- 1College of Science, Huazhong Agricultural University, Wuhan, China
- 2National Biopesticide Engineering Research Centre, Hubei Biopesticide Engineering Research Centre, Hubei Academy of Agricultural Sciences, Wuhan, China
Carbazole alkaloids, as an important class of natural products, have been widely reported to have extensive biological activities. Based on our previous three-component reaction to construct carbazole scaffolds, we introduced a methylene group to provide a rotatable bond, and designed series of carbazole derivatives with structural diversity including carbazole amide, carbazole hydrazide and carbazole hydrazone. All synthesized carbazole derivatives were evaluated for their in vitro cytotoxic activity against 7901 (gastric adenocarcinoma), A875 (human melanoma) and MARC145 (African green monkey kidney) cell lines. The preliminary results indicated that compound 14a exhibited high inhibitory activities on 7901 and A875 cancer cells with the lowest IC50 of 11.8 ± 1.26 and 9.77 ± 8.32 μM, respectively, which might be the new lead compound for discovery of novel carbazole-type anticancer agents.
1 Introduction
Cancer has long been an important disease threatening human health. Except the direct invasive therapy, radiotherapy, the use of chemotherapeutic drugs to kill cancer cells and treat tumors are the most important treatment of intermediate and advanced tumors with clinical metastasis (Sung et al., 2021). In the current clinical trials of cancer treatment, 65% of drugs are derived from natural products (Newman and Cragg, 2020; Bhutani et al., 2021). Despite the extensive use of drug design, compounds in nature are still the focus of drug research and development.
Natural products, especially heterocyclic compounds, have always been an important source of anticancer drugs and a molecular library of potential drugs (Fang et al., 2021; Yang et al., 2021). Many natural products or their derivatives have been demonstrated to have extensive antitumor activities by inducing apoptosis (Chen et al., 2015; Liu et al., 2015; Padmaja et al., 2015; Bailly, 2020; Greco et al., 2021) and inhibiting mitosis (Thomas et al., 2016; Davison and Sperry, 2017; Yokoyama et al., 2019). As an important class of aromatic heterocyclic compounds, carbazole alkaloid derivatives have long been used in medicinal chemistry (Murali et al., 2017; Sun et al., 2017; Zhou et al., 2018; Cao et al., 2019; Issa et al., 2019; Liu et al., 2019; Rassias et al., 2019; Liu et al., 2020; Liu et al., 2021; Wang et al., 2022), agrochemicals (Kong et al., 2016; Wang et al., 2017; Dang et al., 2018; Sun et al., 2020; Xie et al., 2020) and other fields. So, how to realize the functional modification of carbazole ring to improve its activity is always an important research direction.
In our previous work, a series of thirty carbazole arylhydrazone derivatives were conveniently synthesized and evaluated as potential cytotoxic agents, using two of the same cells as this paper, namely A875 (human melanoma) and MARC145 (African green monkey kidney cell line MA-104) cells. Among them, compound 2021-7e (Figure 5) elicited the lowest IC50 against A875 (4.15 μM) and IC50 of 26.64 μM on MARC145 (Huang et al., 2021). Meanwhile, a three-components indole-to-carbazole reaction (Wu et al., 2021) was adopted to construct the carbazole scaffold, which has high yield compared to the traditional reactions (Aggarwal and Verma, 2019; Wu et al., 2021). However, the carbazole unit is directly connected with the carbonyl group forming a p-π rigid conjugated system, which may not be conducive to the binding with the receptor protein. So, in order to further investigate the derivatization ability and drug potential of carbazole analogues, a methylene unit was introduced to provide a rotatable bond attached to the carbazole scaffold (Figure 1), and a series of carbazole derivatives with structural diversity including carbazole amide, carbazole hydrazide and carbazole hydrazone were designed and synthesized. We hope that the introduction of this flexibility is proposed to increase the binding ability of target molecules to receptor proteins.
Thus, we wish to report herein the convenient synthesis of novel carbazole derivatives with structural diversity as indicated in Scheme 1, and their potential cytotoxic activities against several cell lines including human gastric adenocarcinoma cells (7901), human melanoma (A875), and a subclone of African green monkey kidney cell line MA-104 (MARC145) were investigated using classical MTT colorimetric method.
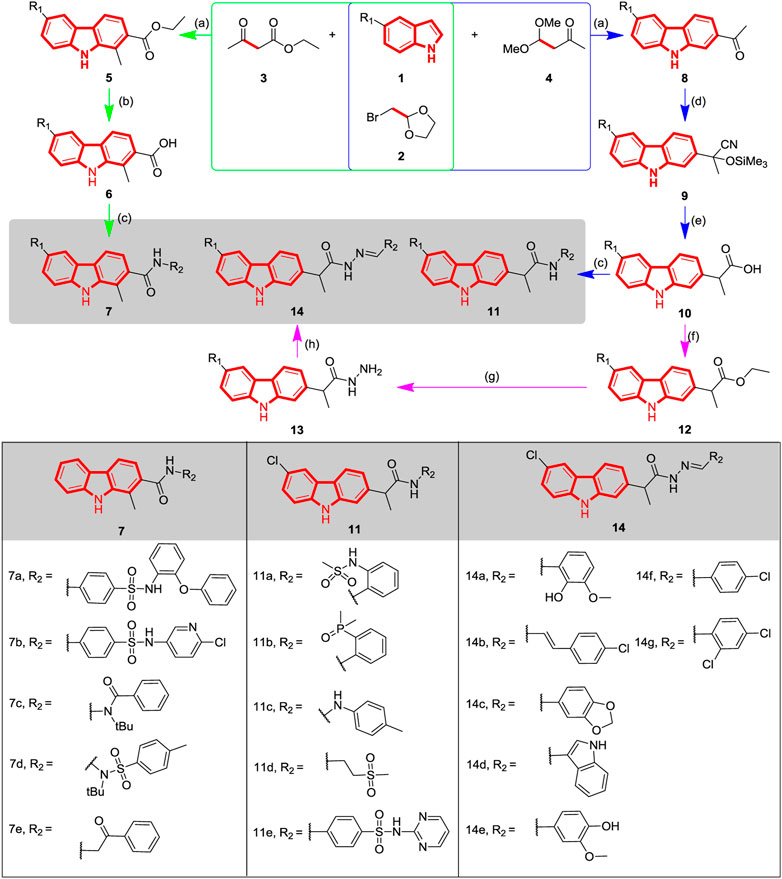
SCHEME 1. Synthetic route and structure of carbazole derivatives 7a-e, 11a-e and 14a-g. Reagents and conditions: (a) AlCl3, CH3CN, reflux; (b) KOH, EtOH, rt; (c) i. Py, MsCl, CH3CN, 0°C; ii. R2-NH2 or R2=NNH2, 40-50 °C; (d) Me3SiCN, ZnI2, rt; (e) i. SnCl2, AcOH, rt; ii. conc. HCl; (f) EtOH, Conc. H2SO4, reflux; (g) NH2NH2·H2O, EtOH, reflux; (h) Aldehyde, EtOH, reflux.
2 Results and discussion
2.1 Chemistry
In this work, three series of carbazole analogues with structural diversity were designed and synthesized by integrating the carbazole alkaloid moiety with diverse pharmacophores including amide, hydrazide and acylhydrazone etc. The general synthetic route and structures is depicted in Scheme 1.
In previous studies it was demonstrated that a series of carbazole structures could be obtained by the three-component reaction of bromoacetal acetal, ketone and indole catalyzed by bismuth trifluoromethanesulfonate (Gu et al., 2018), but the carbazole structure is directly connected to the carbonyl group, which formed a rigid conjugated structure. In order to further explore the potential effect of adding rotatable bonds to improve molecular flexibility on activity, different types of carbazole derivatives were designed and synthesized. Firstly, 1-methyl-9H-carbazoles 7a-e were synthesized via three steps according to our previous procedure. The carbazole scaffold 5 was synthesized using indole, ethyl acetoacetate and 2-(bromomethyl)-1,3-dioxolane, which was then hydrolyzed to obtain the corresponding acids 6, and then treated with a pharmacophore-bearing amine to form various amide derivatives. Similarly, the key carbazole acids with rotatable bonds of type 10 were constructed via three steps (Percy et al., 1994) as indicated in Scheme 1; In particular, the carbazole acetyl 8 was obtained using a similar method to compounds 5 by using ethyl acetoacetate instead of 4,4-dimethoxybutan-2-one. Therefore, the carbonyl group of compounds 8 was cyanated through trimethylcyanosilane, and then hydrolyzed to obtain the intermediate carbazole acids 10, which were isolated as a white solid by column chromatography. A series of compounds 11a-e were conveniently synthesized by direct reaction of carbazole acids 10 with the appropriate amines. Compounds 10 were also transformed into the corresponding carbazole hydrazide 13 via two steps: esterification of the acid group followed by reaction with hydrazine hydrate. The compounds 13 were also reacted with a carbonyl-bearing substrate to obtain compounds 14a-g, which bridges the carbazole structure to the potentially active pharmacophore (Popiolek et al., 2018; Xie et al., 2019; Duangdee et al., 2020; Han et al., 2020; Peng et al., 2020; Wang et al., 2020). Because the condensation method used is not stereoselective, the resulting acylhydrazone derivatives are a mixed (E/Z) configuration.
2.2 Spectroscopy
All obtained carbazole derivatives were elucidated based on the chemical analyses including 1H NMR, 1C NMR, ESI-MS, HRMS, and X-Ray analyses. For the 1H NMR spectra of all 1-methyl-9H-carbazole derivatives 7a-e, the obvious signals at 2.08–2.66 ppm were attributed to the methyl group attached to the carbazole ring as shown in the molecular structure. With respect to other carbazole derivatives 11a-e and 14a-g, the 1H NMR spectrum indicated a unique signal for the flexible bond CHCH3 unit, with a quartet signal at about 4.92–3.73 ppm and a doublet signal at about 1.63–1.40 ppm, respectively. Both the NH protons on the carbazole ring and the NH protons next to the carbonyl appear in the range of 11.52–9.56 ppm. The remaining signal peaks at low fields are attributed to the proton peaks of the carbazole ring or other aromatic rings. All the characteristic peaks observed within the 1H NMR spectra for title compounds are given in materials and methods. In addition, the representative single crystal diffraction analysis of target compound 14a is shown in Figure 2.
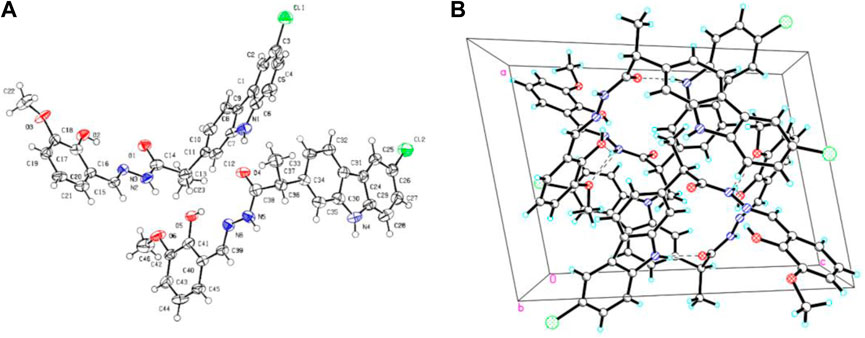
FIGURE 2. Single crystal structure (A) and packing diagram (B) for compound 14a, and the dashed lines denote intermolecular hydrogen bonds.
2.3 Pharmacology evaluation
Using 5-fluorouracil (5-FU) as a positive control, the inhibition effects of all carbazole derivatives on 7901 (gastric adenocarcinoma cells), A875 (human melanoma) and MARC145 (African green monkey kidney cell line MA-104) cells in vitro were evaluated by standard MTT assay, and the preliminary bioassay results were described in Figure 3. Generally, as shown in Figure 3, some compounds showed higher activities compared with the control 5-FU. Among them, carbazole derivatives 7b, 11a, 11b, 14a, and 14b had higher in vitro inhibition rates on the two cancer cells 7901 and A875 (>70%). In addition, compounds 7b, 11a and 14a had less toxicity to normal cells MARC-145 than cancer cells, especially, it is worth noting that, compound 14a have little toxicity to normal cells MARC-145 and show moderate selectivity to cancer cells.
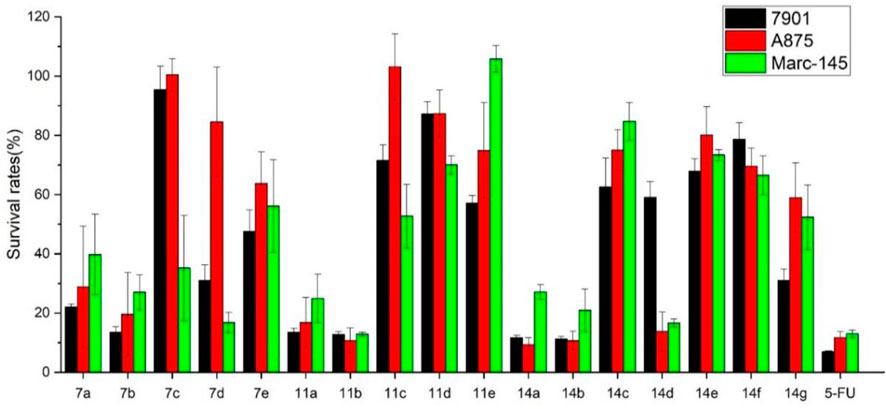
FIGURE 3. Cytotoxic activities of synthesized compounds at the concentration of 40 μg/mL. Abbreviations: 7901—Human gastric cancer cell line; A875—Human melanoma cell line; MARC145—A subclone of African green monkey kidney cell line MA-104; 5-FU—5-Fluorouracil, used as a positive control.
Through the preliminary screening results, some molecules showed good inhibitory effects on target cancer cells. In order to further verify the potential activity of these carbazole derivatives, we determined the IC50 values of all compounds 7a-e, 11a-e, and 14a-g. It is further proved that compounds 11a, 11b, 14a, 14b are the highly potential scaffolds with excellent anticancer activities.
Generally, the carbazole derivatives with flexible bonds attached to carbazole ring have some advantages compared with the carbazole derivatives with rigid structures, and overall exhibit better inhibitory activity against cancer cells. It is worth noting that compounds 11a, 11b, 14a, and 14b exhibited good inhibition activities on all tested cells. Especially, compound 14a derived from o-vanillin was most active molecule against all tested cell lines with the IC50 value of 11.8, 9.77, and 7.47 μM, respectively, which was significantly better than that of the control 5-FU. However, compound 14e containing vanillin scaffold almost lost its activity, which indicated the hydrophilic groups in the neighborhood of the aromatic ring might be very important for the activity of these class of compounds. In addition, the four compounds 11a, 11b, 14a, 14b have similar structures to carprofen and may act as a pharmacophore to enhance its antitumor activity (Favia et al., 2012; Mellini et al., 2012; Zhou et al., 2018).
The relationship between concentration and inhibition rate is the key to whether a potential compound can be use as potential medicinal agents. It is very important to maintain good activity at low concentration. Based on the preliminary screening data, in order to verify the effect of concentration on the potential cytotoxic activities for all compounds, the inhibition activity data of the compounds at different concentrations were detected using the same method. All experiments were carried out on three kinds of cell lines with six concentrations of 40, 20, 10, 5, 1, and 0.5 μg/mL. The inhibition activities for highly potential compounds 7b, 11a, 11b, 14a, and 14b were described in Figure 4. It can be seen that with the decrease of the concentration, the inhibition rate of the cells also decreased, but good activity was also guaranteed for compound 14a at a concentration of 5 μg/mL, which was significantly better than that of other compounds tested.
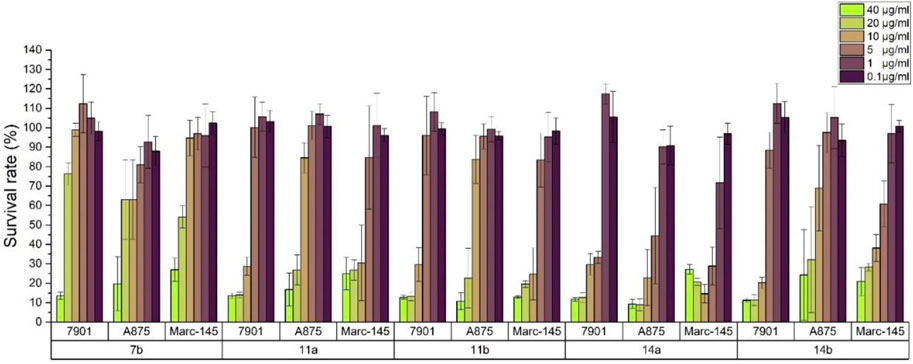
FIGURE 4. Survival rates of 7901, A875 and Marc-145 in different concentration of 7b,11a, 11b, and 14a, 14b.
The structure evolution here was to modify carbazole scaffold and different pharmacophore. According to the in vitro results described in Table 1, the possible structure-activity relationships for these synthesized carbazole derivatives can be concluded. Considering the type of pharmacophore, it can be clearly seen that the sulfonamide group can efficiently improve the potential activities of these compounds as indicated in Figure 5. When there is no hydrogen on the amino group, the compound’s activity (such as 7d) decreases significantly, as it may affect the formation of hydrogen bonds. However, the molecule 11d containing only sulfone group also lost activity. The structural isomerism and the length of the carbon chain significantly affected the activity of the compound. For example, the activity of compound 14a bearing 2-hydroxy-3-methoxybenzylidene unit is significantly better than that of compound 14e containing 4-hydroxy-3-methoxybenzylidene moiety, as well as for compound 14b characterized with one more vinyl group than derivative 14f. In contrast to the previous work, the addition of flexible bonds produced complex effects. On the one hand, the activity of some compounds was improved (such as 14d), likely due to the increase of rotatable bonds which i) reduced the permeability to the cell membrane, or ii) increased the entropy penalty when binding proteins. However, the rotatable bonds also reduced the activity of some compounds (for example, compounds 14a). It is worth noting that the 2-hydroxy-3-methoxybenzylidene group is the most effective pharmacophore in the previous work (Compound 2021-7e) and this work (Compound 14a), and has the value of further research (Huang et al., 2021) (Figure 5).
According to the aforementioned analysis, the potential inhibitor activity is relatively good for those carbazole derivatives with higher molecular flexibility. In the rigid structure, only 7b has moderate inhibitory activity on tested cells, which reflects the effect of molecular flexibility on the activity of these compounds. The increase of rotatable bonds may facilitate the binding of compounds to possible receptors, so as to improve its inhibitory activity (Peng et al., 2019; Caron et al., 2020).
3 Conclusion
In this study, several types of carbazole derivatives including carbazole amides, carbazole hydrazides and carbazole hydrazones were designed and synthesized based on the scaffold of carbazole alkaloids. All obtained molecules were fully characterized by 1H NMR, 13C NMR, ESI-MS, HRMS and X-Ray diffraction analyses, and their potential inhibitory activities on cancer cell lines were bio-evaluated. The bioassay indicated that some of the target compounds had good inhibitory effects on 7901 and A875 cancer cells, especially, the best potential compound 14a exhibited high inhibitory activities with the lowest IC50 of 11.8 ± 1.26 and 9.77 ± 8.32 μM, respectively, which might be the new lead molecule for discovery of novel carbazole-type anticancer agents.
4 Materials and methods
4.1 Instrumentation and chemicals
All melting points (m.p.) were measured using a digital model X-5 apparatus and are uncorrected. 1H NMR and 13C NMR spectra were recorded on a Bruker Avance III 600 MHz FT-NMR spectrometer using DMSO-d6 as the solvent and tetramethylsilane (TMS) as the internal standard. Mass spectra were performed on a WATERS ACQUITY UPLC® H-CLASS PDA (Waters®) instrument. High resolution mass spectrometry analysis (HRMS) was done with Agilent 6224 TOF mass spectrometer. Thin-layer chromatography was carried out on precoated silica gel plates GF254 (Qingdao Haiyang Chemical, China), and spots were visualized with ultraviolet light. All starting materials and reagents commercially available were used without further purification, unless otherwise specified.
4.2 General synthetic procedure for 1-methyl-9H-carbazole-2-carboxylic acid 6
Indole (10.0 mmol), ethyl acetoacetate (10.0 mmol), 2-(bromomethyl)-1,3-dioxolane (12.0 mmol), AlCl3 (0.5 mmol, 5 mol%) and acetonitrile (35 mL) was added in a 100 mL single-neck flask. The mixture was stirred at 80°C for 6 h obtaining a solid which was filtered under reduced pressure. The residue product was rinsed by brine, and the aqueous phase was extracted by ethyl acetate. The organic phase was combined, dried over anhydrous Na2SO4 and filtered. The solvent was removed by rotary evaporation to obtain the crude intermediate 5. The latter was added in a 100 mL single-neck flask with methanol (10 mL) and sodium hydroxide (15 mmol), and the reaction was stirred at room temperature overnight. The mixture was added dilute hydrochloric acid adjust to pH 2-3 under ice-bath, and the precipitate was 1-methyl-9H-carbazole-2-carboxylic acid 6. The compound was brown powder, MS (ESI) m/z calcd for C14H10NO2 [M - H]- 224.08, found 224.48.
4.3 General synthetic procedure for 2-(6-chloro-9H-carbazol-2-yl)propanoic acid 10
Indole (10.0 mmol), 4,4-dimethoxybutan-2-one (10.0 mmol), 2-(bromomethyl)-1,3-dioxolane (12.0 mmol), AlCl3 (0.5 mmol, 5 mol%) and acetonitrile (35 mL) was added in a 100 mL single-neck flask. The mixture was stirred at 80°C for 8 h. After reaction, the solid was filtered under reduced pressure. The residue product was rinsed by brine, and the aqueous phase was extracted by ethyl acetate, then combine the residue and the organic phase, which was dried over anhydrous Na2SO4. After that, the solvent was removed by rotary evaporation, and the residue was used directly in subsequent reactions without purification. The residue was added in a 100 mL single-neck flask, and treated with trimethylsilyl cyanide and ZnI2 under the condition of reflux. After reaction, the mixture was cooled to room temperature and filtered, which was concentrated to obtain crude product 9. Subsequently, compounds 9 was treated with stannous chloride in acetic acid, then hydrolysis with concentrated hydrochloric acid, and the carbazole derivative 2-(6-chloro-9H-carbazol-2-yl)propanoic acid 10 will be obtained through a series of acid-base treatment. MS (ESI) m/z calcd for C15H12ClNO2 [M - H]- 272.06, found 272.41.
4.4 General synthetic procedure for 2-(6-chloro-9H-carbazol-2-yl)propanehydrazide 13
To a solution of substituted 2-(6-chloro-9H-carbazol-2-yl)propanoic acid 10 (10 mmol) in ethanol was added the catalytic amount of concentrated sulfuric acid, which was refluxed at 80°C for 6–7 h. Then, the solution was washed with water and extracted with ethyl acetate, and the organic phase was directly used for the next reaction after drying and concentration. The residue was dissolved in 10 mL of ethanol, and 5 eq. of hydrazine hydrate was added, the mixture was heated at 70–75°C for 4 h. The precipitate was filtered and washed with a small amount of cold ethanol to obtain the desired molecule 2-(6-chloro-9H-carbazol-2-yl)propanehydrazide 13: MS (ESI) m/z calcd for C15H14ClN3O [M + H]+ 288.08, found 288.25.
4.5 General synthetic procedure for the target compounds 7a-e and 11a-e
The general synthetic procedure of carbazole-based amide 7a-e and 11a-e: To a solution of carbazole-based carboxylic acids 6 or 10 (0.8 mmol) in 10 mL anhydrous acetonitrile was added pyridine (2.4 mmol) under the condition of 0–5°C. Then, methanesulfonyl chloride (1.2 mmol) was added dropwise to the mixture, which was then allowed to warm to room temperature and stirred for additional half hour, the various amines (0.8 mmol) were added, and the reaction mixture was heated to 40–45°C and detected by thin-layer chromatography. After completion of the reaction, the mixture was quenched by the addition of water, and the suspended solid was collected by filtration and washed with water to afford the crude products, which were purified by silica gel column chromatography (petroleum ether/ethyl acetate) to give the target compounds. Their physico-chemical properties and the spectra data are as follows:
1-Methyl-N-(4-(N-(2-phenoxyphenyl)sulfamoyl)phenyl)-9H-carbazole-2-carboxamide (7a): white solid; yield: 71%; m. p. 78°C; 1H NMR (600 MHz, DMSO-d6, 25°C) δ = 11.42 (s, 1H), 10.69 (s, 1H), 9.87 (s, 1H), 8.17 (d, J = 7.8 Hz, 1H), 8.08 (d, J = 8.0 Hz, 1H), 7.87—7.82 (m, 2H), 7.71—7.66 (m, 2H), 7.56 (dt, J = 8.1, 0.9 Hz, 1H), 7.46—7.40 (m, 2H), 7.33—7.29 (m, 3H), 7.21—7.18 (m, 1H), 7.12—7.08 (m, 3H), 6.75—6.71 (m, 1H), 6.71—6.67 (m, 2H), 2.65 (s, 3H) ppm; 13C NMR (151 MHz, DMSO-d6, 25°C) δ = 169.26, 156.62, 149.93, 143.65, 141.11, 139.65, 133.74, 130.22, 130.15, 128.27, 126.64, 125.95, 124.07, 123.84, 123.56, 122.77, 121.23, 119.59, 119.40, 119.00, 118.98, 118.93, 118.66, 117.94, 111.77, 40.39, 40.26, 40.12, 39.98, 39.84, 39.70, 39.56, 14.82, 0.59 ppm; MS (ESI) m/z 548.38 = [M + H]+, HRMS m/z 548.1633 = [M + H]+, calcd. for C32H25N3O4S m/z = 547.1566.
N-(4-(N-(6-Chloropyridin-3-yl)sulfamoyl)phenyl)-1-methyl-9H-carbazole-2-carboxamide (7b): brown solid; yield: 56%; m. p. 93°C; 1H NMR (600 MHz, DMSO-d6, 25°C) δ = 11.41 (s, 1H), 10.76 (s, 1H), 10.68 (s, 1H), 8.16 (d, J = 7.7 Hz, 1H), 8.13 (d, J = 2.9 Hz, 1H), 8.06 (d, J = 8.0 Hz, 1H), 7.99—7.94 (m, 2H), 7.78 (d, J = 8.9 Hz, 2H), 7.59 (dd, J = 8.7, 2.9 Hz, 1H), 7.55 (d, J = 8.1 Hz, 1H), 7.44 (d, J = 8.5 Hz, 2H), 7.31 (d, J = 8.0 Hz, 1H), 7.22—7.17 (m, 1H), 2.62 (s, 3H) ppm; MS (ESI) m/z 491.45 = [M + H]+, HRMS m/z 491.0922 = [M + H]+, calcd. for C25H19ClN4O3S m/z = 490.0866.
N'-Benzoyl-N'-(tert-butyl)-1-methyl-9H-carbazole-2-carbohydrazide (7c): white solid; yield: 79%; m. p. 212°C; 1H NMR (600 MHz, DMSO-d6, 25°C) δ = 11.23 (s, 1H), 10.53 (s, 1H), 8.08 (d, J = 7.8 Hz, 1H), 7.87 (d, J = 8.0 Hz, 1H), 7.51—7.47 (m, 3H), 7.44—7.36 (m, 4H), 7.16—7.13 (m, 1H), 6.52 (d, J = 8.0 Hz, 1H), 2.08 (s, 3H), 1.55 (s, 9H) ppm; 13C NMR (151 MHz, DMSO-d6, 25°C) δ = 172.62, 168.54, 140.91, 139.46, 138.59, 131.17, 129.71, 128.01, 127.37, 126.62, 123.45, 122.63, 121.14, 119.33, 119.21, 117.70, 117.56, 111.68, 60.59, 28.06, 13.74 ppm; MS (ESI) m/z 422.40 = [M + Na]+, HRMS m/z 400.2014 = [M + H]+, calcd. for C25H25N3O2 m/z = 399.1947.
N-(tert-Butyl)-4-methyl-N'-(1-methyl-9H-carbazole-2-carbonyl)benzenesulfonohydrazide (7d): yellow solid; yield: 85%; m. p. 97°C; 1H NMR (600 MHz, DMSO-d6, 25°C) δ = 11.33 (s, 1H), 10.31 (s, 1H), 8.14 (d, J = 7.7 Hz, 1H), 8.02—8.00 (m, 3H), 7.54 (d, J = 7.9 Hz, 1H), 7.44—7.40 (m, 3H), 7.20—7.13 (m, 2H), 2.39 (s, 3H), 1.40 (d, J = 3.2 Hz, 9H) ppm; 13C NMR (151 MHz, DMSO-d6, 25°C) δ = 169.98, 143.70, 141.00, 139.59, 139.32, 131.78, 129.74, 128.36, 126.58, 123.51, 122.76, 121.15, 119.34, 118.74, 117.59, 111.73, 63.29, 28.79, 21.50, 14.51 ppm; MS (ESI) m/z 450.23 = [M + H]+, HRMS m/z 450.1836 = [M + H]+, calcd. for C25H27N3O3S m/z = 449.1773.
1-Methyl-N-(2-oxo-2-phenylethyl)-9H-carbazole-2-carboxamide (7e): yellow solid; yield: 67%; m. p. 153°C; 1H NMR (600 MHz, DMSO-d6) δ = 11.32 (s, 1H), 8.59 (t, J = 5.7 Hz, 1H), 8.15—8.00 (m, 4H), 7.72—7.67 (m, 1H), 7.61—7.53 (m, 3H), 7.44—7.41 (m, 1H), 7.28 (d, J = 8.0 Hz, 1H), 7.19—7.17 (m, 1H), 4.80 (d, J = 5.8 Hz, 2H), 2.66 (s, 3H) ppm; 13C NMR (151 MHz, DMSO) δ = 196.02, 170.49, 140.97, 139.75, 135.63, 134.01, 133.87, 129.32, 128.37, 126.38, 123.12, 122.88, 121.05, 119.28, 119.03, 118.63, 117.69, 111.70, 46.92, 14.75 ppm; MS (ESI) m/z 343.33 = [M + H]+, HRMS m/z 343.1469 = [M + H]+, calcd. for C22H18N2O2 m/z = 342.1368.
2-(6-Chloro-9H-carbazol-2-yl)-N-(2-(methylsulfonamido)phenyl)propanamide (11a): yellow solid; yield: 64%; m. p. 163°C; 1H NMR (600 MHz, DMSO-d6, 25°C) δ = 11.39 (s, 1H), 9.56 (s, 1H), 8.79 (s, 1H), 8.18 (d, J = 2.1 Hz, 1H), 8.11 (d, J = 8.1 Hz, 1H), 7.64 (dd, J = 8.1, 1.5 Hz, 1H), 7.54—7.51 (m, 1H), 7.48 (dd, J = 8.5, 0.5 Hz, 1H), 7.36 (dd, J = 8.6, 2.1 Hz, 1H), 7.33 (dd, J = 7.9, 1.6 Hz, 1H), 7.25—7.22 (m, 2H), 7.17 (td, J = 7.7, 1.6 Hz, 1H), 4.07 (q, J = 7.0 Hz, 1H), 2.64 (s, 3H), 1.53 (d, J = 7.0 Hz, 3H) ppm; MS (ESI) m/z 442.48 = [M + H]+, HRMS m/z 442.0985 = [M + H]+, calcd. for C22H20ClN3O3S m/z = 441.0914.
2-(6-Chloro-9H-carbazol-2-yl)-N-(2-((dimethylphosphoryl)amino)phenyl)propanamide (11b): yellow solid; yield: 77%; m. p. 148°C; 1H NMR (600 MHz, DMSO-d6, 25°C) δ = 11.79 (s, 1H), 11.41 (s, 1H), 8.42 (dd, J = 8.5, 3.9 Hz, 1H), 8.17 (d, J = 2.1 Hz, 1H), 8.10 (d, J = 8.1 Hz, 1H), 7.53 (ddd, J = 13.8, 7.7, 1.6 Hz, 1H), 7.51—7.46 (m, 3H), 7.36 (dd, J = 8.6, 2.1 Hz, 1H), 7.20 (dd, J = 8.1, 1.5 Hz, 1H), 7.14—7.10 (m, 1H), 3.82 (q, J = 7.0 Hz, 1H), 1.77 (d, J = 13.6 Hz, 3H), 1.62 (d, J = 13.5 Hz, 3H), 1.54 (d, J = 7.0 Hz, 3H) ppm; 13C NMR (151 MHz, DMSO-d6, 25°C) δ = 172.56, 143.62, 143.60, 141.05, 140.28, 138.81, 132.93, 131.27, 131.20, 125.59, 124.04, 123.32, 121.21, 121.02, 120.15, 119.11, 112.82, 110.19, 48.74, 18.78 ppm; MS (ESI) m/z 425.38 = [M + H]+, HRMS m/z 425.1176 = [M + H]+, calcd. for C23H23ClN3O2P m/z = 424.1216.
2-(6-Chloro-9H-carbazol-2-yl)-N'-(p-tolyl)propanehydrazide (11c): white solid; yield: 88%; m. p. 188°C; 1H NMR (600 MHz, DMSO-d6, 25°C) δ = 11.38 (s, 1H), 9.83 (d, J = 3.2 Hz, 1H), 8.18 (d, J = 2.1 Hz, 1H), 8.09 (d, J = 8.1 Hz, 1H), 7.55 (d, J = 3.2 Hz, 1H), 7.51—7.46 (m, 2H), 7.36 (dd, J = 8.6, 2.2 Hz, 1H), 7.19 (dd, J = 8.2, 1.5 Hz, 1H), 6.84 (d, J = 8.1 Hz, 2H), 6.51—6.47 (m, 2H), 3.85 (q, J = 7.0 Hz, 1H), 2.12 (s, 3H), 1.46 (d, J = 7.0 Hz, 3H) ppm; 13C NMR (151 MHz, DMSO-d6, 25°C) δ = 173.63, 147.59, 141.02, 140.74, 138.80, 129.48, 127.40, 125.47, 124.14, 123.28, 120.87, 120.81, 120.12, 119.30, 112.80, 112.65, 110.13, 44.17, 20.54, 19.10 ppm; MS (ESI) m/z 378.18 = [M + H]+, HRMS m/z 378.1366 = [M + H]+, calcd. for C22H20ClN3O m/z = 377.1295.
2-(6-Chloro-9H-carbazol-2-yl)-N-(2-(methylsulfonyl)ethyl)propanamide (11d): white solid; yield: 73%; m. p. 149°C; 1H NMR (600 MHz, DMSO-d6, 25°C) δ = 11.38 (s, 1H), 8.31 (t, J = 5.7 Hz, 1H), 8.17 (d, J = 2.1 Hz, 1H), 8.06 (d, J = 8.1 Hz, 1H), 7.48 (d, J = 8.5 Hz, 1H), 7.43 (d, J = 1.4 Hz, 1H), 7.36 (dd, J = 8.6, 2.2 Hz, 1H), 7.13 (dd, J = 8.2, 1.5 Hz, 1H), 3.75 (q, J = 7.0 Hz, 1H), 3.47—3.40 (m, 2H), 3.25—3.17 (m, 2H) 2.90 (s, 3H), 1.41 (d, J = 7.0 Hz, 3H) ppm; 13C NMR (151 MHz, DMSO) δ = 174.26, 141.01, 140.95, 138.77, 125.46, 124.11, 123.26, 120.92, 120.75, 120.07, 119.26, 112.78, 110.12, 53.45, 46.02, 41.22, 33.35, 19.39 ppm; MS (ESI) m/z 378.90 = [M + H]+, HRMS m/z 379.0872 = [M + H]+, calcd. for C18H19ClN2O3S m/z = 378.0805.
2-(6-Chloro-9H-carbazol-2-yl)-N-(4-(N-(pyrimidin-2-yl)sulfamoyl)phenyl)propanamide (11e): yellow solid; yield: 65%; m. p. 157°C; 1H NMR (600 MHz, DMSO-d6, 25°C) δ = 11.37 (s, 1H), 10.49 (s, 1H), 8.46 (d, J = 4.9 Hz, 2H), 8.15 (d, J = 2.1 Hz, 1H), 8.08 (d, J = 8.1 Hz, 1H), 7.91—7.88 (m, 2H), 7.79—7.75 (m, 2H), 7.50—7.45 (m, 2H), 7.35 (dd, J = 8.6, 2.1 Hz, 1H), 7.20 (dd, J = 8.2, 1.5 Hz, 1H), 7.01 (t, J = 4.9 Hz, 1H), 4.00 (q, J = 6.9 Hz, 1H), 1.49 (d, J = 6.9 Hz, 3H) ppm; 13C NMR (151 MHz, DMSO) δ = 173.53, 157.37, 143.61, 141.02, 140.41, 138.80, 129.86, 129.33, 125.57, 124.05, 123.32, 121.11, 120.94, 120.12, 119.15, 118.93, 117.89, 112.81, 110.08, 46.99, 19.44 ppm; MS (ESI) m/z 506.31 = [M + H]+, HRMS m/z 506.1039 = [M + H]+, calcd. for C25H20ClN5O3S m/z = 505.0975.
4.6 General synthetic procedure for the target compounds 14a-g
The general procedure for the synthesis of carbazole hydrazones 14a-g: Various aldehyde (0.55 mmol) were added to solutions of substituted carbazole-based carbohydrazides 13 (0.5 mmol) in EtOH (6 mL), and then the mixtures were heated at reflux. After completion of reaction, the corresponding precipitation were collected by filtration, and washed with small amount of alcohol to give crude compounds, which were purified by flash chromatography and characterized by ESI-MS, HRMS, 1H NMR and 13C NMR spectra. Most of the obtained acylhydrazone derivatives are a mixed (E/Z) configuration, and so the NMR data of the mixture are linked with “and” in the follows.
2-(6-Chloro-9H-carbazol-2-yl)-N'-(2-hydroxy-3-methoxybenzylidene)propanehydrazide (14a): white solid; yield: 79%; m. p. 217°C; 1H NMR (600 MHz, DMSO-d6, 25°C) δ = 8.30 (s, 1H), 8.00—7.97 (m, 2H), 7.81—7.79 (m, 2H), 7.52—7.50 (m, 1H), 7.38 (d, J = 8.6 Hz, 1H), 7.31 (d, J = 2.0 Hz, 1H), 7.21 (dd, J = 8.2, 1.5 Hz, 1H), 7.02 (dd, J = 7.8, 1.4 Hz, 1H), 6.97 (dd, J = 8.1, 1.4 Hz, 1H), 6.84 (t, J = 8.0 Hz, 1H), 3.89 (s, 1H), 3.86 (s, 3H), 1.63—1.61 (m, 3H) ppm; 13C NMR (151 MHz, DMSO-d6, 25°C) δ = 170.11, 148.34, 147.44 and 147.40, 146.30, 141.04, 140.35, 138.81, 125.57, 124.07, 123.32, 121.10, 120.95, 120.14, 119.43, 119.32, 119.25, 114.15, 113.23 and 112.75, 112.82, 110.11, 56.25, 44.83, 19.36 ppm; MS (ESI) m/z 422.41 = [M + H]+, HRMS m/z 422.1257 = [M + H]+, calcd. for C23H20ClN3O3 m/z = 421.1193.
2-(6-Chloro-9H-carbazol-2-yl)-N'-(3-(4-chlorophenyl)allylidene)propanehydrazide (14b): white solid; yield: 82%; m. p. 186°C; 1H NMR (600 MHz, DMSO-d6, 25°C) δ = 11.46 and 11.32 (s, 1H), 11.37 and 11.24 (s, 1H), 8.17 and 8.15 (d, J = 2.1 Hz, 1H), 8.09 and 8.07 (d, 8.1 Hz, 1H), 7.96 and 7.77 (dd, J = 6.0, 2.4 Hz, 1H), 7.62 (dd, J = 10.1, 7.8 Hz, 2H), 7.49—7.41 (m, 4H), 7.35 (td, J = 8.8, 2.2 Hz, 1H), 7.17 (dt, J = 8.2, 1.4 Hz, 1H), 7.00—6.95 (m, 2H), 1.46 (t, J = 7.3 Hz, 3H) ppm; 13C NMR (151 MHz, DMSO-d6, 25°C) δ = 170.26, 148.69, 141.02, 140.58, 138.80, 137.26, 130.13, 129.29, 129.18, 129.14, 126.87, 121.04, 120.93, 120.12, 112.81, 110.07, 44.89, 40.40, 40.27, 40.13, 39.99, 39.85, 39.71, 39.57, 29.55, 29.49, 29.04, 22.56, 19.45 and 19.07 ppm; MS (ESI) m/z 436.34 = [M + H]+, HRMS m/z 436.0976 = [M + H]+, calcd. for C24H19Cl2N3O m/z = 435.0905.
N'-(Benzo[d][1,3]dioxol-5-ylmethylene)-2-(6-chloro-9H-carbazol-2-yl)propanehydrazide (14c): white solid; yield: 84%; m. p. 240°C; 1H NMR (600 MHz, DMSO-d6, 25°C) δ = 11.45 and 11.37 (s, 1H), 11.34 and 11.18 (s, 1H), 8.15 and 814 (d, J = 2.1 Hz, 1H), 8.11 and 7.82 (s, 1H), 8.07 and 8.06 (d, J = 8.1 Hz, 1H), 7.49—7.44 (m, 2H), 7.35 (m, 1H), 7.29 and 7.21 (d, J = 1.6 Hz, 1H), 7.20—7.18 (m, 1H), 7.11 and 7.06 (dd, J = 8.0, 1.6 Hz, 1H), 6.95 (d, J = 5.5 Hz, 1H), 6.09 (d, J = 1.0 Hz, 1H), 6.07 (d, J = 1.0 Hz, 1H), 4.82 and 3.83 (q, J = 7.0 Hz, 1H), 1.47 (d, J = 7.0 Hz, 3H) ppm; 13C NMR (151 MHz, DMSO-d6, 25°C) δ = 175.43 and 170.17, 149.44 and 149.19, 148.44 and 148.38, 146.83 and 142.78, 141.20 and 141.06, 141.03 and 140.63, 138.80 and 138.73, 129.25 and 129.14, 125.53 and 125.43, 124.10 and 124.09, 123.57 and 123.34, 123.30 and 123.24, 121.01 and 120.96, 120.87 and 120.64, 120.11 and 120.04, 119.61 and 119.26, 112.80 and 112.74, 110.15 and 110.08, 108.88, 105.53 and 105.29, 101.97 and 101.93, 44.90 and 41.53, 19.49 and 19.38 ppm; MS (ESI) m/z 442.01 = [M + Na]+, HRMS m/z 420.1110 = [M + H]+, calcd. for C23H18ClN3O3 m/z = 419.1037.
N'-((1H-Indol-3-yl)methylene)-2-(6-chloro-9H-carbazol-2-yl)propanehydrazide (14d): white solid; yield: 78%; m. p. 209°C; 1H NMR (600 MHz, DMSO-d6, 25°C) δ = 11.52 and 11.49 (d, J = 2.7 Hz, 1H), 11.37 and 11.32 (s, 1H), 11.20 and 11.00 (s, 1H), 8.36 and 8.13 (s, 1H), 8.21—8.11 (m, 2H), 8.09—8.05 (d, J = 8.1 Hz, 1H), 7.75 and 7.71 (d, J = 2.8 Hz, 1H), 7.52 and 7.50 (s, 1H), 7.47 and 7.44 (d, J = 0.5 Hz, 1H), 7.43—7.40 (m, 1H), 7.35 and 7.32 (dd, J = 8.6, 2.2 Hz, 1H), 7.27—7.20 (m, 2H), 7.19—7.09 (m, 1H), 4.91 and 3.84 (q, J = 7.0 Hz, 1H), 1.51 (d, J = 3.5 Hz, 3H) ppm; 13C NMR (151 MHz, DMSO-d6, 25°C) δ = 174.75 and 169.51, 144.17 and 141.37, 141.07 and 141.05, 141.02 and 140.76, 138.80 and 138.73, 137.47 and 137.44, 130.59 and 130.54, 125.48 and 125.39, 124.74 and 124.51, 124.12, 123.27 and 123.21, 123.01, 122.35 and 122.09, 120.95 and 120.80, 120.73 and 120.61, 120.09 and 120.00, 119.57 and 119.33, 112.78 and 112.71, 112.30 and 112.23, 112.05 and 111.96, 110.10 and 110.06, 44.88 and 41.46, 19.75 and 19.49 ppm; MS (ESI) m/z 415.26 = [M + H]+, HRMS m/z 415.1324 = [M + H]+, calcd. for C24H19ClN4O m/z = 414.1247.
2-(6-Chloro-9H-carbazol-2-yl)-N'-(4-hydroxy-3-methoxybenzylidene)propanehydrazide (14e): white solid; yield: 90%; m. p. 239°C; 1H NMR (600 MHz, DMSO-d6, 25°C) δ = 11.37 and 11.30 (s, 1H), 11.36 and 11.12 (s, 1H), 9.49 and 9.45 (s, 1H), 8.17—6.78 (m, 10H), 4.78 and 3.82 (q, J = 7.0 Hz, 1H), 3.86 and 3.79 (s, 3H), 1.48 and 1.45 (d, J = 7.0 Hz, 3H) ppm; 13C NMR (151 MHz, DMSO-d6, 25°C) δ = 175.23 and 170.00, 149.32 and 148.95, 148.43, 147.54 and 143.19, 141.47 and 140.74, 141.07 and 141.03, 138.80 and 138.73, 126.27 and 126.12, 125.52 and 125.43, 124.13 and 124.09, 123.30 and 123.25, 122.41 and 121.65, 120.99, 120.84 and 120.61, 120.11 and 120.03, 119.71 and 119.25, 115.87 and 115.83, 112.80 and 112.72, 110.05 and 110.03, 109.35 and 109.27, 56.00 and 55.97, 44.87 and 41.85, 19.58 and 19.42 ppm; MS (ESI) m/z 422.13 = [M + H]+, HRMS m/z 422.1266 = [M + H]+, calcd. for C23H20ClN3O3 m/z = 421.1193.
2-(6-Chloro-9H-carbazol-2-yl)-N'-(4-chlorobenzylidene)propanehydrazide (14f): white solid; yield: 85%; m. p. 218°C; 1H NMR (600 MHz, DMSO-d6, 25°C) δ = 11.61 and 11.35 (s, 1H), 11.37 and 11.32 (s, 1H), 8.19 and 7.89 (s, 1H), 8.16 and 8.13 (d, J = 2.1 Hz, 1H), 8.09 and 8.05 (d, J = 8.1 Hz, 1H), 7.71 and 7.68 (s, 1H), 7.70 and 7.67 (s, 1H), 7.51—7.44 (m, 4H), 7.37—7.33 (m, 1H), 7.20—7.18 (m, 1H), 4.82 and 3.86 (q, J = 7.0 Hz, 1H), 1.48 (d, J = 7.0 Hz, 3H) ppm; 13C NMR (151 MHz, DMSO-d6, 25°C) δ = 175.64 and 170.44, 145.71, 141.70, 141.03, 140.94 and 140.48, 138.81 and 138.73, 134.82 and 134.52, 133.77 and 133.71, 129.37 and 129.33, 129.06 and 128.81, 125.55 and 125.47, 124.08, 123.31 and 123.26, 121.05 and 121.01, 120.91 and 120.68, 120.13 and 120.05, 119.54 and 119.25, 112.81 and 112.76, 110.32 and 110.10, 44.93, 41.63, 19.38 and 19.35 ppm; MS (ESI) m/z 410.15 = [M + H]+, HRMS m/z 410.0798 = [M + H]+, calcd. for C22H17ClN3O m/z = 409.0749.
2-(6-Chloro-9H-carbazol-2-yl)-N'-(2,4-dichlorobenzylidene)propanehydrazide (14g): white solid; yield: 82%; m. p. 241°C; 1H NMR (600 MHz, DMSO-d6, 25°C) δ = 11.83 and 11.53 (s, 1H), 11.38 and 11.32 (s, 1H), 8.54 and 8.24 (s, 1H), 8.17 and 8.13 (d, J = 2.1 Hz, 1H), 8.10 and 8.06 (d, J = 8.1 Hz, 1H), 8.02 and 7.91 (d, J = 8.5 Hz, 1H), 7.69 and 7.67 (d, J = 2.1 Hz, 1H), 7.54—7.45 (m, 3H), 7.37—7.33 (m, 1H), 7.19 and 7.18 (d, J = 1.5 Hz, 1H), 4.81 and 3.85 (q, J = 7.0 Hz, 1H), 1.48 (d, J = 7.0 Hz, 3H) ppm; 13C NMR (151 MHz, DMSO-d6, 25°C) δ = 175.76 and 170.56, 141.80 and 141.03, 140.83 and 140.29, 138.82 and 138.74, 137.98, 135.42 and 135.12, 134.16 and 133.96, 131.14 and 131.07, 129.81 and 129.79, 128.47, 128.43 and 128.30, 125.58 and 125.50, 124.06, 123.33 and 123.28, 121.12 and 121.07, 120.96 and 120.71, 120.14 and 120.06, 119.49 and 119.21, 112.83 and 112.78, 110.30 and 110.13, 45.11 and 41.73, 19.38 and 19.36 ppm; MS (ESI) m/z 443.92 = [M + H]+, HRMS m/z 444.0430 = [M + H]+, calcd. for C22H16Cl3N3O m/z = 443.0359.
4.7 Crystal structure determination of 14a
Crystal Data for C23H20ClN3O3 (M = 421.87 g/mol): triclinic, space group P-1 (no. 2), a = 10.7893 (14) Å, b = 13.0199 (15) Å, c = 15.497 (2) Å, α = 111.749 (12)°, β = 100.232 (11)°, γ = 90.146 (10)°, V = 1984.2 (5) Å3, Z = 4, T = 149.99 (10) K, μ(Cu Kα) = 1.966 mm−1, Dcalc = 1.412 g/cm3, 13,393 reflections measured (7.33° ≤ 2Θ ≤ 148.834°), 7661 unique (Rint = 0.1060, Rsigma = 0.1452) which were used in all calculations. The final R1 was 0.1024 (I > 2σ(I)) and wR2 was 0.2727 (all data).
4.8 In vitro cytotoxicity assays
The in vitro cytotoxicity of these carbazole derivatives against human melanoma (A875), human gastric adenocarcinoma cells (7901), and a subclone of African green monkey kidney cell line MA-104 (MARC145) cell lines was evaluated using the MTT assay. Briefly, A875, 7901, and MARC145 cells were seeded at 2 × 104 cells per well in 96-well plates and grown to subconfluence. After removal of the growth medium, six serial dilutions of each tested compound in 200 µL test medium were added. Plates were incubated at 37°C in a humidified atmosphere containing 5% CO2. After 72 h of exposure, the culture medium was removed and 30 µL of the MTT solution (5 mg/mL in PBS) was added to each well. The plate was further incubated for 4 h to allow MTT formazan formation. To dissolve the resulting MTT formazan, 50 µL of DMSO was added to each well, followed by thorough mixing with a microplate shaker. Absorbance at 570 nm was measured on a microplate reader (Thermo Scientific, MK3). All data were analyzed with SPSS software, and the 50% inhibitory concentrations (IC50) of each compound for the different cell lines were determined. All assays were performed in triplicate on three independent experiments.
Data availability statement
The original contributions presented in the study are included in the article/Supplementary Material, further inquiries can be directed to the corresponding authors.
Author contributions
Conceptualization, XC and SK; methodology, ZG; validation, YC, YN, and KC; formal analysis, ZG and XC; writing—original draft preparation, ZG; writing—review and editing, XC and SK; funding acquisition, XC All authors have read and agreed to the published version of the manuscript.
Funding
This work was financially supported by the Natural Science Foundation of Hubei Province (2022CFB244), and the National Innovation and Entrepreneurship Training Program for College Students (202210504083).
Conflict of interest
The authors declare that the research was conducted in the absence of any commercial or financial relationships that could be construed as a potential conflict of interest.
Publisher’s note
All claims expressed in this article are solely those of the authors and do not necessarily represent those of their affiliated organizations, or those of the publisher, the editors and the reviewers. Any product that may be evaluated in this article, or claim that may be made by its manufacturer, is not guaranteed or endorsed by the publisher.
Supplementary material
The Supplementary Material for this article can be found online at: https://www.frontiersin.org/articles/10.3389/fchem.2023.1104868/full#supplementary-material
References
Aggarwal, T. S., and Verma, A. K. (2019). Recent advances in the synthesis of carbazoles from indoles. Org. Biomol. Chem. 17 (36), 8330–8342. doi:10.1039/c9ob01381d
Bailly, C. (2020). Anticancer properties and mechanism of action of the quassinoid ailanthone. Phytother. Res. 34 (9), 2203–2213. doi:10.1002/ptr.6681
Bhutani, P., Joshi, G., Raja, N., Bachhav, N., Rajanna, P. K., Bhutani, H., et al. U.S. (2021). U.S. FDA approved drugs from 2015–june 2020: A perspective. J. Med. Chem. 64 (5), 2339–2381. doi:10.1021/acs.jmedchem.0c01786
Cao, S.-Y., Li, Y., Meng, X., Zhao, C.-N., Li, S., Gan, R.-Y., et al. (2019). Dietary natural products and lung cancer: Effects and mechanisms of action. J. Funct. Foods 52, 316–331. doi:10.1016/j.jff.2018.11.004
Caron, G., Digiesi, V., Solaro, S., and Ermondi, G. (2020). Flexibility in early drug discovery: Focus on the beyond-Rule-of-5 chemical space. Drug Discov. Today 25 (4), 621–627. doi:10.1016/j.drudis.2020.01.012
Chen, Y., Tang, C., Wu, Y., Mo, S., Wang, S., Yang, G., et al. (2015). Glycosmisines A and B: Isolation of two new carbazole–indole-type dimeric alkaloids from glycosmis pentaphylla and an evaluation of their antiproliferative activities. Org. Biomol. Chem. 13 (24), 6773–6781. doi:10.1039/c5ob00695c
Dang, Z., Xu, S., Zhang, H., Gui, W., Zhao, Y., Duan, L., et al. (2018). In vitro and in vivo efficacies of carbazole aminoalcohols in the treatment of alveolar echinococcosis. Acta Trop. 185, 138–143. doi:10.1016/j.actatropica.2018.05.007
Davison, E. K., and Sperry, J. (2017). Natural products with heteroatom-rich ring systems. J. Nat. Prod. 80 (11), 3060–3079. doi:10.1021/acs.jnatprod.7b00575
Duangdee, N., Mahavorasirikul, W., and Prateeptongkum, S. (2020). Design synthesis and anti-proliferative activity of some new coumarin substituted hydrazide–hydrazone derivatives. J. Chem. Sci. 132 (1), 66–12. doi:10.1007/s12039-020-01767-4
Fang, Y., Li, H., Ji, B., Cheng, K., Wu, B., Li, Z., et al. (2021). Renieramycin-type alkaloids from marine-derived organisms: Synthetic chemistry, biological activity and structural modification. Eur. J. Med. Chem. 210, 113092. doi:10.1016/j.ejmech.2020.113092
Favia, A. D., Habrant, D., Scarpelli, R., Migliore, M., Albani, C., Bertozzi, S. M., et al. (2012). Identification and characterization of carprofen as a multitarget fatty acid amide hydrolase/cyclooxygenase inhibitor. J. Med. Chem. 55 (20), 8807–8826. doi:10.1021/jm3011146
Greco, G., Catanzaro, E., and Fimognari, C. (2021). Natural products as inducers of non-canonical cell death: A weapon against cancer. Cancers (Basel) 13 (2), 304. doi:10.3390/cancers13020304
Gu, Y., Huang, W., Chen, S., and Wang, X. (2018). Bismuth(III) triflate catalyzed three-component reactions of indoles, ketones, and alpha-bromoacetaldehyde acetals enable indole-to-carbazole transformation. Org. Lett. 20 (14), 4285–4289. doi:10.1021/acs.orglett.8b01707
Han, Y., Tian, Y., Wang, R., Fu, S., Jiang, J., Dong, J., et al. (2020). Design, synthesis and biological evaluation of thieno[3, 2-d]pyrimidine derivatives containing aroyl hydrazone or aryl hydrazide moieties for PI3K and mTOR dual inhibition. Bioorg Chem. 104, 104197. doi:10.1016/j.bioorg.2020.104197
Huang, W., Gao, Z., Zhang, Z., Fang, W., Wang, Z., Wan, Z., et al. (2021). Selective and effective anticancer agents: Synthesis, biological evaluation and structure-activity relationships of novel carbazole derivatives. Bioorg Chem. 113, 104991. doi:10.1016/j.bioorg.2021.104991
Issa, S., Prandina, A., Bedel, N., Rongved, P., Yous, S., Le Borgne, M., et al. (2019). Carbazole scaffolds in cancer therapy: A review from 2012 to 2018. J. Enzyme Inhib. Med. Chem. 34 (1), 1321–1346. doi:10.1080/14756366.2019.1640692
Kong, X., Zhang, H., Cao, C., Zhou, S., Pang, G., and Shi, Y. (2016). Synthesis of fluorinated carbazoles via C-H arylation catalyzed by Pd/Cu bimetal system and their antibacterial activities. Bioorg Med. Chem. 24 (6), 1376–1383. doi:10.1016/j.bmc.2016.02.013
Liu, L. X., Wang, X. Q., Zhou, B., Yang, L. J., Li, Y., Zhang, H. B., et al. (2015). Synthesis and antitumor activity of novel N-substituted carbazole imidazolium salt derivatives. Sci. Rep. 5, 13101. doi:10.1038/srep13101
Liu, Y. P., Hu, S., Liu, Y. Y., Zhang, M. M., Zhang, W. H., Qiang, L., et al. (2019). Anti-inflammatory and antiproliferative prenylated carbazole alkaloids from Clausena vestita. Bioorg Chem. 91, 103107. doi:10.1016/j.bioorg.2019.103107
Liu, Y. P., Li, Y. J., Zhao, Y. Y., Guo, J. M., Liu, Y. Y., Wang, X. P., et al. (2021). Carbazole alkaloids from the fruits of Clausena anisum-olens with potential PTP1B and alpha-glucosidase inhibitory activities. Bioorg Chem. 110, 104775. doi:10.1016/j.bioorg.2021.104775
Liu, Y., Wu, Y., Sun, L., Gu, Y., and Hu, L. (2020). Synthesis and structure-activity relationship study of water-soluble carbazole sulfonamide derivatives as new anticancer agents. Eur. J. Med. Chem. 191, 112181. doi:10.1016/j.ejmech.2020.112181
Mellini, P., Carafa, V., Di Rienzo, B., Rotili, D., De Vita, D., Cirilli, R., et al. (2012). Carprofen analogues as sirtuin inhibitors: Enzyme and cellular studies. ChemMedChem 7 (11), 1905–1908. doi:10.1002/cmdc.201200318
Murali, K., Sparkes, H. A., and Rajendra Prasad, K. J. (2017). Synthesis of hetero annulated isoxazolo-pyrido- and pyrimido carbazoles: Screened for in vitro antitumor activity and structure activity relationships, a novel 2-amino-4-(3'-bromo-4'-methoxyphenyl)-8-chloro-11H-pyrimido[4, 5-a]carbazole as an antitumor agent. Eur. J. Med. Chem. 128, 319–331. doi:10.1016/j.ejmech.2017.02.009
Newman, D. J., and Cragg, G. M. (2020). Natural products as sources of new drugs over the nearly four decades from 01/1981 to 09/2019. J. Nat. Prod. 83 (3), 770–803. doi:10.1021/acs.jnatprod.9b01285
Padmaja, P., Koteswara Rao, G., Indrasena, A., Subba Reddy, B. V., Patel, N., Shaik, A. B., et al. (2015). Synthesis and biological evaluation of novel pyrano[3, 2-c]carbazole derivatives as anti-tumor agents inducing apoptosis via tubulin polymerization inhibition. Org. Biomol. Chem. 13 (5), 1404–1414. doi:10.1039/c4ob02015d
Peng, S., Wang, Y., Zhou, Y., Ma, T., Wang, Y., Li, J., et al. (2019). Rare ginsenosides ameliorate lipid overload-induced myocardial insulin resistance via modulating metabolic flexibility. Phytomedicine 58, 152745. doi:10.1016/j.phymed.2018.11.006
Peng, X., Sun, Z., Kuang, P., Li, L., Chen, J., and Chen, J. (2020). Copper-catalyzed selective arylation of nitriles with cyclic diaryl iodonium salts: Direct access to structurally diversified diarylmethane amides with potential neuroprotective and anticancer activities. Org. Lett. 22 (15), 5789–5795. doi:10.1021/acs.orglett.0c01829
Percy, S., Mancand, D. L. C., Belica, P. S., Wong, F., Harry, S., and Wnng, L. B. (1994). Syntheses of carprofen, a carbazole-based nonsteroidal anti-inflammatory agent. Heterocycles 39 (2), 833–845. doi:10.3987/com-94-s(b)83
Popiolek, L., Piatkowska-Chmiel, I., Gawronska-Grzywacz, M., Biernasiuk, A., Izdebska, M., Herbet, M., et al. (2018). New hydrazide-hydrazones and 1, 3-thiazolidin-4-ones with 3-hydroxy-2-naphthoic moiety: Synthesis, in vitro and in vivo studies. Biomed. Pharmacother. 103, 1337–1347. doi:10.1016/j.biopha.2018.04.163
Rassias, G., Zogali, V., Swarbrick, C. M. D., Ki Chan, K. W., Chan, S. A., Gwee, C. P., et al. (2019). Cell-active carbazole derivatives as inhibitors of the zika virus protease. Eur. J. Med. Chem. 180, 536–545. doi:10.1016/j.ejmech.2019.07.007
Sun, L., Wang, C., Hu, X., Wu, Y., Jiang, Z., Li, Z., et al. (2020). Design, synthesis, and evaluations of the antiproliferative activity and aqueous solubility of novel carbazole sulfonamide derivatives as antitumor agents. Bioorg Chem. 99, 103766. doi:10.1016/j.bioorg.2020.103766
Sun, L., Wu, Y., Liu, Y., Chen, X., and Hu, L. (2017). Novel carbazole sulfonamide derivatives of antitumor agent: Synthesis, antiproliferative activity and aqueous solubility. Bioorg Med. Chem. Lett. 27 (2), 261–265. doi:10.1016/j.bmcl.2016.11.068
Sung, H., Ferlay, J., Siegel, R. L., Laversanne, M., Soerjomataram, I., Jemal, A., et al. (2021). Global cancer statistics 2020: GLOBOCAN estimates of incidence and mortality worldwide for 36 cancers in 185 countries. CA Cancer J. Clin. 71 (3), 209–249. doi:10.3322/caac.21660
Thomas, S. M., Purmal, A., Pollastri, M., and Mensa-Wilmot, K. (2016). Discovery of a carbazole-derived lead drug for human african trypanosomiasis. Sci. Rep. 6, 32083. doi:10.1038/srep32083
Wang, G., Sun, S., and Guo, H. (2022). Current status of carbazole hybrids as anticancer agents. Eur. J. Med. Chem. 229, 113999. doi:10.1016/j.ejmech.2021.113999
Wang, W., Li, Q., Wei, Y., Xue, J., Sun, X., Yu, Y., et al. (2017). Novel carbazole aminoalcohols as inhibitors of beta-hematin formation: Antiplasmodial and antischistosomal activities. Int. J. Parasitol. Drugs Drug Resist 7 (2), 191–199. doi:10.1016/j.ijpddr.2017.03.007
Wang, Y., Yao, H., Hua, M., Jiao, Y., He, H., Liu, M., et al. (2020). Direct N-glycosylation of amides/amines with glycal donors. J. Org. Chem. 85 (11), 7485–7493. doi:10.1021/acs.joc.0c00975
Wu, X. T., Xiao, E. K., Ma, F., Yin, J., Wang, J., Chen, P., et al. (2021). Substrate-controlled regiodivergent synthesis of fluoroacylated carbazoles via Friedel-Crafts acylation. J. Org. Chem. 86 (9), 6734–6743. doi:10.1021/acs.joc.1c00473
Xie, J., Xu, W., Song, H., Liu, Y., Zhang, J., and Wang, Q. (2020). Synthesis and antiviral/fungicidal/insecticidal activities study of novel chiral indole diketopiperazine derivatives containing acylhydrazone moiety. J. Agric. Food Chem. 68 (20), 5555–5571. doi:10.1021/acs.jafc.0c00875
Xie, Z., Wei, Y., Xu, J., Lei, J., and Yu, J. (2019). Alkaloids from piper nigrum synergistically enhanced the effect of paclitaxel against paclitaxel-resistant cervical cancer cells through the downregulation of Mcl-1. J. Agric. Food Chem. 67 (18), 5159–5168. doi:10.1021/acs.jafc.9b01320
Yang, Y., Liu, Q., Shi, X., Zheng, Q., Chen, L., and Sun, Y. (2021). Advances in plant-derived natural products for antitumor immunotherapy. Arch. Pharm. Res. 44 (11), 987–1011. doi:10.1007/s12272-021-01355-1
Yokoyama, T., Yukuhiro, M., Iwasaki, Y., Tanaka, C., Sankoda, K., Fujiwara, R., et al. (2019). Identification of candidate molecular targets of the novel antineoplastic antimitotic NP-10. Sci. Rep. 9 (1), 16825. doi:10.1038/s41598-019-53259-2
Keywords: carbazole derivatives, synthesis, biological evaluation, anticancer, SARs
Citation: Gao Z, Chen Y, Nie Y, Chen K, Cao X and Ke S (2023) Structural diversity-guided optimization of carbazole derivatives as potential cytotoxic agents. Front. Chem. 11:1104868. doi: 10.3389/fchem.2023.1104868
Received: 22 November 2022; Accepted: 09 January 2023;
Published: 18 January 2023.
Edited by:
Giovanna Li Petri, Ri.MED Foundation, ItalyReviewed by:
Mohammad Abrar Alam, Arkansas State University, United StatesNarsimha Sirassu, Chaitanya (Deemed to be University), India
Copyright © 2023 Gao, Chen, Nie, Chen, Cao and Ke. This is an open-access article distributed under the terms of the Creative Commons Attribution License (CC BY). The use, distribution or reproduction in other forums is permitted, provided the original author(s) and the copyright owner(s) are credited and that the original publication in this journal is cited, in accordance with accepted academic practice. No use, distribution or reproduction is permitted which does not comply with these terms.
*Correspondence: Xiufang Cao, Y2FveGl1ZmFuZ0BtYWlsLmh6YXUuZWR1LmNu; Shaoyong Ke, c2hhb3lvbmcua2VAbmJlcmMuY29t