4 (10 ng/mL) and cotinine-d3 (10 ng/mL), was slowly added to sample via pipette tip to prepare a fixed amount. Under such conditions, the wooden tip that contained internal standards and potential analytes (nicotine and cotinine) was fixed in front of the MS inlet. The distance between wooden tip-end and MS inlet is ∼10.0 mm. High voltage (+3.5 kV) was applied to the wooden tips, and the spray ionization could be generated. All experiments were carried on the mass spectrometer (Thermo Fisher Scientific, Bremen, Germany), which could perform tandem mass spectrometry experiments. All the mass spectral data acquisition and instrumental control were conducted by using Xcalibur 3.0 software. The acquisition speed was 20 scans/sec. Typically, first 0.5 min of signal duration was averaged to obtain the high-resolution mass spectra. Under MS/MS experiments, the isolation window is 0.4 Da and the collision energy is 25% for nicotine and cotinine.
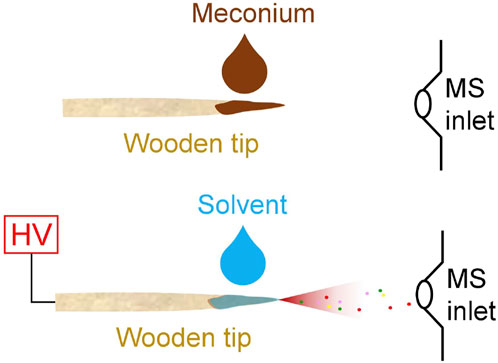
FIGURE 1. WTS-MS analysis of meconium samples: sample loading (upper), direct spray ionization (lower).
Results and discussion
Detection and identification of nicotine and cotinine
To determinate nicotine and cotinine, their standard solutions were analyzed by WTS-MS to obtain their characteristic mass spectra. Figure 2A shows WTS-MS detection of nicotine standard at 10 ng/mL (5.0 μL). The based peak at m/z 163.1231 clearly shows the detection of protonated nicotine (M + H)+ (calculated m/z 163.1235) with high signal-to-noise (S/N), showing an accurate high-resolution mass spectrum with an ultra-low mass error at 2.5 ppm. Although there are some background signals at a low relative abundance, the trace nicotine on wooden tip is ambiguously detected, due the highly efficient ionization of WTS. Upon MS/MS, the characteristic fragment ions at m/z 106.0652, m/z 117.0573, m/z 120.0808, m/z 130.0653, and 163.0808 were observed, as shown in inset of Figure 2A. Because nicotine is a bicyclic compound with a pyridine cycle and a pyrrolidine cycle, these fragment ions were produced by the dissociation of pronated nicotine. To further identify the nicotine, detection of isotopic nicotine-d4 was also performed by WTS-MS/MS, as shown in Figure 2B. The based peak at m/z 167.1481 is corresponding to the protonated nicotine-d4 (M + H)+. Upon MS/MS, the characteristic fragment ions at m/z 110.0903, m/z 121.0824, m/z 124.1059, m/z 134.0902, and 136.106 were observed (inset of Figure 2B). It is found that these fragment ions are corresponding to residues of n-methylpyrrolidine in the nicotine molecule, because the H/D sites are remined in these fragment ions (Figure 2B).
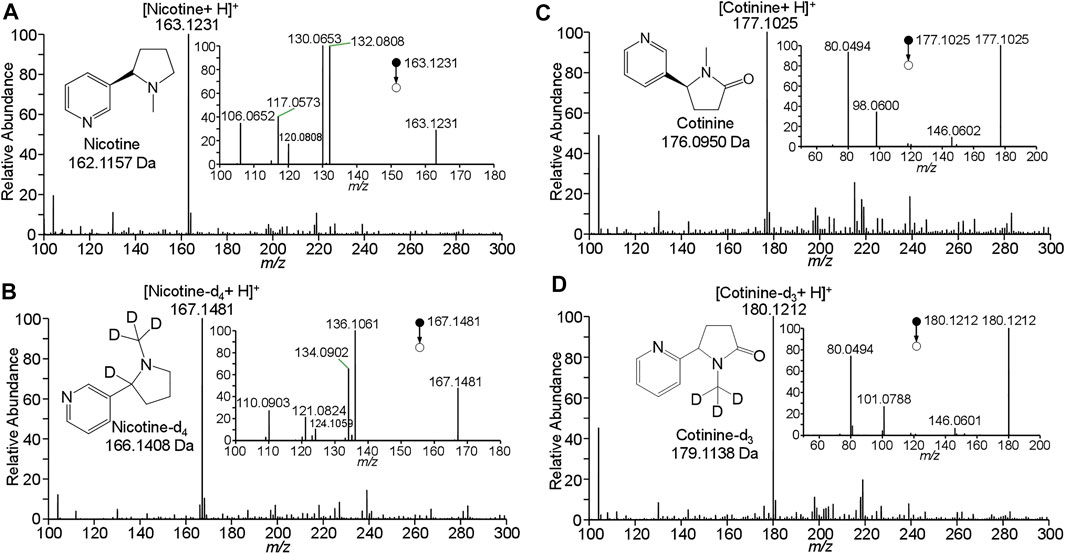
FIGURE 2. WTS-MS detection of nicotine and cotinine: (A) nicotine, (B) nicotine-d4, (C) cotinine, (D) cotinine-d3.
Figure 2C shows WTS-MS detection of cotinine standard at 10 ng/mL (5.0 μL). The based peak at m/z 177.1025 display the protonated cotinine (M + H)+ with high signal-to-noise (S/N). Upon MS/MS, the characteristic fragment ions at m/z 80.0494, m/z 98.0600, and m/z 146.0602 were observed (inset of Figure 2C). Because cotinine also contains a pyridine cycle and a pyrrolidine cycle, these fragment ions were produced by the dissociation of pronated cotinine. The fragment ions at m/z 146.0602 is corresponding to the loss CH3-NH2 (calculated 31.0422 Da) from protonated cotinine. The ions at m/z 80.0494 is corresponding to the protonated pyridine (calculated m/z 80.0500), while the ions at m/z 98.0600 is the fragment ions of by loss of pyridine (calculated 79.0422 Da) from protonated cotinine. These fragment ions were further confirmed by the isotopic cotinine-d3, as shown in Figure 2D. There is base peak of pronated cotinine-d3 (M + H)+at m/z 180.1212. Because three H/D sites are in the pyrrolidine cycle. The ions at m/z 146.0602 is produced by the loss of CD3-NH2 from protonated cotinine. The peak at m/z 80.0494 is confirmed for protonated pyridine (calculated m/z 80.0500), while the ions at m/z 101.0788 is produced from protonated cotinine-d3 by loss of pyridine, as shown inset of Figure 2C. These data clearly show the detection and identification of nicotine and cotinine by high-resolution and MS/MS spectra. The results of characteristic mass spectra are useful for further identification and quantification of nicotine and cotinine in complex clinical samples.
Quantitative detection of nicotine and cotinine in meconium
To improve the detection of nicotine and cotinine, the spray solvent was optimized to extract and ionize nicotine and cotinine. Pure nicotine and cotinine solution was loaded on wooden tip and was dried in the air, and the solvent was loaded onto wooden tip for extracting the analytes and generating spray ionization. In this study, various organic solvents including n-hexane, acetonitrile, methanol, and ethanol, which are common organic solvent and can used for wooden-tip ESI under ambient conditions (Hu and Yao, 2018; Huang et al., 2019), were investigated by WTS-MS, as shown in Figure 3. It is found that the highest signal responses of nicotine and cotinine were obtained by use of methanol. Under the optimized solvent (i.e., methanol), meconium samples were analyzed by WTS-MS. As shown in Figure 4A, the high-resolution mass spectrum of blank meconium samples was obtained, showing various metabolites in meconium samples. However, in this negative meconium sample, no nicotine and cotinine were found in the high-resolution mass spectrum and MS/MS spectra; Figure 4B shows WTS-MS spectrum obtained from meconium samples spiked with nicotine (50 ng/mL), cotinine (50 ng/mL) and their isotopic internal standards (nicotine-d4: 10.0 ng/mL, cotinine-d3: 10. ng/mL). In the high-resolution spectrum, although there are many peaks of biomatrices of meconium, the protonated analytes, including nicotine, cotinine, nicotine-4, and cotinine-3, are also observed according to their special mass-to-charges. Upon MS/MS experiments, these target analytes were further confirmed, as shown in Figures 4C–F, which are good agree with the MS/MS spectra obtained from standards (Figure 2). By using WTS-MS, sampling and analysis of single sample can be completed with minutes, showing a rapid screening. The results show that WTS-MS is promising method for detecting trac nicotine and cotinine in meconium samples.
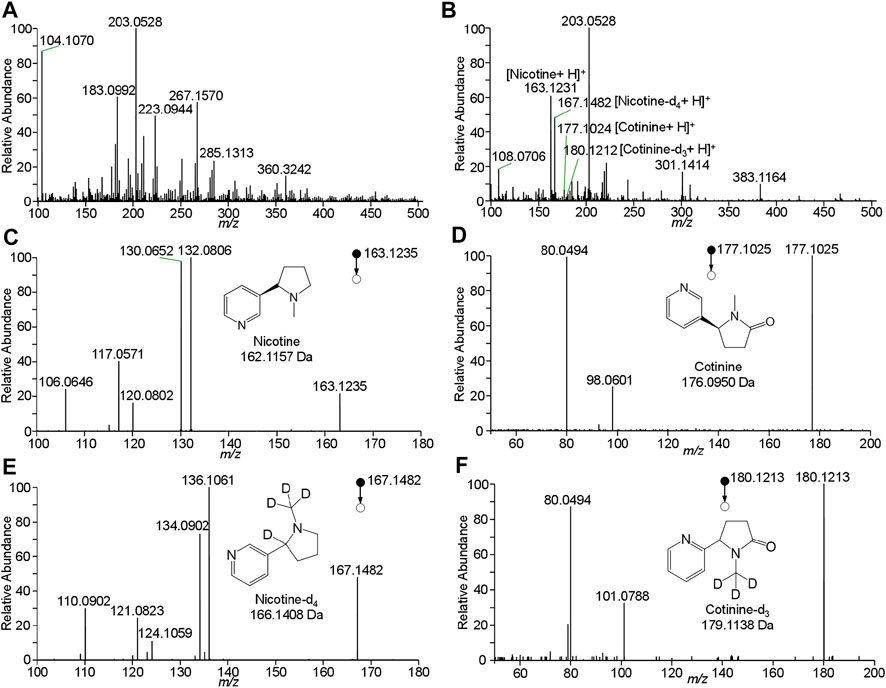
FIGURE 4. WTS-MS detection of nicotine and cotinine in meconium: (A) blank meconium, (B) meconium spiked with nicotine and cotinine, (C) MS/MS spectrum of nicotine, (D) MS/MS spectrum of cotinine, (E) MS/MS spectrum of nicotine-d4, (F) MS/MS spectrum of cotinine-d3.
To further investigate the quantitative analysis of nicotine and cotinine in meconium samples, the analytical performances of WTS-MS were investigated. To compensate the variations of WTS-MS, the limits of detection (LOD) and limits of quantitation (LOQ) were determined by comparing the ratios of signal intensity of spiked samples (i.e., nicotine and cotinine) and blank sample with internal standards (i.e., nicotine-d4 and cotinine-d3) and thus the ratios of (Ianalyte/IIS)spiked/(Ianalyte/IIS)blank were obtained, as shown in Table 1. The LOD (S/N = 3) and LOQ (S/N = 10) of nicotine were found to be 0.36 ng/mL and 1.19 ng/mL, respectively, while LOD (S/N = 3) and LOQ (S/N = 10) of cotinine were found to be 0.18 ng/mL and 3.94 ng/mL, respectively. These data indicated that WTS-MS is a sensitive method for detecting trace nicotine and cotinine in complex meconium. To investigate the reproducibility of WTS-MS, different concentrations of nicotine and cotinine were analyzed, as shown in Table 2. It found that relative standard deviations (RSDs) were found to be at 9.8%–12.6% (n = 6) for six repeated detections of nicotine at low (5.0 ng/mL), middle (50.0 ng/mL), and high concentration (500.0 ng/mL), respectively. At same concentrations, RSDs of cotinine were found to be 8.4%–11.0% (n = 6). These data show the WTS-MS is reliable method of ambient MS analysis of meconium samples.
To conduct the quantitative detection of nicotine and cotinine, the linear responses of nicotine (Figure 5A) and cotinine (Figure 5B) were obtained ranging from 5.0 ng/mL to 500.0 ng/mL using fixed internal standards (nicotine-d4 for nicotine, cotinine-d3 for cotinine). The calibration was constructed by averaging five sets of experimental data of the ratios of nicotine-to-nicotine-d4 and cotinine-to-cotinine-d3. The use of internal standards provides excellent performances to compensate fluctuation of WTS-MS processes, showing a wide linear range and good coefficients (R2 > 0.99). In this study, further evaluation of nicotine and cotinine in 22 puerpera volunteers were conducted by WTS-MS, the results show that nicotine and cotinine are below the LODs, showing there is ultra-low health risk of nicotine exposure. Owing to advantages of disposable wooden tips that involves no sample preparation and no chromatographic separation, our results show showed that WTS-MS is useful tool for newborn screening of nicotine and cotinine in meconium with high reproducibility, speed, sensitivity, and specificity.
Conclusion
In this work, WTS-MS was used for detection of nicotine and cotinine in meconium samples. Compared to conventional MS method (Baranowski et al., 1998; Chetiyanukornkul et al., 2004; Yuan et al., 2013; Yuan et al., 2018), the advantage of WTS-MS for direct meconium analysis is that the disposable, simple, and low-cost wooden tip were used for sample loading and direct ionization without sample pretreatment and separation. In this work, trace nicotine and cotinine were successfully detected by high-resolution mass spectra and MS/MS spectra. Although no positive samples were observed, the sensitivity, specificity, reproducibility, and quantitation of WTS-MS were investigated by detection of spiked samples, showing good analytical performances for ambient MS analysis. Overall, our results show that WTS-MS is promising simple, rapid, and effective analytical tool for detection of nicotine and continue in newborn screening. Due the complicated matrices in clinical samples, further improvement for sensitive and accurate detection of trace analytes is required, the fast microextraction techniques such as solid-phase microextraction is high expected in the future (So et al., 2019; Hu and Ouyang, 2021).
Data availability statement
The original contributions presented in the study are included in the article/supplementary material, further inquiries can be directed to the corresponding authors.
Ethics statement
The studies involving human participants were reviewed and approved by the Ethics Committee of Jiangxi Provincial Maternal and Child Health Hospital. Written informed consent to participate in this study was provided by the participant’s; legal guardian/next of kin.
Author contributions
All authors listed have made a substantial, direct, and intellectual contribution to the work and approved it for publication.
Funding
This study received funding from Jiangxi provincial key research and development program (Nos: 20181BBG70032 and No. 20202BBG73015). The funder was not involved in the study design, collection, analysis, interpretation of data, the writing of this article or the decision to submit it for publication.
Conflict of interest
The reviewer GY declared a past co-authorship with the author BY to the handling editor.
The authors declare that the research was conducted in the absence of any commercial or financial relationships that could be construed as a potential conflict of interest.
Publisher’s note
All claims expressed in this article are solely those of the authors and do not necessarily represent those of their affiliated organizations, or those of the publisher, the editors and the reviewers. Any product that may be evaluated in this article, or claim that may be made by its manufacturer, is not guaranteed or endorsed by the publisher.
References
Baranowski, J., Pochopień, G., and Baranowska, I. (1998). Determination of nicotine, cotinine and caffeine in meconium using high-performance liquid chromatography. J. Chromatogr. B 707 (1-2), 317–321. doi:10.1016/s0378-4347(97)00619-1
Bruin, J. E., Gerstein, H. C., and Holloway, A. C. (2010). Long-term consequences of fetal and neonatal nicotine exposure: A critical review. Toxicol. Sci. 116 (2), 364–374. doi:10.1093/toxsci/kfq103
Cai, S.-H., Di, D., Yuan, Z.-C., Chen, W., and Hu, B. (2021). Paper-in-Facemask device for direct mass spectrometry analysis of human respiratory aerosols and environmental exposures via wearable continuous-flow adsorptive sampling: A proof-of-concept study. Anal. Chem. 93 (41), 13743–13748. doi:10.1021/acs.analchem.1c03406
Cardenas, V. M., Fischbach, L. A., and Chowdhury, P. (2019). The use of electronic nicotine delivery systems during pregnancy and the reproductive outcomes: A systematic review of the literature. Tob. Induc. Dis. 17, 52. doi:10.18332/tid/104724
Chan, D., Caprara, D., Blanchette, P., Klein, J., and Koren, G. (2004). Recent developments in meconium and hair testing methods for the confirmation of gestational exposures to alcohol and tobacco smoke. Clin. Biochem. 37 (6), 429–438. doi:10.1016/j.clinbiochem.2004.01.010
Chetiyanukornkul, T., Toriba, A., Kizu, R., Kimura, K., and Hayakawa, K. (2004). Hair analysis of nicotine and cotinine for evaluating tobacco smoke exposure by liquid chromatography–mass spectrometry. Biomed. Chromatogr. 18 (9), 655–661. doi:10.1002/bmc.369
Ferreira, C. R., Yanne, K. E., Jarmusch, A. K., Pirro, V., Ouyang, Z., and Cooks, R. G. (2016). Ambient ionization mass spectrometry for point-of-care diagnostics and other clinical measurements. Clin. Chem. 62 (1), 99–110. doi:10.1373/clinchem.2014.237164
Fung, A. W. S., Sugumar, V., Ren, A. H., and Kulasingam, V. (2020). Emerging role of clinical mass spectrometry in pathology. J. Clin. Pathology 73 (2), 61–69. doi:10.1136/jclinpath-2019-206269
Gibbs, K., Collaco, J. M., and McGrath-Morrow, S. A. (2016). Impact of tobacco smoke and nicotine exposure on lung development. Chest 149 (2), 552–561. doi:10.1378/chest.15-1858
Gray, T. R., Eiden, R. D., Leonard, K. E., Connors, G., Shisler, S., and Huestis, M. A. (2010). Nicotine and metabolites in meconium as evidence of maternal cigarette smoking during pregnancy and predictors of neonatal growth deficits. Nicotine Tob. Res. 12 (6), 658–664. doi:10.1093/ntr/ntq068
Himes, S. K., Stroud, L. R., Scheidweiler, K. B., Niaura, R. S., and Huestis, M. A. (2013). Prenatal tobacco exposure, biomarkers for tobacco in meconium, and neonatal growth outcomes. J. Pediatr. 162 (5), 970–975. doi:10.1016/j.jpeds.2012.10.045
Hoofnagle, A. N., Laha, T. J., Rainey, P. M., and Sadrzadeh, S. M. (2006). Specific detection of anabasine, nicotine, and nicotine metabolites in urine by liquid chromatography–tandem mass spectrometry. Am. J. Clin. pathology 126 (6), 880–887. doi:10.1309/lq8u3ul956et324x
Hu, B., Lai, Y.-H., So, P.-K., Chen, H., and Yao, Z.-P. (2012). Direct ionization of biological tissue for mass spectrometric analysis. Analyst 137 (16), 3613–3619. doi:10.1039/c2an16223g
Hu, B., and Ouyang, G. (2021). In situ solid phase microextraction sampling of analytes from living human objects for mass spectrometry analysis. TrAC Trends Anal. Chem. 143, 116368. doi:10.1016/j.trac.2021.116368
Hu, B. (2022). Recent advances in facemask devices for in vivo sampling of human exhaled breath aerosols and inhalable environmental exposures. TrAC Trends Anal. Chem. 151, 116600. doi:10.1016/j.trac.2022.116600
Hu, B., So, P.-K., Chen, H., and Yao, Z.-P. (2011). Electrospray ionization using wooden tips. Anal. Chem. 83 (21), 8201–8207. doi:10.1021/ac2017713
Hu, B., So, P.-K., and Yao, Z.-P. (2014). Electrospray ionization with aluminum foil: A versatile mass spectrometric technique. Anal. Chim. Acta 817, 1–8. doi:10.1016/j.aca.2014.02.005
Hu, B., and Yao, Z.-P. (2018). Detection of native proteins using solid-substrate electrospray ionization mass spectrometry with nonpolar solvents. Anal. Chim. Acta 1004, 51–57. doi:10.1016/j.aca.2017.11.079
Hu, B., and Yao, Z.-P. (2022). Electrospray ionization mass spectrometry with wooden tips: A review. Anal. Chim. Acta 1209, 339136. doi:10.1016/j.aca.2021.339136
Huang, Z., Yao, Y.-N., Li, W., and Hu, B. (2019). Analytical properties of electrospray ionization mass spectrometry with solid substrates and nonpolar solvents. Anal. Chim. Acta 1050, 105–112. doi:10.1016/j.aca.2018.10.064
Klampfl, C. W., and Himmelsbach, M. (2015). Direct ionization methods in mass spectrometry: An overview. Anal. Chim. Acta 890, 44–59. doi:10.1016/j.aca.2015.07.012
Meyer-Monath, M., Chatellier, C., Rouget, F., Morel, I., and Lestremau, F. (2014). Development of a multi-residue method in a fetal matrix: Analysis of meconium. Anal. Bioanal. Chem. 406 (30), 7785–7797. doi:10.1007/s00216-014-8243-4
Millán-Santiago, J., Lucena, R., and Cárdenas, S. (2022). Wooden-based materials: Eco-friendly materials for direct mass spectrometric analysis and microextraction. J. Sep. Sci. 45 (1), 223–232. doi:10.1002/jssc.202100660
Naritaka, N., Suzuki, M., Sato, H., Takei, H., Murai, T., Kurosawa, T., et al. (2015). Profile of bile acids in fetal gallbladder and meconium using liquid chromatography-tandem mass spectrometry. Clin. Chim. Acta 446, 76–81. doi:10.1016/j.cca.2015.04.008
Ng, T.-T., So, P.-K., Hu, B., and Yao, Z.-P. (2019). Rapid detection and quantitation of drugs-of-abuse by wooden-tip electrospray ionization mass spectrometry. J. Food Drug Analysis 27 (2), 428–438. doi:10.1016/j.jfda.2018.09.002
Ostrea, E. M., Knapp, D. K., Romero, A., Montes, M., and Ostrea, A. R. (1994). Meconium analysis to assess fetal exposure to nicotine by active and passive maternal smoking. J. Pediatr. 124 (3), 471–476. doi:10.1016/s0022-3476(94)70378-7
Price, L. R., and Martinez, J. (2019). Cardiovascular, carcinogenic and reproductive effects of nicotine exposure: A narrative review of the scientific literature. F1000Research 8, 1586. doi:10.12688/f1000research.20062.2
Slotkin, T., Lappi, S., McCook, E., Lorber, B., and Seidler, F. (1995). Loss of neonatal hypoxia tolerance after prenatal nicotine exposure: Implications for sudden infant death syndrome. Brain Res. Bull. 38 (1), 69–75. doi:10.1016/0361-9230(95)00073-n
So, P.-K., Yang, B.-C., Li, W., Wu, L., and Hu, B. (2019). Simple fabrication of solid-phase microextraction with surface-coated aluminum foil for enhanced detection of analytes in biological and clinical samples by mass spectrometry. Anal. Chem. 91 (15), 9430–9434. doi:10.1021/acs.analchem.9b02428
Urakawa, N., Nagata, T., Kudo, K., Kimura, K., and Imamura, T. (1994). Simultaneous determination of nicotine and cotinine in various human tissues using capillary gas chromatography/mass spectrometry. Int. J. Leg. Med. 106 (5), 232–236. doi:10.1007/bf01225411
Wickstrom, R. (2007). Effects of nicotine during pregnancy: Human and experimental evidence. Curr. Neuropharmacol. 5 (3), 213–222. doi:10.2174/157015907781695955
Wong, M. K., Barra, N. G., Alfaidy, N., Hardy, D. B., and Holloway, A. C. (2015). Adverse effects of perinatal nicotine exposure on reproductive outcomes. Reproduction 150 (6), R185–R193. doi:10.1530/rep-15-0295
Yang, B.-c., Wang, F., Yang, X., Zou, W., Wang, J.-c., Zou, Y., et al. (2016). Medical swab touch spray-mass spectrometry for newborn screening of nicotine and cotinine in meconium. J. Mass Spectrom. 51 (12), 1237–1242. doi:10.1002/jms.3892
Yuan, C., Kosewick, J., and Wang, S. (2013). A simple, fast, and sensitive method for the measurement of serum nicotine, cotinine, and nornicotine by LC–MS/MS. J. Sep. Sci. 36 (15), 2394–2400. doi:10.1002/jssc.201300220
Yuan, Y., Wang, Y., Yang, M., Xu, Y., Chen, W., Zou, X., et al. (2018). Application of response surface methodology to vortex-assisted dispersive liquid–liquid extraction for the determination of nicotine and cotinine in urine by gas chromatography–tandem mass spectrometry. J. Sep. Sci. 41 (10), 2261–2268. doi:10.1002/jssc.201701167
Keywords: wooden tip, meconium, nicotine, ambient mass spectrometry, electrospray ionization
Citation: Wang X, Yang M, Xiao H, Liu D, Pan L, Zhang L, Yang Y, Lu Q, Liu Y, Yang X and Yang B (2023) Determination of nicotine in newborn meconium by high-Resolution ambient mass spectrometry using wooden-Tip spray. Front. Chem. 11:1122137. doi: 10.3389/fchem.2023.1122137
Received: 12 December 2022; Accepted: 10 January 2023;
Published: 19 January 2023.
Edited by:
Haixing Wang, Second Affiliated Hospital and Yuying Children’s Hospital of Wenzhou Medical University, ChinaReviewed by:
Guozhu Ye, Institute of Urban Environment (CAS), ChinaBin Hu, Jinan University, China
Wenqing Tu, Jiangxi Agricultural University, China
Copyright © 2023 Wang, Yang, Xiao, Liu, Pan, Zhang, Yang, Lu, Liu, Yang and Yang. This is an open-access article distributed under the terms of the Creative Commons Attribution License (CC BY). The use, distribution or reproduction in other forums is permitted, provided the original author(s) and the copyright owner(s) are credited and that the original publication in this journal is cited, in accordance with accepted academic practice. No use, distribution or reproduction is permitted which does not comply with these terms.
*Correspondence: Bicheng Yang, eWFuZ2JjMTk4NUAxMjYuY29t; Xiao Yang, eWFuZ3hpYW8zNDIxQDEyNi5jb20=; Yanqiu Liu, THlxMDkxNEAxMjYuY29t