- 1Nano-BioTechnology Group, Department of Biotechnology, Faculty of Bioscience Engineering, Ghent University, Ghent, Belgium
- 2Bavarian Polymer Institute, Friedrich-Alexander-Universität Erlangen-Nürnberg, Bayreuth, Germany
Hybrid materials or hybrids incorporating organic and inorganic constituents are emerging as a very potent and promising class of materials due to the diverse but complementary nature of their properties. This complementarity leads to a perfect synergy of properties of the desired materials and products as well as to an extensive range of their application areas. Recently, we have overviewed and classified hybrid materials describing inorganics-in-organics in Part-I (Saveleva, et al., Front. Chem., 2019, 7, 179). Here, we extend that work in Part-II describing organics–on-inorganics, i.e., inorganic materials modified by organic moieties, their structure and functionalities. Inorganic constituents comprise of colloids/nanoparticles and flat surfaces/matrices comprise of metallic (noble metal, metal oxide, metal-organic framework, magnetic nanoparticles, alloy) and non-metallic (minerals, clays, carbons, and ceramics) materials; while organic additives can include molecules (polymers, fluorescence dyes, surfactants), biomolecules (proteins, carbohydtrates, antibodies and nucleic acids) and even higher-level organisms such as cells, bacteria, and microorganisms. Similarly to what was described in Part-I, we look at similar and dissimilar properties of organic-inorganic materials summarizing those bringing complementarity and composition. A broad range of applications of these hybrid materials is also presented whose development is spurred by engaging different scientific research communities.
1 Introduction
The growth of research in the area of hybrid materials is continuing to be robust, benefiting from advanced processing methods (Lutich et al., 2009; Yang et al., 2019; Ariga et al., 2020), emerging technologies (Moreels et al., 2008; Zhu et al., 2021) and materials (Gaponik and Rogach, 2010; Yan et al., 2014; Zuaznabar-Gardona and Fragoso, 2018; Karthick et al., 2022), which facilitate tremendous growth and establish interconnections between associated scientific research areas and create new functionalities (Balazs et al., 2006; Christau et al., 2014; Leroux et al., 2015; Cui et al., 2016; Gao et al., 2016; Taubert et al., 2019; Cao et al., 2022; Stavitskaya et al., 2022). In the past, different approaches to the classification of hybrid materials have been discussed: those based on the interactions (Sanchez and Soler-Illia, 2006), wherein those associated with van der Waals, hydrogen bonding, and electrostatics are distinguished from those based on covalent and no-covalent bonds. On the other hand, distinction was made based on their composition (Kickelbick, 2003; Saveleva et al., 2019).
Recently and in the latter work, classification of “hybrid materials” was performed by Saveleva and co-authors, where modification of organic materials by inorganics was analyzed (Saveleva et al., 2019). It was emphasized that perfect complementarity of these two types of materials stemming from the fact that they are used to improve their respective properties or to bring-in additional functionalities into resultant hybrid materials (Ruiz-Hitzky et al., 2008; Wang et al., 2018b; Guo et al., 2020; Li et al., 2021a). A typically highlighted example is an assembly of hard and soft–two antagonist properties–materials which are different but complementary in regard with their properties. Peculiarly, such modifications have been used even from the time of ancient Greece (Sanchez et al., 2005) to particle-enhanced tires fabricated using carbon black, ZnO, MgS with rubber (Goodyear, 1856), extending to one a very practical material Bakelite produced by Leo Baekeland by admixing clays with resin (Baekeland, 1909). In contemporary research and development areas, functionalities and applications of hybrid materials continue to be extended, including, for example, water purification (Rigoletto et al., 2022) and antibacterial properties (Chen et al., 2022).
Hybrids were structurally and compositionally classified as follows (Saveleva et al., 2019):
i) Inorganic-modified organic materials (inorganics-in-organics);
ii) Organic molecule-modified inorganic materials (organics-on-inorganics), which can be sub-divided into:
a) Colloids and nanoparticles functionalized by organic molecules;
b) Inorganic structures modified and functionalized by organic molecules.
This review discusses the modification of inorganic materials and matrices by organic molecules, organics-on-inorganics (ii). The structure of these hybrid materials is discussed together with interactions of constituent blocks and applications. It thus provides the second part of the previously described Part-I by (Saveleva et al., 2019), which discussed inorganics-in-organics.
The hierarchy or classification of organics-on-inorganics is presented in Figure 1 and is extended analysing their composition, structure, chemical bonds, properties and functionalities, and applications. There, Part-I (Saveleva et al., 2019) is seen in grey rectangles (right-hand side), which introduced some modifications and functions of inorganics covering a range of materials from inorganic minerals, clays, metals, semiconductors, carbons, ceramics to organic ones: hydrogels, layer-by-layer (LbL) assembled polymer structures, brushes, and so on. Here, we focus on modifying inorganic particles, structures, and matrices with organic molecules–highlighted part (on the left-hand side) in Figure 1—and discuss their composition, interactions, and applications. Then, organic molecules and modifiers (organics) are introduced identifying the range of properties they can enable. Subsequently, we generally describe hybrid materials, summarize their properties, and provide conclusions and outlook.
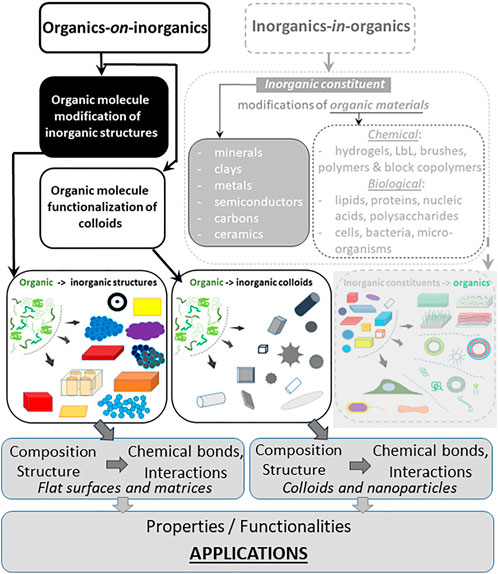
FIGURE 1. General classification of hybrid materials incorporating both organic and inorganic components. Adsorption of organic molecules on inorganic particles, structures, or matrices, referred to as organics-in-inorganics, is shown on the right-hand side in the grey-dashed rectangle constituted the Part-I of this classification (Saveleva et al., 2019). Functionalization of both inorganic colloidal particles and flat surfaces by organic molecules referred to as organics-on-inorganics, shown on the left-hand side, is the focus of this review. The bottom rows depict composition, chemical bonds, properties, and functionalities leading to development of a broad range of applications.
2 Inorganic colloidal particle functionalization by organic molecules
Developments in the area of colloidal particles has had a particularly profound impact in the field of biomedical applications including bioactive molecule sensing, targeted drug delivery, release systems, selective imaging agents, and diagnostics (Parakhonskiy et al., 2014; Tarakanchikova et al., 2020; Zhou et al., 2021). One of the reasons nanoparticles (NPs) are appealing for applications in biomedicine is because nano-size objects possess unique physicochemical properties, especially in a combination with an external electromagnetic field, which can mediate biological responses, for example, enhancing 1O2 generation (Sun et al., 2020a). Arguably, one of the most notable advantages brought by the NPs is the enhanced permeability and retention (EPR), which is considered to be a “golden standard” for designing new anticancer agents. Due to the EPR passive targeting and active endocytosis, cellular uptake (Figure 2) of nanocarriers could significantly improve anticancer/tumour drug efficiency and reduce their side effect and even rediscover drugs that may have tested failed clinically applications (Duan et al., 2019; McMahon et al., 2020; Peer et al., 2020; Majumder and Minko, 2021).
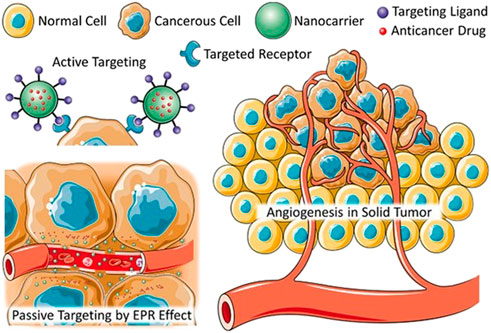
FIGURE 2. Schematic diagram of active and passive absorption of nanomedicines (Majumder and Minko, 2021), with the permission of the Wiley Online Library.
Compared with organic NPs, inorganic NPs’ inherent unique conductivity, magnetism, optical properties and variety in size, structure, and shape have become the hot spot in therapeutic diagnosis (Tan et al., 2018; Wang et al., 2018a; Sun et al., 2020b), whereas one of the broadest areas of research applying organic NPs is drug delivery. For example, superparamagnetic NPs can enhance magnetic resonance imaging (MRI) contrast, Au NPs are widely used in catalysis, drug delivery, imaging and photothermal therapy (Wang et al., 2006; Cui et al., 2014; Xiong et al., 2014; Fraire et al., 2019), while carbon quantum dots (CQDs) have inspired development of numerous applications particularly in biosensing and bioimaging due to their optical and fluorescence emission properties (Lim et al., 2015). Further, Figure 3 summarizes inorganic colloidal structures often functionalized with denoted organic molecules. Figure 4 summarizes applications of inorganic colloids modified by organics, particularly relevant in biomedical applications, and Table 1 summarizes selected applications of hybrid colloidal particles, where biomedicine is identified to be one of the most widely used application areas.
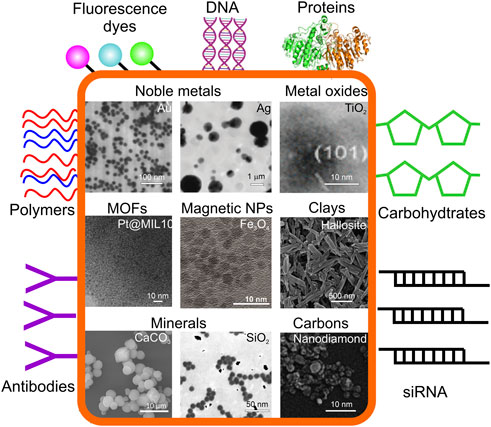
FIGURE 3. Organic molecules often used for inorganic NP functionalization in biomedical applications. The inorganic constituents (inside orange frame): noble metals (TEM images of Au NPs and Ag NPs reproduced from (Parakhonskiy et al., 2010), with permission from the RSC); metal oxides (TEM image of TiO2 NPS reproduced from (Sang et al., 2014) with permission from ACS); metal organic framework (MOFs; TEM image of Pt@MIL-101 reproduced from (Aijaz et al., 2012) with permission from ACS); magnetic NPs (TEM image of Fe3O4 reproduced from (Kozlova et al., 2020), with permission from MDPI); clays (TEM image of halloysite nanotubes reproduced from (Lvov et al., 2008), with permission from ACS), minerals (SEM image of CaCO3 microparticles reproduced from (Abalymo v et al., 2022), with permission from Elsivier, TEM image of SiO2 reproduced from (Ung et al., 2001), with permission from J. Phys.Chem.B), diamonds (TEM image of nanodiamonds reproduced from (Smith et al., 2009) with permission from Wiley-VCH. Organic molecules used for functionalization of inorganic particles and nanostructures are shown outside of the orange frame.
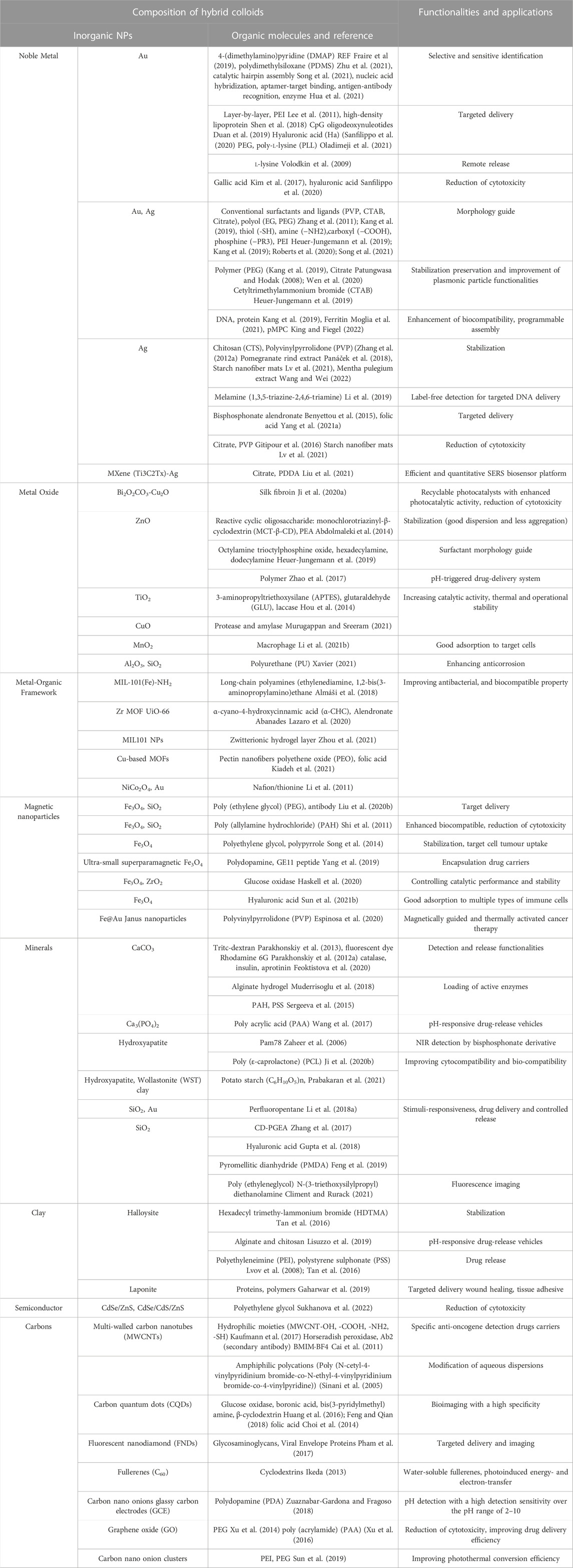
TABLE 1. The overview of the studies of hybrid organics-on-inorganics colloidal and their composition, feature/functionalities.
2.1 Stabilization
2.1.1 The meaning and theory behind colloidal stabilization
Colloids are referred to as a system consisting of nanoparticles with the sizes from 10 nm to ∼1 μm dispersed in a fluid, most frequently the liquid phase. The Derjaguin–Landau–Verwey–Overbeek (DLVO) theory can quantify effectively the colloidal stability, but it can only work for aqueous solutions containing simple electrolytes. Briefly, the balance of attractive van der Waals (vdW) (Figure 5A) and repulsive forces caused by the electrostatic double layer (EDL) (Figure 5B) determines the colloidal stability in an aqueous suspension. Actually, besides vdW and EDL, steric repulsion (Figure 5C) inherent to macromolecules such as synthetic polymers or proteins could dramatically enhance the colloidal stability due to osmotic pressure and elastic recoiling effects (de Gennes, 1987). Of course, there are other forces needed to be taken into consideration, like depletion forces and magnetic forces controlled by an extra electromagnetic field (Petry et al., 2019; Espinosa et al., 2020). The study showed that fullerenes can be water-soluble and have a high stability achieved by host-guest interactions with cyclodextrins (Ikeda, 2013). It should be noted that taking into account the energy-distance curves, it would be possible to predict the behaviour of colloids. One example, aqueous solutions containing simple electrolytes, is shown in Figure 5D.
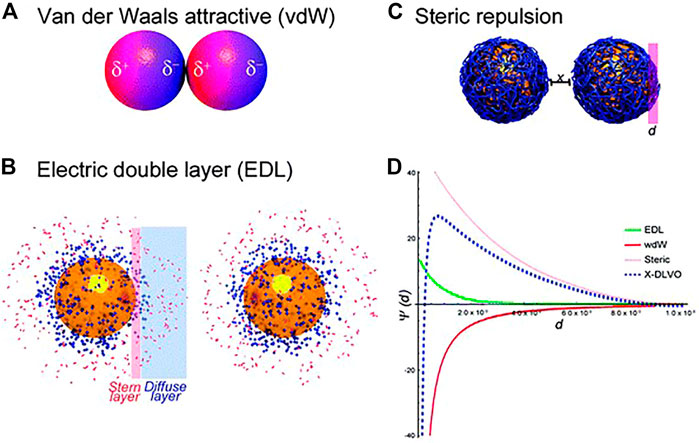
FIGURE 5. Schematics of colloidal interactions (A–C) and illustration of potential energy-distance curves describing colloidal interactions (D) (Moore et al., 2015).
Despite significant breakthroughs in biomedical applications of nano- and micro-particles, the gap between research and their translation into clinics is still significant (Venditto and Szoka Jr, 2013). One of the primary challenges is the complexity of the system, while one should not exclude the lack of understanding of all mechanisms governing particle interactions and effects on biological objects, especially when they are immersed in a complex cellular environment (Moore et al., 2015). In part, this is because the actual system and environment including cell surface, extracellular matrix, and cell culture medium are quite complex compared to commonly used cell culture media like DMEM, MEM, and PBS solution. Most of the particles are taken up via active endocytosis, during which they are exposed to different conditions, like pH changes from 7.4 (in the extracellular medium) to 5.5 (in late acidified endosomes) to 4.5 (in endolysosomes). Moreover, endolysosomes are rich in hydrolytic enzymes that can affect/dissolve the entire NPs or their coatings. Internalized AgNPs were reported to degrade quickly into Ag+ when immersed in endolysosomes (Foldbjerg et al., 2015). Besides, behaviour of particles is sensitive to the ionic strength of the solution. For example, calcium carbonate (CaCO3) can recrystalize in hydroxyapatite (Ca10(PO4)6(OH)2) in cell medium, which contains the PO43− groups or aggregate in the presence of sodium/chloride ions (Na+/Cl−) (Parakhonskiy et al., 2012b) as well as crystalize and dissolve at relatively acidic pH values inside the cell (Abalymov et al., 2018).
Affected by the dynamic complex nature of the cellular environment, the loss of colloidal stability of particles would likely lead to colloidal aggregation into larger and irregularly shaped clusters and even dissolve entirely or stimulate recrystallisation or structural changes. The poor stability of colloids would lead to misrepresentative results or low reproducibility and, at the same time, influence their biodistribution, pharmacokinetics and systemic toxicity (Moore et al., 2015). Another problem is the corona effect which requires additional stabilization. This is because when nanoparticles are injected into the intravenous stream, the blood’s proteins would adsorb on the surface of the particles, thus forming a protein corona layer (Lee, 2021). Protein corona may lead to undesirable effects, for example, facilitating or inhibiting cellular uptake and mitigating or stimulating the immune response (Del Pino et al., 2014; Mosquera et al., 2018; Liu et al., 2020a). To guarantee that nanoparticle functions remain as stable as possible, organic molecules like PEG (Poly (ethylene glycol)), PAA (Poly (acrylic acid)) (Tambunlertchai et al., 2017; Xiao et al., 2020), nanocelluloses (Kaushik and Moores, 2016) are commonly reported to be added as stabilizing agents during the formation process.
2.1.2 Approaches to stabilize inorganic colloids
One of the most widely and effectively accepted strategies to enhance colloidal stability is to avoid particle aggregation via surface modification (Albanese and Chan, 2011), involving for example steric and electrostatic stabilization (Bihari et al., 2008; Liu et al., 2021). Table 2 juxtaposes these three methods used to stabilize the inorganic particles illustrated with respective organic molecules.
2.1.2.1 Electrostatic interaction
When NPs are exposed to a cell medium, colloidal interactions take place at the surface of particles (in the surrounding medium). The overall ionic strength of the new-mixture system determines the EDL fate. Typically, low ionic strength enlarges ion clouds extending further from the particle surface, which diminishes or resists particle-particle interaction. In contrast, an environment with a high ionic strength will suppress the EDL and enforce the attractive vdW leading to NP aggregation (Edwards and Williams, 2004). Electrostatic stabilization is generally reported to be poor in cell culture media (Moore et al., 2015).
2.1.2.2 Steric stabilization
Steric stabilization is the universal method to reinforce colloidal stability. This is often achieved via natural macromolecules (e.g., chitosan, alginate) or synthetic polymer coatings, e.g. PVA (polyvinyl alcohol), PEG (polyethene glycol) (Zhang et al., 2009). Based on numerous studies, there are two ways to make the system more stable: a) coat the NPs with an uniform monolayer of macromolecules, which could provide extra robust steric stabilization, and b) prevent the proteins from attaching to NPs surfaces, which paves the way for bridging effects and leads to aggregation. This is because proteins contained in the cell culture media could cover the surface of particles forcing proteins to interact with more than one NP and facilitate aggregation (Biggs et al., 2000). It has been also shown that the effects of PEG molecules on AuNPs’ colloidal stabilization depend on their molecular weight and concentration (Zhang et al., 2012b).
2.1.2.3 Electrostatic stabilization
One approach to improve colloidal stability is electrostatic stabilization which combines the effects of electrostatic and steric stabilization. In this case, macromolecules strongly coat the NP surface as a physical barrier and therefore impede proteins attaching to NPs’ surfaces. Meanwhile, these charged macromolecules would provide repulsive static electricity given that the environment of the cell culture medium is usually weak alkaline and negatively charged; the charge on NPs can be negative through functionalization by, for example, poly (acrylic acid) (PAA) (Chanteau et al., 2009) or it can be positive (for example, DMAP).
It should be noted that the interactions between the colloidal particles and the capping agents are sophisticatedly complex and depending on media, NPs, functional ligands, iron strength, etc. To interpret and mimic the relation of the colloidal and biological media precisely in vitro, there is still significant needs and that largely depends on interdisciplinary efforts to be made in materials science, cell biology, pharmaceutics, biophysics, etc.
2.2 Controlled loading and release
One of the most feasible ways to enhance the drug efficacy is to load a suitable amount of drug and release it at the lesions. The hybrid nanocomplex is a powerful tool to modify drug loading and optimize release behaviour (Li et al., 2017). The porous inorganic particles are frequently used as drug carriers. Functionalizing them with additional polymer layers improves the stability and contributes to controlled release mechanisms. For example, the functionalisation of porous CaCO3 particles with polymer layers would stabilize the containers on hour-, week-, even months scales, leading to a prolonged drug release. APTES-modified halloysites have a higher loading capacity and were shown to prolong the release of ibuprofen (Tan et al., 2016). The drug delivery system release behavior based on metal-organic framework (MOF) like MIL-101(Fe)-NH2 could be modified by long-chain polyamines (Almáši et al., 2018). Zhou etc., designed a pH-triggered self-unpacking capsules consisting of a MOF and zwitterionic hydrogel coating (Zhou et al., 2021). The MOF core with a large pore size and surface guarantees high loading efficiency. The outer layer of hydrogel is made of pH-sensitive poly-Eudragit L100-55, which would dissolve at a pH > 5.5 and therefore protect the oral peptide drug from degradation when passes through an acidic gastrointestinal environment and is released in the intestinal fluid. Multi-walled carbon nanotubes functionized by hydrophilic organics might be explored as an efficient nanotransporters for oligodeoxynucleotide (Kaufmann et al., 2017). The nanohybrid graphene oxide (GO)-PEG/paclitaxel (PTX) shows relatively high loading capacity for PTX (11.2 wt%) and existed significantly higher cytotoxicity to human lung cancer A549 and human breast cancer MCF-7 cells compared to free PTX (Xu et al., 2014). Loading and release may differ for various drug deivery carriers, and in this regard polyelectrolyte multlayer nano- and micro-capsules are attractive carriers (Li et al., 2022b), which are composed of polyelectrolytes (organic molecules), but their functionalization with such inorganic particles as gold (Skirtach et al., 2005) or silver (Skirtach et al., 2004; Radziuk et al., 2007) allows to perform release.
2.3 Reduction of cytotoxicity and improvement of biocompatibility
There have been reports on drugs providing undesirable toxic effects (Hirt and Body-Malapel, 2020). Due to the EPR effect, the clearance of NPs rates from circulation streams and tissues is much lower, and it would result in the accumulation of NPs at the surface of the tissue or within cells and pose the risk of disruption of organelle integrity or gene alterations (Gitipour et al., 2016). The toxicity of NPs is highly correlated with their complexity and diversity in terms of size, shape, charge, production methods, chemical composition, surface functionalization, and aggregation (Lewinski et al., 2008; Lv et al., 2021; Nitti et al., 2022). In order to improve inorganic NPs safety, organic protective layers or coating agents are usually used to cover their surface, which would help to prolong the circulating half-life and minimize the reticuloendothelial system uptake (Nie, 2010; Rabie et al., 2019). Recent study found that when AuNPs are coated with ferritin, their biocompatibility is dramatically enhanced as the cellular uptake of transferrin-receptor-rich cell lines increased more than seven times and showed very low toxicity on different human cell lines (Moglia et al., 2021). It was reported that biocompatibility of graphene oxide could be enhanced by PEG, PAM, and PAA (Xu et al., 2014; Xu et al., 2016).
2.4 Targeted delivery
The lack of efficient delivery systems has impeded the efficiency of drugs, especially for small molecule drugs for chemotherapy which are always accompanied by noticeable side effects (Mu et al., 2020). In order to take the best advantages of drugs, various materials such as lipids, polymers (Go and Leal, 2021), hydrogels (Lengert et al., 2017), metal-organic framework (MOF) (Zhou et al., 2021; Kiadeh et al., 2021) and NPs (Jun et al., 2008; Xie et al., 2012; Biju, 2014; Tarakanchikova et al., 2020) have been exploited. Also recently, SiO2 (Gupta et al., 2018), Fe3O4 (Liu et al., 2020b), CaCO3 (Huang et al., 2022), and AuNPs (Sharifi et al., 2019) have been investigated extensively as emerging delivery carriers. Especially, AuNPs have many advantages, such as simple synthesis, easily tunable size, chemically inert, oxidation-free, facile surface modification, and versatile conjugation with biomolecules (Kang et al., 2019). Organic molecules can be used for functionalization of AuNP targeting specific drug delivery routes with the LbL method. For example, Lee etc., designed the nanocomplex-AuNPs/siRNA/polyethyleneimine (PEI)/hyaluronic acid (Ha) modified by cysteamine, which could target specific intracellular delivery of siRNA via Ha receptor-mediated endocytosis (Lee et al., 2011). CaCO3 has proved to be useful for intracellular delivery through its crystal phase transition (Parakhonskiy et al., 2013). Recently, sub-micro vaterite CaCO3 particles loaded with photosensitizer drug porphyrazine (pz) was found to mainly accumulate in the tumor blood vessels, and the tumor uptake of pz was enhanced by 1.8 times; while loaded gold nanorods improved delivery by 3.4 times, showing extensive potential to be explored as tumor therapeutic agent for photodynamic therapy (Parakhonskiy et al., 2021).
2.5 Detection
Plasmonic nanoparticles like AuNPs and AgNPs, exhibit distinct colours throughout the visible and near-infrared regions due to their localized surface plasmon resonance (LSPR) absorption and scattering (Mulvaney et al., 1992; Qin et al., 2015; Kang et al., 2019; Cui et al., 2020), and have been used in applications in sensing, energy, catalysis, and biomedicine (Lin et al., 2016; Vita et al., 2018; Dai et al., 2021; Song et al., 2021). LSPR provides a higher detection accuracy than traditionally used surface plasmon resonance (SPR). SPR emanates from coherent oscillations of conduction electrons caused by electromagnetic radiation excitation at the surface, because the size of NPs is comparable to the mean free path of electrons in these metals, which restricts the plasmon to NPs. Besides the LSPR, plasmonic nanoparticles (for example, AuNPs and AgNPs) can be also used for surface-enhanced Raman scattering (SERS) sensing, which could dramatically improve the detection sensitivity by 102 to 1014 times (Yashchenok et al., 2012; Lengert et al., 2018). When plasmonic nanoparticles are combined with specific aptamer, antigen-antibody recognition, enzyme etc., they could be modified as a specificity detection platform (Figure 6).
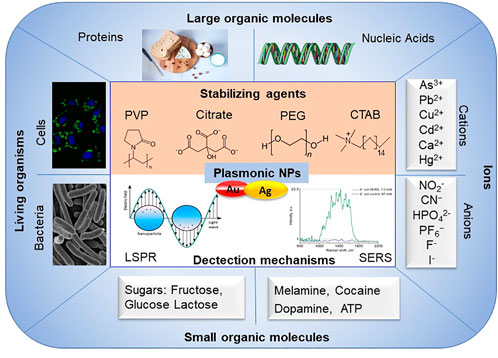
FIGURE 6. A schematic overview of mechanisms for plasmonic NPs-based biosensors for detection and their applications.
Magnetic NPs possess special effectsf, for example, Yang reported a combinination of polydopamine (PDA), GE11 peptide and ultra-small superparamagnetic iron oxide NPs, GE11-PDA-Pt@USPIOs which have a high specificity for EGFR-positive tumor cells; that would be used in radio-chemo combination therapy, in which such NPs enhanced magnetic resonance imaging/photoacoustic imaging (MRI/PAI) (Yang et al., 2019). Zhu et al. (2021) explored a selective and sensitive detection platform for Staphylococcus aureus by coating the AuNPs with polydimethylsiloxane (PDMS) film. But, it should be noted that the plasmonic NPs would lose plasmonic functionality once NPs dissolve. So one of the most critical aspects in designing and exploring the detection tools based on the plasmonic nanoparticles is to find suitable stabilizers. Commonly used stabilizing agents and size controllers include the following molecules: cetyltrimethylammonium bromide (CTAB), citrate, PVP, PEG (Kang et al., 2019). Surface plasmon resonance imaging (SPRi) was used for label-free detection based on plasmonic NPs (Guner et al., 2017). The detection platforms based on gold and silver NPs can be used to analyze many types of samples, including small organic molecules (Liu et al., 2011), nucleic acids (Thaxton et al., 2006), proteins (Anfossi et al., 2019), sugars (Brasiunas et al., 2021), cells (Zhang et al., 2010), bacteria (Li et al., 2022a), cations and anions (Liu et al., 2011; Dutta et al., 2019), etc.
Besides plasmonic nanoparticles, carbon quantum dots (CQDs) represent an emerging fluorescent nanomaterial which was used in various applications, especially in chemosensing and biosensing (Ding et al., 2014; Nekoueian et al., 2019). When they are functionalized by suitable organic molecules, they can be used as a versatile detection platform that includes small bioactive molecules like Vitamin B2, amino acids, big molecules (DNA, RNA), enzymes, or cells. When CQDs are functionalized by folic acid, a biomarker for cancer, hybrid nanoprobes could target cancer cells due to their high affinity to folate receptors (Feng and Qian, 2018).
2.6 Other applications outside of biomedicine
Hybrid nanocomposites based on inorganic NPs with organic molecule modifiers have various functions covering a wide range of applications, particularly in the following sectors and applications: health sector (Byrappa et al., 2008; Kopecek, 2009; Chanana et al., 2013; Ni et al., 2020; Lavrador et al., 2021), wound healing applications (Chen et al., 2022), long-term tracking stem cell transplantation therapy in vivo (Xie et al., 2022), electrochemical immunosensing (Campuzano et al., 2017), optics (Shavel et al., 2004; Melnikau et al., 2016; Melnikau et al., 2018), micro-electronics (Banerjee et al., 2009), transportation, packaging (Moustafa et al., 2021), energy (Li et al., 2016; Mahmood et al., 2016; Liu et al., 2019), housing (Saba et al., 2016), catalysis (Shahmoradi et al., 2011; Liu et al., 2014; Tomai et al., 2021) and the environment (Sanchez et al., 2011). Cyclodextrins (CDxs) were reported as solubilizing agents, which could improve C60 water solubility when coated by CDxs (Ikeda, 2013).
TiO2 based bio-catalytic nanoparticles modified by 2, 2′-Azino-bis-(3-ethyl benzothiazoline-6-sulfonicacid) (APTES), glutaraldehyde (GLU) and laccase possess a high degradation rate for such micropollutants as bisphenol-A (Hou et al., 2014) A wide-range and fast response solid-state potentiometric pH sensors based on PDA coated carbon nano-onion (CNO) electrodes were reported (Zuaznabar-Gardona and Fragoso, 2018). PDA films endow this system with a fast response toward pH changes, while CNO is responsible for transferring the signal quickly due to its large surface area and electrochemical properties. This pH sensor could work in the range from 2.2 to 8.3. Ji et al. (2020a) found that silk fibroin (SF)/Bi2O2CO3-Cu2O nanofibers produced by electrospinning can be easily recycled and have excellent photocatalytic and antibacterial activities, and low-cytotoxicity, which paves the way to promoting their practical applications in, for example, water treatment. This is because SF has a large surface to immobilize powder Bi2O2CO3-Cu2O photocatalysts, and the hybrid mat-like structure separates itself from water efficiently. The nanocomposite coating – polyurethane (PU)/SiO2-Al2O3 - showed significant resistance against corrosion compared to that of pure steel (Xavier, 2021).
3 Inorganic surfaces functionalized by organic molecules
Typically in the field of biomedicine, organic coatings provide the same functionalities as those described above for colloids, except for such applications as targeted delivery, which involves the movement of an inorganic object by itself. In Figure 7, selected inorganic surface functionalization modalities via organics are shown. Table 3 summarizes modifications of representative inorganic surfaces by organics highlighting their applications and gained functionalities. In the following part, the role of organic molecules is illustrated mainly for metallic and inorganic (non-metallic) materials.
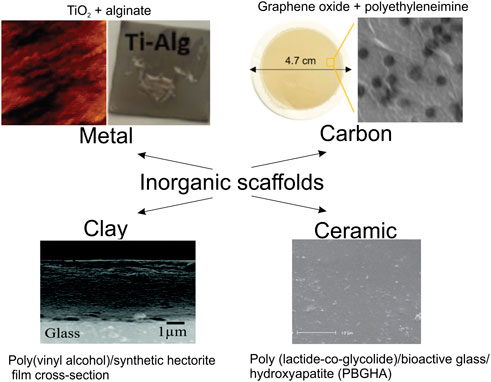
FIGURE 7. Functionalization of inorganic surfaces by organic molecules. Metal (AFM images of TiO2 coated by alginate hydrogel coatings reproduced from (Muderrisoglu et al., 2018) with permission Wiley-VCH), Carbon (optical and SEM images of the graphene oxide modified by polyethyleneimine film; reproduced from (Nam et al., 2016) with permission of ACS), Clay (SEM images of the Poly(vinyl alcohol)/synthetic hectorite film cross-section with a thickness of 4.3 μm (Teepakakorn and Ogawa, 2021) with permission of the Mater. Adv.), Ceramic (SEM images of the poly (lactide-co-glycolide)/bioactive glass/hydroxyapatite (PBGHA) (Mehdikhani-Nahrkhalaji et al., 2015) reproduced from Dent. Res. J. (Isfahan)).
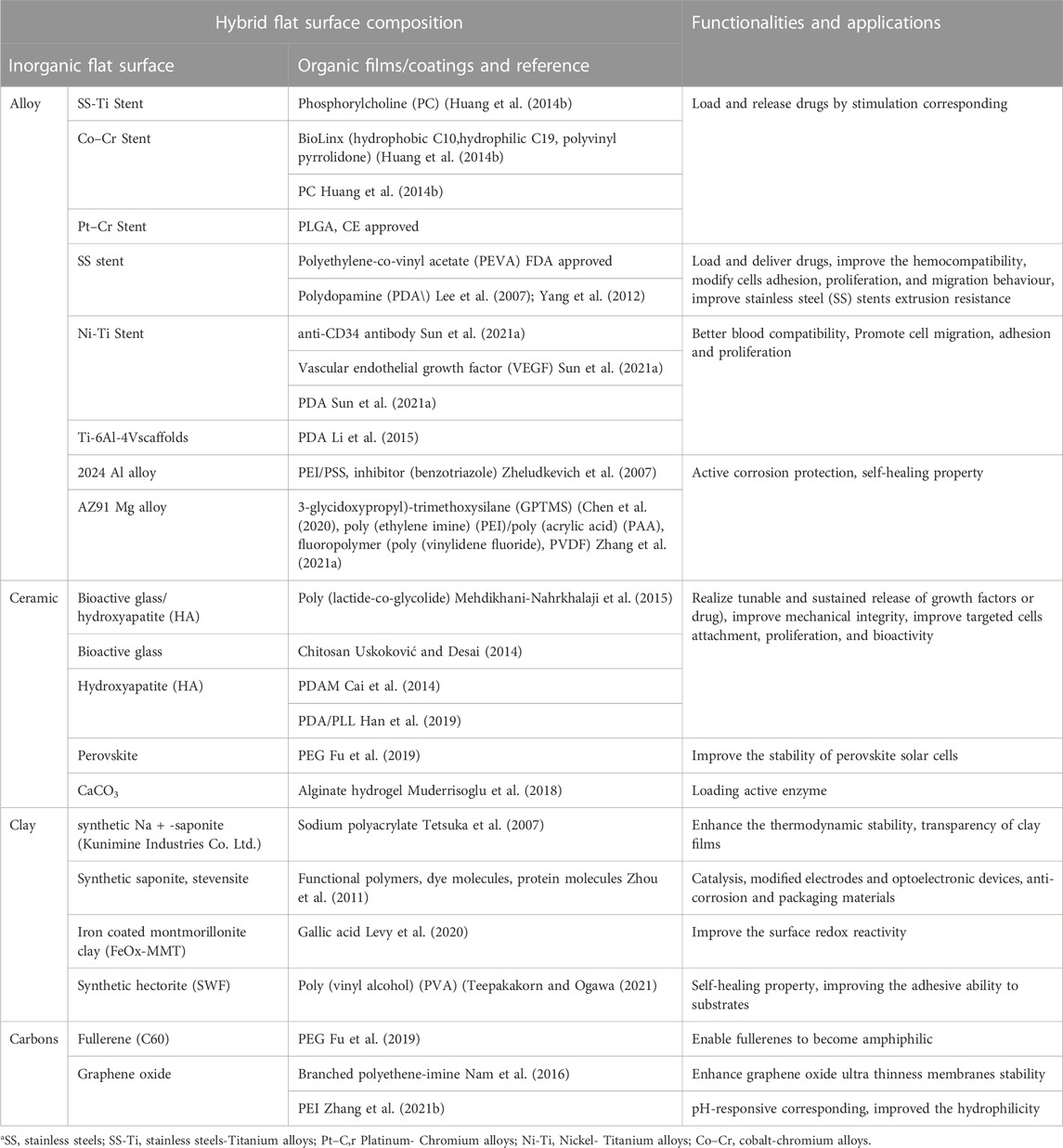
TABLE 3. Overview of hybrid organic films/coatings on inorganic substrates/flat surfaces, their composition and functionalities.
Functionalization of soft hydrogel-like films composed of poly-L-lysine and hyaluronic acid by inorganic nanoparticles has been shown to extend their application range (Skirtach et al., 2010; Volodkin et al., 2012), while their functionalization by capsules with nanoparticles allows to perform remote release (Volodkin et al., 2009).
3.1 Challenges in regard with clinical applications of biomedical materials
Metallic biomaterials are essential for development and implementation of clinical implants to reconstruct failed tissue, especially failed hard tissue (Basova et al., 2021). Around 70%–80% of implants are made of metallic biomaterials; among which the most representative clinically used metallic biomaterials are stainless steel (SS), cobalt-chromium (Co–Cr) and titanium (Ti) alloys (Niinomi et al., 2012). Even though metallic materials have been widely used, there are still many obstacles that impede broad clincal applications of implants once and for all time, especially for long-life implantations. The common problems are mainly identified as follows (Abdel-Hady Gepreel and Niinomi, 2013):
1) mismatch of mechanical properties (Figure 8): this impedes cell attachement to the surface of the implant and leads to fibrous encapsulation at the site. Potential solution: Modification of mechanic properties and facilitation of cell attachment by organic molecules or coatings;
2) corrosion: show low fatigue strength and are vulnerable to corrosion, leading to metal ions or monomer release into the body and may damage the surrounding cell tissue. Potential solution: Application of anti-corrosion coatings;
3) lack of cell biocompatibility: allogeneic transplantation may trigger an immune response with serious side effects such as inflammation. Potential solution: Loading and covering with biocompatible polymers and growth factors.
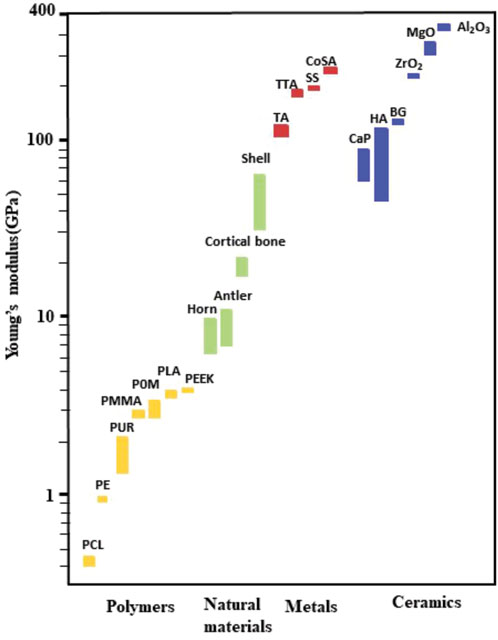
FIGURE 8. Mechanical properties (the Young’s modulus) of natural bone materials in comparison with those for other materials often used in biomedicine; data are based on (Orlovskii et al., 2002) and (Butscher et al., 2011).
Polymers: (PCL: poly (caprolactone), PE: polyethene, PUR: polyurethane, PMMA: poly (methyl methacrylate), POM: Polyoxymethylene, PLA: Polylactic acid, PEEK: polyetheretherketone), Metals: (TA: Titanium-based alloy, TTA: Tantalum based alloy, SS: stainless steels, CoSA: Cobalt-based super alloy), Ceramics (CaP: Calcium phosphate bio-ceramic, HA: hydroxyapatite based bio-ceramic, BG: Bioglass based bio-ceramic, ZrO2: Zirconia based bio-ceramic, MgO: Magnesia based bio-ceramic, Al2O3:Alumina based bio-ceramic).
3.2 Optimization of mechanical biocompatibility
There are two strategies to optimize the material’s mechanical biocompatibility. One strategy is towards modifying its element and structural composition from the inside. With addition of a small amount of graphene to chitosan (0.1–0.3 wt%), the hybrid graphene/chitosan films’ elastic modulus was increased by ∼ 2 times compared to that for pure chitosan (Fan et al., 2010). Taking bone repair engineering as a representative example, bone is a natural hybrid material consisting of two major parts: soft inner cancellous and hard cortical shell (Wubneh et al., 2018). Around 70% of it is composed of inorganic hydroxyapatite (HA, Ca10(PO4)6(OH)2), which is a member of the calcium phosphate (CaP) mineral family (Pal, 2014) and the rest (20%–30%) is extracellular organic matrix—a mixture of water, collagenous and non-collagenous proteins. In contrast, only around 2% is made of bone-resident cells (Belinha, 2014). Inspired by the sophisticated composite structure of bone tissue and clinical trials, the strategies of developing hybrid composites used to boost bone repair have become a hot topic.
The other one resorts to the surface modification and function by films or coatings to facilitate the attachment of cells to the surface of materials and to stimulate cell proliferation and differentiation (Miyazawa et al., 2008; Glazebrook et al., 2013; Ho-Shui-Ling et al., 2018). Yang et al. (2021b) found that the Ti-based implants could obtain dual function: antibacterial properties and facilitation of osteointegration in vivo when hyperbranched poly-L-lysine is grafted on its surface. In the area of stents, Sethi and Lee reported a successful strategy to design the combo stent where the stainless steel surface was coated by anti-human CD34 antibodies intermediated by a polysaccharide (Sethi and LEE, 2012). This combo stent showed excellent endothelial progenitor cell adhesion capability from the circulating system with the antibody coatings (Figure 9). In bone repair engineering, composites like collagen/HA, β-TCP/collagen, HA/Starch, HA/gelatin, and PCL/HA are frequently reported for surface modification (Butscher et al., 2013; Ruiz-Aguilar et al., 2017; Ho-Shui-Ling et al., 2018; Prabakaran et al., 2021). It was described that hydrogel coatings loaded with active enzymes coated on top of a titanium plate could significantly stimulate osteoblast colonization and growth (Muderrisoglu et al., 2018). It was recently shown that introduction of poly-hydrobutyrate (PHB) enables piezoresponse of CaCO3 mineralized 3D scaffolds, which can be used for local delivery of bioactive molecules and for enhancement of tissue repair (Chernozem et al., 2022).
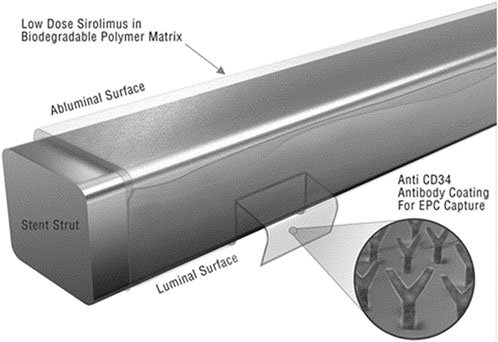
FIGURE 9. Surface functionalization of a stent by antibody coatings (Sethi and LEE, 2012) with permission of the Wiley Online Library.
Table 4 provides summary of hybrid bone scaffolds, their components, and working mechanisms.
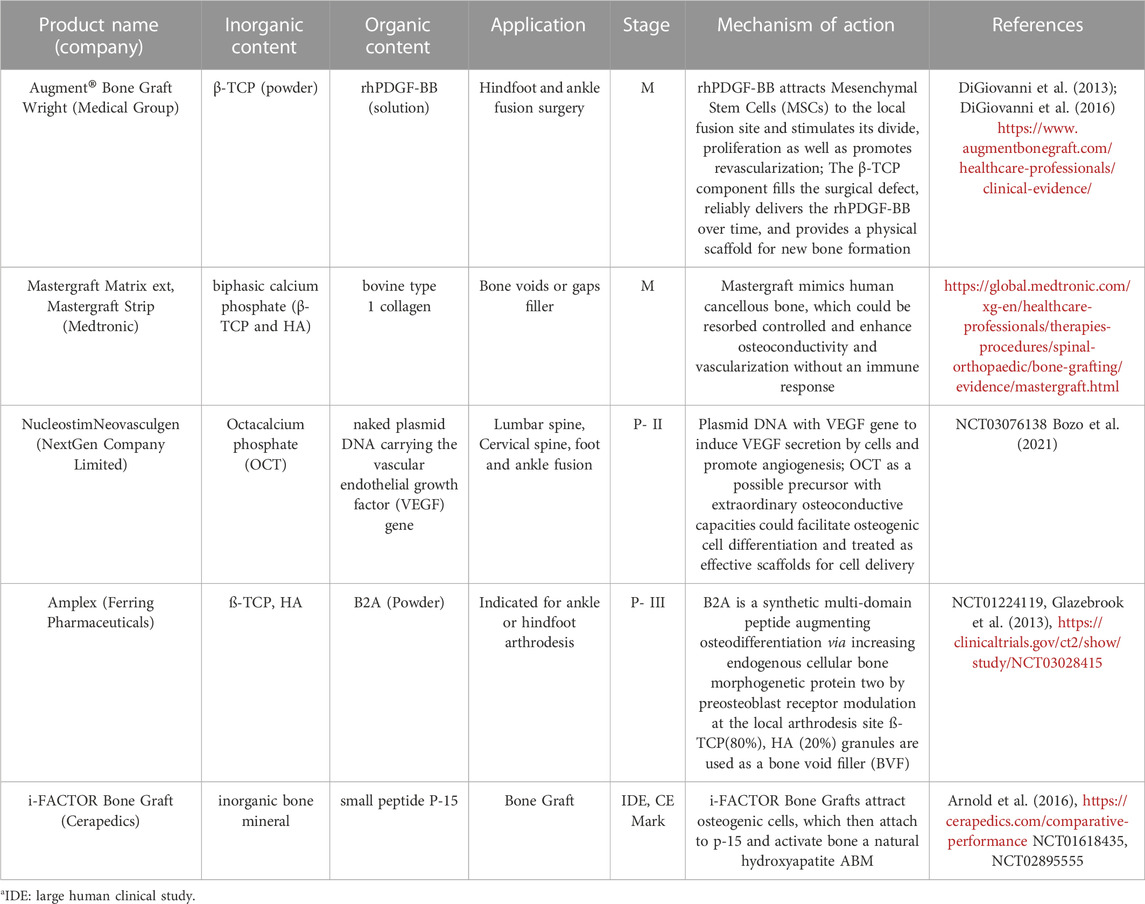
TABLE 4. List of commercial products with organic bioactive molecules (growth factors, peptides or small molecules) and inorganic or hybrid material carrier and their stage of development in the bone scaffolds.
3.3 Anticorrosion protection
One of the most successful applications of coatings is anticorrosion protection. Recently, various groups developed advanced smart coatings which were made not only from the polymer layer protecting it from oxygen and aggressive ions, but also from intelligent delivery systems containing carriers with corrosion inhibitors. For example, the degradation of the organic part allows to release inhibitor and provides a self-healing effect to the damaged place (Zheludkevich et al., 2007; Deng et al., 2021).
The most remarkable breakthroughs occured frequently in fully-erodible drug-eluting stents (EDES). Among the top three studied biodegradable metals (magnesium, iron, zinc), magnesium and its alloys are advancing to commercial products (Huang et al., 2014b; Zheng et al., 2014). This is because mechanical properties of magnesium are closer to the natural bone, which can dramatically minimize the stress-shielding effect–the biggest issue with non-degradable implant materials such as stainless steel and titanium alloys (Abdel-Hady Gepreel and Niinomi, 2013). However, magnesium alloys usually corrode too quickly in the human body and play a supporting role. Hybrid coatings improved their degradation resistance significantly, as Figure 10 shows (Rahman et al., 2021). Al Zoubi et al. (2020) also presented a successful strategy to enhance its corrosion resistance via organic coatings made up of 2-mercaptobenzimidazole (MBI). In 2016, Magmaris, a biodegradable and resorbable stent (BRS), was produced by biotronic, a German medical device company that obtained certificate approval in Europe. This remarked the first metallic BRS that became available on the market (Rapetto and Leoncini, 2017). It consists of a Mg alloy backbone and biodegradable poly-l-lactic acid (PLLA) coating, which could load sirolimus. Within the last 2 years and following-up clinical data published in May of 2021, it was reported for BIOSOLVE-IV that target lesion failure (TLF) of Magmaris is 6.6% (71/1,075 patients) and without scaffold thrombosis after 12 months of implantation, confirming its long-term safety.
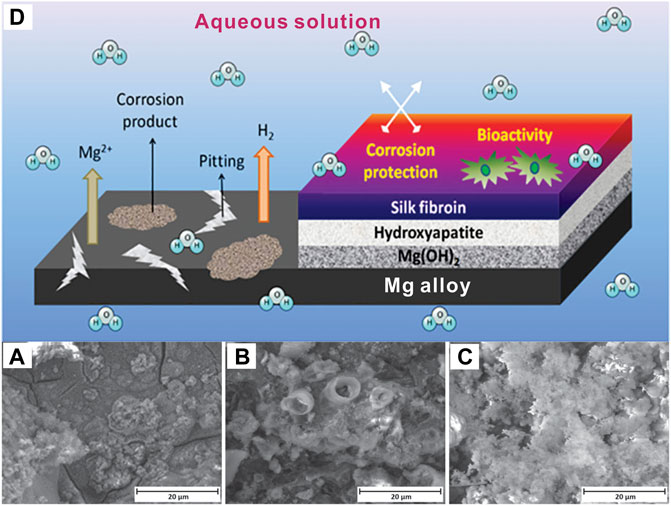
FIGURE 10. Comparison of the degradation of (A) pure Mg, (B) Hydroxyapatite on Mg, (C) Silk fibroin + hydroxyapatite on Mg, after immersion in Hank’s solution for 7 days, (D) Schematic illustration of the protection coating on Ma alloy (Rahman et al., 2021), with the permission of the ACS.
3.4 Immune response and inflammation treatments
Organic coatings or films are explored extensively as drug and growth factor carriers to optimize implant performance and reduce or prevent the high potential risks and side effects like those mentioned above. Some representative applications are drug-eluting stents (DES), while another is bioresorable scaffolds (BRS). They comprise metal body and polymer coatings, often used in cardiovascular stents. Polymer coatings are responsible for loading and delivering drugs, such as sirolimus and paclitaxel (Garg and Serruys, 2010; Papafaklis et al., 2012; Iqbal et al., 2013). Taking three kinds of evaluated clinical Zotarolimus-eluting (ZEs) stents as a reference, a comparison between ZESs is summarized in Table 5. It can be noticed that the drug release profiles could be modified by altering the composite of metallic platforms or polymeric coatings.
In bone repair engineering, Yang reported a successful strategy to make bipolar metal flexible fibrous membranes based on MOFs(ZIF-11 and HKUST-1), acting as carriers and achieving sustainable release of bone regeneration factors such as Cu2+, Zn2+ (Yang et al., 2022). This flexible fibrous membrane has been verified in regard with multiple tissue synchronous regeneration at the damaged tendon-to-bone interface, like tendon and bone tissue repair as well as fibrocartilage reconstruction (Figure 11).
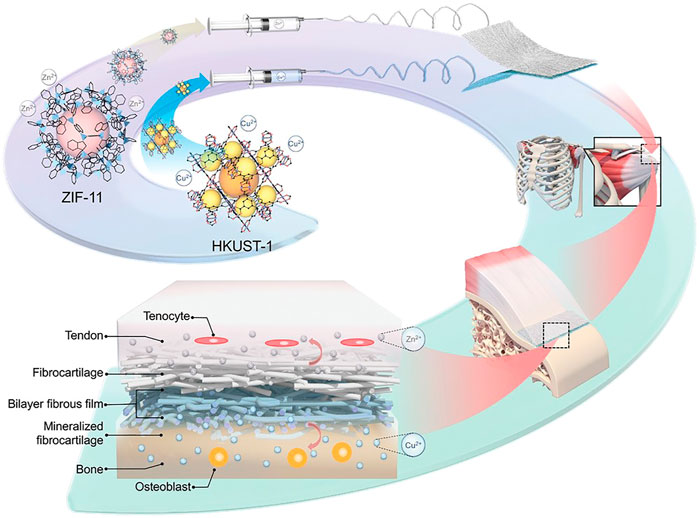
FIGURE 11. Schematic illustration of hybrid nanofibrous membrane fabrication and the effect of regulating the synchronous regeneration of the bone-tendon interface by metal ions released from the nanofiber in situ (Yang et al., 2022) with the permission of Wiley Online Library.
Some antagonistic but complementary properties of organic and inorganic materials are summarized in Figure 12, which is useful for designing or optimizing hybrid materials with appropriate properties.
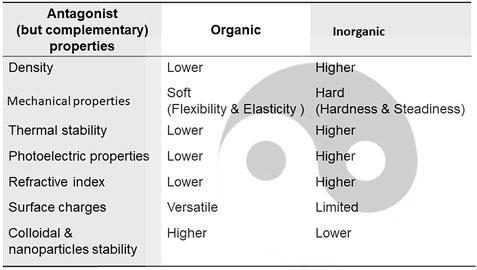
FIGURE 12. Antagonist (yin-and-yang), but complementary, properties of most common inorganic and organic compounds motivating their incorporation into hybrid materials.
3.5 Other applications outside of biomedicine
Using organic films or coatings on the flat surface of the inorganics has accelerated the advancement in many areas, also outside of biomedicine. It includes protection and exploration of such materials as steel and copper, electrocatalysts (Yu et al., 2019), batteries (Li et al., 2018b; Wang et al., 2022) to advanced materials like sensors (Nugroho et al., 2018), wearable and flexible devices (Shim et al., 2008), marine ships, and aerospace vehicles (Mann, 2009; Huang et al., 2014a; Xu et al., 2021). Lee et al. (2007) reported polymer coatings synthesized from dopamine, which could coat virtually all types of material surfaces. Therefore, it could be used as a versatile platform for secondary reactions and to explore the coatings according to requirements necessitated by applications. A highly transparent flexible clay film has been obtained by organic polymer modification, which would be promising for display and other electronic devices (Tetsuka et al., 2007). A safer solid lithium battery was achieved by composite materials with polymer electrolyte (Wan et al., 2019). Teepakakorn and Ogawa reported that the poly (vinyl alcohol)-clay hybrid film has a high adhesive capability to substrates like glass and displayed self-healing soaking in water (Teepakakorn and Ogawa, 2021). Huang et al. (2019) summarized properties of organic and hybrid hydrophobic/superhydrophobic icephobic coatings for aerospace applications. PDMS-Ag@SiO2 core-shell nanocomposite antifouling coating was found to exhibit significant inhibitory effects on different bacterial strains, yeasts and fungi (Selim et al., 2018). Gu et al. (2020) reported the strategies to develop environmentally friendly marine antifouling coatings to protect ships from corrosion. The coating contains silicone polymer that prevents marine biological fouling on the ship’s surface by making it difficult for fouling organisms to adhere but facilitate removal after attachment.
Further, carbons can be functionalized with polymers. It was reported that introduction of branched polyetheneimine (bPEI) could enhance graphene oxide membranes’ stability and improve filtration efficiency (Nam et al., 2016). PEG provides fullerenes with amphiphilic properties, which enhance perovskite film quality and the perovskite solar cells’ stability (Fu et al., 2019). The utilization of PEI could make the pH-responsive graphene oxide a candidate for high-performance nanofiltration (Zhang et al., 2021b). Recently, hybrid conductive organic polymers like polyaniline, poly (dioxy-3,4-ethylenethiophene) and polypyrrole were used with traditional additive manufacturing materials like metals, paving the transition from 3D to 4D printing. Compared with traditional 3D, 4D printed material structures could response to external stimuli (voltage, force, heat), which is the core properties of soft-robotics or flexible wearable electronics (Martinelli et al., 2022).
4 Conclusion
The recent decades have seen a dramatic rise and extensive developments in the areas of hybrid materials and their advanced applications. Hybrid materials composed of organic and inorganic constituents can be logically divided into two parts:
1) organic materials modified with inorganic constituents (inorganics-in-organics),
2) inorganic materials modified or functionalized with organic molecules (organics-on-inorganics).
In this review, we have described and analyzed organic molecule-modified inorganic material (organics-on-inorganics) compositions and their selected applications in biomedicine and other areas. According to the size and shape of the modified inorganics, organic molecule-modified inorganic materials (organics-on-inorganics), can be sub-divided into:
a) inorganic colloids and nanoparticles functionalized by organic molecules;
b) inorganic flat surfaces/matrices functionalized by organic molecules.
Incorporation of organic constituents is selected with a specific goal—to bring or complement properties not provided by inorganics, relevant for improving their stability and biocompatibility, reducing their cytotoxicity, enhancing corrosion resistance, providing organic molecule recognition, binding specificity, detection, loading and controlled delivery, release, etc. And as a matter of fact, in the field of biomedicine, organic molecules provide the same functionalities for inorganic colloidal particle and inorganic flat surfaces, perhaps with exception of applications like targeted delivery. Because targeted delivery of molecules involves movement of an inorganic object by itself. More specifically, in order to clarify the synergy of organics-on-inorganics, we have illustrated the roles of organic-inorganic hybrids in various applications.
Noble metals such as gold are chemically inert, but, in addition, they possess a high photothermal sensitivity and electromagnetic conductivity. The presence of such organic constituents as polymers, antibodies or macromolecules could be used for developing and designing targeted drug delivery vehicles and achieving multi-response drug release as well as real-time in situ detection. For traditional non-degradable metal implants like stainless steel and titanium (Ti) alloys, the surface-coated organic antibacterial and anti-inflammation drugs could dramatically reduce the risk of secondary surgery. For degradable metals like Mg and Zn, introduction of organic polymers could be used for controlling release profile to fulfil its function and implement fully-degradable drug-eluting stents (EDES), which would safely resorb in the body. Combining MOF with organics has pushed development of highly effective multi-drug loading and multistimuli release platforms, which is achieved via hydrogels and amino functional groups. In the area of magnetic nanoparticles like Fe3O4, complementarity of inorganic and organic materials is achieved through dual functionality: magnetic targeting (inorganic NPs) and biocompatibility enhancement (surface functionalization by biopolymers).
Such non-metal composite-like ceramics as CaCO3, Ca3(PO4)2, HA (Ca10(PO4)6(OH)2) have been extensively used in bio-medicine. It is not only because its porous crystalline configuration provides a higher loading efficiency compared to organic carriers like liposomes, but also due to its elemental composition, which provides Ca2+ necessary for remodelling. Introduction of small peptides, nucleic acids, and growth factors would contribute to optimizing bone grafts and circumvent potential immune response problems. This has been tested and used in commercial bone grafts. Clays, like halloysite (Al2Si2O5(OH)4), have also attracted extensive attention due to their atomically thin layered structure and charge characteristics. Their applicability can be enhanced by adding organic molecules. For instance, in drug delivery: combining clay NPs with organic polymers, targeted proteins or polysaccharides would be useful for enhancing biocompatibility, enabling targeting, and controlling release profile. Nano carbons, like carbon quantum dots, fluorescent nanodiamonds, fullerenes, and carbon nano onion are emerging biomedical materials due to their outstanding thermal and electrical conductivity, extraordinary fluorescent brightness and photostability as well as their low density and high strength. The presence of organic molecules such as oligosaccharides, peptides, and biopolymers would pave the way for accurate imaging diagnosis.
5 Outlook
Although to-date developments have been very promising, innovations and breakthrough’s potential in the area of hybrid materials is yet to be fully realized. It is expected that higher levels of sophistication and miniaturization, environmental friendliness and lower production costs are expected to be achieved. Smart hybrid materials and devices will be further designed, synthesized and assembled showing excellent stimulus responses according to environmental changes based on the complementary yin-and-yang properties of organics and inorganics.
Taking as an example micro- and nano-hybrid material applications in biomedicine, the most obvious and key points are to open the “black box” of the interactions among hybrid materials with the organisms in real working situations lies in: setting-up construction strategies to synthesize the hybrids precisely with controlled stability, good biocompatibility, and excellent stimuli-responsiveness to various stimuli These smart hybrid materials would lead explorations toward such multifunctional platforms as soft-robot sensors in vivo, electrocatalysis, photoelectrocatalysis, wearable devices, and so on. Hybrid materials comprising organic coatings on inorganic materials are indispensable to combat modern threats such as antimicrobial resistance and nosocomial infections in general. The hybrid structure can endow the contact surfaces with specific (bio) functionalities keeping their mechanical integrity at a high level and, at the same time, providing controlled presentation of active compounds hosted/protected in organic part on demand.
A critical mass of interdisciplinary knowledge is needed for realization of this vision. To be more specific, the advanced characterization methods, emerging materials, and a collaboration of different research communities would dig out deep into the properties of hybrid materials and facilitate advanced materials applications.
Author contributions
JS contributed to writing the first draft and made subsequent modifications. AV contributed to describing sub-subjects of this work, references, and provided contribution to the outlook. BP provided valuable suggestions for improving the framework of the review and the final draft. AS has organized work and led some of the research directions on which this work is based. All authors have read and agreed to the published version of the manuscript.
Acknowledgments
We thank the Special Research Fund (BOF) of Ghent University (01IO3618), FWO-Vlaanderen (G043322N; I002620N), and EOS of FWO-F.N.R.S. (project # 40007488) for support. JS acknowledges the support of the China Research Council (CSC, No. 202006150025). AV acknowledges financial support from the Staedtler Foundation in the frames of the project “Function by Design: Cellular Hybrids.”
Conflict of interest
The authors declare that the research was conducted in the absence of any commercial or financial relationships that could be construed as a potential conflict of interest.
Publisher’s note
All claims expressed in this article are solely those of the authors and do not necessarily represent those of their affiliated organizations, or those of the publisher, the editors and the reviewers. Any product that may be evaluated in this article, or claim that may be made by its manufacturer, is not guaranteed or endorsed by the publisher.
References
Abalymov, A. A., Verkhovskii, R. A., Novoselova, M. V., Parakhonskiy, B. V., Gorin, D. A., Yashchenok, A. M., et al. (2018). Live-Cell imaging by confocal Raman and fluorescence microscopy recognizes the crystal structure of calcium carbonate particles in HeLa cells. Biotechnol. J. 13, e1800071. doi:10.1002/biot.201800071
Abalymov, A., Van Poelvoorde, L., Atkin, V., Skirtach, A. G., Konrad, M., and Parakhonskiy, B. (2020). Alkaline phosphatase delivery system based on calcium carbonate carriers for acceleration of ossification. ACS Appl. Bio Mater. 3, 2986–2996. doi:10.1021/acsabm.0c00053
Abanades Lazaro, I., Wells, C. J. R., and Forgan, R. S. (2020). Multivariate modulation of the Zr MOF UiO-66 for defect-controlled combination anticancer drug delivery. Angewandte Chemie Int. ed. Engl. 59, 5249–5255. doi:10.1002/ange.201915848
Abdel-Hady Gepreel, M., and Niinomi, M. (2013). Biocompatibility of Ti-alloys for long-term implantation. J. Mech. Behav. Biomed. Mater. 20, 407–415. doi:10.1016/j.jmbbm.2012.11.014
Abdolmaleki, A., Mallakpour, S., and Borandeh, S. (2014). Tailored functionalization of ZnO nanoparticle via reactive cyclodextrin and its bionanocomposite synthesis. Carbohydr. Polym. 103, 32–37. doi:10.1016/j.carbpol.2013.12.013
Aijaz, A., Karkamkar, A., Choi, Y. J., Tsumori, N., Rönnebro, E., Autrey, T., et al. (2012). Immobilizing highly catalytically active Pt nanoparticles inside the pores of metal–organic framework: A double solvents approach. J. Am. Chem. Soc. 134, 13926–13929. doi:10.1021/ja3043905
Al Zoubi, W., Yoon, D. K., Kim, Y. G., and Ko, Y. G. (2020). Fabrication of organic-inorganic hybrid materials on metal surface for optimizing electrochemical performance. J. Colloid Interface Sci. 573, 31–44. doi:10.1016/j.jcis.2020.03.117
Albanese, A., and Chan, W. C. (2011). Effect of gold nanoparticle aggregation on cell uptake and toxicity. ACS Nano 5, 5478–5489. doi:10.1021/nn2007496
Almáši, M., Zeleňák, V., Palotai, P., Beňová, E., and Zeleňáková, A. (2018). Metal-organic framework MIL-101(Fe)-NH 2 functionalized with different long-chain polyamines as drug delivery system. Inorg. Chem. Commun. 93, 115–120. doi:10.1016/j.inoche.2018.05.007
Anfossi, L., Di Nardo, F., Russo, A., Cavalera, S., Giovannoli, C., Spano, G., et al. (2019). Silver and gold nanoparticles as multi-chromatic lateral flow assay probes for the detection of food allergens. Anal. Bioanal. Chem. 411, 1905–1913. doi:10.1007/s00216-018-1451-6
Ariga, K., Jia, X., Song, J., Hill, J. P., Leong, D. T., Jia, Y., et al. (2020). Nanoarchitectonics beyond self-assembly: Challenges to create bio-like hierarchic organization. Angew. Chem. Int. Ed. 59, 15424–15446. doi:10.1002/anie.202000802
Arnold, P. M., Sasso, R. C., Janssen, M. E., Fehlings, M. G., Heary, R. F., Vaccaro, A. R., et al. (2016). i-Factor™ bone graft versus autograft in anterior cervical discectomy and fusion: Two-year follow-up of the randomized single-blinded food and drug administration investigational device exemption study. Spine J. 16, S153–S154. doi:10.1016/j.spinee.2016.07.051
Balazs, A. C., Emrick, T., and Russell, T. P. (2006). Nanoparticle polymer composites: Where two small worlds meet. Science 314, 1107–1110. doi:10.1126/science.1130557
Banerjee, S., Das, R. K., and Maitra, U. (2009). Supramolecular gels 'in action'. J. Mater. Chem. 19, 6649–6687. doi:10.1039/b819218a
Basova, T. V., Vikulova, E. S., Dorovskikh, S. I., Hassan, A., and Morozova, N. B. (2021). The use of noble metal coatings and nanoparticles for the modification of medical implant materials. Mater. Des. 204 204, 109672. doi:10.1016/j.matdes.2021.109672
Benyettou, F., Rezgui, R., Ravaux, F., Jaber, T., Blumer, K., Jouiad, M., et al. (2015). Synthesis of silver nanoparticles for the dual delivery of doxorubicin and alendronate to cancer cells. J. Mater. Chem. B 3, 7237–7245. doi:10.1039/c5tb00994d
Biggs, S., Habgood, M., Jameson, G. J., and Yan, Y.-D. (2000). Aggregate structures formed via a bridging flocculation mechanism. Chem. Eng. J. 80, 13–22. doi:10.1016/s1383-5866(00)00072-1
Bihari, P., Vippola, M., Schultes, S., Praetner, M., Khandoga, A. G., Reichel, C. A., et al. (2008). Optimized dispersion of nanoparticles for biological in vitro and in vivo studies. Part. fibre Toxicol. 5, 14. doi:10.1186/1743-8977-5-14
Biju, V. (2014). Chemical modifications and bioconjugate reactions of nanomaterials for sensing, imaging, drug delivery and therapy. Chem. Soc. Rev. 43, 744–764. doi:10.1039/c3cs60273g
Bozo, I. Y., Drobyshev, A. Y., Redko, N. A., Komlev, V. S., Isaev, A. A., and Deev, R. V. (2021). Bringing a gene-activated bone substitute into clinical practice: From bench to bedside. Front. Bioeng. Biotechnol. 9, 599300. doi:10.3389/fbioe.2021.599300
Brasiunas, B., Popov, A., Ramanavicius, A., and Ramanaviciene, A. (2021). Gold nanoparticle based colorimetric sensing strategy for the determination of reducing sugars. Food Chem. 351, 129238. doi:10.1016/j.foodchem.2021.129238
Butscher, A., Bohner, M., Doebelin, N., Galea, L., Loeffel, O., and Müller, R. (2013). Moisture based three-dimensional printing of calcium phosphate structures for scaffold engineering. Acta Biomater. 9, 5369–5378. doi:10.1016/j.actbio.2012.10.009
Butscher, A., Bohner, M., Hofmann, S., Gauckler, L., and Müller, R. (2011). Structural and material approaches to bone tissue engineering in powder-based three-dimensional printing. Acta Biomater. 7, 907–920. doi:10.1016/j.actbio.2010.09.039
Byrappa, K., Ohara, S., and Adschiri, T. (2008). Nanoparticles synthesis using supercritical fluid technology - towards biomedical applications. Adv. Drug Deliv. Rev. 60, 299–327. doi:10.1016/j.addr.2007.09.001
Cai, Y., Li, H., Du, B., Yang, M., Li, Y., Wu, D., et al. (2011). Ultrasensitive electrochemical immunoassay for BRCA1 using BMIM·BF4-coated SBA-15 as labels and functionalized graphene as enhancer. Biomaterials 32, 2117–2123. doi:10.1016/j.biomaterials.2010.11.058
Cai, Y., Wang, X., Poh, C. K., Tan, H. C., Soe, M. T., Zhang, S., et al. (2014). Accelerated bone growth in vitro by the conjugation of BMP2 peptide with hydroxyapatite on titanium alloy. Colloids and Surfaces B:. Biointerfaces 116, 681–686. doi:10.1016/j.colsurfb.2013.11.004
Campuzano, S., Pedrero, M., Nikoleli, G. P., Pingarron, J. M., and Nikolelis, D. P. (2017). Hybrid 2D-nanomaterials-based electrochemical immunosensing strategies for clinical biomarkers determination. Biosens. Bioelectron. 89, 269–279. doi:10.1016/j.bios.2016.01.042
Cao, L., Huang, Y., Parakhonskiy, B., and Skirtach, A. (2022). Nanoarchitectonics beyond perfect order–not quite perfect but quite useful. Nanoscale 14, 15964–16002. doi:10.1039/d2nr02537j
Casals, E., Pfaller, T., Duschl, A., Oostingh, G. J., and Puntes, V. F. (2011). Hardening of the nanoparticle–protein corona in metal (Au, Ag) and oxide (Fe3O4, CoO, and CeO2) nanoparticles. Small 7, 3479–3486. doi:10.1002/smll.201101511
Chanana, M., Rivera_Gil, P., Correa-Duarte, M. A., Liz-Marzán, L. M., and Parak, W. J. (2013). Physicochemical properties of protein-coated gold nanoparticles in biological fluids and cells before and after proteolytic digestion. Angew. Chem. Int. Ed. 52, 4273–4277. doi:10.1002/ange.201208019
Chanteau, B., Fresnais, J., and Berret, J. F. (2009). Electrosteric enhanced stability of functional sub-10 nm cerium and iron oxide particles in cell culture medium. Langmuir 25, 9064–9070. doi:10.1021/la900833v
Chen, Y. G., Li, C. X., Zhang, Y., Qi, Y. D., Liu, X. H., Feng, J., et al. (2022). Hybrid suture coating for dual-staged control over antibacterial actions to match well wound healing progression. Mater. Horizons 9, 2824–2834. doi:10.1039/d2mh00591c
Chen, Y., Lu, X., Lamaka, S. V., Ju, P., Blawert, C., Zhang, T., et al. (2020). Active protection of Mg alloy by composite PEO coating loaded with corrosion inhibitors. Appl. Surf. Sci. 504, 144462. doi:10.1016/j.apsusc.2019.144462
Chernozem, R. V., Pariy, I., Surmeneva, M. A., Shvartsman, V. V., Plankaert, G., Verduijn, J., et al. (2022). Cell behavior changes and enzymatic biodegradation of hybrid electrospun poly(3-hydroxybutyrate)-based scaffolds with an enhanced piezoresponse after the addition of reduced graphene oxide. Adv. Healthc. Mater., 2201726. doi:10.1002/adhm.202201726
Choi, Y., Kim, S., Choi, M. H., Ryoo, S. R., Park, J., Min, D. H., et al. (2014). Highly biocompatible carbon nanodots for simultaneous bioimaging and targeted photodynamic therapy in vitro and in vivo. Adv. Funct. Mater. 24, 5781–5789. doi:10.1002/adfm.201400961
Christau, S., MöLler, T., Yenice, Z., Genzer, J., and Von Klitzing, R. (2014). Brush/gold nanoparticle hybrids: Effect of grafting density on the particle uptake and distribution within weak polyelectrolyte brushes. Langmuir 30, 13033–13041. doi:10.1021/la503432x
Climent, E., and Rurack, K. (2021). Combining electrochemiluminescence detection with aptamer-gated indicator releasing mesoporous nanoparticles enables ppt sensitivity for strip-based rapid tests. Angew. Chemie-International Ed. 60, 26287–26297. doi:10.1002/anie.202110744
Cui, H., Shao, Z.-S., Song, Z., Wang, Y.-B., and Wang, H.-S. (2020). Development of gold nanoclusters: From preparation to applications in the field of biomedicine. J. Mater. Chem. C 8, 14312–14333. doi:10.1039/d0tc03443f
Cui, Q., He, F., Li, L., and Möhwald, H. (2014). Controllable metal-enhanced fluorescence in organized films and colloidal system. Adv. Colloid Interface Sci. 207, 164–177. doi:10.1016/j.cis.2013.10.011
Cui, W., Wang, A., Zhao, J., and Li, J. (2016). Biomacromolecules based core/shell architecture toward biomedical applications. Adv. Colloid Interface Sci. 237, 43–51. doi:10.1016/j.cis.2016.10.001
Dai, X., Zhao, X., Liu, Y., Chen, B., Ding, X., Zhao, N., et al. (2021). Controlled synthesis and surface engineering of janus chitosan-gold nanoparticles for photoacoustic imaging-guided synergistic gene/photothermal therapy. Small 17, 2006004. doi:10.1002/smll.202006004
De Gennes, P. G. (1987). Polymers at an interface; a simplified view. Adv. Colloid Interface Sci. 27, 189–209. doi:10.1016/0001-8686(87)85003-0
Del Pino, P., Pelaz, B., Zhang, Q., Maffre, P., Nienhaus, G. U., and Parak, W. J. (2014). Protein corona formation around nanoparticles–from the past to the future. Mater. Horizons 1, 301–313. doi:10.1039/c3mh00106g
Deng, M., Wang, L., Hoche, D., Lamaka, S. V., Wang, C., Snihirova, D., et al. (2021). Approaching "stainless magnesium" by Ca micro-alloying. Mater. horizons 8, 589–596. doi:10.1039/d0mh01380c
Digiovanni, C. W., Lin, S. S., Baumhauer, J. F., Daniels, T., Younger, A., Glazebrook, M., et al. (2013). Recombinant human platelet-derived growth factor-BB and beta-tricalcium phosphate (rhPDGF-BB/β-TCP): An alternative to autogenous bone graft. J. Bone Jt. Surg. Am. Volume 95, 1184–1192. doi:10.2106/jbjs.k.01422
Digiovanni, C. W., Lin, S. S., Daniels, T. R., Glazebrook, M., Evangelista, P., Donahue, R., et al. (2016). The importance of sufficient graft material in achieving foot or ankle fusion. J. Bone Jt. Surg. Am. Volume 98, 1260–1267. doi:10.2106/jbjs.15.00879
Ding, C., Zhu, A., and Tian, Y. (2014). Functional surface engineering of C-dots for fluorescent biosensing and in vivo bioimaging. Accounts Chem. Res. 47, 20–30. doi:10.1021/ar400023s
Duan, X. P., Chan, C., and Lin, W. B. (2019). Nanoparticle-Mediated immunogenic cell death enables and potentiates cancer immunotherapy. Angew. Chemie-International Ed. 58, 670–680. doi:10.1002/anie.201804882
Dutta, S., Strack, G., and Kurup, P. (2019). Gold nanostar electrodes for heavy metal detection. Sensors and Actuators B:. Chemical 281, 383–391. doi:10.1016/j.snb.2018.10.111
Edwards, S., and Williams, D. (2004). Double layers and interparticle forces in colloid science and biology: Analytic results for the effect of ionic dispersion forces. Phys. Rev. Lett. 92, 248303. doi:10.1103/physrevlett.92.248303
Elbakry, A., Zaky, A., Liebl, R., Rachel, R., Goepferich, A., and Breunig, M. (2009). Layer-by-Layer assembled gold nanoparticles for siRNA delivery. Nano Lett. 9, 2059–2064. doi:10.1021/nl9003865
Espinosa, A., Reguera, J., Curcio, A., Munoz-Noval, A., Kuttner, C., Van De Walle, A., et al. (2020). Janus magnetic-plasmonic nanoparticles for magnetically guided and thermally activated cancer therapy. Small (Weinheim der Bergstrasse, Ger. 16, e1904960. doi:10.1002/smll.201904960
Fan, H., Wang, L., Zhao, K., Li, N., Shi, Z., Ge, Z., et al. (2010). Fabrication, mechanical properties, and biocompatibility of graphene-reinforced chitosan composites. Biomacromolecules 11, 2345–2351. doi:10.1021/bm100470q
Feng, H., and Qian, Z. (2018). Functional carbon quantum dots: A versatile platform for chemosensing and biosensing. Chemical record (New York, N.Y.). Chem. Rec. 18, 491–505. doi:10.1002/tcr.201700055
Feng, L., Li, W., Bao, J., Zheng, Y., Li, Y., Ma, Y., et al. (2019). Synthesis and luminescence properties of core-shell-shell composites: SiO2@PMDA-Si-Tb@SiO2 and SiO2@PMDA-Si-Tb-phen@SiO2. Nanomaterials (basel, Switzerland) 9.
Feoktistova, N. A., Balabushevich, N. G., Skirtach, A. G., Volodkin, D., and Vikulina, A. S. (2020). Inter-protein interactions govern protein loading into porous vaterite CaCO3 crystals. Phys. Chem. Chem. Phys. PCCP 22, 9713–9722. doi:10.1039/d0cp00404a
Foldbjerg, R., Jiang, X., Miclăuş, T., Chen, C., Autrup, H., and Beer, C. (2015). Silver nanoparticles – wolves in sheep's clothing? Toxicol. Res. 4, 563–575. doi:10.1039/c4tx00110a
Fraire, J. C., Stremersch, S., Bouckaert, D., Monteyne, T., De Beer, T., Wuytens, P., et al. (2019). Improved label-free identification of individual exosome-like vesicles with Au@Ag nanoparticles as SERS substrate. ACS Appl. Mater. interfaces 11, 39424–39435. doi:10.1021/acsami.9b11473
Fu, Q., Xiao, S., Tang, X., Chen, Y., and Hu, T. (2019). Amphiphilic fullerenes employed to improve the quality of perovskite films and the stability of perovskite solar cells. ACS Appl. Mater. Interfaces 11, 24782–24788. doi:10.1021/acsami.9b07149
Gaharwar, A. K., Cross, L. M., Peak, C. W., Gold, K., Carrow, J. K., Brokesh, A., et al. (2019). 2D nanoclay for biomedical applications: Regenerative medicine, therapeutic delivery, and additive manufacturing. Adv. Mater. 31, 1900332. doi:10.1002/adma.201900332
Gao, C., Lin, Z., Wu, Z., Lin, X., and He, Q. (2016). Stem-cell-membrane camouflaging on near-infrared photoactivated upconversion nanoarchitectures for in vivo remote-controlled photodynamic therapy. ACS Appl. Mater. interfaces 8, 34252–34260. doi:10.1021/acsami.6b12865
Gaponik, N., and Rogach, A. L. (2010). Thiol-capped CdTe nanocrystals: Progress and perspectives of the related research fields. Phys. Chem. Chem. Phys. 12, 8685–8693. doi:10.1039/c000916d
Garg, S., and Serruys, P. W. (2010). Coronary stents: Current status. J. Am. Coll. Cardiol. 56, S1–S42. doi:10.1016/j.jacc.2010.06.007
Gitipour, A., Thiel, S. W., Scheckel, K. G., and Tolaymat, T. (2016). Anaerobic toxicity of cationic silver nanoparticles. Sci. Total Environ. 557-558, 363–368. doi:10.1016/j.scitotenv.2016.02.190
Glazebrook, M., Younger, A., Wing, K., and Lalonde, K.-A. (2013). A prospective pilot study of B2A-coated ceramic granules (Amplex) compared to autograft for ankle and hindfoot arthrodesis. Foot Ankle Int. 34, 1055–1063. doi:10.1177/1071100713481459
Go, Y. K., and Leal, C. (2021). Polymer-lipid hybrid materials. Chem. Rev. 121, 13996–14030. doi:10.1021/acs.chemrev.1c00755
Graf, C., Gao, Q., Schütz, I., Noufele, C. N., Ruan, W., Posselt, U., et al. (2012). Surface functionalization of silica nanoparticles supports colloidal stability in physiological media and facilitates internalization in cells. Langmuir 28, 7598–7613. doi:10.1021/la204913t
Gu, Y., Yu, L., Mou, J., Wu, D., Xu, M., Zhou, P., et al. (2020). Research strategies to develop environmentally friendly marine antifouling coatings. Mar. Drugs 18 18, 371. doi:10.3390/md18070371
Guner, H., Ozgur, E., Kokturk, G., Celik, M., Esen, E., Topal, A. E., et al. (2017). A smartphone based surface plasmon resonance imaging (SPRi) platform for on-site biodetection. Sensors and actuators B:. Chemical 239, 571–577. doi:10.1016/j.snb.2016.08.061
Guo, X., Li, X., Chan, L., Huang, W., and Chen, T. (2021). Edible CaCO3 nanoparticles stabilized Pickering emulsion as calcium-fortified formulation. J. nanobiotechnology 19, 67–16. doi:10.1186/s12951-021-00807-6
Guo, Y., Jia, H. R., Zhang, X., Zhang, X., Sun, Q., Wang, S. Z., et al. (2020). A glucose/oxygen-exhausting nanoreactor for starvation-and hypoxia-activated sustainable and cascade chemo-chemodynamic therapy. Small 16, 2000897. doi:10.1002/smll.202000897
Gupta, B., Poudel, B. K., Ruttala, H. B., Regmi, S., Pathak, S., Gautam, M., et al. (2018). Hyaluronic acid-capped compact silica-supported mesoporous titania nanoparticles for ligand-directed delivery of doxorubicin. Acta Biomater. 80, 364–377. doi:10.1016/j.actbio.2018.09.006
Han, L., Jiang, Y., Lv, C., Gan, D., Wang, K., Ge, X., et al. (2019). Mussel-inspired hybrid coating functionalized porous hydroxyapatite scaffolds for bone tissue regeneration. Colloids and Surfaces B:. Biointerfaces 179, 470–478. doi:10.1016/j.colsurfb.2019.04.024
Haskell, A. K., Sulman, A. M., Golikova, E. P., Stein, B. D., Pink, M., Morgan, D. G., et al. (2020). Glucose oxidase immobilized on magnetic zirconia: Controlling catalytic performance and stability. ACS omega 5, 12329–12338. doi:10.1021/acsomega.0c01067
Heuer-Jungemann, A., Feliu, N., Bakaimi, I., Hamaly, M., Alkilany, A., Chakraborty, I., et al. (2019). The role of ligands in the chemical synthesis and applications of inorganic nanoparticles. Chem. Rev. 119, 4819–4880. doi:10.1021/acs.chemrev.8b00733
Hirt, N., and Body-Malapel, M. (2020). Immunotoxicity and intestinal effects of nano- and microplastics: A review of the literature. Part. Fibre Toxicol. 17, 57. doi:10.1186/s12989-020-00387-7
Ho-Shui-Ling, A., Bolander, J., Rustom, L. E., Johnson, A. W., Luyten, F. P., and Picart, C. (2018). Bone regeneration strategies: Engineered scaffolds, bioactive molecules and stem cells current stage and future perspectives. Biomaterials 180, 143–162. doi:10.1016/j.biomaterials.2018.07.017
Hou, J., Dong, G., Luu, B., Sengpiel, R. G., Ye, Y., Wessling, M., et al. (2014). Hybrid membrane with TiO2 based bio-catalytic nanoparticle suspension system for the degradation of bisphenol-A. Bioresour. Technol. 169, 475–483. doi:10.1016/j.biortech.2014.07.031
Hua, Z., Yu, T., Liu, D., and Xianyu, Y. (2021). Recent advances in gold nanoparticles-based biosensors for food safety detection. Biosens. Bioelectron. 179, 113076. doi:10.1016/j.bios.2021.113076
Huang, X., Tan, C. L., Yin, Z. Y., and Zhang, H. (2014a). 25th anniversary article: Hybrid nanostructures based on two-dimensional nanomaterials. Adv. Mater. 26, 2185–2204. doi:10.1002/adma.201304964
Huang, X., Tepylo, N., Pommier-Budinger, V., Budinger, M., Bonaccurso, E., Villedieu, P., et al. (2019). A survey of icephobic coatings and their potential use in a hybrid coating/active ice protection system for aerospace applications. Prog. Aerosp. Sci. 105, 74–97. doi:10.1016/j.paerosci.2019.01.002
Huang, Y., Cao, L., Parakhonskiy, B. V., and Skirtach, A. G. (2022). Hard, soft, and hard-and-soft drug delivery carriers based on CaCO3 and alginate biomaterials: Synthesis, properties, pharmaceutical applications. Pharmaceutics 14, 909. doi:10.3390/pharmaceutics14050909
Huang, Y., Ng, H. C., Ng, X. W., and Subbu, V. (2014b). Drug-eluting biostable and erodible stents. J. Control. release official J. Control. Release Soc. 193, 188–201. doi:10.1016/j.jconrel.2014.05.011
Huang, Y., Zhou, J., Feng, H., Zheng, J., Ma, H.-M., Liu, W., et al. (2016). A dual-channel fluorescent chemosensor for discriminative detection of glutathione based on functionalized carbon quantum dots. Biosens. Bioelectron. 86, 748–755. doi:10.1016/j.bios.2016.07.081
Ikeda, A. (2013). Water-soluble fullerenes using solubilizing agents, and their applications. J. Inclusion Phenom. Macrocycl. Chem. 77, 49–65. doi:10.1007/s10847-013-0319-9
Iqbal, J., Gunn, J., and Serruys, P. W. (2013). Coronary stents: Historical development, current status and future directions. Br. Med. Bull. 106, 193–211. doi:10.1093/bmb/ldt009
Ji, S., Wang, Q., Xu, Q., Wu, M., and Shi, W. (2020a). Electrospun organic/inorganic hybrid nanofibers as low-cytotoxicity and recyclable photocatalysts. Appl. Surf. Sci. 532 532, 147430. doi:10.1016/j.apsusc.2020.147430
Ji, X., Yuan, X., Ma, L., Bi, B., Zhu, H., Lei, Z., et al. (2020b). Mesenchymal stem cell-loaded thermosensitive hydroxypropyl chitin hydrogel combined with a three-dimensional-printed poly(ε-caprolactone)/nano-hydroxyapatite scaffold to repair bone defects via osteogenesis, angiogenesis and immunomodulation. Theranostics 10, 725–740. doi:10.7150/thno.39167
Jiang, G., Erdem, O., Hübner, R., Georgi, M., Wei, W., Fan, X., et al. (2021). Mechanosynthesis of polymer-stabilized lead bromide perovskites: Insight into the formation and phase conversion of nanoparticles. Nano Res. 14, 1078–1086. doi:10.1007/s12274-020-3152-7
Jun, Y. W., Seo, J. W., and Cheon, A. (2008). Nanoscaling laws of magnetic nanoparticles and their applicabilities in biomedical sciences. Accounts Chem. Res. 41, 179–189. doi:10.1021/ar700121f
Kang, H., Buchman, J. T., Rodriguez, R. S., Ring, H. L., He, J., Bantz, K. C., et al. (2019). Stabilization of silver and gold nanoparticles: Preservation and improvement of plasmonic functionalities. Chem. Rev. 119, 664–699. doi:10.1021/acs.chemrev.8b00341
Karthick, V., Shrestha, L. K., Kumar, V. G., Pranjali, P., Kumar, D., Pal, A., et al. (2022). Nanoarchitectonics horizons: Materials for life sciences. Nanoscale 14, 10630–10647. doi:10.1039/d2nr02293a
Kaufmann, A., Hampel, S., Rieger, C., Kunhardt, D., Schendel, D., Füssel, S., et al. (2017). Systematic evaluation of oligodeoxynucleotide binding and hybridization to modified multi-walled carbon nanotubes. J. nanobiotechnology 15, 53. doi:10.1186/s12951-017-0288-z
Kaushik, M., and Moores, A. (2016). Review: Nanocelluloses as versatile supports for metal nanoparticles and their applications in catalysis. Green Chem. 18, 622–637. doi:10.1039/c5gc02500a
Kiadeh, S. Z. H., Ghaee, A., Farokhi, M., Nourmohammadi, J., Bahi, A., and Ko, F. K. (2021). S., Ghaee, A., Farokhi, M., Nourmohammadi, J., Bahi, A., and Ko, F.KElectrospun pectin/modified copper-based metal-organic framework (MOF) nanofibers as a drug delivery system. Int. J. Biol. Macromol. 173, 351–365. doi:10.1016/j.ijbiomac.2021.01.058
Kickelbick, G. (2003). Concepts for the incorporation of inorganic building blocks into organic polymers on a nanoscale. Prog. Polym. Sci. 28, 83–114. doi:10.1016/s0079-6700(02)00019-9
Kim, D.-Y., Kim, M., Shinde, S., Sung, J.-S., and Ghodake, G. (2017). Cytotoxicity and antibacterial assessment of gallic acid capped gold nanoparticles. Colloids and Surfaces B:. Biointerfaces 149, 162–167. doi:10.1016/j.colsurfb.2016.10.017
King, B. M., and Fiegel, J. (2022). Zwitterionic polymer coatings enhance gold nanoparticle stability and uptake in various biological environments. AAPS J. 24, 18. doi:10.1208/s12248-021-00652-3
Kopecek, J. (2009). Hydrogels: From soft contact lenses and implants to self-assembled nanomaterials. J. Polym. Sci. Part a-Polymer Chem. 47, 5929–5946. doi:10.1002/pola.23607
Kozlova, A. A., German, S. V., Atkin, V. S., Zyev, V. V., Astle, M. A., Bratashov, D. N., et al. (2020). Magnetic composite submicron carriers with structure-dependent MRI contrast. Inorganics 8, 11. doi:10.3390/inorganics8020011
Lavrador, P., Esteves, M. R., Gaspar, V. M., and Mano, J. F. (2021). Stimuli-responsive nanocomposite hydrogels for biomedical applications. Adv. Funct. Mater. 31, 2005941. doi:10.1002/adfm.202005941
Lee, H. (2021). Molecular modeling of protein corona formation and its interactions with nanoparticles and cell membranes for nanomedicine applications. Pharm. 13 13, 637. doi:10.3390/pharmaceutics13050637
Lee, H., Dellatore, S. M., Miller, W. M., and Messersmith, P. B. (2007). Mussel-inspired surface chemistry for multifunctional coatings. Sci. (New York, N.Y.) 318, 426–430. doi:10.1126/science.1147241
Lee, J. W., Choi, S.-R., and Heo, J. H. (2021). Simultaneous stabilization and functionalization of gold nanoparticles via biomolecule conjugation: Progress and perspectives. ACS Appl. Mater. Interfaces 13, 42311–42328. doi:10.1021/acsami.1c10436
Lee, M.-Y., Park, S.-J., Park, K., Kim, K. S., Lee, H., and Hahn, S. K. (2011). Target-specific gene silencing of layer-by-layer assembled gold-cysteamine/siRNA/PEI/HA nanocomplex. ACS Nano 5, 6138–6147. doi:10.1021/nn2017793
Lengert, E., Parakhonskiy, B., Khalenkow, D., Zečić, A., Vangheel, M., Moreno, J. M. M., et al. (2018). Laser-induced remote release in vivo in C. elegans from novel silver nanoparticles-alginate hydrogel shells. Nanoscale 10, 17249–17256. doi:10.1039/c8nr00893k
Lengert, E., Saveleva, M., Abalymov, A., Atkin, V., Wuytens, P. C., Kamyshinsky, R., et al. (2017). Silver alginate hydrogel micro- and nanocontainers for theranostics: Synthesis, encapsulation, remote release, and detection. ACS Appl. Mater. interfaces 9, 21949–21958. doi:10.1021/acsami.7b08147
Leroux, F., Rabu, P., Sommerdijk, N. A., and Taubert, A. (2015). Two-Dimensional hybrid materials: Transferring technology from biology to society. Eur. J. Inorg. Chem. 2015, 1089–1095. doi:10.1002/ejic.201500153
Levy, L., Gurov, A., and Radian, A. (2020). The effect of gallic acid interactions with iron-coated clay on surface redox reactivity. Water Res. 184, 116190. doi:10.1016/j.watres.2020.116190
Lewinski, N., Colvin, V., and Drezek, R. (2008). Cytotoxicity of nanoparticles. Small (Weinheim Der Bergstrasse, Ger. 4, 26–49. doi:10.1002/smll.200700595
Li, B. K., Cui, Y. H., Wang, X. Y., and Tang, R. K. (2021a). Novel nanomaterial-organism hybrids with biomedical potential. Wiley Interdiscip. Reviews-Nanomedicine Nanobiotechnology 13 13, e1706. doi:10.1002/wnan.1706
Li, C., Zhang, Y., Li, Z., Mei, E., Lin, J., Li, F., et al. (2018a). Theranostics: Light-Responsive biodegradable nanorattles for cancer theranostics (adv. Mater. 8/2018). Adv. Mater. 30, 1870049. doi:10.1002/adma.201870049
Li, C., Zhao, Z. H., Luo, Y. F., Ning, T. T., Liu, P. X., Chen, Q. J., et al. (2021b). Macrophage-disguised manganese dioxide nanoparticles for neuroprotection by reducing oxidative stress and modulating inflammatory microenvironment in acute ischemic stroke. Adv. Sci. 8 8, 2101526. doi:10.1002/advs.202101526
Li, J., Feng, X., Fei, J., Cai, P., Huang, J., and Li, J. (2016). Integrating photosystem II into a porous TiO2 nanotube network toward highly efficient photo-bioelectrochemical cells. J. Mater. Chem. A 4, 12197–12204. doi:10.1039/c6ta04964h
Li, J., Khalenkow, D., Volodkin, D., Lapanje, A., Skirtach, A. G., and Parakhonskiy, B. V. (2022a). Surface enhanced Raman scattering (SERS)-active bacterial detection by Layer-by-Layer (LbL) assembly all-nanoparticle microcapsules.” in Colloids and surfaces A: Physicochemical and engineering aspects.
Li, J., Parakhonskiy, B. V., and Skirtach, A. G. (2022b). A decade of developing applications exploiting the properties of polyelectrolyte multilayer capsules. Chem. Commun. Camb. Engl. doi:10.1039/d2cc04806j
Li, Q. F., Zeng, L. X., Wang, J. C., Tang, D. P., Liu, B. Q., Chen, G. N., et al. (2011). Magnetic mesoporous organic-inorganic NiCo2O4 hybrid nanomaterials for electrochemical immunosensors. Acs Appl. Mater. Interfaces 3, 1366–1373. doi:10.1021/am200228k
Li, X., Luo, W., Ng, T. W., Leung, P. C., Zhang, C., Leung, K. C.-F., et al. (2017). Nanoparticle-encapsulated baicalein markedly modulates pro-inflammatory response in gingival epithelial cells. Nanoscale 9, 12897–12907. doi:10.1039/c7nr02546g
Li, X., Wei, J., Li, Q., Zheng, S., Xu, Y., Du, P., et al. (2018b). Nitrogen-doped cobalt oxide nanostructures derived from cobalt–alanine complexes for high-performance oxygen evolution reactions. Adv. Funct. Mater. 28, 1800886. doi:10.1002/adfm.201800886
Li, Y., He, L., Huang, C. Z., and Li, Y. F. (2019). Silver-based metal-organic gels as novel coreactant for enhancing electrochemiluminescence and its biosensing potential. Biosens. Bioelectron. 134, 29–35. doi:10.1016/j.bios.2019.03.058
Li, Y., Yang, W., Li, X., Zhang, X., Wang, C., Meng, X., et al. (2015). Improving osteointegration and osteogenesis of three-dimensional porous Ti6Al4V scaffolds by polydopamine-assisted biomimetic hydroxyapatite coating. ACS Appl. Mater. interfaces 7, 5715–5724. doi:10.1021/acsami.5b00331
Lim, S. Y., Shen, W., and Gao, Z. (2015). Carbon quantum dots and their applications. Chem. Soc. Rev. 44, 362–381. doi:10.1039/c4cs00269e
Lin, L. S., Yang, X. Y., Niu, G., Song, J. B., Yang, H. H., and Chen, X. Y. (2016). Dual-enhanced photothermal conversion properties of reduced graphene oxide-coated gold superparticles for light-triggered acoustic and thermal theranostics. Nanoscale 8, 2116–2122. doi:10.1039/c5nr07552a
Lisuzzo, L., Cavallaro, G., Milioto, S., and Lazzara, G. (2019). Layered composite based on halloysite and natural polymers: A carrier for the pH controlled release of drugs. New J. Chem. 43, 10887–10893. doi:10.1039/c9nj02565k
Liu, D., Wang, Z., and Jiang, X. (2011). Gold nanoparticles for the colorimetric and fluorescent detection of ions and small organic molecules. Nanoscale 3, 1421–1433. doi:10.1039/c0nr00887g
Liu, J. L., Shi, W. X., and Wang, X. (2019). Cluster-nuclei coassembled into two-dimensional hybrid CuO-pma sub-1 nm nanosheets. J. Am. Chem. Soc. 141, 18754–18758. doi:10.1021/jacs.9b08818
Liu, M., Qiu, X. Q., Hashirnoto, K., and Miyauchi, M. (2014). Cu(II) nanocluster-grafted, Nb-doped TiO2 as an efficient visible-light-sensitive photocatalyst based on energy-level matching between surface and bulk states. J. Mater. Chem. A 2, 13571–13579. doi:10.1039/c4ta02211d
Liu, N., Tang, M., and Ding, J. (2020a). The interaction between nanoparticles-protein corona complex and cells and its toxic effect on cells. Chemosphere 245, 125624. doi:10.1016/j.chemosphere.2019.125624
Liu, R., Jiang, L., Yu, Z., Jing, X., Liang, X., Wang, D., et al. (2021). MXene (Ti3C2Tx)-Ag nanocomplex as efficient and quantitative SERS biosensor platform by in-situ PDDA electrostatic self-assembly synthesis strategy. Sensors and Actuators B:. Chemical 333, 129581. doi:10.1016/j.snb.2021.129581
Liu, S., Chen, X., Bao, L., Liu, T., Yuan, P., Yang, X., et al. (2020b). Treatment of infarcted heart tissue via the capture and local delivery of circulating exosomes through antibody-conjugated magnetic nanoparticles. Nat. Biomed. Eng. 4, 1063–1075. doi:10.1038/s41551-020-00637-1
Lutich, A. A., Jiang, G., Susha, A. S., Rogach, A. L., Stefani, F. D., and Feldmann, J. (2009). Energy transfer versus charge separation in type-ii hybrid organic− inorganic nanocomposites. Nano Lett. 9, 2636–2640. doi:10.1021/nl900978a
Lv, H., Cui, S., Yang, Q., Song, X., Wang, D., Hu, J., et al. (2021). AgNPs-incorporated nanofiber mats: Relationship between AgNPs size/content, silver release, cytotoxicity, and antibacterial activity. Mater. Sci. Eng. C 118, 111331. doi:10.1016/j.msec.2020.111331
Lvov, Y. M., Shchukin, D. G., Mohwald, H., and Price, R. R. (2008). Halloysite clay nanotubes for controlled release of protective agents. ACS Nano 2, 814–820. doi:10.1021/nn800259q
Mahmood, A., Guo, W. H., Tabassum, H., and Zou, R. Q. (2016). Metal-Organic framework-based nanomaterials for electrocatalysis. Adv. Energy Mater. 6 6, 1600423. doi:10.1002/aenm.201600423
Majumder, J., and Minko, T. (2021). Targeted nanotherapeutics for respiratory diseases: Cancer, fibrosis, and coronavirus. Adv. Ther. 4, 2000203.
Mann, S. (2009). Self-assembly and transformation of hybrid nano-objects and nanostructures under equilibrium and non-equilibrium conditions. Nat. Mater. 8, 781–792. doi:10.1038/nmat2496
Martinelli, A., Nitti, A., Giannotta, G., Po, R., and Pasini, D. (2022). 3D printing of conductive organic polymers: Challenges and opportunities towards dynamic and electrically responsive materials. Mater. Today Chem. 26, 101135. doi:10.1016/j.mtchem.2022.101135
Mcmahon, A., Chen, W., and Li, F. (2020). Old wine in new bottles: Advanced drug delivery systems for disulfiram-based cancer therapy. J. Control. release official J. Control. Release Soc. 319, 352–359. doi:10.1016/j.jconrel.2020.01.001
Mehdikhani-Nahrkhalaji, M., Fathi, M. H., Mortazavi, V., Mousavi, S. B., Akhavan, A., Haghighat, A., et al. (2015). Biodegradable nanocomposite coatings accelerate bone healing: In vivo evaluation. Dent. Res. J. 12, 89–99. doi:10.4103/1735-3327.150342
Melnikau, D., Esteban, R., Savateeva, D., Sánchez-Iglesias, A., Grzelczak, M., Schmidt, M. K., et al. (2016). Rabi splitting in photoluminescence spectra of hybrid systems of gold nanorods and J-aggregates. J. Phys. Chem. Lett. 7, 354–362. doi:10.1021/acs.jpclett.5b02512
Melnikau, D., Hendel, T., Linkov, P. A., Samokhvalov, P. S., Nabiev, I. R., and Rakovich, Y. P. (2018). Energy transfer between single semiconductor quantum dots and organic dye molecules. Zeitschrift Fur Physikalische Chemie-International J. Res. Phys. Chem. Chem. Phys. 232, 1513–1526. doi:10.1515/zpch-2018-1144
Mirkin, C. A., Letsinger, R. L., Mucic, R. C., and Storhoff, J. J. (1996). A DNA-based method for rationally assembling nanoparticles into macroscopic materials. Nature 382, 607–609. doi:10.1038/382607a0
Miyazawa, A., Ako, J., Hongo, Y., Hur, S.-H., Tsujino, I., Courtney, B. K., et al. (2008). Comparison of vascular response to zotarolimus-eluting stent versus sirolimus-eluting stent: Intravascular ultrasound results from ENDEAVOR III. Am. Heart J. 155, 108–113. doi:10.1016/j.ahj.2007.08.008
Moglia, I., Santiago, M., Guerrero, S., Soler, M., Olivera-Nappa, A., and Kogan, M. J. (2021). Enhanced cellular uptake of H-chain human ferritin containing gold nanoparticles. Pharm. 13 13, 1966. doi:10.3390/pharmaceutics13111966
Moore, T. L., Rodriguez-Lorenzo, L., Hirsch, V., Balog, S., Urban, D., Jud, C., et al. (2015). Nanoparticle colloidal stability in cell culture media and impact on cellular interactions. Chem. Soc. Rev. 44, 6287–6305. doi:10.1039/c4cs00487f
Moreels, I., Fritzinger, B., Martins, J. C., and Hens, Z. (2008). Surface chemistry of colloidal PbSe nanocrystals. J. Am. Chem. Soc. 130, 15081–15086. doi:10.1021/ja803994m
Mosquera, J., García, I., Henriksen-Lacey, M., González-Rubio, G., and Liz-Marzán, L. M. (2018). Reducing protein corona formation and enhancing colloidal stability of gold nanoparticles by capping with silica monolayers. Chem. Mater. 31, 57–61. doi:10.1021/acs.chemmater.8b04647
Moustafa, H., Morsy, M., Ateia, M. A., and Abdel-Haleem, F. M. (2021). Ultrafast response humidity sensors based on polyvinyl chloride/graphene oxide nanocomposites for intelligent food packaging. Sensors Actuators a-Physical 331 331, 112918. doi:10.1016/j.sna.2021.112918
Mu, W., Chu, Q., Liu, Y., and Zhang, N. (2020). A review on nano-based drug delivery system for cancer chemoimmunotherapy. Nano-Micro Lett. 12, 142–165. doi:10.1007/s40820-020-00482-6
Muderrisoglu, C., Saveleva, M., Abalymov, A., Van Der Meeren, L., Ivanova, A., Atkin, V., et al. (2018). Nanostructured biointerfaces based on bioceramic calcium carbonate/hydrogel coatings on titanium with an active enzyme for stimulating osteoblasts growth. Adv. Mater. Interfaces 5 5, 1800452. doi:10.1002/admi.201800452
Mulvaney, P., Giersig, M., and Henglein, A. (1992). Surface chemistry of colloidal gold: Deposition of lead and accompanying optical effects. J. Phys. Chem. 96, 10419–10424. doi:10.1021/j100204a056
Murugappan, G., and Sreeram, K. J. (2021). Nano-biocatalyst: Bi-Functionalization of protease and amylase on copper oxide nanoparticles. Colloids surfaces. B, Biointerfaces 197, 111386. doi:10.1016/j.colsurfb.2020.111386
Nam, Y. T., Choi, J., Kang, K. M., Kim, D. W., and Jung, H.-T. (2016). Enhanced stability of laminated graphene oxide membranes for nanofiltration via interstitial amide bonding. ACS Appl. Mater. Interfaces 8, 27376–27382. doi:10.1021/acsami.6b09912
Nekoueian, K., Amiri, M., Sillanpää, M., Marken, F., Boukherroub, R., and Szunerits, S. (2019). Carbon-based quantum particles: An electroanalytical and biomedical perspective. Chem. Soc. Rev. 48, 4281–4316. doi:10.1039/c8cs00445e
Ni, K. Y., Luo, T. K., Nash, G. T., and Lin, W. B. (2020). Nanoscale metal-organic frameworks for cancer immunotherapy. Accounts Chem. Res. 53, 1739–1748. doi:10.1021/acs.accounts.0c00313
Nie, S. (2010). Understanding and overcoming major barriers in cancer nanomedicine. Nanomedicine Lond. Engl. 5, 523–528. doi:10.2217/nnm.10.23
Niinomi, M., Nakai, M., and Hieda, J. (2012). Development of new metallic alloys for biomedical applications. Acta biomater. 8, 3888–3903. doi:10.1016/j.actbio.2012.06.037
Nitti, A., Carfora, R., Assanelli, G., Notari, M., and Pasini, D. (2022). Single-Chain polymer nanoparticles for addressing morphologies and functions at the nanoscale: A review. ACS Appl. Nano Mater. 5, 13985–13997. doi:10.1021/acsanm.2c02313
Nugroho, F. a. A., Eklund, R., Nilsson, S., and Langhammer, C. (2018). A fiber-optic nanoplasmonic hydrogen sensor via pattern-transfer of nanofabricated PdAu alloy nanostructures. Nanoscale 10, 20533–20539. doi:10.1039/c8nr03751e
Oladimeji, O., Akinyelu, J., Daniels, A., and Singh, M. (2021). Modified gold nanoparticles for efficient delivery of betulinic acid to cancer cell mitochondria. Int. J. Mol. Sci. 22, 5072. doi:10.3390/ijms22105072
Orlovskii, V., Komlev, V., and Barinov, S. (2002). Hydroxyapatite and hydroxyapatite-based ceramics. Inorg. Mater. 38, 973–984. doi:10.1023/a:1020585800572
Panáček, A., Kvítek, L., Smékalová, M., Večeřová, R., Kolář, M., Röderová, M., et al. (2018). Bacterial resistance to silver nanoparticles and how to overcome it. Nat. Nanotechnol. 13, 65–71. doi:10.1038/s41565-017-0013-y
Papafaklis, M. I., Chatzizisis, Y. S., Naka, K. K., Giannoglou, G. D., and Michalis, L. K. (2012). Drug-eluting stent restenosis: Effect of drug type, release kinetics, hemodynamics and coating strategy. Pharmacol. Ther. 134, 43–53. doi:10.1016/j.pharmthera.2011.12.006
Parakhonskiy, B., Bedard, M., Bukreeva, T., Sukhorukov, G., Mohwald, H., and Skirtach, A. (2010). Nanoparticles on polyelectrolytes at low concentration: Controlling concentration and size. J. Phys. Chem. C 114, 1996–2002. doi:10.1021/jp904564v
Parakhonskiy, B. V., Foss, C., Carletti, E., Fedel, M., Haase, A., Motta, A., et al. (2013). Tailored intracellular delivery via a crystal phase transition in 400 nm vaterite particles. Biomaterials Sci. 1, 1273–1281. doi:10.1039/c3bm60141b
Parakhonskiy, B. V., Haase, A., and Antolini, R. (2012a). Sub-micrometer vaterite containers: Synthesis, substance loading, and release. Angewandte Chemie Int. ed. Engl. 51, 1221–1223. doi:10.1002/ange.201104316
Parakhonskiy, B. V., Haase, A., and Antolini, R. (2012b). Sub-micrometer vaterite containers: Synthesis, substance loading, and release. Angew. Chem. 124, 1221–1223. doi:10.1002/ange.201104316
Parakhonskiy, B. V., Shilyagina, N. Y., Gusliakova, О. I., Volovetskiy, A. B., Kostyuk, A. B., Balalaeva, I. V., et al. (2021). A method of drug delivery to tumors based on rapidly biodegradable drug-loaded containers. Appl. Mater. Today 25 25, 101199. doi:10.1016/j.apmt.2021.101199
Parakhonskiy, B. V., Svenskaya, Y. I., Yashchenok Acapital Em, C., Fattah, H. A., Inozemtseva, O. A., Tessarolo, F., et al. (2014). Size controlled hydroxyapatite and calcium carbonate particles: Synthesis and their application as templates for SERS platform. Colloids surfaces. B, Biointerfaces 118, 243–248. doi:10.1016/j.colsurfb.2014.03.053
Patungwasa, W., and Hodak, J. H. (2008). pH tunable morphology of the gold nanoparticles produced by citrate reduction. Mater. Chem. Phys. 108, 45–54. doi:10.1016/j.matchemphys.2007.09.001
Peer, D., Karp, J. M., Hong, S., Farokhzad, O. C., Margalit, R., and Langer, R. (2020). Nanocarriers as an emerging platform for cancer therapy. Nano-Enabled Med. Appl., 61–91. doi:10.1201/9780429399039-2
Petry, R., Saboia, V. M., Franqui, L. S., Holanda, C. D. A., Garcia, T. R. R., De Farias, M. A., et al. (2019). On the formation of protein corona on colloidal nanoparticles stabilized by depletant polymers. Mater. Sci. Eng. C, Mater. Biol. Appl. 105, 110080. doi:10.1016/j.msec.2019.110080
Pham, M. D., Epperla, C. P., Hsieh, C.-L., Chang, W., and Chang, H.-C. (2017). Glycosaminoglycans-specific cell targeting and imaging using fluorescent nanodiamonds coated with viral envelope proteins. Anal. Chem. 89, 6527–6534. doi:10.1021/acs.analchem.7b00627
Prabakaran, S., Rajan, M., Geng, Z., and Liu, Y. (2021). Fabrication of substituted hydroxyapatite-starch-clay bio-composite coated titanium implant for new bone formation. Carbohydr. Polym. 271, 118432. doi:10.1016/j.carbpol.2021.118432
Qin, C., Fei, J., Wang, A., Yang, Y., and Li, J. (2015). Rational assembly of a biointerfaced core@shell nanocomplex towards selective and highly efficient synergistic photothermal/photodynamic therapycore@shell nanocomplex towards selective and highly efficient synergistic photothermal/photodynamic therapy. Nanoscale 7, 20197–20210. doi:10.1039/c5nr06501a
Rabie, H., Zhang, Y., Pasquale, N., Lagos, M. J., Batson, P. E., and Lee, K. B. (2019). NIR biosensing of neurotransmitters in stem cell-derived neural interface using advanced core–shell upconversion nanoparticles. Adv. Mater. 31, 1806991. doi:10.1002/adma.201806991
Radziuk, D., Shchukin, D. G., Skirtach, A., Möhwald, H., and Sukhorukov, G. (2007). Synthesis of silver nanoparticles for remote opening of polyelectrolyte microcapsules. Langmuir 23, 4612–4617. doi:10.1021/la063420w
Rahman, M. M., Balu, R., Abraham, A., Dutta, N. K., and Choudhury, N. R. (2021). Engineering a bioactive hybrid coating for in vitro corrosion control of magnesium and its alloy. ACS applied bio materials 4, 5542–5555.
Rapetto, C., and Leoncini, M. (2017). Magmaris: A new generation metallic sirolimus-eluting fully bioresorbable scaffold: Present status and future perspectives. J. Thorac. Dis. 9, S903–S913. doi:10.21037/jtd.2017.06.34
Rigoletto, M., Calza, P., Gaggero, E., and Laurenti, E. (2022). Hybrid materials for the removal of emerging pollutants in water: Classification, synthesis, and properties. Chem. Eng. J. Adv. 10 10, 100252. doi:10.1016/j.ceja.2022.100252
Roberts, T. C., Langer, R., and Wood, M. J. A. (2020). Advances in oligonucleotide drug delivery. Nat. Rev. Drug Discov. 19, 673–694. doi:10.1038/s41573-020-0075-7
Ruiz-Aguilar, C., Aguilar-Reyes, E., Flores-Martínez, M., León-Patiño, C., and Nuñéz-Anita, R. (2017). Synthesis and characterisation of β-TCP/bioglass/zirconia scaffolds. Adv. Appl. Ceram. 116, 452–461. doi:10.1080/17436753.2017.1356043
Ruiz-Hitzky, E., Ariga, K., and Lvov, Y. M. E. (2008). Bio-inorganic hybrid nanomaterials: Strategies, synthesis, characterization and applications. Wiley-VCH Verlag GmbH Co. KGaA, Weinheim, Ger.
Saba, N., Jawaid, M., Alothman, O. Y., and Paridah, M. T. (2016). A review on dynamic mechanical properties of natural fibre reinforced polymer composites. Constr. Build. Mater. 106, 149–159. doi:10.1016/j.conbuildmat.2015.12.075
Safi, M., Courtois, J., Seigneuret, M., Conjeaud, H., and Berret, J. F. (2011). The effects of aggregation and protein corona on the cellular internalization of iron oxide nanoparticles. Biomaterials 32, 9353–9363. doi:10.1016/j.biomaterials.2011.08.048
Sanchez, C., Belleville, P., Popall, M., and Nicole, L. (2011). Applications of advanced hybrid organic-inorganic nanomaterials: From laboratory to market. Chem. Soc. Rev. 40, 696–753. doi:10.1039/c0cs00136h
Sanchez, C., Julian, B., Belleville, P., and Popall, M. (2005). Applications of hybrid organic-inorganic nanocomposites. J. Mater. Chem. 15, 3559–3592. doi:10.1039/b509097k
Sanfilippo, V., Caruso, V. C. L., Cucci, L. M., Inturri, R., Vaccaro, S., and Satriano, C. (2020). Hyaluronan-Metal gold nanoparticle hybrids for targeted tumor cell therapy. Int. J. Mol. Sci. 21 21, 3085. doi:10.3390/ijms21093085
Sang, L., Zhao, Y., and Burda, C. (2014). TiO2 nanoparticles as functional building blocks. Chem. Rev. 114, 9283–9318. doi:10.1021/cr400629p
Saveleva, M. S., Eftekhari, K., Abalymov, A., Douglas, T. E., Volodkin, D., Parakhonskiy, B. V., et al. (2019). Hierarchy of hybrid materials—the place of inorganics-in-organics in it, their composition and applications. Front. Chem. 7, 179. doi:10.3389/fchem.2019.00179
Selim, M. S., Yang, H., Wang, F. Q., Li, X., Huang, Y., and Fatthallah, N. A. (2018). Silicone/Ag@ SiO2 core–shell nanocomposite as a self-cleaning antifouling coating material. RSC Adv. 8, 9910–9921. doi:10.1039/c8ra00351c
Sergeeva, A., Sergeev, R., Lengert, E., Zakharevich, A., Parakhonskiy, B., Gorin, D., et al. (2015). Composite magnetite and protein containing CaCO3 crystals. External manipulation and Vaterite--> calcite recrystallization-mediated release performance. ACS Appl. Mater. interfaces 7, 21315–21325. doi:10.1021/acsami.5b05848
Sethi, R., and Lee, C. H. (2012). Endothelial progenitor cell capture stent: Safety and effectiveness. J. interventional Cardiol. 25, 493–500. doi:10.1111/j.1540-8183.2012.00740.x
Shahmoradi, B., Maleki, A., and Byrappa, K. (2011). Photocatalytic degradation of Amaranth and Brilliant Blue FCF dyes using in situ modified tungsten doped TiO2 hybrid nanoparticles. Catal. Sci. Technol. 1, 1216–1223. doi:10.1039/c1cy00023c
Sharifi, M., Attar, F., Saboury, A. A., Akhtari, K., Hooshmand, N., Hasan, A., et al. (2019). Plasmonic gold nanoparticles: Optical manipulation, imaging, drug delivery and therapy. J. Control. Release Official J. Control. Release Soc. 311-312, 170–189. doi:10.1016/j.jconrel.2019.08.032
Shavel, A., Gaponik, N., and Eychmüller, A. (2004). Efficient UV-blue photoluminescing thiol-stabilized water-soluble alloyed ZnSe (S) nanocrystals. J. Phys. Chem. B 108, 5905–5908. doi:10.1021/jp037941t
Shen, N., Yan, F., Pang, J., Gao, Z., Al-Kali, A., Haynes, C. L., et al. (2018). HDL-AuNPs-BMS nanoparticle conjugates as molecularly targeted therapy for leukemia. ACS Appl. Mater. Interfaces 10, 14454–14462. doi:10.1021/acsami.8b01696
Shi, M., Liu, Y., Xu, M., Yang, H., Wu, C., and Miyoshi, H. (2011). Core/shell Fe3O4 @SiO2 nanoparticles modified with PAH as a vector for EGFP plasmid DNA delivery into HeLa cells. Macromol. Biosci. 11, 1563–1569. doi:10.1002/mabi.201100150
Shim, B. S., Chen, W., Doty, C., Xu, C., and Kotov, N. A. (2008). Smart electronic yarns and wearable fabrics for human biomonitoring made by carbon nanotube coating with polyelectrolytes. Nano Lett. 8, 4151–4157. doi:10.1021/nl801495p
Sinani, V. A., Gheith, M. K., Yaroslavov, A. A., Rakhnyanskaya, A. A., Sun, K., Mamedov, A. A., et al. (2005). Aqueous dispersions of single-wall and multiwall carbon nanotubes with designed amphiphilic polycations. J. Am. Chem. Soc. 127, 3463–3472. doi:10.1021/ja045670+
Singh, P., Srivastava, S., and Singh, S. K. (2019). Nanosilica: Recent progress in synthesis, functionalization, biocompatibility, and biomedical applications. ACS biomaterials Sci. Eng. 5, 4882–4898. doi:10.1021/acsbiomaterials.9b00464
Skirtach, A. G., Antipov, A. A., Shchukin, D. G., and Sukhorukov, G. B. (2004). Remote activation of capsules containing Ag nanoparticles and IR dye by laser light. Langmuir 20, 6988–6992. doi:10.1021/la048873k
Skirtach, A. G., Dejugnat, C., Braun, D., Susha, A. S., Rogach, A. L., Parak, W. J., et al. (2005). The role of metal nanoparticles in remote release of encapsulated materials. Nano Lett. 5, 1371–1377. doi:10.1021/nl050693n
Skirtach, A. G., Volodkin, D. V., and Möhwald, H. (2010). Bio-interfaces—interaction of PLL/HA thick films with nanoparticles and microcapsules. ChemPhysChem 11, 822–829. doi:10.1002/cphc.200900676
Slyusarenko, N., Gerasimova, M., Plotnikov, A., Gaponik, N., and Slyusareva, E. (2019). Photoluminescence properties of self-assembled chitosan-based composites containing semiconductor nanocrystals. Phys. Chem. Chem. Phys. 21, 4831–4838. doi:10.1039/c8cp07051b
Smith, B. R., Inglis, D. W., Sandnes, B., Rabeau, J. R., Zvyagin, A. V., Gruber, D., et al. (2009). Five-nanometer diamond with luminescent nitrogen-vacancy defect centers. small 5, 1649–1653. doi:10.1002/smll.200801802
Song, C., Zhang, J., Jiang, X., Gan, H., Zhu, Y., Peng, Q., et al. (2021). SPR/SERS dual-mode plasmonic biosensor via catalytic hairpin assembly-induced AuNP network. Biosens. Bioelectron. 190, 113376. doi:10.1016/j.bios.2021.113376
Song, X. J., Gong, H., Yin, S. N., Cheng, L., Wang, C., Li, Z. W., et al. (2014). Ultra- small iron oxide doped polypyrrole nanoparticles for in vivo multimodal imaging guided photothermal therapy. Adv. Funct. Mater. 24, 1194–1201. doi:10.1002/adfm.201302463
Stavitskaya, A., Rubtsova, M., Glotov, A., Vinokurov, V., Vutolkina, A., Fakhrullin, R., et al. (2022). Architectural design of core–shell nanotube systems based on aluminosilicate clay. Nanoscale Adv. 4, 2823–2835. doi:10.1039/d2na00163b
Sukhanova, A., Bozrova, S., Gerasimovich, E., Baryshnikova, M., Sokolova, Z., Samokhvalov, P., et al. (2022). Dependence of quantum dot toxicity in vitro on their size, chemical composition, and surface charge. Nanomaterials 12, 2734. doi:10.3390/nano12162734
Sun, A., Huang, X., Jiao, Y., Wang, X., and Wen, J. (2021a). Construction of biological factor-coated stent and its effect on promoting endothelialization. Mater. Sci. Eng. C 122, 111943. doi:10.1016/j.msec.2021.111943
Sun, B., Chang, R., Cao, S., Yuan, C., Zhao, L., Yang, H., et al. (2020a). “Acid-activatable transmorphic peptide-based nanomaterials for photodynamic therapy,” Angewandte Chemie Int. Ed. Engl., 59, 20582–20588. doi:10.1002/anie.202008708
Sun, W., Luo, L., Feng, Y., Qiu, Y., Shi, C., Meng, S., et al. (2020b). Gadolinium–rose bengal coordination polymer nanodots for MR-/Fluorescence-Image-Guided radiation and photodynamic therapy. Adv. Mater. 32, 2000377. doi:10.1002/adma.202000377
Sun, W. S., Yang, J. X., Hou, M. F., Xie, S. W., Xiong, L. Q., Li, B., et al. (2021b). A nano “immune-guide” recruiting lymphocytes and modulating the ratio of macrophages from different origins to enhance cancer immunotherapy. Adv. Funct. Mater. 31 31, 2009116. doi:10.1002/adfm.202009116
Sun, W., Zhang, X., Jia, H.-R., Zhu, Y.-X., Guo, Y., Gao, G., et al. (2019). Water-dispersible candle soot-derived carbon nano-onion clusters for imaging-guided photothermal cancer therapy. Small (Weinheim Der Bergstrasse, Ger. 15, e1804575. doi:10.1002/smll.201804575
Tambunlertchai, S., Srisang, S., and Nasongkla, N. (2017). Erratum to: Development of antimicrobial coating by layer-by-layer dip coating of chlorhexidine-loaded micelles. Medicine 28, 118. doi:10.1007/s10856-017-5929-0
Tan, D., Yuan, P., Liu, D., and Du, P. (2016). “Surface modifications of halloysite,” in Nanosized tubular clay minerals (Guangzhou: Halloysite and Imogolite), 167–201.
Tan, M., Del Rosal, B., Zhang, Y., Rodríguez, E. M., Hu, J., Zhou, Z., et al. (2018). Rare-earth-doped fluoride nanoparticles with engineered long luminescence lifetime for time-gated in vivo optical imaging in the second biological window. Nanoscale 10, 17771–17780. doi:10.1039/c8nr02382d
Tarakanchikova, Y., Muslimov, A., Sergeev, I., Lepik, K., Yolshin, N., Goncharenko, A., et al. (2020). A highly efficient and safe gene delivery platform based on polyelectrolyte core-shell nanoparticles for hard-to-transfect clinically relevant cell types. J. Mater. Chem. B 8, 9576–9588. doi:10.1039/d0tb01359e
Taubert, A., Leroux, F., Rabu, P., and De Zea Bermudez, V. (2019). „Advanced hybrid nanomaterials”. Beilstein-Institut).
Teepakakorn, A., and Ogawa, M. (2021). Self-healing polymer–clay hybrids by facile complexation of a waterborne polymer with a clay. Mater. Adv. 2, 3770–3776. doi:10.1039/d1ma00099c
Tetsuka, H., Ebina, T., Nanjo, H., and Mizukami, F. (2007). Highly transparent flexible clay films modified with organic polymer: Structural characterization and intercalation properties. J. Mater. Chem. 17, 3545–3550. doi:10.1039/b705063a
Thaxton, C. S., Georganopoulou, D. G., and Mirkin, C. A. (2006). Gold nanoparticle probes for the detection of nucleic acid targets. Clin. Chim. Acta 363, 120–126. doi:10.1016/j.cccn.2005.05.042
Tomai, T., Tang, L. Y., Yoko, A., Omura, Y., Seong, G., and Adschiri, T. (2021). Facile regeneration strategy for facet-controlled nanocatalysts via the dissolution-reprecipitation process promoted by an organic modifier. Chem. Mater. 33, 7780–7784. doi:10.1021/acs.chemmater.1c02145
Ung, T., Liz-Marzán, L. M., and Mulvaney, P. (2001). Optical properties of thin films of Au@ SiO2 particles. J. Phys. Chem. B 105, 3441–3452. doi:10.1021/jp003500n
Uskoković, V., and Desai, T. A. (2014). In vitro analysis of nanoparticulate hydroxyapatite/chitosan composites as potential drug delivery platforms for the sustained release of antibiotics in the treatment of osteomyelitis. J. Pharm. Sci. 103, 567–579. doi:10.1002/jps.23824
Venditto, V. J., and Szoka, F. C. (2013). Cancer nanomedicines: So many papers and so few drugs!. Adv. drug Deliv. Rev. 65, 80–88. doi:10.1016/j.addr.2012.09.038
Vita, F., Innocenti, C., Secchi, A., Albertini, F., Grillo, V., Fiore, A., et al. (2018). Colloidal Au/iron oxide nanocrystal heterostructures: Magnetic, plasmonic and magnetic hyperthermia properties. J. Mater. Chem. C 6, 12329–12340. doi:10.1039/c8tc01788c
Volodkin, D., Delcea, M., Mohwald, H., and Skirtach, A. (2009). Remote near-IR light activation of a hyaluronic acid/poly (l-lysine) multilayered film and film-entrapped microcapsules. ACS Appl. Mater. interfaces 1, 1705–1710. doi:10.1021/am900269c
Volodkin, D., Skirtach, A., and Möhwald, H. (2012). Bioapplications of light-sensitive polymer films and capsules assembled using the layer-by-layer technique. Polym. Int. 61, 673–679. doi:10.1002/pi.4182
Wan, J. Y., Xie, J., Kong, X., Liu, Z., Liu, K., Shi, F. F., et al. (2019). Ultrathin, flexible, solid polymer composite electrolyte enabled with aligned nanoporous host for lithium batteries. Nat. Nanotechnol. 14, 705–711. doi:10.1038/s41565-019-0465-3
Wang, B., Chen, K., Jiang, S., Reincke, F., Tong, W., Wang, D., et al. (2006). Chitosan-mediated synthesis of gold nanoparticles on patterned poly (dimethylsiloxane) surfaces. Biomacromolecules 7, 1203–1209. doi:10.1021/bm060030f
Wang, C., Ying, C., Shang, J., Karcher, S. E., Mccloy, J., Liu, J., et al. (2022). A bioinspired coating for stabilizing Li metal batteries. ACS Appl. Mater. Interfaces 14, 43886–43896. doi:10.1021/acsami.2c10667
Wang, D., Wu, H., Zhou, J., Xu, P., Wang, C., Shi, R., et al. (2018a). In situ one-pot synthesis of MOF–polydopamine hybrid nanogels with enhanced photothermal effect for targeted cancer therapy. Adv. Sci. 5, 1800287. doi:10.1002/advs.201800287
Wang, H., Chen, Q., and Zhou, S. (2018b). Carbon-based hybrid nanogels: A synergistic nanoplatform for combined biosensing, bioimaging, and responsive drug delivery. Chem. Soc. Rev. 47, 4198–4232. doi:10.1039/c7cs00399d
Wang, X., Zhang, M., Zhang, L., Li, L., Li, S., Wang, C., et al. (2017). Designed synthesis of lipid-coated polyacrylic acid/calcium phosphate nanoparticles as dual pH-responsive drug-delivery vehicles for cancer chemotherapy. Chem. (Weinheim Der Bergstrasse, Ger. 23, 6586–6595. doi:10.1002/chem.201700060
Wang, Y., and Wei, S. (2022). Green fabrication of bioactive silver nanoparticles using Mentha pulegium extract under alkaline: An enhanced anticancer activity. ACS Omega 7, 1494–1504. doi:10.1021/acsomega.1c06267
Wen, C., Broholm, M. M., Dong, J., Uthuppu, B., Jakobsen, M. H., and Fjordbøge, A. S. (2020). Transport of citrate and polymer coated gold nanoparticles (AuNPs) in porous media: Effect of surface property and Darcy velocity. J. Environ. Sci. (China) 92, 235–244. doi:10.1016/j.jes.2020.02.026
Wubneh, A., Tsekoura, E. K., Ayranci, C., and Uludag, H. (2018). Current state of fabrication technologies and materials for bone tissue engineering. Acta biomater. 80, 1–30. doi:10.1016/j.actbio.2018.09.031
Xavier, J. R. (2021). Electrochemical and dynamic mechanical studies of newly synthesized polyurethane/SiO2-Al2O3 mixed oxide nanocomposite coated steel immersed in 3.5% NaCl solution. Surfaces Interfaces 22, 100848. doi:10.1016/j.surfin.2020.100848
Xiao, H., Guo, Y., Li, B., Li, X., Wang, Y., Han, S., et al. (2020). M2-Like tumor-associated macrophage-targeted codelivery of STAT6 inhibitor and IKKβ siRNA induces M2-to-M1 repolarization for cancer immunotherapy with low immune side effects. ACS Central Sci. 6, 1208–1222. doi:10.1021/acscentsci.9b01235
Xie, L., Tong, W., Yu, D., Xu, J., Li, J., and Gao, C. (2012). Bovine serum albumin nanoparticles modified with multilayers and aptamers for pH-responsive and targeted anti-cancer drug delivery. J. Mater. Chem. 22, 6053–6060. doi:10.1039/c2jm16831f
Xie, P. G., Ling, H. Q., Pang, M., He, L., Zhuang, Z. Y., Zhang, G. L., et al. (2022). Umbilical cord mesenchymal stem cells promoting spinal cord injury repair visually monitored by AIE-tat nanoparticles (adv. Therap. 12/2022). Adv. Ther. 5, 2270028. doi:10.1002/adtp.202270028
Xiong, R., Raemdonck, K., Peynshaert, K., Lentacker, I., De Cock, I., Demeester, J., et al. (2014). Comparison of gold nanoparticle mediated photoporation: Vapor nanobubbles outperform direct heating for delivering macromolecules in live cells. ACS Nano 8, 6288–6296. doi:10.1021/nn5017742
Xu, B., Li, A., Wang, R., Zhang, J., Ding, Y., Pan, D., et al. (2021). Elastic janus film for wound dressings: Unidirectional biofluid transport and effectively promoting wound healing. Adv. Funct. Mater. 31, 2105265. doi:10.1002/adfm.202105265
Xu, M., Zhu, J., Wang, F., Xiong, Y., Wu, Y., Wang, Q., et al. (2016). Improved in vitro and in vivo biocompatibility of graphene oxide through surface modification: Poly(acrylic acid)-functionalization is superior to PEGylation. ACS Nano 10, 3267–3281. doi:10.1021/acsnano.6b00539
Xu, Z., Wang, S., Li, Y., Wang, M., Shi, P., and Huang, X. (2014). Covalent functionalization of graphene oxide with biocompatible poly(ethylene glycol) for delivery of paclitaxel. ACS Appl. Mater. Interfaces 6, 17268–17276. doi:10.1021/am505308f
Yan, L., Wang, Y., Li, J., Kalytchuk, S., Susha, A. S., Kershaw, S. V., et al. (2014). Highly luminescent covalently bonded layered double hydroxide–fluorescent dye nanohybrids. J. Mater. Chem. C 2, 4490–4494. doi:10.1039/c3tc32483d
Yang, C., Mi, X., Su, H., Yang, J., Gu, Y., Zhang, L., et al. (2019). GE11-PDA-Pt@ USPIOs nano-formulation for relief of tumor hypoxia and MRI/PAI-guided tumor radio-chemotherapy. Biomaterials Sci. 7, 2076–2090. doi:10.1039/c8bm01492b
Yang, R., Zheng, Y., Zhang, Y., Li, G., Xu, Y., Zhang, Y., et al. (2022). Bipolar metal flexible electrospun fibrous membrane based on metal-organic framework for gradient healing of tendon-to-bone interface regeneration. Adv. Healthc. Mater. 11, 2200072. doi:10.1002/adhm.202200072
Yang, Y., Guo, L., Wang, Z., Liu, P., Liu, X., Ding, J., et al. (2021a). Targeted silver nanoparticles for rheumatoid arthritis therapy via macrophage apoptosis and Re-polarization. Biomaterials 264, 120390. doi:10.1016/j.biomaterials.2020.120390
Yang, Z., Tu, Q., Zhu, Y., Luo, R., Li, X., Xie, Y., et al. (2012). Mussel-inspired coating of polydopamine directs endothelial and smooth muscle cell fate for re-endothelialization of vascular devices. Adv. Healthc. Mater. 1, 548–559. doi:10.1002/adhm.201200073
Yang, Z., Xi, Y., Bai, J., Jiang, Z., Wang, S., Zhang, H., et al. (2021b). Covalent grafting of hyperbranched poly-L-lysine on Ti-based implants achieves dual functions of antibacteria and promoted osteointegration in vivo. Biomaterials 269, 120534. doi:10.1016/j.biomaterials.2020.120534
Yashchenok, A. M., Borisova, D., Parakhonskiy, B. V., Masic, A., Pinchasik, B., Möhwald, H., et al. (2012). Nanoplasmonic smooth silica versus porous calcium carbonate bead biosensors for detection of biomarkers. Ann. Phys. 524, 723–732. doi:10.1002/andp.201200158
Yu, P., Wang, L., Sun, F., Xie, Y., Liu, X., Ma, J., et al. (2019). Co Nanoislands rooted on Co–N–C nanosheets as efficient oxygen electrocatalyst for Zn–air batteries. Adv. Mater. 31, 1901666. doi:10.1002/adma.201901666
Zaheer, A., Murshed, M., De Grand, A. M., Morgan, T. G., Karsenty, G., and Frangioni, J. V. (2006). Optical imaging of hydroxyapatite in the calcified vasculature of transgenic animals. Arteriosclerosis, thrombosis, Vasc. Biol. 26, 1132–1136. doi:10.1161/01.atv.0000210016.89991.2a
Zhang, D., Peng, F., and Liu, X. (2021a). Protection of magnesium alloys: From physical barrier coating to smart self-healing coating. J. Alloys Compd. 853, 157010. doi:10.1016/j.jallcom.2020.157010
Zhang, G., Yang, Z., Lu, W., Zhang, R., Huang, Q., Tian, M., et al. (2009). Influence of anchoring ligands and particle size on the colloidal stability and in vivo biodistribution of polyethylene glycol-coated gold nanoparticles in tumor-xenografted mice. Biomaterials 30, 1928–1936. doi:10.1016/j.biomaterials.2008.12.038
Zhang, L., Bai, X., Tian, H., Zhong, L., Ma, C., Zhou, Y., et al. (2012a). Synthesis of antibacterial film CTS/PVP/TiO2/Ag for drinking water system. Carbohydr. Polym. 89, 1060–1066. doi:10.1016/j.carbpol.2012.03.063
Zhang, Q., Li, N., Goebl, J., Lu, Z., and Yin, Y. (2011). A systematic study of the synthesis of silver nanoplates: Is citrate a "magic" reagent? J. Am. Chem. Soc. 133, 18931–18939. doi:10.1021/ja2080345
Zhang, Q., Shen, C., Zhao, N., and Xu, F. J. (2017). Redox-responsive and drug-embedded silica nanoparticles with unique self-destruction features for efficient gene/drug codelivery. Adv. Funct. Mater. 27, 1606229. doi:10.1002/adfm.201606229
Zhang, X., Servos, M. R., and Liu, J. (2012b). Ultrahigh nanoparticle stability against salt, pH, and solvent with retained surface accessibility via depletion stabilization. J. Am. Chem. Soc. 134, 9910–9913. doi:10.1021/ja303787e
Zhang, Z., Jia, J., Lai, Y., Ma, Y., Weng, J., and Sun, L. (2010). Conjugating folic acid to gold nanoparticles through glutathione for targeting and detecting cancer cells. Bioorg. Med. Chem. 18, 5528–5534. doi:10.1016/j.bmc.2010.06.045
Zhang, Z., Xiao, X., Zhou, Y., Huang, L., Wang, Y., Rong, Q., et al. (2021b). Bioinspired graphene oxide membranes with pH-responsive nanochannels for high-performance nanofiltration. ACS Nano 15, 13178–13187. doi:10.1021/acsnano.1c02719
Zhao, W., Wei, J.-S., Zhang, P., Chen, J., Kong, J.-L., Sun, L.-H., et al. (2017). Self-Assembled ZnO nanoparticle capsules for carrying and delivering isotretinoin to cancer cells. ACS Appl. Mater. interfaces 9, 18474–18481. doi:10.1021/acsami.7b02542
Zheludkevich, M. L., Shchukin, D. G., Yasakau, K. A., Möhwald, H., and Ferreira, M. G. S. (2007). Anticorrosion coatings with self-healing effect based on nanocontainers impregnated with corrosion inhibitor. Chem. Mater. 19, 402–411. doi:10.1021/cm062066k
Zheng, T., Perona Martínez, F., Storm, I. M., Rombouts, W., Sprakel, J., Schirhagl, R., et al. (2017). Recombinant protein polymers for colloidal stabilization and improvement of cellular uptake of diamond nanosensors. Anal. Chem. 89, 12812–12820. doi:10.1021/acs.analchem.7b03236
Zheng, Y. F., Gu, X. N., and Witte, F. (2014). Biodegradable metals. Mater. Sci. Eng. R Rep. 77, 1–34. doi:10.1016/j.mser.2014.01.001
Zhou, C.-H., Shen, Z.-F., Liu, L.-H., and Liu, S.-M. (2011). Preparation and functionality of clay-containing films. J. Mater. Chem. 21, 15132–15153. doi:10.1039/c1jm11479d
Zhou, Y., Chen, Z., Zhao, D., Li, D., He, C., and Chen, X. (2021). A pH-triggered self-unpacking capsule containing zwitterionic hydrogel-coated MOF nanoparticles for efficient oral exendin-4 delivery. Adv. Mater. Deerf. Beach, Fla.) 33, e2102044. doi:10.1002/adma.202102044
Zhu, A., Ali, S., Xu, Y., Ouyang, Q., and Chen, Q. (2021). A SERS aptasensor based on AuNPs functionalized PDMS film for selective and sensitive detection of Staphylococcus aureus. Biosens. Bioelectron. 172, 112806. doi:10.1016/j.bios.2020.112806
Zuaznabar-Gardona, J. C., and Fragoso, A. (2018). A wide-range solid state potentiometric pH sensor based on poly-dopamine coated carbon nano-onion electrodes. Sensors and actuators B:. Chemical 273, 664–671.
Glossary
α-CHC α-cyano-4-hydroxycinnamic acid
AHAPS N-(6-aminohexyl)-3-aminopropyltrimethoxy silane
ALP alkaline phosphatase
APTES 3-aminopropyltriethoxysilane
BPEI branched polyethylene-imine
CDxs Cyclodextrins
CHA catalytic hairpin assembly
CNO carbon nano-onion
CQDs carbon quantum dots
CTAB cetyltrimethylammonium bromide
CTS Chitosan
DDA dodecyl amine
DMAP 4-(dimethylamino)pyridine
GO graphene oxide
GLU glutaraldehyde
Ha hyaluronic acid
HA hydroxyapatite
HAD hexadecyl amine
HDL high-density lipoprotein
HDTMA Hexadecyl trimethy-lammonium bromide
MCT-β-CD Monochlorotriazinyl-β-cyclodextrin
MOF Organic Framework
MWCNTs multi-walled carbon nanotubes
PAA polyacrylic acid
PAH poly(allylamine hydrochloride)
PC Phosphorylcholine
PCL poly(caprolactone)
PDA polydopamine
PDDA poly (diallyl dimethyl ammonium chloride)
PDMS polydimethylsiloxane
PE polyethene
PEA poly(ester-amide)
PDMS polydimethylsiloxane
PEEK polyetheretherketone
PEG poly(ethylene glycol)
PEI polyethyleneimine
PEO poly ethylene oxide
PEVA Polyethylene-co-vinyl acetate
PLA Polylactic acid
PLL poly(L-lysine)
PMDA Pyromellitic dianhydride
PMMA poly(methyl methacrylate)
PMPC polymethacryloyloxyethyl phosphorylcholine
POM Polyoxymethylene
PSS poly(sodium styrenesulfonate)
PU Polyurethane
PUR Polyurethane
PVP polyvinyl pyrrolidone
PVDF poly (vinylidene fluoride)
SPA polyacrylate
SS stainless steels
TOPO trioctylphosphine oxide
Keywords: hybrid materials, organics, inorganics, nanoparticles, colloids, flat surfaces, modifications and applications
Citation: Song J, Vikulina AS, Parakhonskiy BV and Skirtach AG (2023) Hierarchy of hybrid materials. Part-II: The place of organics-on-inorganics in it, their composition and applications. Front. Chem. 11:1078840. doi: 10.3389/fchem.2023.1078840
Received: 24 October 2022; Accepted: 05 January 2023;
Published: 25 January 2023.
Edited by:
Claudia Caltagirone, University of Cagliari, ItalyReviewed by:
Rajendra Kurapati, Indian Institute of Science Education and Research, Thiruvananthapuram, IndiaDario Pasini, University of Pavia, Italy
Copyright © 2023 Song, Vikulina, Parakhonskiy and Skirtach. This is an open-access article distributed under the terms of the Creative Commons Attribution License (CC BY). The use, distribution or reproduction in other forums is permitted, provided the original author(s) and the copyright owner(s) are credited and that the original publication in this journal is cited, in accordance with accepted academic practice. No use, distribution or reproduction is permitted which does not comply with these terms.
*Correspondence: Junnan Song, SnVubmFuLlNvbmdAVUdlbnQuYmU=; Bogdan V. Parakhonskiy, Ym9nZGFuLnBhcmFraG9uc2tpeUB1Z2VudC5iZQ==; Andre G. Skirtach, YW5kcmUuc2tpcnRhY2hAdWdlbnQuYmU=